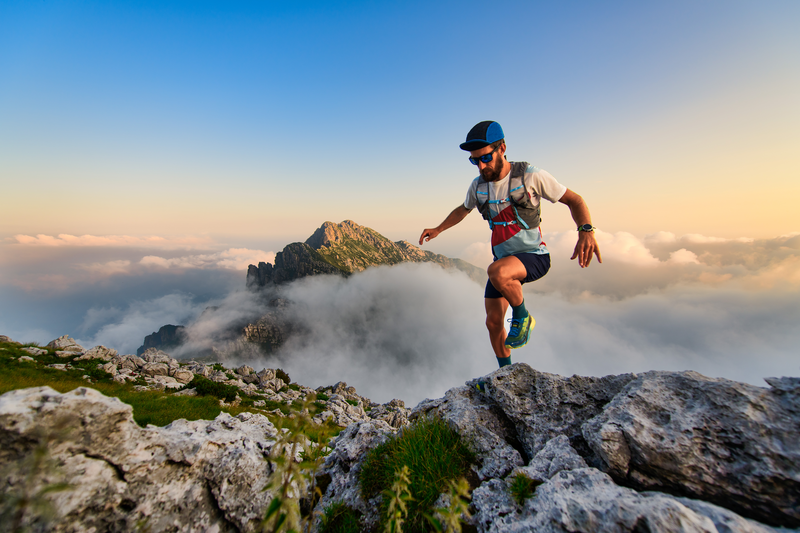
95% of researchers rate our articles as excellent or good
Learn more about the work of our research integrity team to safeguard the quality of each article we publish.
Find out more
ORIGINAL RESEARCH article
Front. Sustain. Food Syst. , 09 October 2020
Sec. Crop Biology and Sustainability
Volume 4 - 2020 | https://doi.org/10.3389/fsufs.2020.560479
This article is part of the Research Topic Intercropping Systems in Sustainable Agriculture View all 17 articles
Forage cover crops are gaining in popularity on the Canadian prairies, where multi-species crop mixtures are grown for soil and ecosystem enhancing benefits, but also harvested for forage. As the use of these forage mixtures increases, more knowledge is needed to understand the impact these mixtures have on forage production systems. The objective of this study was to determine the effects of increasing plant species diversity on forage productivity, soil chemistry, and soil microbial communities. Field trials were conducted in 2016 and 2017 at two separate locations in the Canadian Prairie region that included four treatments: (1) oat monoculture, (2) three spp. mixture (one grass, one legume, one brassica), (3) six spp. mixture (two grasses, two legumes, two brassicas), and (4) nine spp. mixture (three grasses, three legumes, three brassicas). Soil and plant samples were collected at the mid and late growing season to assess soil chemistry, plant biomass and composition, forage nutrition and quality, and soil bacterial and fungal communities. Overall, the oat monoculture had the highest biomass productivity, while the nine spp. mixture produced the lowest biomass among the treatments. All three mixtures had a better nutritional profile [i.e., greater concentrations of Ca, Cu, Fe, total Kjeldahl nitrogen (TKN), total phosphorus (TP) and total potassium (TK), and lower concentrations of acid detergent fiber (ADF) and neutral detergent fiber (NDF)] compared to the monoculture. Differences in forage nutrition were particularly heightened at the end of the growing season. Soil chemical properties did not differ greatly among the treatments with the exception of higher levels of soil nitrate availability in the mixtures compared to the monoculture. Early indicators of a shift in soil microbial diversity and fungal community composition, and an increased abundance of fungal pathotrophs in the mixtures compared to the oat monoculture, was observed at one of the field sites. This study indicates that increasing plant species diversity does not always lead to an increase in biomass production or significant changes or improvements in soil microbial communities. However, the inclusion of multiple plant species can improve the quality and nutritive value of forages over a monoculture forage crop.
Forage cover crops can offer nutritional resources to support grazing livestock while also leaving plant material behind to serve as a mulch or soil amendment. Forage cover crops have been utilized worldwide in multiple cropping systems, especially on organic farms to optimize environmental resource availability and enhance sustainable agricultural practices (Bergtold et al., 2017). Annual forage cover crops can be monocultures of grasses, legumes, or brassicas, or mixtures of these crops, and can be included in rotation with other cash crops. Currently, forage cover crop designs that include multi-species mixtures are becoming increasingly popular for use in forage production due to their perceived benefits to productivity and soil properties. There have been numerous studies showing that increasing cover crop diversity can lead to optimized environmental resources use, increased soil nutrients, restricted disease and pest pressure, enhanced weed suppression, reduced soil erosion and water runoff, all leading to yield increases (Anil et al., 1998; Tilman et al., 2001; Hartwig and Ammon, 2002; Lithourgidis et al., 2011; Bonin and Tracy, 2012). However, information regarding the selection and diversity of suitable crop mixtures is still very limited.
It is important for forage cover crops to contain sufficient amounts of nutrients to support livestock growth, which can lead to reduced grain feeding and purchased supplemental feeds, and result in economic benefits to farmers (Gardner et al., 1991). Mixed forage cover crops can yield better quality forage as the use of plant species from various functional groups can improve the nutritive value of forage and reduce the need for additional mineral supplementation. More specifically, the inclusion of legumes in cover crop mixtures has been shown to produce higher levels of important macronutrients and some micronutrients to support livestock (Pirhofer-Walzl et al., 2011). Choosing effective cover crop mixtures is also critical to maximize the ecological benefits that cover crops can bring into agricultural systems (Wittwer et al., 2017). For example, including legumes into crop mixtures has been shown to increase soil nutrient (nitrogen, phosphorus and potassium) budgets (Malézieux et al., 2009; Dahmardeh et al., 2010). Including other crops such as grasses or brassicas, which have a high capacity for N and C storage, can prevent nutrient loss, water pollution and greenhouse gas emissions from agro-ecosystems (Dabney et al., 2001; Blanco-Canqui et al., 2015). In addition, increasing species richness within plant functional groups has shown positive impacts on forage biomass, which demonstrates that species within the same functional group may not be redundant in their functions (Marquard et al., 2009). However, there may be a limit to the number of plant species in a mixture that are actually beneficial to forage productivity, and some high species mixtures may produce less biomass than a mixture with fewer species (Schellenberg et al., 2012). The positive effects of diverse cover crops may not always be realized by farmers as many factors can compromise these benefits, such as ineffective or incompatible crop selection, soil types, climate conditions, fertilization, and tillage strategies (Gabriel and Quemada, 2011; Dorn et al., 2015; Wittwer et al., 2017).
Despite a considerable amount of cover crop research, there is a limited understanding of forage cover crop impacts on soil microbial communities, which are integral components of functional cropping systems. Previous studies have shown that increasing plant species richness can support more diverse and functional soil microbial communities compared to monocultures in both annual and perennial systems (Zak et al., 2003; Qiao et al., 2012; Lange et al., 2015; Finney et al., 2017). In agroecosystems, the inclusion of specific crop species and plant functional groups in cover crops appear to be important regulators of microbial community composition and soil biological activity (Finney et al., 2017; Venter et al., 2017; Cloutier et al., 2020). Due to the critical role that soil microbial communities play in various agroecosystem services it is important to better understand the impacts of multi-species forage cover crops on soil microbial communities.
To address the uncertainties on how to optimize mixed forage cover crops and to systematically evaluate a selection of mixed forage cover crops and their benefits, we hypothesized that forage biomass productivity, forage nutrition, and soil nutrients would increase with increased cover crop diversity. We also hypothesized that increased cover crop diversity would restructure soil microbial communities and increase bacterial and fungal diversity. To examine these hypotheses, we tested the following objectives: (1) determine the effects of increased cover crop diversity on overall forage productivity and nutritive value; and (2) examine changes to soil chemistry and soil microbial communities due to increased cover crop diversity.
Experimental field trials were conducted at two different sites in the Canadian prairie region. A small plot (0.004 ha plot size) field trial was located on a Brown Chernozem soil zone at the Swift Current Research and Development Center, southeast of Swift Current, Saskatchewan, Canada (50°16′N lat., 107°43′W long.). This field was seeded to wheat in 2014 and chemical fallowed in 2015. A larger plot (~0.16 ha plot size) field trial was located on a loamy Black Chernozem soil zone at the Brookdale Research Farm (managed by Manitoba Beef and Forage Initiatives Inc.), which was located 18 km north of Brandon, Manitoba, Canada (50°05′N lat., 99°92′W long.). The field at the Brookdale research farm was seeded to an oats/peas green feed mixture in 2014, and left fallow in 2015. Both sites were tilled and harrow packed prior to seeding.
To test the effect of crop species diversity, the experimental design included four different cropping mixture treatments: (i) monoculture, (ii) three spp. mixture, (iii) six spp. mixture, and (iv) nine spp. mixture. The experiment was conducted for two consecutive growing seasons in 2016 and 2017, with the same cropping treatments grown on the same plots each year. The monoculture was seeded to oats (Avena sativa “Common No. 1”). The three spp. mixture consisted of one grass species (oats), one legume species (forage peas, Pisum sativum “CDC Leroy”), and one brassica species [Graza forage radish (Raphanus x Brassica, Stewart and Moorhead, 2004)]. The six spp. mixture consisted of two grasses (oats and Italian ryegrass, Festuca perrenis), two legumes [forage peas and hairy vetch (Vicia Villosa, “Common No. 1”)], and two brassica species [Graza and Winfred forage brassica (Brassica napus ssp.)]. The nine spp. mixture consisted of these same six crops, with one additional representative from each functional group: sorghum (Sorghum bicolor), crimson clover (Trifolium incarnatum), Hunter forage brassica (Brassica sp.). In 2017, Graza forage radish was replaced by Groundhog forage radish (Raphanus sativus sp. “Common No. 1”). All treatments were replicated four times in a randomized complete block design for a total of 16 plots per site. Plots at the Swift Current site were 2 by 10 m with crop row spacing of 30 cm. Plots at the Brookdale site were 12 by 120 m with a crop row spacing of 15 cm. Seeding rates at each location were determined based on recommended plants m−2 for these regions (i.e., ~240 grass and 150 brassica and legume plants m−2). The number of live seeds per crop species in the mixtures was calculated as a proportion of the total mix (e.g., three spp. mixture: oats = 1/3*240 live seeds m−2, forage peas = 1/3*150 live seeds m−2, forage radish = 1/3*150 live seeds m−2). A seeding depth of 3 cm was selected as an intermediate depth suitable for all species. Plots were seeded on May 19, 2016 and May 25, 2017 at the Swift Current site, and June 3, 2016 and June 10, 2017 at the Brookdale site.
Soil samples were collected at two different dates during the growing seasons in 2016 and 2017: (i) mid growing season in late July and (ii) late growing season in late August or early September. Soil cores, 15 cm deep and 2.5 cm diameter, were collected from six random locations within each plot. Soil cores from each plot were bulked together and homogenized in the field immediately after sampling to form one composite soil sample per plot. A 10 g soil sub-sample was immediately flash frozen in a liquid nitrogen cryo-shipper for molecular analyses. The remaining soil was stored in a cooler for transportation and then stored at 4°C for up to 1 week until further processing. Soil samples were sieved through a 2 mm sieve, and a subsample was used to determine soil moisture content (gravimetric). The remaining soil was air-dried and ground for chemical analyses. Plant biomass and plant nutrient samples were collected at the same dates and sampling points as the soil cores. Six 1 m rows of crop were cut and bulked together within each plot. Plant tissues were oven-dried and weighed for plant biomass.
Soil organic carbon was determined using the dry combustion method (after acidification with HCl) using an Elementar vario MICRO cube elemental analyzer (Schumacher, 2002). Soil nitrate nitrogen (NO3-N) and phosphate phosphorus (PO4-P) were determined using sodium bicarbonate extractions followed by colorimetric analysis using a Technicon Autoanalyzer (Harm et al., 1973; Gentry and Willis, 1988). Soil sulfate sulfur (SO4-S) was determined using calcium chloride extractions followed by colorimetric analysis using a Technicon Autoanalyzer (Harm et al., 1973). Soil pH was measured in water saturation paste (Hendershot et al., 2008) and paste extracts (Miller and Curtin, 2008).
Dried plant samples were ground using a Wiley laboratory mill with a 1 mm screen. Tissues were analyzed for Ca, Cu, and Fe content using the digestion method (U. S. Environmental Protection Agency, 1996) and analysis was performed by ICP-OES (inductive coupled plasma-optical emission spectroscopy) on a Fisher Scientific iCAP6300 Duo. Plant total Kjeldahl nitrogen, total phosphorus (TP), and total potassium (TK) were determined using the Kjeldahl digest method. Plant acid detergent fiber (ADF) was measured using the procedure of Goering and Van Soest (1970) and the neutral detergent fiber (NDF) was determined using the ANKOM200 fiber analyser (Model 200; ANKOM; Fairport, New York).
DNA was extracted from 0.25 g of soil in duplicate for each sample using the DNeasy PowerSoil kit (Qiagen, Hilden, Germany) in batches of 12 samples using an automated system (QIAcube, Qiagen). Extracted DNA was quantified using a Qubit dsDNA BR Assay Kit (Thermo Fisher, Waltham, MA). For amplicon sequencing, DNA extracts were shipped on dry ice to the Genome Quebec Innovation Center (Montreal, Canada) for amplicon library preparation and Illumina MiSeq sequencing. The bacterial 16S rRNA genes were sequenced using primers 515-F and 806-R (Caporaso et al., 2012). The fungal ITS1 region was sequenced using primers 5.8S-Fun and ITS4-Fun (Taylor et al., 2016) for 2016 samples and ITS1F and 58A2R (Martin and Rygiewicz, 2005) for 2017 samples. For full description of amplicon library preparation and Illumina Miseq sequencing see Delavaux et al. (2020). The raw amplicon sequencing dataset is available in the NCBI Sequence Read Archive under BioProject ID: PRJNA656804.
Raw paired reads were processed using the UPARSE pipeline and USEARCH v.9 (Edgar, 2013). Paired reads were merged using the fastq_mergepairs command with a maximum of five (i.e., default) mismatches in the alignment. Merged reads were quality filtered using the command fastq_filter that discarded all reads that were <200 bp and those with expected errors > 1. Sequences were dereplicated and the command cluster_otus was used to perform operational taxonomic unit (OTU) clustering (based on 97% similarity) and chimera filtering. Taxonomic identity was assigned using the RDP classifier (Wang et al., 2007) and 16S rRNA training set (version 16) for bacteria/archaea and ITS UNITE database for fungi (Kõljalg et al., 2013) in R (v.3.6.1). Before all analyses, we filtered out all unmatching domains (including only archaea and bacteria in bacterial analyses; only fungi in fungal analyses). OTU tables for each analysis were filtered to include OTUs with a minimum of three sequences. Finally, OTU tables for each analysis were normalized to the lowest number of sequences in a sample (12,898 reads for bacteria, 7,035 reads for fungi in 2016, and 12,173 reads for fungi in 2017) using rrarefy function from the R package vegan (Oksanen et al., 2013).
All statistical analyses were performed in R Studio using R 3.6.1 (The R Foundation for Statistical Computing). All univariate analyses (i.e., forage biomass, forage nutrition, soil chemistry, and microbial α-diversity and relative abundance) were conducted using a mixed model fit to test the fixed factors cropping mixture, sampling date and their interaction, and block as a random factor using the packages Lme4 (Bates et al., 2014) and lmerTest (Kuznetsova et al., 2017). Multiple means comparisons were made using the function difflsmeans. The effect of cropping mixture and sampling date on the bacterial and fungal community composition was tested using Permanova (adonis function in R using Bray-Curtis dissimilarity with 5,000 permutations) and visualized using principle coordinate analyses (PCoA). Each eco-site year (two sites × 2 years = four total eco-site years) was analyzed separately for statistical analyses.
The cropping mixtures had a significant effect on crop biomass production in 2016 and 2017 at the Brookdale field trial. The oat monoculture had the highest biomass production in both years and was significantly higher than all the mixtures in 2016 (Figures 1A,B, Table 1). There was no difference in the biomass production between the three mixtures, but in 2017 the three spp. mixture produced significantly higher biomass than the nine spp. mixture. In both years, the biomass significantly increased in all treatments from the mid-part of the growing season until the late growing season (Table 1). Brassicas were the only plant functional group that was affected by the cropping mixtures (Table 1). This was significant in 2016 where we observed higher brassica biomass production in the six spp. and nine spp. mixtures compared to the three spp. mixture at the end of the growing season (Figure 1A). This was primarily due to the poor establishment and production of the Graza forage radish in 2016, which was present in the three, six, and nine spp. mixtures. This variety was replaced with Groundhog forage radish in 2017 and there were no longer any differences in brassica biomass production between the mixtures.
Figure 1. Crop biomass production at Brookdale in (A) 2016 and (B) 2017 and at Swift Current in (C) 2016 and (D) 2017. Mid and late-season crop biomass are presented separately for eco-site years that had a significant mixture by date interaction (i.e., Brookdale 2016 for brassica biomass and Swift Current 2017 for total biomass). Bars with different capital letters are significantly different (p < 0.05) for total biomass, and lowercase letters are significantly different for brassica (yellow portion of bars) biomass.
Table 1. ANOVA results of the effect of cropping mixtures (monoculture, three, six, and nine spp.) and date of sampling (mid or late growing season) on total aboveground crop biomass production and of each functional group (grasses, legumes, brassicas) at the Swift Current and Brookdale research sites in 2016 and 2017.
At the Swift Current field trial, we observed a significant effect of the cropping mixtures and a cropping mixture by sampling date interaction in 2016 (Figure 1C, Table 1). The oat monoculture produced the highest biomass at the mid point of the growing season, but did not exhibit any further growth for the remainder of the season. In contrast, the mixtures increased their biomass by over 50% between the mid and late season sampling dates. As a result, there was no significant difference between the monoculture and the three and six spp. mixtures at the late season sampling date (Figure 1C). Similar to the Brookdale site, the brassica biomass production at Swift Current was significantly higher in the six and nine spp. mixtures compared to the three spp. mixture due to the poor growing Graza forage radish (Figure 1C). In 2017, all plots produced very low crop biomass at the Swift Current site, and the six and nine spp. mixtures produced significantly lower crop biomass compared to the oat monoculture (Figure 1D). The lower biomass production in Swift Current and in Brookdale to a lesser extent is likely due to the very low levels of precipitation and soil moisture content in 2017 compared to 2016 (Supplementary Table 2).
The cropping mixtures had a significant effect on different measures of forage quality and nutrition at both field sites and years. Ca, Cu, TKN, and NDF were all significantly affected by the cropping mixtures in at least two of the four eco-site years (Table 2). Across all eco-site years the Ca, Cu and TKN were all higher and NDF lower in all three mixtures compared to the oat monoculture (Supplementary Table 1). Fe, TP, TK and ADF were significantly affected by the cropping mixtures at the Swift Current site in 2017. The three mixtures had higher concentrations of Fe, TP and TK and lower ADF compared to the oat monoculture. Sampling date was also a critical factor for forage quality and nutrition as TKN, TP, TK, ADF and NDF were all significantly affected and nutritional quality deteriorated at the later sampling date at all eco-site years (Table 2, Supplementary Table 1).
Table 2. ANOVA results of the effect of cropping mixtures (monoculture, three, six, and nine spp.) and date of sampling (mid or late growing season) on forage quality and nutrition at the Swift Current and Brookdale research sites in 2016 and 2017.
The cropping mixtures had a minor impact on the soil chemical properties at both sites. Nitrate (NO3-N) levels were significantly affected by the cropping mixtures in 2017 at the Brookdale site and in both years at the Swift Current site (Table 3). The only difference at the Brookdale site was an increase in soil nitrate levels under the six spp. mixture at the late sampling date compared to the other treatments (Supplementary Table 2). Nitrate levels were also strongly affected by sampling date at all four eco-site years. At the Swift Current site we observed higher levels of nitrate in the three spp. mixture in 2016 and in the nine spp. mixture in 2017. Phosphate (PO4-P) and organic carbon were unaffected by the cropping mixtures, but did significantly change between the sampling dates in 2017 at both sites. Soil pH and soil moisture were only significantly affected by the cropping mixtures at one of the four eco-site years (pH: Brookdale in 2017, soil moisture: Swift Current in 2017). For both years in Swift Current, soil moisture significantly decreased from the mid to late sampling period.
Table 3. ANOVA results of the effect of cropping mixtures (monoculture, three, six, and nine spp.) and date of sampling (mid or late growing season) on soil properties at the Swift Current and Brookdale research sites in 2016 and 2017.
The cropping mixtures and sampling date had no significant effect on bacterial or fungal alpha-diversity (richness and inverse Simpson's index) at the Brookdale site in either 2016 or 2017, except for a decrease in fungal diversity (inverse Simpson's index) in 2016 (Table 4, Figure 2). At the Swift Current site, fungal alpha-diversity was affected by the cropping mixtures in 2016, with the oat monoculture exhibiting a higher inverse Simpson's index compared to the three mixtures (Table 4, Figure 2). In 2017, the bacterial and fungal diversity were both significantly affected by the cropping mixtures and sampling date (Table 4). Bacterial richness was significantly higher in the three and six spp. mixtures compared to the oat monoculture in 2017 (Figure 2). We detected a significant cropping mixture by sampling date interaction for fungal diversity, which is explained by the higher inverse Simpson's index at the late sampling date compared to the mixtures, but no difference at the mid sampling point (Figure 2). The sampling date effect was due to a decrease in bacterial diversity (richness and inverse Simpson's index) and increase in fungal diversity (inverse Simpson's index) between the mid to late sampling dates (Figure 2).
Table 4. ANOVA results of the effect of cropping mixtures (monoculture, three, six, and nine spp.) and date of sampling (mid or late growing season) on bacterial and fungal α-diversity at the Swift Current and Brookdale research sites in 2016 and 2017.
Figure 2. Boxplots of the effect of cropping mixtures (monoculture, three, six, and nine spp.) and date of sampling (mid or late growing season) on bacterial (A,B) and fungal (C–F) α-diversity at the Swift Current and Brookdale research sites. Bars with different letters are significantly different (p < 0.05).
The cropping mixtures had very little impact on the composition and structure of the soil bacterial community at either field site. There was no significant effect on the composition of the total bacterial community (Table 5) or the composition of the top eight most abundant bacterial phyla (Supplementary Table 3) at either the Brookdale or Swift Current sites. In addition, the cropping mixtures had no effect on the relative abundance of these bacterial phyla except the Gemmatimonadetes and Verrucomicrobia at one of the eco-site years (i.e., Swift Current 2016; Supplementary Table 5). Sampling date was a significant factor explaining variation in the shift in bacterial communities between the mid to late sampling periods at all eco-site years except Brookdale in 2016 (Table 5). This effect is primarily explained by a shift in composition of taxa belonging to the Proteobacteria because it was the only phyla that had a significant sampling date effect at all sites (Supplementary Table 3) and it was one of the two most abundant phyla at all eco-site years (Supplementary Table 5).
Table 5. Permanova results of the effect of crop species mixture (monoculture, three, six, and nine spp.) and sampling date (mid or late growing season) on the bacterial and fungal community composition at the Brookdale and Swift Current research sites in 2016 and 2017.
The fungal community was not significantly affected by the cropping mixtures at three of the eco-site years (Table 5). However, the cropping mixtures did explain a significant proportion of the variation in the fungal community at the Swift Current site in 2016. The principle coordinate analysis (PCoA) revealed that the cropping mixture effect was the most evident at the late sampling date with the oat monoculture and nine spp. mixture being the most dissimilar (Figure 3A). Further analysis revealed that the variation between cropping mixtures at this sampling date was due to a shift in taxa belonging to the Ascomycota (Figure 3B; Supplementary Table 4). Although the total fungal community was not affected by the cropping mixtures at the Swift Current site in 2017, the composition of the Mortierellomycota were significantly affected (Supplementary Table 4). The cropping mixture effect was most evident at the late sampling date with the six spp. mixture being the most dissimilar from the other treatments (Figure 3C). Furthermore, when assessing the relative abundance of fungal taxa based on functional guilds, we found that the oat monoculture had a significantly lower abundance of pathotrophs compared to at least two of the mixtures at the Brookdale site in 2017 and Swift Current site in 2016 (Figure 3; Supplementary Table 7). Sampling date was a significant factor explaining a shift in the fungal community at three of the four eco-sites years (Table 5). This effect is due to a shift in the composition and relative abundance of taxa belonging to the Ascomycota and Basidiomycota to a lesser extent, between the mid to late sampling dates (Supplementary Tables 4, 6).
Figure 3. Principle coordinate analysis (PCoA) demonstrating the effects of cropping mixtures (monoculture, three, six, and nine spp.) and date of sampling (mid or late growing season) on the community composition of the (A) fungi, (B) ascomycota, and (C) mortierellomycota at the Swift Current research site in 2016 (A,B) and 2017 (C).
Figure 4. Boxplots of the effect of cropping mixtures (monoculture, three, six, and nine spp.) on the relative abundance (proportion) of fungal pathotrophs at the (A) Brookdale site in 2017 and (B) Swift Current site in 2016. Bars with different letters are significantly different (p < 0.05).
We found that the oat monoculture had significantly higher crop biomass than the diverse annual forage cover crops we tested at both experimental sites in 2016 and 2017, indicating that an increase in diversity does not necessarily lead to an increase in annual crop biomass. Similar studies have found that crop biomass was higher in monocultures and pure stands of a forage crop than in polycultures and cropping mixtures, and is suggested to be a result of plant competitiveness (Assefa and Ledin, 2001; Griffith et al., 2011; Sadeghpour et al., 2013). As oats are tall plants with rapid early-season growth, it could have caused interspecific competition for light and supressed the growth of the slower growing and shorter species of legumes and brassicas in the mixtures. Likewise, the growth of oats in the mixtures may have also been affected by competition from the other crops in the mixtures. In our study, the nine species cropping mixtures produced the lowest crop biomass at both experimental sites in 2016 and 2017, which could have been due to high interspecific competition in the mixtures that limited plant growth and thus reduced total forage biomass. Lithourgidis et al. (2011) also reported a reduced growth rate and lower dry biomass of cereals when intercropped with peas compared to cereals in monoculture due to interspecific competition. In addition, some of the individual crops had relatively low productivity in the mixtures (data not shown) which indicates that of the total amount of crop that was seeded, not all crops grew equally well. Therefore, selecting proper cover crop species to reduce interspecific competition in mixtures could be beneficial in increasing overall forage crop biomass.
Although the total biomass of annual forage cover crops did not increase with increasing crop diversity in this study, we did find that mixtures can have beneficial effects for improving forage nutrition. In general, significantly higher levels of Ca, Cu, Fe, TP, TK, and TKN in the mixtures were found compared to the oat monoculture, especially at the Swift Current site in 2017. These results indicate that using forage cover crop mixtures of grasses, legumes and brassicas can significantly increase the nutritive value of forage compared to a grass monoculture. There are several reasons why mixtures can have better nutritive values than monocultures, related to the specific functional groups and species selected. For example, legumes are known to be high in nitrogen, which is a direct measure of crude protein and a key factor in forage nutrition (e.g., Schultz and Stubbendieck, 1983). Brassicas are also high in nitrogen and various micronutrients such as calcium (de Ruiter et al., 2009). Other studies have indicated that crop mixtures are beneficial for increasing forage nutrition such as P, Mg, K, S, and Zn (Assefa and Ledin, 2001; Pirhofer-Walzl et al., 2011), which can be attributed to the ability of different plant functional groups to uptake different minerals (Zhang et al., 2017). Including legumes in forage crop mixtures can boost biological nitrogen fixation in the system, which can further increase other soil nutrient availability such as available P (Dahmardeh et al., 2010). Brassica crops are good soil N and C sinks which can also increase soil nutrient use efficiency, while reducing the runoff and soil water loss when applied in forage cover crop mixtures (Blanco-Canqui et al., 2015). In this study, the selection of cover crops from three different functional groups (grasses, legumes, and brassicas) allowed diversified plants species to uptake nutrients from the soil both temporally and at different rates.
In regards to forage digestibility, NDF content was much lower in mixed forage cover crops than the oat monoculture, with two of the four eco-site years having significantly lower NDF. Lower levels of NDF are generally preferred, as NDF will impact the animal's intake of dry matter and the time of rumination, thus the concentration of NDF is usually negatively related to energy uptake. It is well-known that the inclusion of legumes at higher ratios in grass-legume mixtures will exhibit lower NDF values compared to cereal monocultures (Caballero et al., 1995; Ghanbari-Bonjar and Lee, 2003). Similarly, lower levels of ADF are generally indicators of higher quality forage, and lower levels of ADF were found in the mixtures at the Swift Current site in 2017. As NDF and ADF are widely used as good indicators of forage quality, our results demonstrated that mixing legume and brassica cover crops with grasses can be a good way to increase forage digestibility compared to an oat monoculture.
Significant differences were detected in forage quality and nutrition between sampling dates, as we found forage nutrition decreased in the late-season samples at both experimental sites in 2016 and 2017. The significant increase in NDF content in late-season samples is attributed to changes in the morphological growth of plants as they mature. For example, Assefa and Ledin (2001) observed that as the leaf : stem ratio of oats decreased during maturation, the NDF content of forage mixtures increased. The overall improvement of forage quality in mixtures vs. the cereal monoculture was particularly evident in Swift Current during the very dry conditions of 2017, which might indicate that annual forage mixtures might be a useful crop management option to provide higher quality forage under stressful growing conditions. In addition, the improved forage quality in mixtures could also lead to greater potential market value and improve animal performance in beef, dairy, and sheep production systems (Buza et al., 2014; McKenzie et al., 2017; Fruet et al., 2019; Tufail et al., 2020).
Soil nitrate levels were significantly higher in the cropping mixtures than in the oat monoculture in 2017 at the Brookdale site and in both years at the Swift Current site. Adding nitrogen-fixing legume species to a cropping mixture can affect total N levels within the soil during the growing season, increasing the amount of available N in soil, which is beneficial for other non-legume crops applied in mixtures (Dybzinski et al., 2008; Malézieux et al., 2009; Dahmardeh et al., 2010). Previous studies have shown changes in soil chemical properties with an increase in plant species (Dahmardeh et al., 2010; Zhang et al., 2016), although it may take several years to show effects (van Ruijven and Berendse, 2005; Dybzinski et al., 2008). This is especially true for changes in soil C in the Canadian prairies, due to the cool climate and decomposition rates (VandenBygaart et al., 2008). For example, Blanco-Canqui et al. (2013) found that including triticale and legume cover crops in cereal based monocultures increased soil N, P, and organic C content after 5 years of cropping. Thus, significant changes in soil properties other than N levels, such as soil C and available P, may not have been observed in our study due to the shorter study period.
We detected significant changes in soil N and P between sampling dates, indicating that soil properties can change significantly within a growing season. Many N-based soil nutrients, such as ammonium, nitrate, potentially mineralizable N, and urease activity, show seasonal changes due to changes in soil water content (López-Poma et al., 2020) and crop uptake, suggesting seasonal changes of soil chemical properties may be related to the variation in soil moisture and crop phenology. Soil moisture is the driver of many soil functions especially in arid and semi-arid regions, such as earthworm activity and the soil functional microbial community, all of which relates to soil nutrient cycles (He et al., 1997; Cavagnaro, 2016; Kooch et al., 2020). In our study, soil moisture at both the Brookdale site and Swift Current site decreased significantly in 2016 and 2017 from the mid to late sampling dates, therefore the observed change in soil nutrients may be linked to variation in soil moisture.
We identified minor shifts in the composition of the soil microbial communities among the forage cover crop mixtures and oat monoculture, and early indicators of changes to the microbial diversity. Previous research has shown that increasing plant diversity in polycultures can increase microbial diversity (Qiao et al., 2012; LeBlanc et al., 2015). In this study we found mixed results. At the Brookdale site we found no effect of increasing cover crop diversity on microbial diversity or community composition. This is similar to a study by Dassen et al. (2017), who found that the inclusion of specific plant functional groups and soil abiotic properties were more important than plant species richness. We included equal proportions of each plant functional group (e.g., grass, legume, brassica) in all of our mixtures and that may explain why we didn't observe differences in soil microbial diversity among the forage cover crop mixtures. This doesn't explain the lack of differences between the mixtures and the oat monoculture, which may instead be linked to the minimal changes in soil abiotic properties at Brookdale. Malý et al. (2000) also observed no significant effects of plant species composition or diversity on soil microbial properties and speculated that the length of their study (2 years) was not long enough to detect differences. A grassland plant diversity study supports this hypothesis as they were only able to detect significant effects of plant diversity on soil microbial communities after 4 years, suggesting a lag time for soil microbial responses to changes in aboveground vegetation (Habekost et al., 2008). The short time frame of our study (2 years) may partially explain the lack of changes we observed in the microbial community at Brookdale.
In contrast to the Brookdale site, at the Swift Current site we observed differences in the soil microbial diversity and composition among the crop treatments in both years. This included an increase in bacterial diversity in the mixtures (three and six spp.) compared to the oat monoculture in 2017, and decrease in fungal diversity in all three mixtures compared to the oat monoculture in both years. The decrease in fungal diversity in the mixtures could be linked to differences in plant biomass production and soil nitrate levels compared to the oat monoculture. A grassland biodiversity experiment revealed a strong link between plant productivity and subsequent resource availability and fungal diversity (Zak et al., 2003; Waldrop et al., 2006). In our study, the oat monoculture had higher aboveground plant biomass production than the mixtures (although not significant at all sampling dates) and likely had higher belowground production as well due to the larger rooting capacity of grasses compared to leguminous and some brassica crops (Liu et al., 2011), which may have provided greater resource availability for the fungal community. Swift Current was also the site where we observed the most differences in soil properties among the cropping treatments, including increased soil nitrate levels in the mixtures during both years. Detheridge et al. (2016) found that soil nitrate levels were one of the key factors driving shifts in soil fungal diversity in forage cropping systems. An alternate explanation for the decreased fungal diversity in the mixtures may be linked to the inclusion of leguminous forage crop species. For example, Bainard et al. (2017) found that increasing the frequency of leguminous crops in annual crop rotations led to a decrease in fungal diversity, particularly in comparison to wheat monocultures.
We also observed temporal shifts in the composition of the bacterial and fungal communities between the mid and late season sampling dates for three of the four eco-site years. Temporal shifts in bacterial and fungal communities within an agricultural growing season has been consistently reported (Gomes et al., 2001; Smalla et al., 2001; Habekost et al., 2008). Within a regional context, soil microbial community composition and activity in the Canadian prairies has been correlated with seasonal shifts in soil moisture and nutrients (Hamel et al., 2006; Bainard et al., 2016). This was particularly evident at the Swift Current site, where we detected a significant drop in soil moisture and nitrate levels between the sampling dates in both years, and corresponding shifts in the bacterial and fungal communities.
Interestingly, we found that the oat monoculture had a significantly lower abundance of fungal pathotrophs compared to the cover crop mixtures at the Brookdale site in 2017 and the Swift Current site in 2016. This contradicts previous studies that have reported higher disease suppression in intercropping systems (Boudreau, 2013). This may be due to the selection of crop species or functional groups that were included in our mixtures. Although we did not specifically assess the crops for disease symptoms, the relative abundance of fungal pathotrophs provides a potential indicator that these type of forage mixtures may not necessarily provide a break in the disease cycle for susceptible crops that follow in the rotation. This highlights the need for producers to focus on crop selection rather than the level of plant diversity in forage cover crop mixtures in order to reduce the potential carry-over or build-up of soil pathogens (Panth et al., 2020).
Given the many suggested benefits of diverse annual forage cover crops, it is important to quantitatively evaluate the impact of these cropping mixtures. Over 2 years and two research sites on the Canadian prairies, we found minimal impact of increased forage crop diversity on forage productivity, soil nutrients and soil microbial communities. However, diverse mixtures did have better forage nutrition compared to an oats monoculture, indicating that it is possible to improve forage quality of a crop, while not sacrificing productivity or negatively impacting the soil community. While the mixtures performed better than the monoculture, there wasn't any strong evidence to show that increasing species diversity improved forage nutrition, suggesting that the inclusion of different functional groups is the most important consideration. Within the functional groups tested here, producers should select specific species based on local growing conditions and plant-pathogen dynamics.
The raw data supporting the conclusions of this article will be made available by the authors, without undue reservation, to any qualified researcher.
LB and JB contributed to all aspects of the work. BE contributed to the field and laboratory work and writing of the manuscript. EM and TY contributed to the writing of the manuscript. All authors approved the manuscript for publication.
The authors declare that this study received funding from the Manitoba Beef and Forage Initiatives, Inc. This funder was involved with seeding and maintaining the field trial at the Brookdale Research Farm, but was not involved in the study design, collection of data, analysis, interpretation of data, the writing of this article or the decision to submit it for publication.
The authors declare that the research was conducted in the absence of any commercial or financial relationships that could be construed as a potential conflict of interest.
We gratefully appreciate the technical support from the Brookdale Research Farm staff, Keith Hanson, Perry Coward, Russ Muri, and various Agriculture and Agri-Food Canada summer students.
The Supplementary Material for this article can be found online at: https://www.frontiersin.org/articles/10.3389/fsufs.2020.560479/full#supplementary-material
Anil, L., Park, J., Phipps, R., and Miller, F. (1998). Temperate intercropping of cereals for forage: a review of the potential for growth and utilization with particular reference to the UK. Grass Forage Sci. 53, 301–317. doi: 10.1046/j.1365-2494.1998.00144.x
Assefa, G., and Ledin, I. (2001). Effect of variety, soil type and fertiliser on the establishment, growth, forage yield, quality and voluntary intake by cattle of oats and vetches cultivated in pure stands and mixtures. Anim. Feed Sci. Technol. 92, 95–111. doi: 10.1016/S0377-8401(01)00242-5
Bainard, L. D., Hamel, C., and Gan, Y. (2016). Edaphic properties override the influence of crops on the composition of the soil bacterial community in a semiarid agroecosystem. Appl. Soil Ecol. 105, 160–168. doi: 10.1016/j.apsoil.2016.03.013
Bainard, L. D., Navarro-Borrell, A., Hamel, C., Braun, K., Hanson, K., and Gan, Y. (2017). Increasing the frequency of pulses in crop rotations reduces soil fungal diversity and increases the proportion of fungal pathotrophs in a semiarid agroecosystem. Agric. Ecosyst. Environ. 240, 206–214. doi: 10.1016/j.agee.2017.02.020
Bates, D., Mächler, M., Bolker, B., and Walker, S. (2014). Fitting linear mixed-effects models using lme4. arXiv arXiv preprint:1406.5823. doi: 10.18637/jss.v067.i01
Bergtold, J. S., Ramsey, S., Maddy, L., and Williams, J. R. (2017). A review of economic considerations for cover crops as a conservation practice. Renewable Agric. Food Syst. 34, 62–76. doi: 10.1017/S1742170517000278
Blanco-Canqui, H., Holman, J. D., Schlegel, A. J., Tatarko, J., and Shaver, T. M. (2013). Replacing fallow with cover crops in a semiarid soil: effects on soil properties. Soil Sci. Soc. Am. J. 77, 1026–1034. doi: 10.2136/sssaj2013.01.0006
Blanco-Canqui, H., Shaver, T. M., Lindquist, J. L., Shapiro, C. A., Elmore, R. W., Francis, C. A., et al. (2015). Cover crops and ecosystem services: insights from studies in temperate soils. Agron. J. 107, 2449–2474. doi: 10.2134/agronj15.0086
Bonin, C. L., and Tracy, B. F. (2012). Diversity influences forage yield and stability in perennial prairie plant mixtures. Agric. Ecosyst. Environ. 162, 1–7. doi: 10.1016/j.agee.2012.08.005
Boudreau, M. A. (2013). Diseases in intercropping systems. Annu. Rev. Phytopathol. 51, 499–519. doi: 10.1146/annurev-phyto-082712-102246
Buza, M. H., Holden, L. A., White, R. A., and Ishler, V. A. (2014). Evaluating the effect of ration composition on income over feed cost and milk yield. J. Dairy Sci. 97, 3073–3080. doi: 10.3168/jds.2013-7622
Caballero, R., Goicoechea, E., and Hernaiz, P. (1995). Forage yields and quality of common vetch and oat sown at varying seeding ratios and seeding rates of vetch. Field Crops Res. 41, 135–140. doi: 10.1016/0378-4290(94)00114-R
Caporaso, J. G., Lauber, C. L., Walters, W. A., Berg-Lyons, D., Huntley, J., Fierer, N., et al. (2012). Ultra-high-throughput microbial community analysis on the illumina HiSeq and MiSeq platforms. ISME J. 6, 1621–1624. doi: 10.1038/ismej.2012.8
Cavagnaro, T. R. (2016). Soil moisture legacy effects: impacts on soil nutrients, plants and mycorrhizal responsiveness. Soil Biol. Biochem. 95, 173–179. doi: 10.1016/j.soilbio.2015.12.016
Cloutier, M. L., Murrell, E., Barbercheck, M., Kaye, J., Finney, D., García-González, I., et al. (2020). Fungal community shifts in soils with varied cover crop treatments and edaphic properties. Sci. Rep. 10:6198. doi: 10.1038/s41598-020-63173-7
Dabney, S. M., Delgado, J. A., and Reeves, D. W. (2001). Using winter cover crops to improve soil and water quality. Commun. Soil Sci. Plant Anal. 37, 1221–1250. doi: 10.1081/CSS-100104110
Dahmardeh, M., Ghanbari, A., Syahsar, B., and Ramrodi, M. (2010). The role of intercropping maize (Zea mays L.) and cowpea (Vigna unguiculata L.) on yield and soil chemical properties. Afr. J. Agric. Res. 5, 631–636. doi: 10.5897/AJAR09.607
Dassen, S., Cortois, R., Martens, H., de Hollander, M., Kowalchuk, G. A., van der Putten, W. H., et al. (2017). Differential responses of soil bacteria, fungi, archaea and protists to plant species richness and plant functional group identity. Mol. Ecol. 26, 4085–4098. doi: 10.1111/mec.14175
de Ruiter, J., Wilson, D., Maley, S., Fletcher, A., Fraser, T., Scott, W., et al. (2009). Management Practices for Forage Brassicas. Christchurch: Forage Brassica Development Group.
Delavaux, C. S., Bever, J. D., Karppinen, E. M., and Bainard, L. D. (2020). Keeping it cool: soil sample cold pack storage and DNA shipment up to 1 month does not impact metabarcoding results. Ecol. Evol. 10, 4652–4664. doi: 10.1002/ece3.6219
Detheridge, A. P., Brand, G., Fychan, R., Crotty, F. V., Sanderson, R., and Griffith, G. W. (2016). The legacy effect of cover crops on soil fungal populations in a cereal rotation. Agric. Ecosyst. Environ. 228, 49–61. doi: 10.1016/j.agee.2016.04.022
Dorn, B., Jossi, W., and van der Heijden, M. G. (2015). Weed suppression by cover crops: comparative on-farm experiments under integrated and organic conservation tillage. Weed Res. 55, 586–597. doi: 10.1111/wre.12175
Dybzinski, R., Fargione, J. E., Zak, D. R., Fornara, D., and Tilman, D. (2008). Soil fertility increases with plant species diversity in a long-term biodiversity experiment. Oecologia 158, 85–93. doi: 10.1007/s00442-008-1123-x
Edgar, R. C. (2013). UPARSE: highly accurate OTU sequences from microbial amplicon reads. Nat. Methods 10, 996–998. doi: 10.1038/nmeth.2604
Finney, D. M., Buyer, J. S., and Kaye, J. P. (2017). Living cover crops have immediate impacts on soil microbial community structure and function. J. Soil Water Conserv. 72, 361–373. doi: 10.2489/jswc.72.4.361
Fruet, A. P. B., Stefanello, F. S., Trombetta, F., De Souza, A. N. M., Rosado Júnior, A. G., Tonetto, C. J., et al. (2019). Growth performance and carcass traits of steers finished on three different systems including legume-grass pasture and grain diets. Animal 13, 1552–1562. doi: 10.1017/S1751731118003142
Gabriel, J., and Quemada, M. (2011). Replacing bare fallow with cover crops in a maize cropping system: yield, N uptake and fertiliser fate. Eur. J. Agron. 34, 133–143. doi: 10.1016/j.eja.2010.11.006
Gardner, J., Faulkner, D., and Hargrove, W. (1991). Use of cover crops with integrated crop-livestock production systems. Cover crops for clean water. Soil Water Conserv. Soc. 185–191.
Gentry, C. E., and Willis, R. B. (1988). Improved methods for automated determination of ammonium on soil extracts. Commun. Soil Sci. Plant Anal. 19, 721–737. doi: 10.1080/00103628809367970
Ghanbari-Bonjar, A., and Lee, H. (2003). Intercropped wheat (Triticum aestivum L.) and bean (Vicia faba L.) as a whole-crop forage: effect of harvest time on forage yield and quality. Grass Forage Sci. 58, 28–36. doi: 10.1046/j.1365-2494.2003.00348.x
Goering, H. K., and Van Soest, P. J. (1970). Forage Fiber Analysis (Apparatus, Reagents, Procedures, and Some Applications). Washington, DC: USDA Agricultural Research Service.
Gomes, N., Heuer, H., Schönfeld, J., Costa, R., Mendonca-Hagler, L., and Smalla, K. (2001). Bacterial diversity of the rhizosphere of maize (Zea mays) grown in tropical soil studied by temperature gradient gel electrophoresis. Plant Soil 232, 167–180. doi: 10.1007/978-94-010-0566-1_17
Griffith, A. P., Epplin, F. M., Fuhlendorf, S. D., and Gillen, R. (2011). A comparison of perennial polycultures and monocultures for producing biomass for biorefinery feedstock. Agron. J. 103, 617–627. doi: 10.2134/agronj2010.0336
Habekost, M., Eisenhauer, N., Scheu, S., Steinbeiss, S., Weigelt, A., and Gleixner, G. (2008). Seasonal changes in the soil microbial community in a grassland plant diversity gradient four years after establishment. Soil Biol. Biochem. 40, 2588–2595. doi: 10.1016/j.soilbio.2008.06.019
Hamel, C., Hanson, K., Selles, F., Cruz, A. F., Lemke, R., McConkey, B., et al. (2006). Seasonal and long-term resource-related variations in soil microbial communities in wheat-based rotations of the Canadian prairie. Soil Biol. Biochem. 38, 2104–2116. doi: 10.1016/j.soilbio.2006.01.011
Harm, J., Bettany, J., and Halstead, E. (1973). A soil test for sulphur and interpretative criteria for Saskatchewan. Commun. Soil Sci. Plant Anal. 4, 219–231. doi: 10.1080/00103627309366440
Hartwig, N. L., and Ammon, H. U. (2002). Cover crops and living mulches. Weed Sci. 50, 688–699. doi: 10.1614/0043-1745(2002)050[0688:AIACCA]2.0.CO;2
He, Z., Wu, J., O'Donnell, A., and Syers, J. (1997). Seasonal responses in microbial biomass carbon, phosphorus and sulphur in soils under pasture. Biol. Fertil. Soils 24, 421–428. doi: 10.1007/s003740050267
Hendershot, W. H., Lalande, H., and Duquette, M. (2008). “Soil reaction and exchangeable acidity,” in Soil Sampling and Methods of Analysis, 2nd edn, eds M. R. Carter, and E. G. Gregorich (Boca Raton, FL: Canadian Society of Soil Science and CRC Press), 173–178. doi: 10.1201/9781420005271.ch16
Kõljalg, U., Nilsson, R. H., Abarenkov, K., Tedersoo, L., Taylor, A. F. S., Bahram, M., et al. (2013). Towards a unified paradigm for sequence-based identification of fungi. Mol. Ecol. 22, 5271–5277. doi: 10.1111/mec.12481
Kooch, Y., Ehsani, S., and Akbarinia, M. (2020). Stratification of soil organic matter and biota dynamics in natural and anthropogenic ecosystems. Soil Tillage Res. 200:104621. doi: 10.1016/j.still.2020.104621
Kuznetsova, A., Brockhoff, P. B., and Christensen, R. H. B. (2017). lmerTest package: tests in linear mixed effects models. J. Stat. Softw. 82, 1–26. doi: 10.18637/jss.v082.i13
Lange, M., Eisenhauer, N., Sierra, C. A., Bessler, H., Engels, C., Griffiths, R. I., et al. (2015). Plant diversity increases soil microbial activity and soil carbon storage. Nat. Commun. 6:6707. doi: 10.1038/ncomms7707
LeBlanc, N., Kinkel, L. L., and Kistler, H. C. (2015). Soil fungal communities respond to grassland plant community richness and soil edaphics. Microb. Ecol. 70, 188–195. doi: 10.1007/s00248-014-0531-1
Lithourgidis, A., Vlachostergios, D., Dordas, C., and Damalas, C. (2011). Dry matter yield, nitrogen content, and competition in pea–cereal intercropping systems. Eur. J. Agron. 34, 287–294. doi: 10.1016/j.eja.2011.02.007
Liu, L., Gan, Y., Bueckert, R., and Van Rees, K. (2011). Rooting systems of oilseed and pulse crops. II: vertical distribution patterns across the soil profile. Field Crops Res. 122, 248–255. doi: 10.1016/j.fcr.2011.04.003
López-Poma, R., Pivello, V. R., de Brito, G. S., and Bautista, S. (2020). Impact of the conversion of Brazilian woodland savanna (cerradão) to pasture and Eucalyptus plantations on soil nitrogen mineralization. Sci. Total Environ. 704:135397. doi: 10.1016/j.scitotenv.2019.135397
Malézieux, E., Crozat, Y., Dupraz, C., Laurans, M., Makowski, D., Ozier-Lafontaine, H., et al. (2009). “Mixing plant species in cropping systems: concepts, tools and models: a review,” in Sustainable Agriculture, eds E. Lichtfouse, M. Navarrete, P. Debaeke, S. Véronique, and C. Alberola (Dordrecht: Springer), 329–353. doi: 10.1007/978-90-481-2666-8_22
Malý, S., Korthals, G., Van Dijk, C., Van der Putten, W., and De Boer, W. (2000). Effect of vegetation manipulation of abandoned arable land on soil microbial properties. Biol. Fertil. Soils 31, 121–127. doi: 10.1007/s003740050634
Marquard, E., Weigelt, A., Temperton, V. M., Roscher, C., Schumacher, J., Buchmann, N., et al. (2009). Plant species richness and functional composition drive overyielding in a six-year grassland experiment. Ecology 90, 3290–3302. doi: 10.1890/09-0069.1
Martin, K. J., and Rygiewicz, P. T. (2005). Fungal-specific PCR primers developed for analysis of the ITS region of environmental DNA extracts. BMC Microbiol. 5:28. doi: 10.1186/1471-2180-5-28
McKenzie, S. C., Goosey, H. B., O'Neill, K. M., and Menalled, F. D. (2017). Integration of sheep grazing for cover crop termination into market gardens: agronomic consequences of an ecologically based management strategy. Renewable Agric. Food Syst. 32, 389–402. doi: 10.1017/S1742170516000326
Miller, J. J., and Curtin, D. (2008). “Electrical conductivity and soluble ions,” in Soil Sampling and Methods of Analysis, 2nd edn, eds M. R. Carter, and E. G. Gregorich (Boca Raton, FL: Canadian Society of Soil Science and CRC Press), Pages 161–171
Oksanen, J., Blanchet, F., Kindt, R., Legendre, P., Minchin, R., O'hara, R., et al. (2013). Vegan: Community Ecology Package Version 2.0–10.
Panth, M., Hassler, S. C., and Baysal-Gurel, F. (2020). Methods for management of soilborne diseases in crop production. Agriculture 10:16. doi: 10.3390/agriculture10010016
Pirhofer-Walzl, K., Søegaard, K., Høgh-Jensen, H., Eriksen, J., Sanderson, M., Rasmussen, J., et al. (2011). Forage herbs improve mineral composition of grassland herbage. Grass Forage Sci. 66, 415–423. doi: 10.1111/j.1365-2494.2011.00799.x
Qiao, Y., Li, Z., Wang, X., Zhu, B., Hu, Y., and Zeng, Z. (2012). Effect of legume-cereal mixtures on the diversity of bacterial communities in the rhizosphere. Plant Soil Environ. 58, 174–180. doi: 10.17221/351/2011-PSE
Sadeghpour, A., Jahanzad, E., Esmaeili, A., Hosseini, M., and Hashemi, M. (2013). Forage yield, quality and economic benefit of intercropped barley and annual medic in semi-arid conditions: additive series. Field Crops Res. 148, 43–48. doi: 10.1016/j.fcr.2013.03.021
Schellenberg, M., Biligetu, B., and Iwaasa, A. (2012). Species dynamic, forage yield, and nutritive value of seeded native plant mixtures following grazing. Can. J. Plant Sci. 92, 699–706. doi: 10.4141/cjps2011-273
Schultz, R. D., and Stubbendieck, J. (1983). Herbage quality of fertilized cool-season grass-legume mixtures in Western Nebraska. J. Range Manage. 36, 571–575. doi: 10.2307/3898342
Schumacher, B. A. (2002). Methods for the Determination of Total Organic Carbon (TOC) in Soils and Sediments. Las Vegas, NV: Ecological Risk Assessment Support Center, US Environmental Protection Agency.
Smalla, K., Wieland, G., Buchner, A., Zock, A., Parzy, J., Kaiser, S., et al. (2001). Bulk and rhizosphere soil bacterial communities studied by denaturing gradient gel electrophoresis: plant-dependent enrichment and seasonal shifts revealed. Appl. Environ. Microbiol. 67, 4742–4751. doi: 10.1128/AEM.67.10.4742-4751.2001
Stewart, A. V., and Moorhead, A. J. (2004). The development of a fodder radish suitable for multiple grazing. Agronomy NZ 34, 1–7.
Taylor, D. L., Walters, W. A., Lennon, N. J., Bochicchio, J., Krohn, A., Caporaso, J. G., et al. (2016). Accurate estimation of fungal diversity and abundance through improved lineage-specific primers optimized for Illumina amplicon sequencing. Appl. Environ. Microbiol. 82, 7217–7226. doi: 10.1128/AEM.02576-16
Tilman, D., Reich, P. B., Knops, J., Wedin, D., Mielke, T., and Lehman, C. (2001). Diversity and productivity in a long-term grassland experiment. Science 294, 843–845. doi: 10.1126/science.1060391
Tufail, M. S., Krebs, G. L., Southwell, A., Piltz, J. W., Norton, M. R., and Wynn, P. C. (2020). Enhancing performance of berseem clover genotypes with better harvesting management through farmers' participatory research at smallholder farms in Punjab. Sci. Rep. 10:3545. doi: 10.1038/s41598-020-60503-7
U. S. Environmental Protection Agency (1996). Method 3052: Microwave Assisted Acid Digestion of Siliceous and Organically Based Matrices. Test Methods for Evaluating Solid Waste, Physical/Chemical Methods. EPA.
van Ruijven, J., and Berendse, F. (2005). Diversity–productivity relationships: initial effects, long-term patterns, and underlying mechanisms. Proc. Natl. Acad. Sci. U.S.A. 102, 695–700. doi: 10.1073/pnas.0407524102
VandenBygaart, A., McConkey, B., Angers, D., Smith, W., De Gooijer, H., Bentham, M., et al. (2008). Soil carbon change factors for the Canadian agriculture national greenhouse gas inventory. Can. J. Soil Sci. 88, 671–680. doi: 10.4141/CJSS07015
Venter, Z. S., Scott, S. L., Strauss, J., Jacobs, K., and Hawkins, H. J. (2017). Increasing crop diversity increased soil microbial activity, nitrogen-sourcing and crop nitrogen, but not soil microbial diversity. S. Afr. J. Plant Soil 34, 371–378. doi: 10.1080/02571862.2017.1317852
Waldrop, M. P., Zak, D. R., Blackwood, C. B., Curtis, C. D., and Tilman, D. (2006). Resource availability controls fungal diversity across a plant diversity gradient. Ecol. Lett. 9, 1127–1135. doi: 10.1111/j.1461-0248.2006.00965.x
Wang, Q., Garrity, G. M., Tiedje, J. M., and Cole, J. R. (2007). Naive bayesian classifier for rapid assignment of rRNA sequences into the new bacterial taxonomy. Appl. Environ. Microbiol. 73, 5261–5267. doi: 10.1128/AEM.00062-07
Wittwer, R. A., Dorn, B., Jossi, W., and van der Heijden, M. G. A. (2017). Cover crops support ecological intensification of arable cropping systems. Sci. Rep. 7:41911. doi: 10.1038/srep41911
Zak, D. R., Holmes, W. E., White, D. C., Peacock, A. D., and Tilman, D. (2003). Plant diversity, soil microbial communities, and ecosystem function: are there any links? Ecology 84, 2042–2050. doi: 10.1890/02-0433
Zhang, C., Liu, G., Xue, S., and Wang, G. (2016). Soil bacterial community dynamics reflect changes in plant community and soil properties during the secondary succession of abandoned farmland in the Loess Plateau. Soil Biol. Biochem. 97, 40–49. doi: 10.1016/j.soilbio.2016.02.013
Keywords: forage cover crop, soil chemistry, bacteria, fungi, forage nutrition, polyculture, microbial community
Citation: Bainard LD, Evans B, Malis E, Yang T and Bainard JD (2020) Influence of Annual Plant Diversity on Forage Productivity and Nutrition, Soil Chemistry, and Soil Microbial Communities. Front. Sustain. Food Syst. 4:560479. doi: 10.3389/fsufs.2020.560479
Received: 09 May 2020; Accepted: 28 August 2020;
Published: 09 October 2020.
Edited by:
Autar Krishen Mattoo, Agricultural Research Service (USDA), United StatesReviewed by:
Tahira Fatima, Purdue University, United StatesCopyright © 2020 Bainard, Evans, Malis, Yang and Bainard. This is an open-access article distributed under the terms of the Creative Commons Attribution License (CC BY). The use, distribution or reproduction in other forums is permitted, provided the original author(s) and the copyright owner(s) are credited and that the original publication in this journal is cited, in accordance with accepted academic practice. No use, distribution or reproduction is permitted which does not comply with these terms.
*Correspondence: Jillian D. Bainard, amlsbGlhbi5iYWluYXJkQGNhbmFkYS5jYQ==
Disclaimer: All claims expressed in this article are solely those of the authors and do not necessarily represent those of their affiliated organizations, or those of the publisher, the editors and the reviewers. Any product that may be evaluated in this article or claim that may be made by its manufacturer is not guaranteed or endorsed by the publisher.
Research integrity at Frontiers
Learn more about the work of our research integrity team to safeguard the quality of each article we publish.