- Soil and Water Management Research Unit, Conservation and Production Research Laboratory, U.S. Department of Agriculture, Agricultural Research Service, Bushland, TX, United States
Continued pumping for irrigation from the non-recharging Ogallala aquifer in Kansas and Texas is unsustainable. Reducing risks for dryland wheat (Triticum aestivum L) and sorghum [Sorghum bicolor (L.) Moench] production, which depends exclusively on precipitation to meet water demand is critical for future adoption. Stormwater runoff reduces the amount of precipitation available to crops, but management practices to minimize runoff concomitantly increase the opportunity time for infiltration and improve precipitation storage as soil water for crop use. Our objectives in this study were to evaluate tillage, slope and the effects of contour or with-slope farming on runoff, soil water at planting, and the growth and yield of wheat and sorghum grown in the 3 years wheat-sorghum-fallow (WSF) rotation. Long-term, 1983 to present, runoff was measured from gauged terraced and contour farmed fields managed in the WSF rotation with no-tillage (NT) or stubble-mulch (SM) tillage. We found significantly greater mean cumulative runoff during fallow for NT than for SM but only for the 1.8% terrace slopes., The corresponding soil water with NT increased by a significant ~27 mm over SM due to reduced evaporation but generally did not differ due to slope. Wheat yield decreased significantly as slope decreased from 1.8 to 1.2% but exhibited no yield response tillage. In contrast, grain sorghum yields were greater with NT than SM tillage residue management. Farming along the contour or slope manifested no differences in soil water, crop grain yield, or water use; however, they did increased significantly with no tillage for sorghum but not wheat. We conclude that management of tillage was more effective than slope effects in increasing water availability to crops because of evaporation reduction with crop residue.
Introduction
Successful irrigated agricultural production requires an adequate source of suitable quality water and for much of Western Kansas in the Texas Panhandle that water is provided by the Ogallala Aquifer. The Ogallala Aquifer is, essentially, non-recharging south of Nebraska (McGuire, 2017) so that continued pumping and ongoing depletion makes irrigation unsustainable and dryland crop production an eventuality. Dryland crop production, by contrast, depends exclusively on water from precipitation during the growing season or stored in the soil, which is governed by evaporation and infiltration processes. Evaporation is largely driven by net solar irradiance that can be intercepted by crop residue mulches, resulting in reduced evaporation for greater stored soil water during the growing season (Lascano and Baumhardt, 1996) or fallow periods (Unger and Baumhardt, 1999; Baumhardt et al., 2017). Likewise, these residue mulches can also intercept raindrop impact and prevent crust formation to improve rain infiltration (Duley, 1939; Baumhardt and Lascano, 1996; Baumhardt et al., 2012). Residue needed to conserve soil water by reducing evaporation and improving infiltration was normally buried by pulverizing tillage used in the early twentieth century while forming a dust mulch to control evaporation as recommended by Campbell (1907). That dust mulch, however, was susceptible to wind erosion.
During the 1930's dust bowl, Howard Finnell observed viable native range lands and suggested the precipitation was adequate to produce crop biomass for residue and marketable yields (Duncan and Burns, 2012). In an assessment of using level terraces as a water conservation practice in the semiarid dustbowl region, Finnell (1930) reported 13.5% less runoff from terraced plots compared with no terraces. Although the associated sorghum [Sorghum bicolor (L.) Moench] and wheat (Triticum aestivum L) grain or forage yield increases ranged from 4.8 to 49.1%, he offered the caveat that the yield increasing function of terraces from their water savings depended on time of excessive rains and the subsequent runoff liability. Or as Finnell more succinctly put it “terraces will certainly not save any water if there is none going to waste as runoff” (1930). His listed factors governing terrace efficiency to conserve water included climate elements like runoff producing storm frequency or storm intensities and duration in addition to soil characteristics like infiltrability and profile water holding capacity. The soil slope was also a contributing component to water conservation in that greater slopes could reduce the opportunity time available for rain infiltration.
Water conservation and crop yields from graded and level terraces were compared beginning in 1949 (Hauser et al., 1962). This study confirmed that level closed-end terraces presented a crop flood hazard by the impounded water not observed on graded terraces; however, the sorghum crop often benefited from the impounded water. Infrequent flood injury by impounded water from the contributing terrace watershed was largely prevented using leveled terrace benches (Zingg and Hauser, 1959). A 10-years evaluation of annually cropped bench terraces had similar sorghum grain yields as the level or graded terraces in a wheat-sorghum-fallow (WSF) rotation (Hauser, 1968). Runoff from the graded terraces was measured with type H flumes beginning in 1958 for improved correlation to precipitation (Hauser and Jones, 1991). Jones et al. (1985) characterized runoff and soil losses from stubble-mulch (SM) tilled graded terraces in the WSF rotation for variable rain event conditions and identified the fallow rotation phases and storm amounts with the greatest losses. Jones et al. (1994) built on this work after adding NT residue management by determining reduced infiltration, greater runoff, but more water conservation with NT than SM tillage. The effects of long-term NT and SM tillage or climate [i.e., El Niño Southern Oscillation phase] have been evaluated using runoff observations in relation to field hydrology (Baumhardt et al., 2017) and crop performance (Baumhardt et al., 2016).
Field runoff is directly governed by soil infiltration capacity and precipitation intensity combined with duration for amount. Rainfall events exceeding 25.5 mm practically always generated runoff while rains of 12.5 – 25.4 mm lost runoff about half of the time, but rain events of 6.4 – 12.5 mm and <6.4 mm had little (~5%) or no runoff (Dockal, 2019). Runoff producing events increased when preceded by at least one storm during the week that would increase soil water content and reduce both water holding capacity and the gradient driving infiltration. Although lower infiltration capacity and greater runoff from NT fields covered with raindrop intercepting residue is counter to findings reported by many (Duley, 1939; Lindstrom et al., 1984; Nielsen et al., 2005; Blanco-Canqui and Lal, 2009), infiltration capacity may be depressed for certain initial soil conditions. That is, if the prevailing surface conditions are crusted or consolidated (Jones et al., 1994) or, as noted before, if the soil water content is higher. Despite contour farming to reduce runoff by increasing infiltration opportunity time, the observed runoff from 1.8% slope landscapes was greater than for landscapes with a slope of 1.2% (Dockal, 2019).
Investigations comparing slope and contour farming effects on runoff from gently sloping fields were not found in the literature. Our objectives in this study were to evaluate tillage, slope and the effects of contour or with-slope farming on runoff, soil water at planting, and the growth and yield of wheat and sorghum grown in the 3 years wheat-sorghum-fallow (WSF) rotation.
Materials and Methods
Long-term research was conducted at the USDA- Agricultural Research Service, Conservation and Production Research Laboratory, Bushland, TX (35° 11' N, 102° 5' W; and 1,170 m MSL), which has ~190 frost-free days, a semiarid climate with mean annual pan evaporation of 2,600 mm and precipitation of ~460 mm. The landscape is gently sloping (~1.5%) with a 1.8-m deep Pullman clay loam (fine, mixed, superactive, thermic Torrertic Paleustoll) having 9 g kg−1 organic carbon in the 0.0–0.15 m depth (Unger and Pringle, 1981; Schwartz et al., 2015). The WSF rotation (Baumhardt and Anderson, 2006) was established in 1949 on six large, 2.0–4.1 ha, terraced fields varying from 1.2 to 1.8% slope, from 630 to 660 m length and from 32 to 62 m width, which were graded to 0.05% channel slope and contour-farmed (Hauser and Jones, 1991; Jones et al., 1994). These six terrace fields were uniformly SM tilled until 1983 when they were divided into SM or NT residue management field pairs (Jones et al., 1994) with all phases of the 3-years WSF rotation present each year. Our first experiment evaluates tillage and slope effects on runoff, soil water, and crop yield for all WSF rotation phases, appearing annually as replicates categorized by year for the 20 years period of record of 1983–2012 that avoids more recent crop failures due to drought and unexpected flooding.
In lieu of an experiment that specifically compared soil water, crop yield, and water use for both contour and with-slope farming practices, our second experiment combines data from 2000–2009 for contour farming on graded terraces and a nearby, ~1 km, secondary site (35°10.25' N, 102°5' W). There, the WSF rotation was maintained with all phases appearing each year in 55 m wide plots that averaged 200 –m long with tillage assigned randomly in blocks. The SM and NT residue management was practically identical to the graded terraces and featured the same gently sloping 1.8-m deep Pullman clay loam but was farmed with-slope (Baumhardt et al., 2011). Soil water, crop yield, and water use comparisons between sites were possible because of common rotation and tillage management at each site. Unlike the contour farmed terraces the secondary site had no runoff measurement facilities and a limited data record when forage production began after 2009 because of drought related grain production failures.
Agronomic Management
The WSF rotation (Figure 1) began in September or October when soil water permitted establishment of wheat (various cultivars) sowed in rows 0.3-m apart using a high-clearance hoe opener grain drill at 45 kg ha−1 for a 200 plants m−2 plant density. Growing season broadleaf weeds in wheat were controlled in the spring using 0.6 kg a.i. ha−1 2,4-D [(2,4-dichlorophenoxy) acetic acid]. After wheat harvest in July, fields were fallowed for approximately 11 months with NT or conventional SM tillage. Weed control in the NT fallow after wheat was by a combination of 0.84 kg a.i. ha−1 2,4-D and 1.1 kg a.i. Ha−1 atrazine [6-chloro-N-ethyl-N′-(1-methylethyl)-1,3,5-triazine-2,4-diamine]., while SM fallow required 3 to 4 tillage operations to a 0.10-m depth using, for example, a 4.6-m-wide Richardson sweep-plow (Sunflower Man. Co., Inc., Beloit, KS).
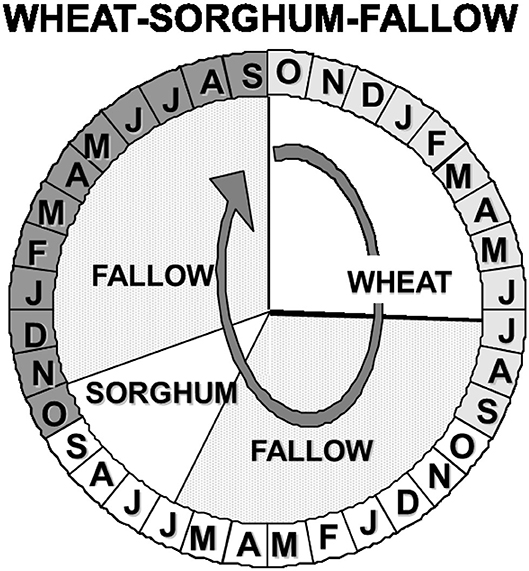
Figure 1. The wheat-sorghum-fallow crop rotation diagramed as a clock-like 3-years cycle beginning with wheat establishment in October. Wheat is harvested in July and subsequently fallowed until June of the 2nd year (11-months) when grain sorghum is established. After sorghum harvest in November of the 3rd year, fallow is again maintained until wheat is planted and the cycle repeated.
During the summer of second rotation-year (Figure 1), grain sorghum (various cultivars) was planted in rows spaced 0.75-m apart at 8.0 seeds m−2 using a 6-row “Max-Emerge” planter (John Deere, East Moline, IL). A pre-emergence application of 1.7 kg a.i. ha−1 propazine [6-chloro-N,N′-bis (1-methylethyl)-1,3,5-triazine-2,4-diamine] or 1.3 kg a.i. ha−1 atrazine, metolachlor (Table 1) provided growing season weed control in sorghum. Beginning in the mid-1990's, sorghum seed was treated with fluxofenim [1-(4-chlorophenyl)-2,2,2-trifluoroethanone O-(1,3-dioxolan-2-ylmethyl)oxime] to permit application of 1.0 kg a.i. ha−1 commercially available mixtures of atrazine and metolachlor [2-chloro-N-(2-ethyl-6-methylphenyl)-N-(2-methoxy-1-methylethyl) acetamide]. Grain sorghum was harvested at maturity in November of the third rotation-year and subsequently fallowed ~10 months when the rotation repeated with wheat planting. We applied 2,4-D plus commercially available premix of 0.045 kg a.i. ha−1 chlorosulfuron [2-chloro-N[[(4-methoxy-6-methyl-1,3,5-triazin-2-yl)amino]carbonyl] benzenesulfanomide] and 0.009 kg a.i. ha−1 metsulfuron-methyl [Methyl 2-[[[[(4-methoxy-6-methyl-1,3,5-triazin-2-yl) amino]carbonyl] amino]sulfonyl]benzoate] for weed control in the spring during fallow after sorghum until subsequent wheat establishment. Any Fallow or pre-plant weed escapes during fallow were controlled with applications of 0.56 kg a.i. ha−1 glyphosate [N-(phosphonomethyl) glycine] or 0.37 kg a.i. ha−1 2,4-D.
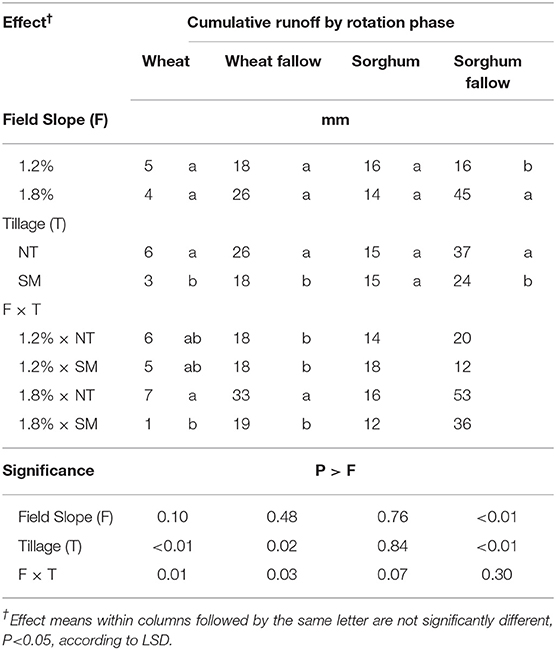
Table 1. Mean cumulative runoff, mm, from wheat, fallow after wheat, sorghum, and fallow after sorghum rotation phases for field slope, tillage, and their interaction effects with the corresponding ANOVA significance levels.
Mineralization of N during the fallow periods between wheat and grain sorghum plus any atmospheric N deposition and residual nitrate in the profile supply the needed 50 kg ha−1 N for meeting dryland crop yields (Schwartz et al., 2015). Eck and Jones (1992) observed that the soil supplied sufficient N to meet potential yields as supported by the expected precipitation and soil water; thus, negating any application of N fertilizer. No supplemental P or K fertilizers were applied because the Pullman clay mineralogy supplies sufficient K to meet crop demand (Johnson et al., 1983) and dryland crop response to broadcast applied P fertilizer has been limited (Eck, 1969, 1988).
Measurements
Our rainfall and runoff observations span 30 years with precipitation measurements using two standard (203 mm diam) United States National Weather Service rain gages. Storm event runoff was measured using 0.91 and 0.76 m type-H flumes (Hauser and Jones, 1991) equipped with Belfort FW-1 stage recorders for flow rate as described by Brakensiek et al. (1979). Breakpoint chart analyses method was used from 1984 to 1990 and horizontal summation has been retained during all remaining years of record. Rotation phase specific runoff was the sum of runoff events for all phases of the WSF rotation. The amount of water actually entering the soil was calculated by deducting observed runoff from precipitation.
Soil water was sampled gravimetrically for the 1.8 m profile in 0.3-m increments during planting and harvest at six locations within each terrace field for spatial replicates. The volumetric soil water content was calculated using previously measured bulk density (Jones et al., 1994) and reported as plant-available soil water (i.e., water held between 0.03- and 1.5-MPa matric water potential). As with the soil water measurements, crop yield was determined from six spatial replicates at sites distributed equidistantly within the field that totaled ~ 6 m2 for wheat and ~ 4.5 m2 for sorghum. Wheat grain yield was estimated from those hand samples and corrected to a standardized 120 g kg−1 water content (wet basis). Above ground hand samples were collected at grain sorghum maturity for biomass and grain yield that was adjusted to a standardized 130 g kg−1 water content.
Analyses
The rotation phase specific cumulative runoff, stored soil water at fallow and planting, crop grain yield and crop water use observations were compared for a 30-years, 1983–2012, study using mixed linear model ANOVA procedures and LSD for mean separation (SAS, 2014). The categorized year of observation provided annual replication of runoff as the random effect when comparing tillage and field slope fixed effects (Milliken and Johnson, 2009). Observed soil water, yields, and calculated water use response to tillage and field slope fixed effects was replicated annually in addition to the spatial replicates sampled within each terraced field as random effects for all rotation phases. Multiple replicate samplings within a single large field is valid provided the distance between observations exceeds the range of spatial dependence (Griffin et al., 2004; Alesso et al., 2019) (i.e., each observation is an independent replicate). In a paper characterizing the spatiotemporal soil profile water content for a Pullman soil using various sensors), Evett et al. (2009) described semivariogram methods to quantify the range where autocorrelation of neutron soil moisture measurements were negligible. The corresponding semivariogram range for autocorrelation, although not provided, was about 30 m (personal communication Evett) or possibly less, [i.e., <6 m] based on Moran's I (Schwartz et al., 2010). Because the separation between our six observations within each terraced field exceeded 90 m, or three times the semivariogram range value, replicates were considered independent.
The SM or NT tillage and contour or with-slope management fixed effects on stored soil water at fallow and planting, crop grain yield, and crop water use were compared between graded terrace fields and the secondary site using a data set truncated to common study years from 2000 to 2009. Study random effects used when comparing tillage and contour management fixed effects included both replications temporally by categorical year and spatially by adhering to site statistical design (Baumhardt et al., 2011, 2017). The annual replicated observations for the truncated, 10-years, record were also plotted by declining rank as a function of the exceedance probability according to Barfield et al. (1981). Unless specified otherwise, all statistical analysis effects were significant at the 0.05 probability level.
Results
Stormwater Runoff and Soil Water
We compared mean cumulative runoff as affected by both field slope and tillage fixed effects for the growing crops and subsequent fallow periods of the WSF rotation (Figure 1), which are shown in Table 1 with the corresponding ANOVA significance levels. These results build upon rain event specific findings of greater runoff losses from graded terrace fields having greater slope (Dockal, 2019). The mean cumulative runoff during the growing wheat and grain sorghum phases appeared to manifest no significant differences due to tillage; however, a significant interaction between tillage and slope effects was identified. The interaction resulted when runoff from NT was significantly greater than SM on higher 1.8% slope and did not occur with SM. Growing season crop water use may have increased the amount of water the soil profile could hold and promoted greater rain infiltration and minimized runoff. By contrast, cumulative runoff from growing grain sorghum averaging 15 mm for both NT and SM did not differ regardless of crop water. For both crops, canopy closure can act to intercept raindrops in the same way as a NT residue cover for improved rain infiltration and limited runoff. Although tillage effects on cumulative runoff amounts were previously identified (Jones et al., 1994; Baumhardt et al., 2017) we evaluated potential interaction between tillage and slope effects. This interaction was also manifested as significant runoff differences between field slopes for SM but no difference in runoff from the NT fields for either field slope.
Cumulative runoff during both fallow after wheat and fallow after sorghum (Figure 1), shown in Table 1, increased significantly in terraced fields with the greater 1.8% slope, but not for wheat fallow at 1.2% slope due to interacting fixed effects. That is, increasing the slope from 1.2 to 1.8% likewise increased runoff ~50% from 19 to 33 mm during wheat fallow and nearly by a factor of three from 16 to 45 mm during sorghum fallow (Table 1). Runoff from stubble mulch tillage, however, eliminated any runoff differences due to slope during fallow after wheat but not during the fallow after sorghum. Tillage related runoff differences were more pronounced during fallow periods than while growing crops. Runoff from less sloping, 1.2%, fields did not differ with tillage effects during fallow after wheat but differed significantly for fallow after sorghum. Runoff from the higher sloping, 1.8%, fields, however, was significantly greater from NT fields than from SM fields during fallow after either wheat or sorghum. For those management combinations with greater runoff, the soil water storage may be expected to decrease.
The mean plant available soil water of the profile measured at wheat and sorghum planting (Figure 1) and the end of preceding fallow after sorghum and wheat (respectively) are listed in Table 2. The mean available soil water generally did not vary with field slope for any phase of the WSF rotation except at wheat planting, which had significantly more available soil water, 176 mm, in the 1.2% sloping field than the 1.8% sloping field with 159 mm. The increased soil water in fields having less slope would be expected due to potentially reduced runoff as was observed during sorghum fallow. By contrast, the available soil water averaged across all phases for NT management was 181 mm compared with the significantly lower 154 mm for similarly averaged SM tillage phases.
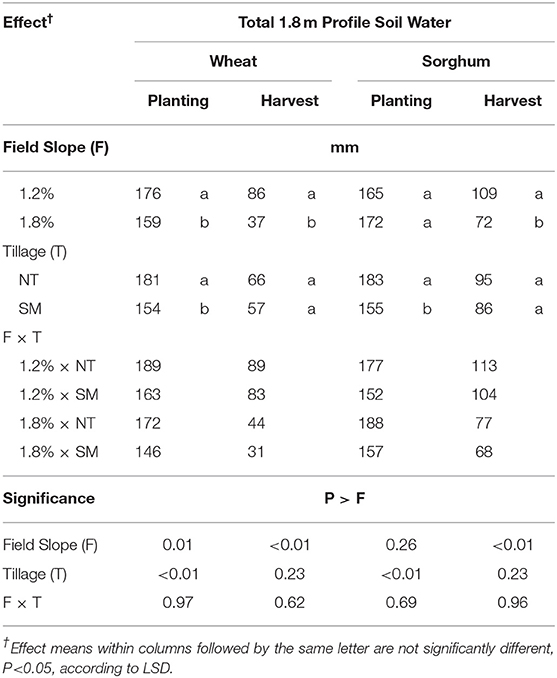
Table 2. Mean 1.8 m profile soil water, mm, at planting and harvest of wheat and sorghum in relation to the field slope, tillage, and interaction effects with the corresponding ANOVA significance levels.
Grain Yield
Mean grain yields and water use for wheat and sorghum are listed for the graded terraces in Table 3 by the field slope and tillage fixed effects. For both wheat and sorghum the field slope had a significant effect on grain yield that was inconsistent between crops. Although wheat grain yield was depressed significantly by the greater slope that could increase runoff, actual measured runoff during the wheat phase differed by only 1 mm. By contrast, sorghum grain yield increased significantly with the greater slope that also resulted in small, 2 mm, runoff difference. The lower wheat yields due to increased runoff losses may have occurred at critical crop growth stages after the profile's stored soil water was depleted. Despite the significant increase in stored soil water with NT, tillage effects on mean wheat grain yield, averaged across slope, did not differ between NT and SM tillage in contrast to grain sorghum yields that increased by a significant 15% for NT compared with SM. The corresponding crop water use (Table 3) for both wheat and grain sorghum indicated no significant differences attributable to the effects of either field slope (P = 0.13) or tillage (P = 0.10).
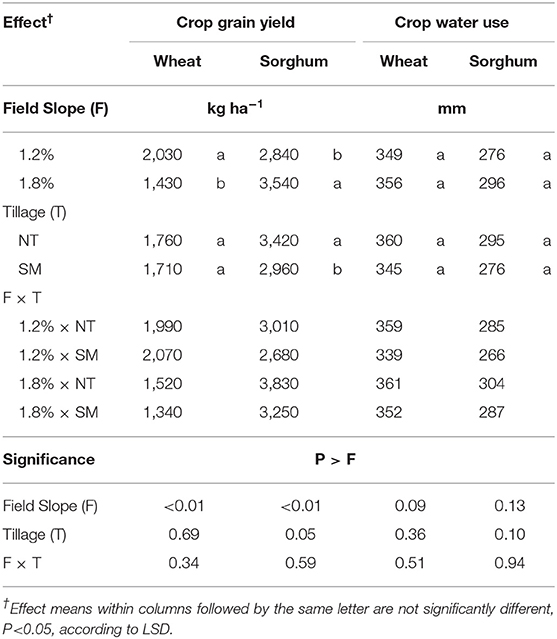
Table 3. Mean grain yield, kg ha−1, and water use, mm, of wheat and sorghum crops in relation to the field slope, tillage, and interaction effects with the corresponding ANOVA significance levels.
Comparisons of runoff reducing contour and runoff prone with-slope farming practices on wheat yield produced no significant difference in yields with contour farming (Table 4) and practically the same yields for both NT and SM tillage levels. The related crop water use for wheat, likewise, did not differ significantly with field slope because, in part, the soil water at wheat planting was practically the same between the contour and with-slope farming. Despite NT residue management increasing profile soil water significantly over SM tillage at wheat planting, crop water use was not significantly different between NT and SM tillage (Table 4). As noted for wheat, contour farmed grain sorghum yield also did not differ significantly (P = 0.12) from the 340 kg ha−1 smaller yield of 3,190 kg ha−1 for with-slope farming. In contrast with the wheat, sorghum grain yield with NT increased > 50% compared with the SM grain sorghum, which reflected the NT benefit of the significantly greater soil water at planting and, probably, residue cover that reduced seasonal evaporative losses. While grain sorghum water use did not differ significantly between contour and with-slope farming, the estimated grain sorghum water use with NT exceeded that for SM tillage significantly, or about 50 mm (Table 4).
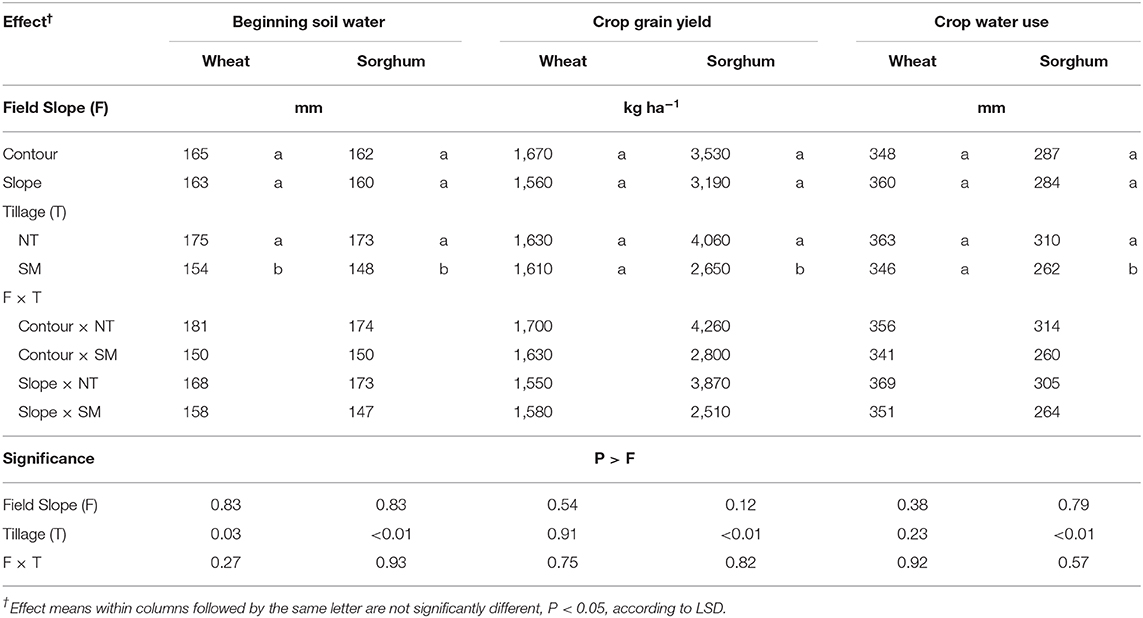
Table 4. Mean initial soil water, mm, grain yield, kg ha−1, and water use, mm, of wheat and sorghum grown on contour farmed or sloping land with stubble-mulch tillage (SM) or no tillage (NT) and their interaction with the corresponding ANOVA significance levels.
Our plots of the wheat grain yield as a function of exceedance probability (Figure 2) revealed that for NT the median yield was 1,750 kg ha−1 for contour farming or about 400 kg greater than with-slope farming at 1,310 kg ha−1. The NT wheat grain yields exceedance probability for 2,000 kg ha−1 averaged 34% for contour and with-slope farming; however, contour farming exceeded 1,000 kg ha−1 77% of observed yields compared with 69% for the with-slope farming. Wheat yields for SM were similarly elevated by contour farming rather than with-slope farming. That is, poor yields that characterize water deficit stress performance were elevated by contour farming probably because of reduced runoff water losses. Corresponding NT and SM grain sorghum yields revealed no similar difference between contour and with-slope farming, but greater yield performance with water conserving NT indicated 60% of the yields exceeded 4,000 kg ha−1 compared with only 15–20% of the yields with SM tillage.
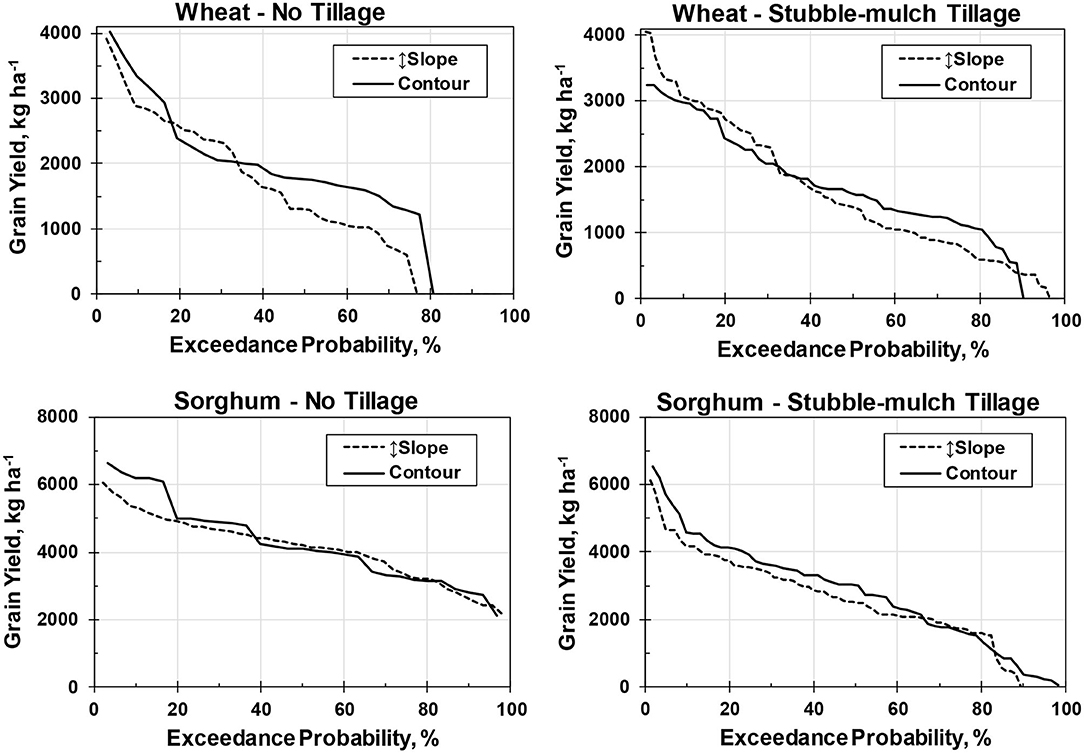
Figure 2. The with-slope (↕Slope) or contour farming grain yield, kg ha−1, of wheat and sorghum for no and stubble-mulch tillage plotted as a function of exceedance probability.
Discussion
Our objective was to quantify the effect of contour farming on stormwater runoff, soil water at planting, and the yield of wheat and sorghum grown in the 3 years wheat-sorghum-fallow (WSF) rotation. To this end, runoff was measured from gauged terraced and contour farmed fields managed in the wheat sorghum fallow rotation with NT or SM tillage beginning in 1983. The data revealed stormwater runoff interaction with tillage and landscape characteristics that in turn governed crop water use and yield. Terracing and contour farming water conservation practices first applied this way during the US “Dust Bowl” were revisited since they could be adapted for dryland crop production on the semi-arid Great Plains as it eventually replaces irrigated cropping systems. The overall goal will become optimizing cultural practices to reduce runoff that limits water availability for dryland crop production.
Runoff, Landscape Slope, and Tillage
The exclusive source of water for dryland crop production is provided by precipitation that does not leave the field as runoff. Results in Table 1 reveal practically negligible cumulative runoff from fields in the growing wheat or sorghum rotation phases but demonstrated that, during the intervening 10 to 11-months fallow periods, the measured runoff from contour farmed terraces increased significantly as the landscape slope increased from 1.2 to 1.8%. Likewise, slope effects on the average measured runoff during the growing wheat or sorghum rotation phases were either negligible or not significantly different (respectively). Runoff, however, was consistently greater with NT than SM tillage during rotation fallow periods after wheat and sorghum, a negative concern for dryland management. Rain infiltration typically increases in the presence of a residue cover provided by NT management and these runoff data, at first glance, appear to contradict that accepted norm. When the soil initial conditions are considered, both higher initial soil water content and the degree of soil surface consolidation or crusting probably reduced infiltration for increased runoff as reported elsewhere (Jones et al., 1994). Although cultural practices to correct NT related surface consolidation or crusting could be applied to increase infiltration, the infiltration reduction because of higher soil water content under NT management would suggest that the goal to improve water conservation succeeded.
Comparisons of the profile soil water after fallow or at planting in Table 2 were not different between the 1.2 and 1.8% slopes for any rotation phase except for wheat planting, but that exception is consistent with the idea that lower slopes capture more runoff. By contrast, the measured soil water for NT was significantly greater than SM tillage, averaging ~27 mm. This reduced water conservation with SM tillage could be attributed to soil disturbance and the reduced residue retained on the soil surface to intercept irradiant energy that increase evaporation. The absence of a significant interaction between the field slope and tillage practices on soil water further supports greater evaporation with SM tillage. Because NT residues intercept irradiant energy that drives evaporation, these data demonstrate that residue retaining management for controlling evaporation will have significant impact in semiarid dryland cropping systems. The principal benefit NT over SM (or reduced) tillage for many soils but especially a Pullman soil has long been to increase the profile soil water content for subsequent crop use (Unger and Wiese, 1979; Unger and Baumhardt, 1999; Nielsen et al., 2002; Baumhardt et al., 2017; Schlegel et al., 2018). In semiarid dryland cropping systems, those management practices that control evaporative losses of soil water are more critical, overall, to conserve water than reducing runoff.
We similarly compared the profile soil water amounts for the contour farmed terraces and the with-slope farmed plots at the secondary site as a means to corroborate the effects of tillage practices on soil water (Table 4). The beginning soil water for both wheat and sorghum were no different between the with-slope farmed field plots and contour farmed terraces, suggesting that runoff control did not improve stored soil water for a Pullman soil. Runoff was, nevertheless, a significant undesirable consequence of the with-slope farming practices based on occasional observations of soil erosion rivulets that required remediation. Significantly greater stored soil water with NT than with SM tillage averaged ~23 mm more for combined wheat and sorghum planting as likewise noted for the graded terrace watersheds. These results confirm the benefit of NT residue management to improve water storage in the soil.
Crop Yield and Water Use
Examination of wheat and sorghum yield responses to arguably subtle differences in landscape slope and contour farming generally indicated runoff reduction by lower slopes and contour farming translated into greater yields. For example, the graded terraces wheat yield for the 1.8% slope at 70% of the yield measured for the 1.2% slope was significantly lower as might be expected if more runoff was stored by the soil for crop use (Table 3). The corresponding water use was, however, not different (P = 0.09) possibly because evaporative losses would result in underestimated water use. Wheat yields and the estimated water use for water conserving contour farmed graded terraces did not differ significantly, however, from those yields and water use for with-slope farming despite possible greater runoff (Table 4). Contradicting the runoff conservation and wheat yield trends, the measured yield from the1.8% slope was a significantly lower 70% of the yield for the 1.2%, while grain sorghum yields for the contour farmed graded terraces were greater than sorghum yields of the with-slope farming.
Tillage may be one of the easier cultural practices to change because NT consistently maintained an advantage over SM in available soil water at planting. Wheat grain yields from the graded terraces, however, differed by only 50 kg ha−1 between the NT and SM tillage with no difference in water use. Wheat yields at the secondary site and those of the graded terraces were similar, differing by only 115 kg ha−1, and a NT and SM yield separation of only 20 kg ha−1. The absence of wheat yield differences due to tillage has often been reported (Jones and Popham, 1997; Schlegel et al., 1999; Baumhardt et al., 2017). Baumhardt et al. (2017) suggested the similar grain yields for wheat may be explained by greater deep drainage from NT than SM soils during the longer growing season. They showed the cumulative drainage offset much of the NT advantage in stored soil water and, when deducted from crop water use, practically equalized the calculated water use efficiency. At both sites, the sorghum grain yields for NT were significantly greater than with SM tillage. Sorghum water use did not, however, differ with tillage on the graded terraces. The yield difference of 1,400 kg ha−1 separating NT over SM at the with-slope farming site was roughly three times larger than at the graded terraces with significantly greater water use with NT.
Application
The dryland cropping system alternative to irrigated crop production will eventually be necessitated in the High Plains region overlying the non-recharging portion of the Ogallala aquifer due to depletion. Dryland crop production is far less forgiving than the irrigated counterpart because all available water is exclusively supplied by often erratic and variable precipitation during the growing season or stored during fallow. The hydrologic abstractions from surface runoff in agricultural fields are primarily evaporation, transpiration, infiltration into the soil for storage or deep drainage, and to a lesser degree detention storage. Finnell (1930) improved infiltration of rainfall into the soil by relying on temporary detention storage in order to meet crop transpiration demands and evaporation through the use of contour farmed terraces. He observed that terraces and contour farming would only be effective for conserving water when rain exceeded soil infiltration capacity to become a runoff liability.
Building on previously identified slope effects on event specific runoff (Dockal, 2019), this investigation determined that tillage and slope effects on cumulative rotation phase runoff were significant. That is, runoff from contour farmed terraces increased with higher slope and NT residue management, although the expected increase in soil water did not consistently increase as the runoff increased. This highlights the need for contours and terraces to improve soil surface detention storage to minimize runoff and control soil erosion (Jones et al., 1985; van Pelt et al., 2017). Despite more runoff with NT residue management than with SM tillage, the stored soil water was consistently greater for NT because residues reduced soil water evaporation by intercepting irradiant energy as described by Lascano and Baumhardt (1996). Increasing stored soil water with NT resulted in greater grain sorghum yields and represents a desirable management practice.
Data Availability Statement
The datasets generated for this study are available on request to the corresponding author.
Author Contributions
All authors were involved in designing, executing, and reporting the described research.
Funding
This research was funded by USDA-ARS under the research plan for CRIS project 3090-13000-15-00-D entitled Precipitation and Irrigation Management to Optimize Profits from Crop Production and contributes to research conducted under the ARS led Ogallala Aquifer Program.
Disclaimer
The mention of trade or manufacturer names is made for information only and does not imply an endorsement, recommendation, or exclusion by the USDA-Agricultural Research Service. The USDA is an equal opportunity provider and employer. The U.S. Department of Agriculture (USDA) prohibits discrimination in all its programs and activities on the basis of race, color, national origin, age, disability, and where applicable, sex, marital status, familial status, parental status, religion, sexual orientation, genetic information, political beliefs, reprisal, or because all or part of an individual's income is derived from any public assistance program (not all prohibited bases apply to all programs). Persons with disabilities who require alternative means for communication of program information (Braille, large print, audiotape, etc.) should contact USDA's TARGET Center at (202) 720-2600 (voice and TDD). To fi le a complaint of discrimination, write to USDA, Director, Office of Civil Rights, 1400 Independence Avenue, S.W., Washington, DC. 20250-9410, or call (800) 795-3272 (voice) or (202) 720-6382 (TDD). USDA is an equal opportunity provider and employer.
Conflict of Interest
The authors declare that the research was conducted in the absence of any commercial or financial relationships that could be construed as a potential conflict of interest.
The reviewer SW declared a shared affiliation, with no collaboration, with the authors to the handling editor at time of review.
References
Alesso, C. A., Cipriotti, P. A., Bollero, G. A., and Martin, N. F. (2019). Experimental designs and estimation methods for on-farm research: a simulation study of corn yields at field Scale. Agron. J. 111, 2724–2735. doi: 10.2134/agronj2019.03.0142
Barfield, B. J., Warner, R. C., and Haan, C. T. (1981). Applied Hydrology and Sedimentology for Disturbed Areas. Stillwater, OK: Oklahoma, 603.
Baumhardt, R. L., and Anderson, R. L. (2006). “Crop choices and rotation principles,” in Dryland Agriculture, 2nd Edn. eds G.A. Peterson, et al. (Agron. Monograph 23. ASA, CSSA, and SSSA, Madison, WI), 113–139.
Baumhardt, R. L., Johnson, G. L., and Schwartz, R. C. (2012). Residue and long-term tillage and crop rotation effects on simulated rain infiltration and sediment transport. Soil Sci. Soc. Am. J. 76, 1370–1378. doi: 10.2136/sssaj2011.0331
Baumhardt, R. L., and Lascano, R. J. (1996). Rain infiltration as affected by wheat residue amount and distribution in ridged tillage. Soil Sci. Soc. Am. J. 60, 1908–1913. doi: 10.2136/sssaj1996.03615995006000060041x
Baumhardt, R. L., Mauget, S. A., Schwartz, R. C., and Jones, O. R. (2016). El Niño Southern oscillation effects on dryland crop production in the texas high plains. Agron. J. 108, 736–744. doi: 10.2134/agronj2015.0403
Baumhardt, R. L., Schwartz, R. C., Jones, O. R., Scanlon, B. R., Reedy, R. C., and Marek, G. W. (2017). Long-term conventional and no-tillage effects on field hydrology and yields of a dryland crop rotation. Soil Sci. Soc. Am. J. 81, 200–209. doi: 10.2136/sssaj2016.08.0255
Baumhardt, R. L., Schwartz, R. C., MacDonald, J. C., and Tolk, J. A. (2011). Tillage and cattle grazing effects on soil properties and grain yields in a dryland wheat-sorghum-fallow rotation. Agron. J. 103, 914–922. doi: 10.2134/agronj2010.0388
Blanco-Canqui, H., and Lal, R. (2009). Crop residue removal impacts on soil productivity and environmental quality. Crit. Rev. Plant Sci. 28, 139–163. doi: 10.1080/07352680902776507
Brakensiek, D. L., Osborn, H. B., and Rawls, W. J. (1979). “Field manual for research in agricultural hydrology,” in USDA, Agriculture Handbook 224, eds B. Osborn and Rawls (Washington, DC: U.S. Gov. Print. Office).
Campbell, H. H. (1907). A Complete Guide to Scientific Agriculture as Adapted to the Semi-Arid Regions. (Lincoln, NE: The Campbell Soil Culture Co.), 320.
Dockal, J. R. (2019). Analysis of precipitation, storm runoff, and soil loss in dryland fields with conservation tillage. [Master's thesis]. West Texas A&M University, Canyon, TX, United States.
Duley, F. L. (1939). Surface factors affecting the rate of intake of water by Soils. Soil Sci. Soc. Am. Proc. 4, 60–64. doi: 10.2136/sssaj1940.036159950004000C0011x
Duncan, D., and Burns, K. (2012). The Dust Bowl: An Illustrated History. San Francisco, CA: Chronicle Books LLC.
Eck, H. V. (1969). Restoring productivity on Pullman silty clay loam subsoil under limited moisture. Soil Sci. Soc. Am. Proc. 33, 578–581. doi: 10.2136/sssaj1969.03615995003300040028x
Eck, H. V. (1988). Winter wheat response to nitrogen and irrigation. Agron. J. 80, 902–908. doi: 10.2134/agronj1988.00021962008000060013x
Eck, H. V., and Jones, O. R. (1992). Soil nitrogen status as affected by tillage, crops, and crop sequences. Agron. J. 84, 660–668. doi: 10.2134/agronj1992.00021962008400040025x
Evett, S. R., Schwartz, R. C., Tolk, J. A., and Howell, T. A. (2009). Soil profile water content determination: spatiotemporal variability of electromagnetic and neutron probe sensors in access tubes. Vadose Zone J. 8, 926–941. doi: 10.2136/vzj2008.0146
Finnell, H. H. (1930). The moisture saving efficiency of level terraces under semiarid conditions. J. Am. Soc. Agron. 22, 522–529. doi: 10.2134/agronj1930.00021962002200060006x
Griffin, T. W., Lambert, D. M., and Lowenberg-DeBoer, J. M. (2004). “Testing for appropriate on-farm trial designs and statistical methods for precision farming: a simulation approach,” in Proceedings of the 7th International Conference on Precision Agriculture and Other Precision Resources Management, ed D. J. Mulla (Minneapolis, MN: ASA, CSSA, and SSSA, Madison, WI), 1733–1748.
Hauser, D. L., van Doren, C. E., and Robbins, J. S. (1962). A comparison of level and graded terraces in the southern High Plains. Trans. Amer. Soc. Agric. Eng. 5, 75–77. doi: 10.13031/2013.40938
Hauser, V. L. (1968). Conservation bench terraces in Texas. Trans. Amer. Soc. Agric. Eng. 11, 385–386, 392.
Hauser, V. L., and Jones, O. R. (1991). Runoff curve numbers for the Southern High Plains. Trans. Amer. Soc. Agric. Eng. 34, 142–148. doi: 10.13031/2013.31636
Johnson, W. C., Skidmore, E. L., Tucker, B. B., and Unger, P. W. (1983). “Soil conservation: central great plains winter wheat and range region,” in Dryland Agriculture. (Agronomy Monograph No. 23). eds H. E. Dregne, and W. O. Willis (Madison, WI: ASA), 197–217.
Jones, O. R., Eck, H. V., Smith, S. J., Coleman, G. A., and Hauser, V. L. (1985). Runoff, soil, and nutrient losses from rangeland and dry-farmed cropland in the Southern High-Plains. J. Soil Water Conserv. 40, 161–164.
Jones, O. R., Hauser, V. L., and Popham, T. W. (1994). No-tillage effects on infiltration, runoff, and water conservation on dryland. Trans. Amer. Soc. Agric. Eng. 37, 473–479. doi: 10.13031/2013.28099
Jones, O. R., and Popham, T. W. (1997). Cropping and tillage systems for dryland grain production in the southern high plains. Agron. J. 89, 222–232. doi: 10.2134/agronj1997.00021962008900020012x
Lascano, R. J., and Baumhardt, R. L. (1996). Effects of crop residue on soil and plant water evaporation in a dryland cotton system. Theor. Appl. Climatol. 54, 69–84. doi: 10.1007/BF00863560
Lindstrom, M. J., Voorhees, W. B., and Onstad, C. A. (1984). Tillage system and residue cover effects on infiltration in North Western corn belt soils. J. Soil Water Conserv. 39, 64–68.
McGuire, V. L. (2017). “Water-level and recoverable water in storage changes, high plains aquifer, predevelopment to 2015 and 2013-15,” in U.S. Geological Survey Scientific Investigations Report 2017-5040, U.S. Geological Survey, (Reston, VA), 14.
Milliken, G. A., and Johnson, D. E. (2009). Analysis of Messy Data, Volume I: Designed Experiments, 2nd edn. New York, NY: Chapman and Hall. doi: 10.1201/EBK1584883340
Nielsen, D. C., Unger, P. W., and Miller, P. R. (2005). Efficient water use in dryland cropping systems in the great plains. Agron. J. 97, 364–372. doi: 10.2134/agronj2005.0364
Nielsen, D. C., Vigil, M. F., Anderson, R. L., Bowman, R. A., Benjamin, J. G., and Halvorson, A. D. (2002). Cropping system influence on planting water content and yield of winter wheat. Agron. J. 94, 962–967. doi: 10.2134/agronj2002.0962
Schlegel, A. J., Assefa, Y., Haag, L. A., Thompson, C. R., and Stone, L. R. (2018). Long-term tillage on yield and water use of grain sorghum and winter wheat. Agron. J. 110, 269–280. doi: 10.2134/agronj2017.02.0104
Schlegel, A. J., Dhuyvetter, K. C., Thompson, C. R., and Havlin, J. L. (1999). Agronomic and economic impacts of tillage and rotation on wheat and sorghum. J. Prod. Agric. 12, 629–636. doi: 10.2134/jpa1999.0629
Schwartz, R. C., Baumhardt, R. L., and Evett, S. R. (2010). Tillage effects on soil water redistribution and bare soil evaporation throughout a season. Soil Tillage Res. 110, 221–229. doi: 10.1016/j.still.2010.07.015
Schwartz, R. C., Baumhardt, R. L., Scanlon, B. R., Bell, J. M., Davis, R. G., Ibragimov, N., et al. (2015). Long-term changes in soil organic carbon and nitrogen under semiarid tillage and cropping practices. Soil Sci. Soc. Am. J. 79, 1771–1781. doi: 10.2136/sssaj2015.06.0241
Unger, P. W., and Baumhardt, R. L. (1999). Factors related to dryland grain sorghum yield increases: 1939 through 1997. Agron. J. 91, 870–875. doi: 10.2134/agronj1999.915870x
Unger, P. W., and Pringle, F. B. (1981). Pullman Soils: Distribution Importance, Variability, and Management. College Station, TX: Texas Agricultural Experiment Station.
Unger, P. W., and Wiese, A. F. (1979). Managing irrigated winter wheat residues for water storage and subsequent dryland grain sorghum production. Soil Sci. Soc. Am. J. 43, 582–588. doi: 10.2136/sssaj1979.03615995004300030030x
van Pelt, R. S., Hushmurodov, S. X., Baumhardt, R. L., Chappell, A., Nearing, M. A., Polyakov, V. O., et al. (2017). The reduction of partitioned wind and water erosion by conservation agriculture. Catena 148, 160–167. doi: 10.1016/j.catena.2016.07.004
Keywords: contour farming, no-tillage, stubble-mulch tillage, wheat, sorghum, fallow
Citation: Baumhardt RL, Dockal JR, Johnson GL, Brauer DK and Schwartz RC (2020) Controlling Stormwater Runoff That Limits Water Availability and Dryland Crop Productivity. Front. Sustain. Food Syst. 4:533687. doi: 10.3389/fsufs.2020.533687
Received: 09 February 2020; Accepted: 07 September 2020;
Published: 09 October 2020.
Edited by:
Stephen Machado, Oregon State University, United StatesReviewed by:
Jorge A. Zegbe, Instituto Nacional de Investigación Forestal, Agropecuaria (INIFAP), MexicoStewart Wuest, Agricultural Research Service (USDA), United States
Copyright © 2020 Baumhardt, Dockal, Johnson, Brauer and Schwartz. This is an open-access article distributed under the terms of the Creative Commons Attribution License (CC BY). The use, distribution or reproduction in other forums is permitted, provided the original author(s) and the copyright owner(s) are credited and that the original publication in this journal is cited, in accordance with accepted academic practice. No use, distribution or reproduction is permitted which does not comply with these terms.
*Correspondence: R. Louis Baumhardt, r.louis.baumhardt@usda.gov