- 1Centro de Citricultura Sylvio Moreira, Instituto Agronômico, Cordeirópolis, Brazil
- 2Centro de Ciências Agrárias, Departamento de Desenvolvimento Rural, Universidade Federal de São Carlos, Araras, Brazil
The management of soil cover plants (intercropping) in orchards can contribute to increase productivity of citrus trees. Thus, the present research aimed to evaluate different planting systems for Tahiti acid lime grafted onto Flying Dragon trifoliate orange, a dwarfing rootstock, at high planting density (1,157 trees ha–1). The study was set up in four tillage systems, using Urochloa ruziziensis as an intercrop species in the orchard, and conducted for 5 years: no-tillage (NT), no-tillage and no-herbicide (NT-NH), minimum tillage (MT) and conventional tillage (CT; without intercropping). Dry matter (DM) production of biomass in the row and interrow of the orchard was evaluated yearly, as well as weed density, soil physical and chemical characteristics, plant water and nutritional status, and fruit yield of trees. Greater deposition of DM of biomass was observed in the row of citrus planting for treatments NT and NT-NH compared to CT and MT treatments, which led to reduced undesirable weed populations. The NT treatment also provided increases of 79% in potassium (K) nutrient concentrations in the leaves of trees and 60% in exchangeable K in the soil surface layer, in the first 2 years evaluated. The maintenance of the Urochloa ruziziensis mulch in the NT system also provided higher soil volumetric moisture content and consequently lower soil resistance penetration and water stress on trees, evidenced by the predawn plant leaf water potential (<1 MPa). Moreover, the NT treatment provided an average increase of 56% of fruit yield from trees compared to the CT treatment during three harvests. This long-term study demonstrated the contribution of the no-tillage system using a favorable cover crop to increase the yield of Tahiti acid lime fruits and maintain the soil quality most required for the sustainability of citrus production.
Introduction
The increasing change in the lifestyle habits of the world’s population has led to changes in the food production situation. Concerns about CO2 emissions have increased, calling for low-carbon cultivation practices (Gregory and George, 2011). With the heightened consumer demand, the link between healthy food and the production system (agroecosystem) has been tightened. Consequently, there is a search for less-impacting production systems, making more conservationist, organic and even so-called urban farming techniques gain space in the world’s agricultural sphere (James and Friel, 2015).
Brazil stands out on the world stage as an agricultural producer, not only for being one of the largest producers, but also for the generation of new techniques and more-sustainable planting systems (Ribeiro et al., 2017). Among the foods most-produced in Brazil, citrus fruits stand out, the country being the world’s largest producer of oranges, the fifth in the ranking of mandarins and the sixth in limes and lemons. However, large variation on average productivity has been observed (Fundo de Defesa da Citricultura [Fundecitrus], 2019), which results from significant differences in the adoption of technology (planting and cultural practices) among producers in the different Brazilian citrus fruit regions (Corá et al., 2019).
The state of São Paulo accounts for 79% of Brazil’s production of limes and lemons, with a production of 1.3 million tons, with the highlight being Tahiti acid lime [Citrus latifolia (Yu Tanaka) Tanaka], with 30.4 thousand hectares planted and nine million productive trees (IBGE, 2018). It is important to highlight the socio-economic impact for the state, since this production area is concentrated in the hands of eight thousand small producers, who are the most in need of sustainable planting and cultural practices for remaining in business (Santos et al., 2016).
In this context, the implementation of the FAO’s Conservation Agriculture (CA) concept, involving (i) minimum soil tillage, (ii) cover crop usage, and (iii) diversity of cultivated species, in sequence (crop rotation) or in association (intercropping) (Food and Agriculture Organization [FAO], 2017), is key to the success of the agricultural activity.
Earlier studies demonstrate the possibility of using minimum soil tillage for the implantation of orange orchards (Auler et al., 2008; Fidalski et al., 2010), keeping the inter-rows permanently planted with cover crops (Urochloa spp.) after the establishment of the orchard (Martinelli et al., 2017). This kind of management is not usual in areas of the conventional tillage system, where the soil is exposed to sunlight and raindrops, increasing erosion. Minimum tillage and no-tillage provide for the accumulation of organic material and carbon content in the soil, for stabilizing its structure, besides cycling nutrients and contributing to the control of weeds (Trumbore and Camargo, 2009).
Two techniques have been highlighted as alternatives for the conservationist production of citrus fruit, replacing the conventional system. The secondary cultivation of species of perennial brachiaria is highlighted, mainly of the Poaceae family [Urochloa (syn). Brachiaria] in the orchards (Matheis et al., 2006), and the handling of this vegetation with the use of the ecological mower. This mower is characterized by cutting the inter-row vegetation and distributing it simultaneously on the row of citrus planting, producing a mulching effect (Corá et al., 2019).
The production of biomass of covering plants in the inter-rows of citrus groves and the launch of it for citrus row planting was the target of a few short-term studies with citrus fruit (a few harvests), focusing on specific evaluations of different species of cover crop, water storage capacity and control of weeds (Bremer Neto et al., 2008; Fidalski et al., 2009; Azevedo et al., 2012; Martinelli et al., 2017). However, the no-tillage and mulching effects on the rows of citrus over a long period of time were not evaluated in these studies, not even the possible responses to new rootstocks, such as the dwarfing varieties, which may differ in the consumption and absorption of water in the soil.
The diversification of rootstock in citriculture has occurred with great speed, with one of the objectives being varieties that induce less vigor to the canopy, thus allowing for denser planting in citriculture (Azevedo et al., 2015). Rootstock and scion with low vigor are essential for achieving better results in high-density planting systems, and mechanical harvest, and one of the dwarfing rootstocks that allows for greater density of planting is the trifoliate orange Flying Dragon [Poncirus trifoliata var. monstrosa (T. Ito) Swingle] (Cantuarias-Avilés et al., 2012). However, this rootstock shows low tolerance for water deficit, which justifies studies of alternative planting and cultural practices to increase the quality of the soil, as regards the storage of water from in the soil and fertility characteristics.
On the basis of the above, this research had the objective of assessing different systems of planting and cultural practices for Tahiti acid lime grafted onto Flying Dragon as a high-density planting orchard, considering the use or non-use of Urochloa ruziziensis as intercropping, and the consequent production and transfer of biomass from the inter-rows to the row of citrus planting, on the physical and chemical characteristics of the soil, over a long time period, which may affect the fruit production of the orchard.
Materials and Methods
Growing Conditions and Experimental Design
The experiment was installed in São Paulo State, Brazil (22° 18′ 26″ S; 47° 23′ 11″ W and 620 m altitude). The climate of the region, according to Köppen, is of the Cwa type, tropical altitude, rainy in summer and dry in winter, with annual rainfall of 1,384.5 mm (Alvares et al., 2013). The soil has been classified as a dystrophic Red Latosol, with clay texture, with a moderate A horizon (Empresa Brasileira de Pesquisa Agropecuária [EMBRAPA], 2013). During the experimental period (2015–2019), maximum and minimum temperatures and the monthly rainfall accumulated were monitored, with data collected at a meteorological station located 1 km from the experimental area.
The initial chemical characteristics of the soil from the experimental area were evaluated (Raij et al., 2001) for a soil sample collected in July 2014 (0.0–0.20 m depth). The following average levels were found: P (phosphorus; 51.5 mg dm–3), K (potassium; 3.7 mmolc dm–3), Ca (calcium; 52 mmolc dm–3), Mg (magnesium; 17 mmolc dm–3), and base saturation (68%). These values are considered suitable for planting citrus fruit (Corá et al., 2019; Mattos-Junior et al., 2020). In November 2014, Crotalaria juncea and Urochloa ruziziensis were sown, 30 and 12 kg ha–1 of seeds, respectively, with the aim of increasing soil carbon and nitrogen, in total area.
In March 2015, when the cover crops exhibited maximum growth (Figure 1A), they were cut. The furrows were opened and 0.45 kg of monoammonium phosphate (MAP) was applied per linear meter of the furrow (Figure 1B). U. ruziziensis was kept as a permanent covering crop (Figure 1C) in the orchard inter-rows, in minimum tillage (MT), no-tillage (NT) and no-tillage + no-herbicide (NT-NH) treatments (Table 1).
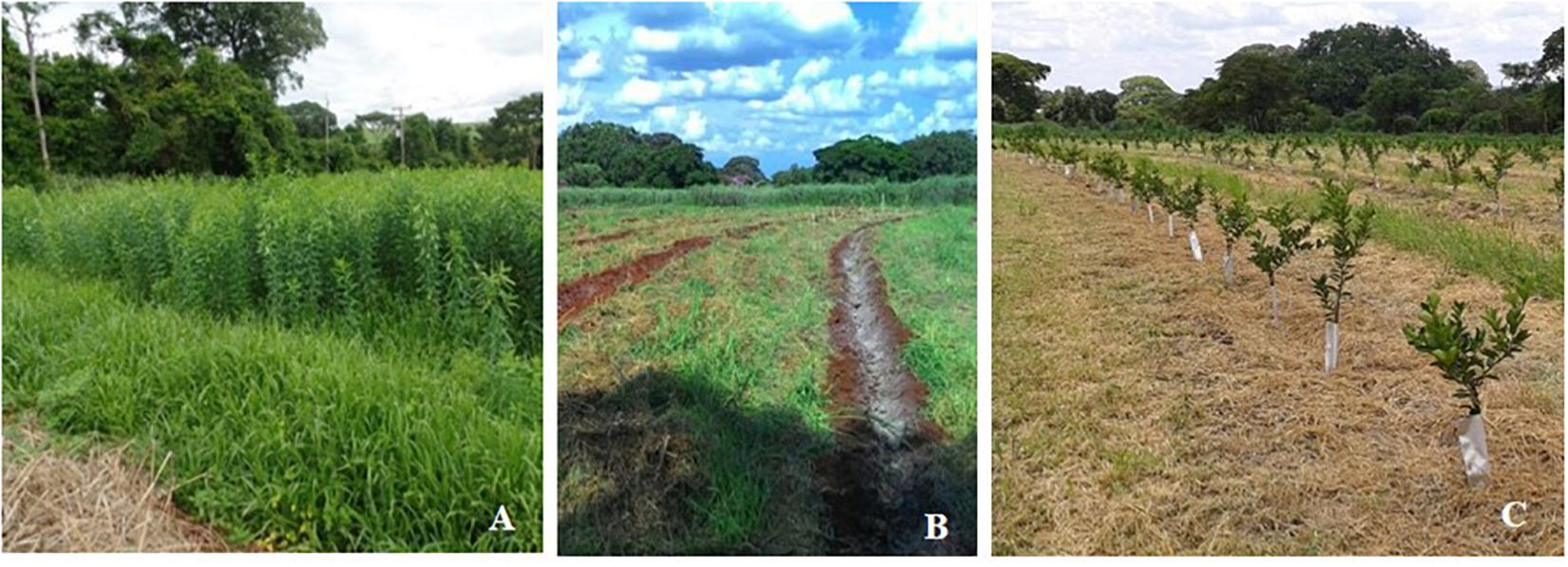
Figure 1. Crotalaria juncea and Urochloa ruziziensis at the most vegetative development, before the cut, February 2015 (A); opening of furrows for planting in no-tillage system, March 2015 (B); and plants of Tahiti acid lime in no-tillage system, October 2015 (C).
The planting of Tahiti acid lime IAC-5, grafted onto Flying Dragon trifoliate orange, was carried out in March 2015, using a spacing of 4.8 m × 1.8 m (1,157 plants ha1). The treatments were as follows: conventional tillage (CT), minimum tillage (MT), no-tillage (NT) and no-tillage + no-herbicide (NT-NH). Different planting operations and cultural practices were performed on each treatment (Table 1). The experiment was installed with four treatments in a randomized block design, with five replications. Each plot was composed of 18 trees, distributed in three rows with six trees each and the evaluations were concentrated on the four central trees of the central row.
The orchard was maintained without irrigation, and the fertilization followed the recommendations by Mattos-Júnior et al. (2009), which were carried out manually, subdivided into four applications from November to March of each harvest. In addition, 2 t ha–1 of limestone was applied in 2014 (before planting), 2016 and 2018, over the total area. The other routine treatments for culture (control of pests and diseases, pruning, etc.) were carried out on all the plots, annually.
Biomass Production
In all the treatments, three mowing of the cover crops were carried out per year, during 2016 to 2019. The inter-rows of the NT and NT-NH treatments were managed with an ecological mower (Kamaq® Ninja Eco 230 model, with six blades and a 2.60-m cutting width, that throws the mowed biomass into the row area), while the MT and CT treatments have been managed with a conventional mower (Kamaq® Ninja 230 model, with four blades and a 2.60-m cutting width, that leaves the mowed biomass in the inter-row area).
After the mowing (all treatments) and manual hoeing in the rows (NT-NH), biomass sampling was carried out on the orchard rows and inter-rows of the orchard, in all treatments, in four points per plot, using a gage area of 0.25 m2. The samples were weighed and dried at 65 ± 2°C for 72 h.
Weed Density
Thirty days after each treatment management (mowing, herbicide and hoeing, according Table 1), the plant protection survey was carried out on all treatments in the four evaluation years (2016 to 2019). An area of 0.25 m2 was randomly evaluating four times in the plot, totaling a sample area of 1 m2 in the citrus planting rows, as described by Martinelli et al. (2017). Weeds (including Urochloa ruziziensis) were counted to obtain the number of individuals per square meter (density).
Soil Penetration Resistance and Soil Moisture
The soil penetration resistance (SPR) was assessed using the Stolf penetrometer in the citrus rows. The measurement was obtained by a calculation using the number of impacts of a known weight driving into the soil and the depth measurement achieved by each impact, according to the methodology described by Stolf et al. (2014). Four points per plot (citrus row) were analyzed in the layers of 0–20 and 20–40 cm in depth, in September of the second (2016) and fourth (2018) years of the experiment, a period of low rainfall (Figure 2).
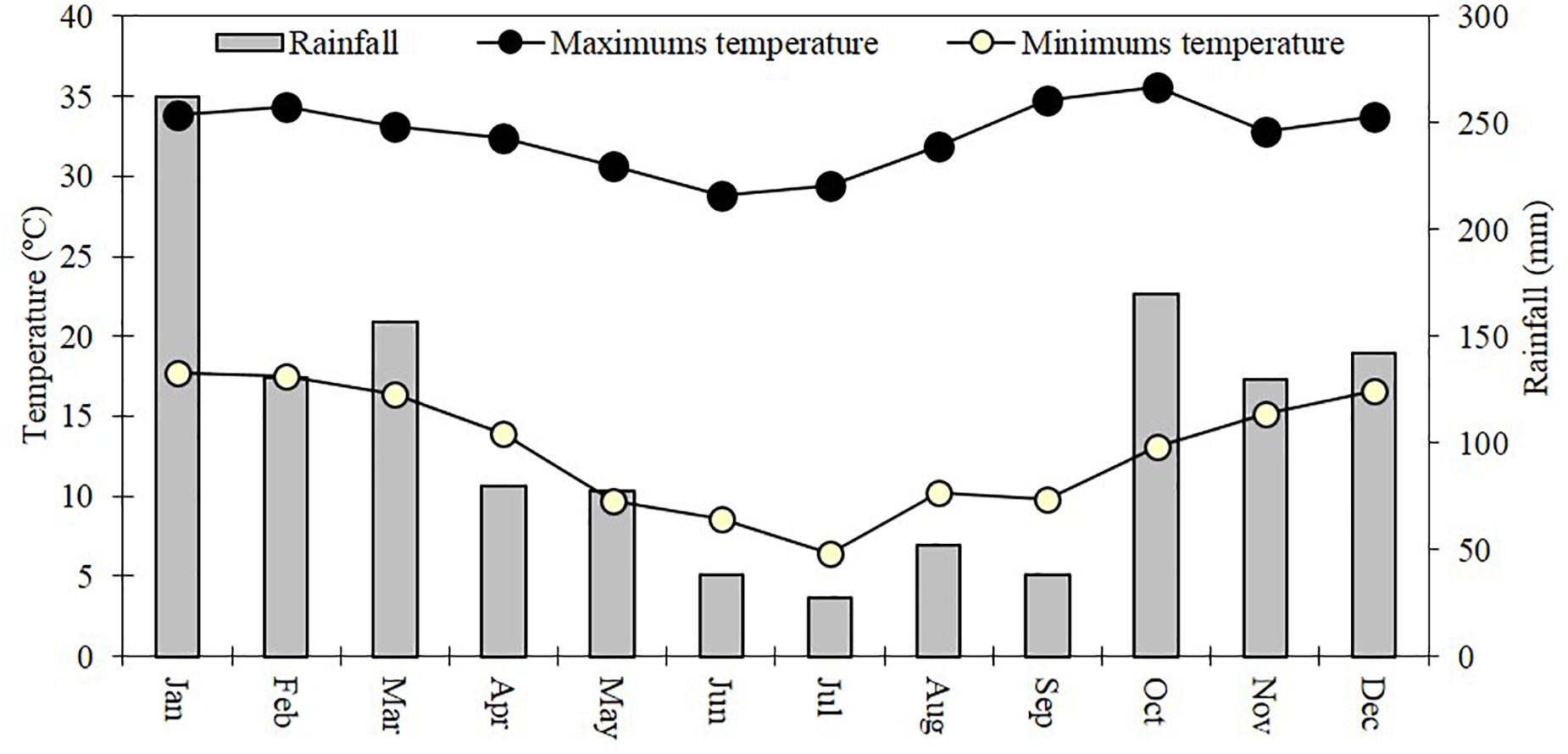
Figure 2. Mean values of air temperature minimums and maximums and of rainfall across the five growing seasons (2015–2019) in which the experiment was conducted.
On the same dates, the soil moisture was determined by the gravimetric method (Empresa Brasileira de Pesquisa Agropecuária [EMBRAPA], 2018), by taking four samples from the row planting, at depths of 0–20 and 20–40 cm, with the aid of an auger. The soil samples collected were packed in aluminum capsules and weighed before and after drying at 105 ± 3°C for 24 h. By difference in mass, the percentage of soil moisture was calculated using the formula:
Where SM is the soil moisture as a percentage of water, WW is the wet weight and DW is the dry weight of the sample.
Leaf Water Potential
Leaf water potential (ΨL) was measured on clear days during the dry season (August to September, Figure 2) annually from 2017 to 2019. Measurements were performed using a pressure chamber (Soil Moisture Equipment Corp., model 3000, Santa Barbara, CA, United States) according to Kauffmann (1968) and following the recommendations of Turner (1988). Sampling was performed at predawn (05:00 AM), collecting the third leaf in reproductive branches, four trees per plot. Leaves were collected and preserved in hermetic plastic bags on ice until measurement. At the same time soil samples (0–20 cm) were collected and soil moisture evaluated, according Empresa Brasileira de Pesquisa Agropecuária [EMBRAPA] (2018).
Chemical Analysis of Soil and Leaves
Each year in April (2016–2019), with the use of “Dutch”-type auger, eight soil subsamples per plot, were collected, in the citrus rows, in the layer of 0–20 cm depth to determine the availability of phosphorus (P), potassium (K), calcium (Ca), and magnesium (Mg), using the methods described by Raij et al. (2001). On the same date, sample leaves were collected for the assessment of the nutritional status of the trees, with the determination of total levels of macronutrients according to Bataglia et al. (1983). The third leaf of fruit branches was collected, with four trees being sampled per plot and five leaves per plant.
Vegetative and Productive Development of Tahiti Acid Lime
The vegetative growth of the Tahiti acid lime plants was assessed annually by measuring the height and diameter of the trees using a graduated ruler, for the subsequent calculation of the canopy volume by the equation proposed by Mendel (1956):
Where CV is the canopy volume (m3), r is the radius (m) and H is the height (m) of Tahiti acid lime plants. In each month of the period 2017–2019, all ripe fruit were harvested and weighed and then the cumulative productivity of each year was calculated, expressed in t ha–1. All evaluation were performed in four central trees per plot and in five replications.
Statistical Analysis
For the statistical analysis, all data were analyzed separately for each growing season, subjected to ANOVA. When the effects were significant, differences among treatments were determined using Tukey’s multiple comparison test at a significance level of 5%.
Results
Biomass of Cover Crops and Weed Density
In the conventional (CT) and minimum-tillage (MT) treatments, where the planting was carried out without the presence of mulch in the citrus rows and the biomass of the inter-rows was managed with a conventional mower, a greater quantity of biomass of the covering plants was deposited in the inter-rows of the Tahiti acid lime orchard (Table 2).
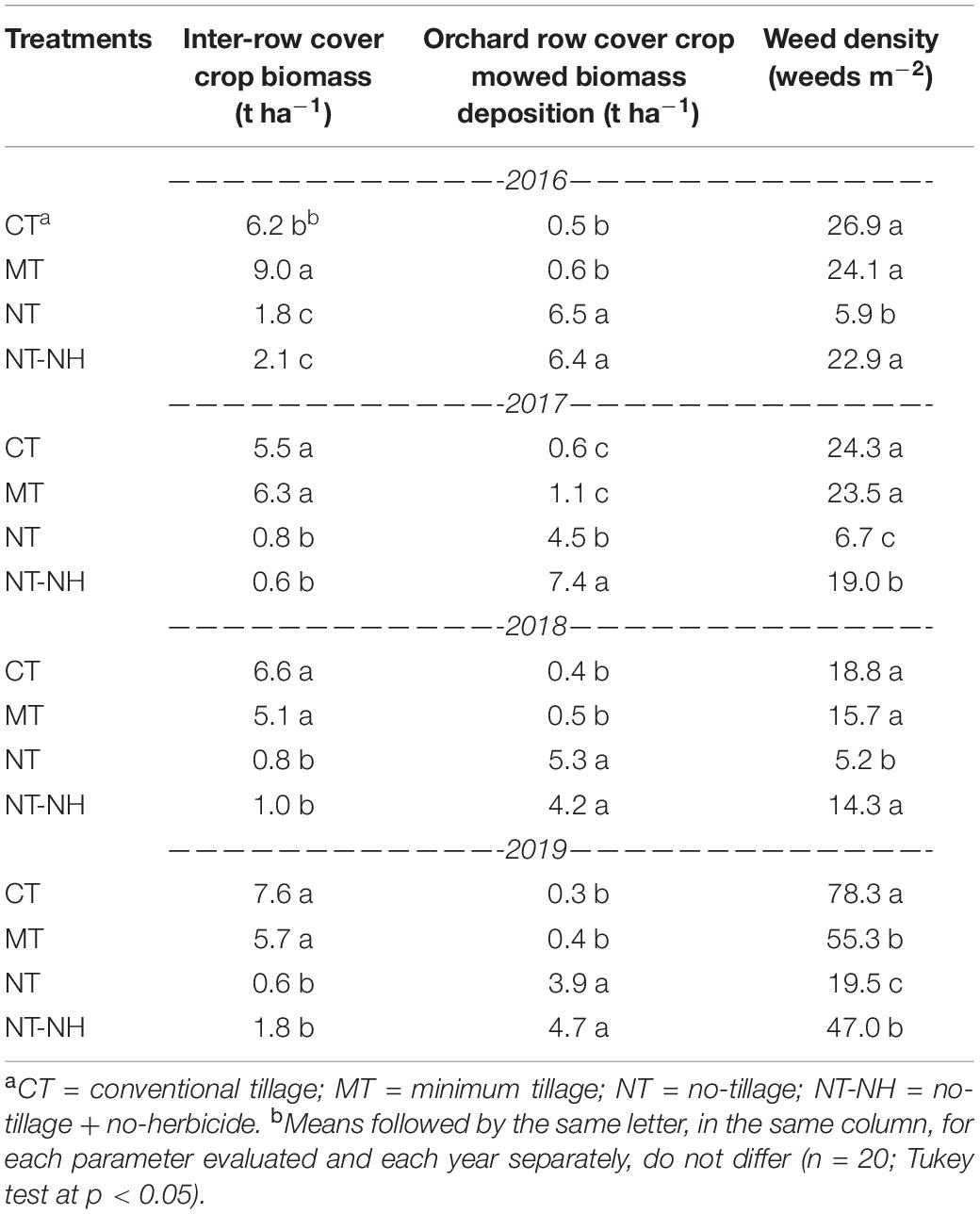
Table 2. Inter-row cover crop biomass yield, orchard row cover crop mowed biomass deposition and weed density, in four different planting systems of Tahiti acid lime, over four growing seasons (2015–2019).
On the other hand, in no-tillage systems, whether with or without herbicide, where the ecological mower was used on the inter-rows, higher biomass values were observed in the orchard row, with 10-fold increases in these treatments (NT and NT-NH) compared to CT and MT (Table 2); these results were similar over four consecutive years (2015–2019).
In NT treatment, the presence of mulch and the use of the herbicide glyphosate, two weed control methods (physical and chemical) in the citrus rows led to a significant reduction in the number of weeds in all years evaluated (Table 2). The average reduction in the number of weeds in the NT in relation to the CT treatment was 75%, reaching close to 80% in the first year evaluated, coming from the use of the herbicide and from the high values of biomass present in the row in this year (Table 2).
Resistance to Soil Penetration and Soil Moisture
With the newly planted experiment (2016), the penetration resistance values (0–20 cm depth) did not differ between treatments and remained below 2 MPa (Figure 3A). On the other hand, in the fourth year (2018), at the same depth, these values doubled in some treatments (NT and NT-NH) and quadrupled in conventional tillage (>8 MPa) (Figure 3A). Value greater than 10 MPa (Figure 3B) of resistance to penetration in the soil was observed in the CT treatment at the deeper layer (20–40 cm depth).
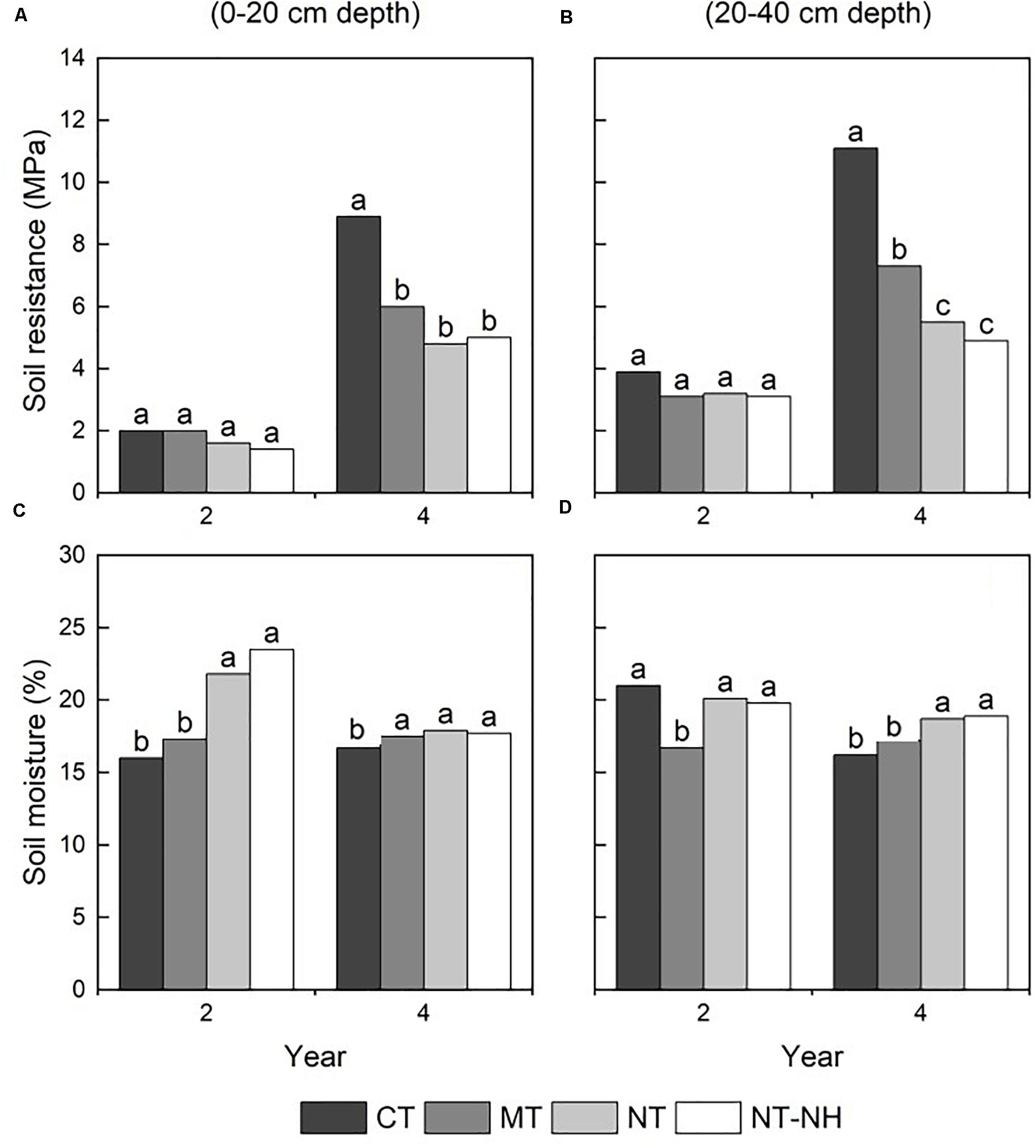
Figure 3. Soil resistance penetration (A), 0–20 cm depth and (B), 20–40 cm depth) and soil moisture (C) 0–20 m depth and (D) 0–20 m depth) in four different planting systems of Tahiti acid lime, over two growing seasons (2016 and 2018). CT, conventional tillage; MT, minimum tillage; NT, no-tillage; NT-NH, no-tillage + no-herbicide; Years: 2 = 2016 and 4 = 2018. Means followed by the same letter, for each evaluating and year separately, do not differ (n = 20; Tukey test at p < 0.05).
The soil moisture, taken together with resistance to penetration, was greater in mulch treatments at a depth of 0–20 cm in the second and fourth years (Figure 3C), however, in the 20–40 cm layer, the CT treatment showed lower soil moisture than the NT and NT-NH treatments in the second year, and the NT and NT-NH treatments had the highest moisture values in the fourth year (Figure 3D).
Leaf Water Potential and Soil Moisture
The leaf water potential was higher in the no-tillage (NT) treatment, in the third- and fourth-year assessments (Figure 4A), demonstrating a higher degree of hydration in the plants, even in low-precipitation periods of the year (Figure 2) when such assessments were carried out (September 2017, 2018, and 2019). The soil moisture, determined in parallel with the leaf water potential, was greater in the NT and NT-NH treatments than the other treatments, in all the years of evaluation (2017–2019) (Figure 4B).
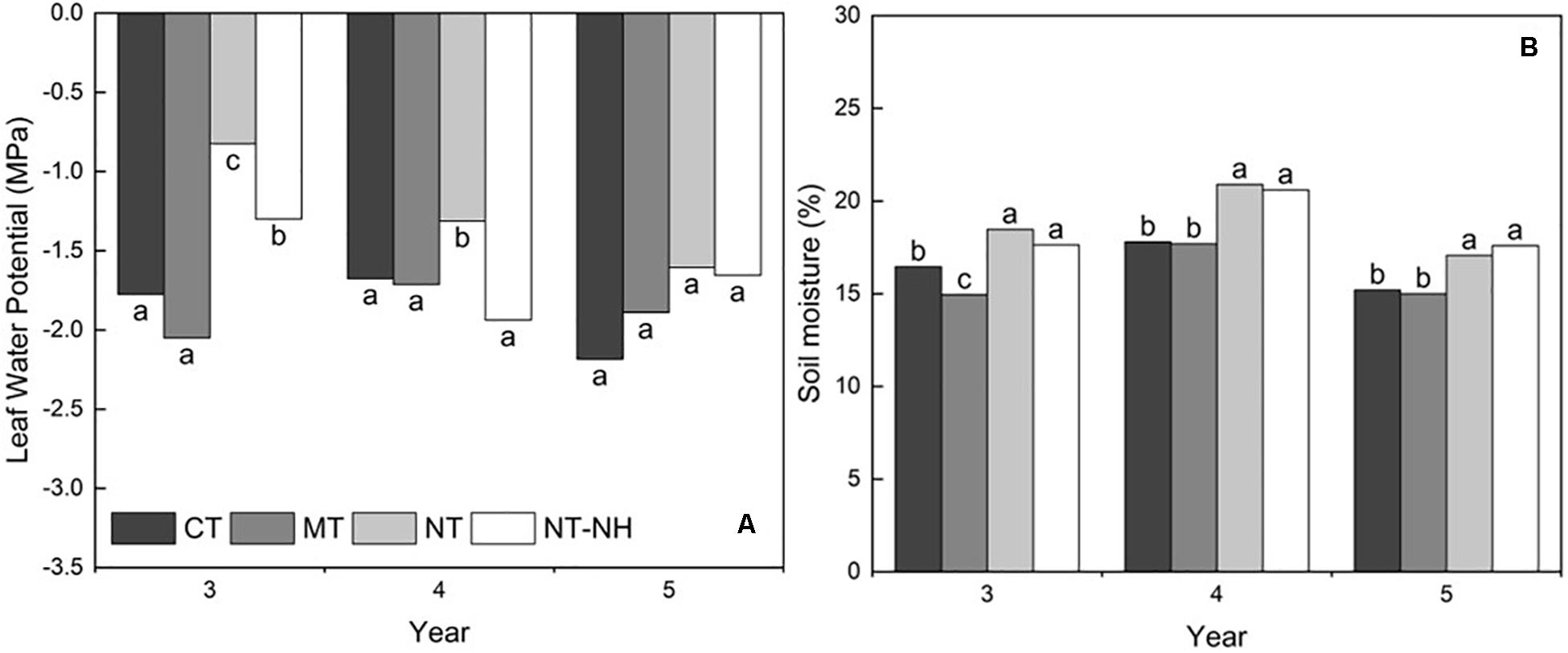
Figure 4. Soil moisture (A) and leaf water potential (B) in four different planting systems of Tahiti acid lime, over three growing seasons (2017–2019). CT, conventional tillage; MT, minimum tillage; NT, no-tillage; NT-NH, no-tillage + no-herbicide. Years: 3 = 2017, 4 = 2018, and 5 = 2019. Means followed by the same letter, for each evaluating and year separately, do not differ (n = 20; Tukey test at p < 0.05).
Soil Fertility and Nutritional Status of the Tahiti Acid Lime
Phosphorus (P) levels in the soil were adequate (13–30 mg dm–3) (Mattos-Junior et al., 2020) for a young Tahiti acid lime orchard (<5 years), for all years evaluated, and there was no significant difference between treatments (data not shown). However, although the concentrations of potassium (K), calcium (Ca) and magnesium (Mg) in the soil did not differ between the treatments in the first year evaluated, they were found to have sufficient levels for the crop, resulted from liming and soil fertilization conducted before tree planting. In the fourth year, the K content in soil in treatment NT (6 mmolcdm–3) was higher than in the other treatments (Figure 5A). In the leaf analyses, the K content was higher in treatment NT in the second and third years than in conventional tillage without mulch (CT) (Figure 5B).
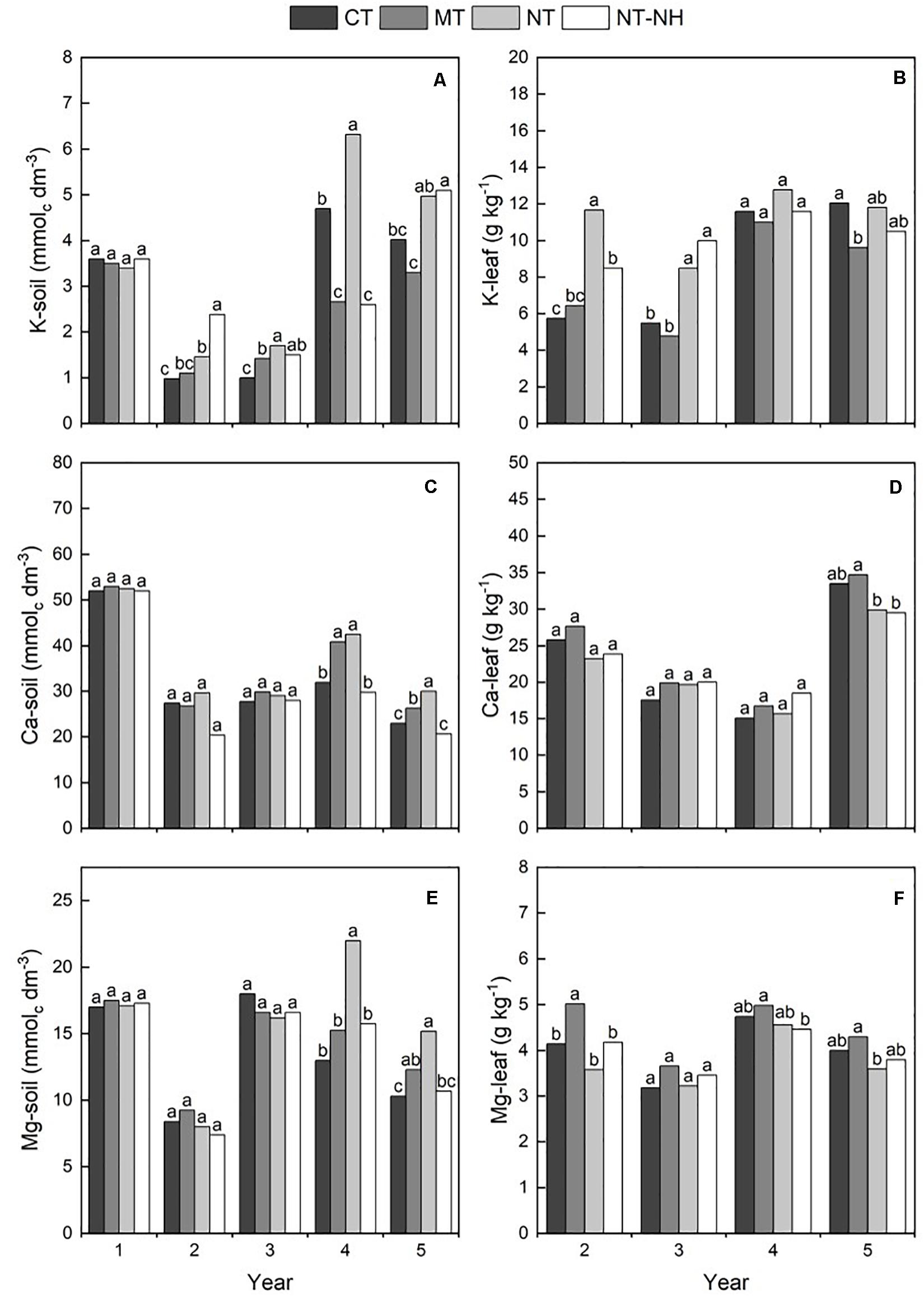
Figure 5. Nutrient soil availability (0–20 cm depth) and leaf content: K – (A,B), Ca – (C,D), Mg – (E,F) in the tree planting row of different planting systems of Tahiti acid lime, over five growing seasons (2015–2019). CT, conventional tillage; MT, minimum tillage; NT, no-tillage; NT-NH, no-tillage + no-herbicide. Years: 1 = 2015, 2 = 2016, 3 = 2017, 4 = 2018 and 5 = 2019. Means followed by the same letter, for each evaluating and year separately, do not differ (n = 20; Tukey test at p < 0.05).
Calcium and magnesium had higher levels, in soil, in NT treatment in years 4 and 5 compared to CT and NT-NH treatments (Figures 5C,E). No differences in leaf Ca levels were observed between years 2 and 4, however, there is a higher Ca content in MT compared to NT and NT-NH in the fifth year (Figure 5D). The leaf Mg content in MT was higher than in the other treatments in the second year (Figure 5F).
In the second year of the orchard (2016), the leaf N levels were higher in mulch treatments (NT and NT-NH) (data not shown). In all evaluation years, the levels were adequate for Tahiti acid lime (>25 g kg–1) in all treatments (Mattos-Junior et al., 2020). The soil organic matter content in treatment NT was higher than in the other treatments in years 4 and 5 (Figure 6A). Corroborating the K, Ca and Mg data in the soil, increased base saturation was attained in NT treatment (Figure 6B) in years 4 and 5 (75 and 58%, respectively). In year 1, high levels of base saturation (∼70%) were also observed, but in all the treatments in this case, as a result of the soil liming carried out before trees planting.
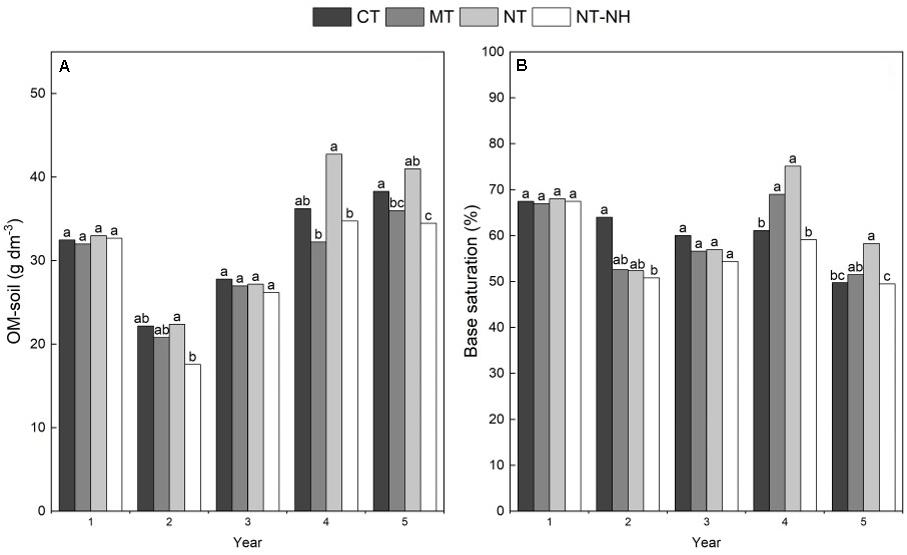
Figure 6. Organic matter (OM) (A) and base saturation (B) of soil in four different planting systems of Tahiti acid lime, over three growing seasons (2015–2019). CONV, conventional tillage; MT, minimum tillage; NT, no-tillage; NT-NH, no-tillage + no-herbicide. Years: 1 = 2015, 2 = 2016, 3 = 2017, 4 = 2018, and 5 = 2019. Means followed by the same letter, for each evaluating and year separately, do not differ (n = 20; Tukey test at p < 0.05).
Vegetative and Productive Development of Tahiti Acid Lime
The NT-NH treatment led to lower vegetative development for Tahiti acid lime plants in the second year (2016). The canopy volume in the CT treatment was lower than in the other treatments in the fifth year of evaluation (Figure 7A). Although the orchard is in the process of being formed, the results showed an early production of the Tahiti acid lime plants in high-density planting grafted to rootstock of Flying Dragon trifoliata orange, since the plots in the no-tillage system with application of herbicide (NT) produced more than 22 t ha–1 in the third year (2017). From the third to the fifth year of evaluation, the NT treatment showed a greater production than the other treatments, reaching 30% greater than the CT system in the last harvest (Figure 7B). Although the NT-NH treatment showed the lowest productivity (9.5 t ha–1) in the third year, this treatment did not differ from the CT and MT treatments in the fourth and fifth years (Figure 7B).
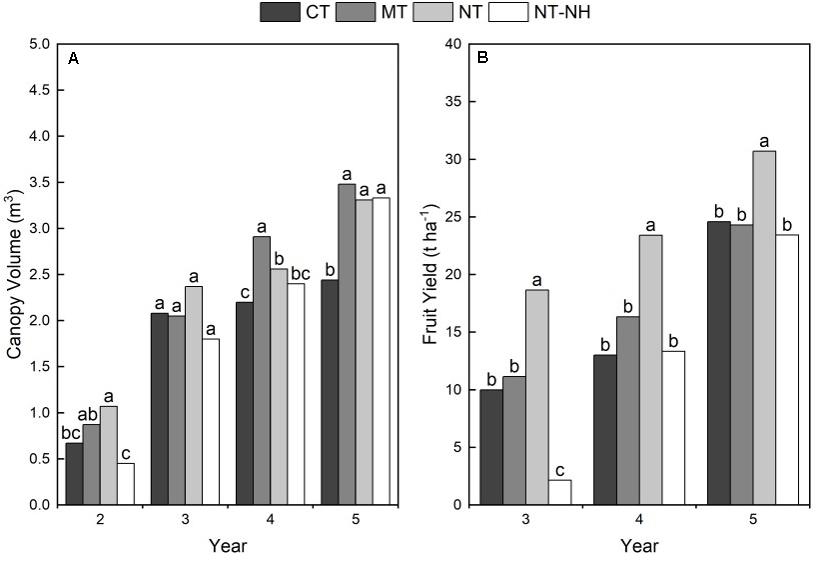
Figure 7. Canopy volume (A) and fruit yield (B) in four different planting systems of Tahiti acid lime trees, over three growing seasons (2017–2019). CT, conventional tillage; MT, minimum tillage; NT, no-tillage; NT-NH, no-tillage + no-herbicide. Years: 1 = 2015, 2 = 2016, 3 = 2017, 4 = 2018, and 5 = 2019. Means followed by the same letter, for each evaluating and year separately, do not differ (n = 20; Tukey test at p < 0.05).
Discussion
Biomass of Cover Crops and Density of Weeds
The largest quantity of biomass in the citrus row, in the NT and NT-NH treatments, was due from the planting carried out in the mulch (Figure 1). The subsequent maintenance of this mulch on the soil surface results from the management of the cover crops in the inter-rows (U. ruziziensis) with the use of an ecological mower. This agricultural equipment throws the mowed biomass into the row area; this is a sustainable management technique, providing greater soil protection and a reduction in the use of herbicides, as an aid in the control of weeds (Bremer Neto et al., 2008; Azevedo et al., 2012; Martinelli et al., 2017).
The presence of the U. ruziziensis as cover crop in the inter-rows of the NT, NT-NH and MT treatments produced quantities of approximately 7 t ha–1 biomass in these systems, on the rows or inter-rows of the orchard. These biomass values are similar to the results reported by other authors for the state of São Paulo and Mato Grosso, Brazil (Bauer et al., 2011; Torres et al., 2016). In spite of other species of Urochloa such as U. decumbens showing biomass production (>9 t ha–1) higher than that of U. ruziziensis, there are reports of U. decumbens. allopathy in citrus trees (Souza et al., 2006). Thus, the use of U. ruziziensis in the inter-rows of Tahiti acid lime orchards has been recommended, because it exhibits the least competition with citrus fruit (Martinelli et al., 2017).
The use of two methods of control, the physical, with the planting and maintenance of the mulch of U. ruziziensis, and the chemical, applying the herbicide glyphosate in the NT treatment, led to an efficient control of weeds (Table 2). This underlines the importance of using more than one control method; a fundamental premise for the integrated management of weeds for citrus fruit and other crops (Christoffoleti et al., 2007). On the other hand, the absence of herbicide use and the presence of mulch alone in the NT-NH treatment, and the use of glyphosate (chemical) alone in the MT and CT treatments, were not sufficient to maintain the density of weeds at low levels, as they use only one control method (Teasdale and Mohler, 2000).
The presence of the natural mulching of the soil in treatment NT likely influenced the density of weeds due to physical effects, as it limits the passage of light, hindering the germination of positive photoblastic seeds, creates a physical barrier for the emergence of such seeds and reduces the germination of seeds that need thermal amplitude to start the germination process (Noce et al., 2008).
The NT-NH treatment was inefficient in controlling weeds, which could lead to lower productivity in relation to treatments where weeds are controlled by the use of cover crop cuttings and herbicide (NT). However, good preparation of the soil and good handling of the soil with covering and mulching plants may be the most economical option for controlling weeds in comparison with the use of organic or chemical herbicides, electroshock or steam (Hiltbrunner et al., 2007).
Soil Penetration Resistance, Soil Moisture and Leaf Water Potential
The SPR in the second year evaluated (2 MPa) for all treatments resulted from good soil preparation for the planting of the orchard (Figures 3A,B). The conventional practices of grazing and gradient modify the structure of the soil, altering its properties, which reduces the resistance to penetration of the soil and improves the porosity of the soil and the capacity for water retention, but these benefits are temporary (Nkakini and Fubara-Manuel, 2012).
In the evaluations carried out in the fourth year, in both soil layers, there were lower resistance values to penetration of the soil in the NT and NT-NH management systems, in which the soil of the citrus row is covered by mulch, compared to those of CT (Figures 3A,B), with values close to 4 MPa. Values up to 4 MPa are considered adequate for root growth, demonstrating that did not adversely affect the physical structures of the soil (Hamza and Anderson, 2005). Lima et al. (2007) consider that SRP values between 2.6 and 5.0 MPa may indicate some problems in plant growth, mainly in the root system.
The two soil layers assessed in treatment CT yielded values greater than 8 MPa of SRP, therefore outside the appropriate range. Several studies showed that the plants tolerate higher resistance to penetration of the soil (above 4 MPa), but in areas with no-tillage systems. This is due to better structure of the soil and greater presence of continuous biopores in this system (Moraes et al., 2014), which is not the case in the CT treatment. The SRP depends on some specific factors, such as the handling of the area, texture, structure and mineralogy, depending highly on the soil moisture (Assis et al., 2009).
The greater moisture in the soil in the no-tillage systems compared to that in the CT, in the fourth year of evaluation, in the layer of 20–40 cm depth (Figures 3C,D), is associated with improvements in the soil structure, by the addition of organic material on the surface (Fidalski et al., 2010), resulting in lower resistance to penetration of the soil in these treatments (NT and NT-NH).
Proper management of soil cover crops is important in improving the soil structure and porosity, protecting it from erosion and increasing water infiltration, as well as improving the soil ability to sustain adequate moisture for plant growth (Melloni et al., 2008), which was observed in NT and NT-NH. Yoshida and Stolf (2019) report that when the soil is moister, its particles are further away from each other, which results in less difficulty in separating them, reducing the resistance to penetration of equipment into the soil.
In China, in apple orchard Liu et al. (2013) report that the covering of the soil with mulch has a positive effect on the conservation of the water content in the soil, besides the control of erosion and the loss of nutrients, such as P and K. Still in this context, according to Ahmad et al. (2020), the Chinese government has encouraged its citriculturists to recover degraded areas and to keep them productive by ways that prioritize the conservation of soil and water, including the use of organic mulching.
Although rainfall rates in August and September were low (Figure 2), treatment NT showed greater water potential of the leaf (about −1.1 MPa) compared to the other treatments (Figure 4A) in the third and fourth years, even using a rootstock highly susceptible to drought such as the Flying Dragon trifoliata orange (Espinoza-Núñez et al., 2011). Values for the predawn water potential should be less than −1.3 MPa in order to avoid negative effects on the final production of citrus fruit (García-Tejero et al., 2010).
Conventional and minimum tillage do not show mulching in the citrus row, due to the use of the conventional mower, keeping the citrus row exposed to the greater incidence of sunrays. As a result, the evaporation of water occurs more quickly, bringing about more-negative values of water potential in the leaves (∼−2 MPa). Reduced hydraulic conductance may be the main cause of the low vigor of some species used as anchoring rootstocks in citrus fruit, as is the case of the Flying Dragon. A lower amount of xylem of Flying dragon trifoliate orange, brings about a reduction in the capacity for transporting water from the roots to the leaves (Martínez-Alcántara et al., 2013).
The treatments that have covering of the soil by mulch (NT and NT-NH) allowed higher soil moisture values (Figure 4B). This occurs due to the protection that the cover crop provides, showing greater protection against the rays of the sun, besides reducing the effects of rain and water leaching, favoring the infiltration of water. Favarin et al. (2015) and Pedrosa et al. (2014) studying Urochloa spp. with intercropping to coffee, obtained a 49% increase in soil moisture resulting from the biomass left by cover crops. Furthermore, this biomass left by brachiaria can release high levels of N into the soil which can be used by the coffee crop.
Soil Fertility and Foliar Analysis
Higher levels of P are often reported in the surface layers of soil in the no-tillage system due to the application of surface fertilizers and non-revolving and the low mobility of P, in oxidic soil, to the deeper layers, in addition to the mineralization of the organic matter of the cover crop (Spera et al., 2011). However, the P levels in the soil and the leaves of Tahiti acid lime did not differ between the proposed planting systems (data not shown). Bremer Neto et al. (2008), working with management of a Pera sweet orange orchard [Citrus sinensis (L.) Osbeck], with and without mulch in the citrus row, also did not observe any differences in the levels of P in the soil. Ulén et al. (2010) and Raghothama and Karthikeyan (2005) emphasized the importance of maintaining vegetation cover on the planting row, since the availability of P is directly related to the aeration, moisture and temperature of the soil, factors influenced by the addition of phytobiomass in productive systems.
The highest concentration of K in the soil, found in the treatments with the presence of U. ruziziensis mulching on the citrus row, the NT and NT-NH (Figure 5A), is also reported for other fruit plants such as apple trees, using spontaneous vegetation as a cover crop (Oliveira et al., 2016), and in various no-tillage researches for maize cultivation, using a consortium of Urochloa spp. (Borghi et al., 2013). The use of Urochloa spp. in a system of agricultural and livestock integration makes it possible, for example, to absorb non-interchangeable forms of K and to subsequently liberate this nutrient in forms of greater availability, for the cultivation of corn (Garcia et al., 2008). Salton and Lamas (2011), assessing the quantity of macronutrients released by the decomposition of the U. brizantha mulch, observed the release of 100 kg ha–1 of K per year.
The release with K is greater in no-tillage systems because of characteristic mineral composition of mulch, enriched with K, as found in the present work (Table 2), since the mineralization of the K of the mulch can be relatively fast, and the nutrient remains almost entirely in the ionic form in the plant tissue and, consequently, the K is quickly released into the soil. This monovalent cation is not associated with any particular component structure in the plant tissue (Boer et al., 2008); the K released from mulch is in the soluble form, easily absorbed by the plants, resulting in rapid absorption (Zalamena et al., 2013), as observed for Tahiti acid lime in NT and NT-NH treatments (Figure 5B).
Calcium and Mg showed similar distribution to K in the soil (Figures 5C,E), result from the cycling of these nutrients in the treatment with mulching and herbicide (NT), leading to an increase in the soil levels in the fourth and fifth years after the planting of Tahiti acid lime, contributing to the increase in soil base saturation (Figure 6B). Oliveira et al. (2001) explained that Ca and Mg have a similar rate of release from the mulch, slower than that of K.
In the second year (2016), the levels of soil nutrients were low, probably because it was a less rainy year in Araras, São Paulo State, Brazil. In 2016 it rained 20% less (1,700 mm) than the average history for the region, which is a 1,500 mm. In February 2016, it rained only 50 mm, less than half the historical average. The low soil moisture, due to the scarcity of rain, negatively affects the availability of nutrients to plants and microorganisms in the soil (Cavagnaro, 2016).
Potassium was more concentrated in citrus plants in treatments with mulching than was Mg (Figures 5B,D,F). This is strong evidence that K is absorbed more rapidly, actively or by facilitated diffusion, and strongly competes with other cations such as those of Ca and Mg (Mattos-Junior et al., 2004; Quaggio et al., 2011; Medeiros et al., 2014).
Although soil Mg levels increased in the fourth and fifth years after planting in the treatments with mulching and herbicide (NT), this did not reflect the leaf levels of this nutrient. Cations such as Ca2+ and K+ compete and depress Mg absorption (Marschner, 2002); in the NT treatment high levels of K in the soil were observed, justifying this assumption of competition at the surface of the roots, and one evidence of competition between cations for absorption is that the sum total of cations in a plant is not very variable with an increase in the supply of a specific cation, since a reduction in the proportion of the other cations present generally occurs (Mengel and Kirkby, 2001).
The greater intake of N in the leaves of the Tahiti acid lime, observed in the NT treatment in the second year of evaluation (data not shown), was also observed by Oliveira et al. (2019) for apple trees, using biomass treatment of spontaneous plants covering the orchard planting row. Fidalski and Stenzel (2006) also observed an increase in the leaf levels of N in the Folha Murcha sweet orange grafted onto Rangpur lime, brought about by the covering of the inter-rows with the use of Urochloa brizantha. Liu et al. (2013) explain that, in addition to increasing the N levels, mulching also significantly reduces the loss of that nutrient by surface rainwater flow.
Vegetative and Productive Development
In the fifth year of evaluation, the canopy volume of the Tahiti acid lime was still small, due to the use of the Flying Dragon trifoliate orange, a dwarfing rootstock (Figure 7A), on the other hand, Martinelli et al. (2017) studying a vigorous rootstock, the Swingle citrumelo, reported values greater than 15 m3 at the same age (5 years), confirming the dwarfing effect of the Flying Dragon. This characteristic of the Flying Dragon makes it possible to manage high-density planting, bringing good results in productivity, without altering the quality of the fruit. For the small citrus fruit growers, this is important because they can increase their income by making better use of the land area and by the precociousness of the fruit production, anticipating the financial return (Azevedo et al., 2015).
The productivity of Tahiti acid lime in the NT treatment reached a value close to 30 t ha–1 in the fifth year (Figure 7 B), exceeding the Brazilian average of mature orchards, which is 25.6 t ha–1 (Food and Agriculture Organization [FAO], 2017). The lower fruit productivity in the CT system, in relation to NT, may be related to the field competition (Figure 7B and Table 2). The weed competition in the NT treatment was minimized by the presence of mulch and by the use of glyphosate, providing lower density of weeds in the citrus row, reducing competition for water and nutrients (Granatstein et al., 2014). Unfavorable productivity results are reported in no-conservative plantings, because of competition for water between citrus plants and the intermediate vegetation in periods of water stress, however, this is only observed when the handling of the covering plants is ineffective (Wright et al., 2003; Auler et al., 2008).
The differences in crop production reflect all the characteristics of the production systems used. Greater efficiency in productivity will depend on effective adaptation to the region’s soil and climate characteristics. There are great prospects for reducing the use of herbicides, but for this to occur it is essential to apply the correct handling of the litter. This will not only benefit the control of weeds but also a series of factors involved in the conduct of the culture, mainly reflecting on productivity (Gomes and Christoffoleti, 2008). Thus, the NT-NH treatment is promising, since it shows similar productivity to CT and MT (Figure 7B), but with the advantage of eliminating a chemical input (herbicide), so more sustainable.
The economic situation of Brazilian citriculture, with the presence of huanglongbing (HLB), the most important citrus disease, is very critical and requires high productivity for the producer to maintain profitability. It is necessary to adopt economically viable and efficient techniques to guarantee the longevity of the orchards, ranging from a suitable planting system to the high-density planting (Azevedo et al., 2015). For dense systems, the use of combinations of scion and rootstock producing smaller mature plant size is fundamental (Moreira et al., 2019). Maintaining the mulch on the planting rows contributes toward the adaptation of dwarfing rootstocks under non-irrigation conditions, due to a more favorable environment for roots grow that increase water and nutrient use efficiency and decreased problems with weeds, which, makes the system more economically profitable and environmentally sustainable. Increased productivity highlights the importance of these systems as sustainable alternatives for medium and small citrus growers.
Conclusion
The reported no-tillage (NT) system, including the correct management of intercropping Urochloa ruziziensis with the use of an ecological mower, and glyphosate in rows, has improved the physical and chemical properties of the soil, in comparison with conventional tillage. Thus, results lead to the conclusion that the NT system tested for Tahiti acid lime grafted onto Flying Dragon trifoliate orange rootstock is effective, increasing crop productivity, in conditions without irrigation.
Data Availability Statement
The raw data supporting the conclusions of this article will be made available by the authors, without undue reservation.
Author Contributions
FA, PC, and DM designed the experiment. AA, AP, FA, RA, PC, RL, and RM performed, collected, and analyzed data. DM, RB, and VD supervised assessment of nutrition and plant physiological traits. AA, DM, FA, PC, RB, RM, and VD critically revised and edited the final manuscript version. All authors discussed the results and commented on the manuscript.
Funding
This study was supported by the São Paulo Research Foundation (FAPESP, Brazil, #2014/21349-4, #2016/04944-1, #2016/19726-0, and #2017/24411-0). DM, FA, and RB acknowledged the National Council for Scientific and Technological Development (CNPq, Brazil) for fellowships granted.
Conflict of Interest
The authors declare that the research was conducted in the absence of any commercial or financial relationships that could be construed as a potential conflict of interest.
References
Ahmad, B. N., Mustafa, F. B., Yusoff, S., and Gideon, D. A. (2020). A systematic review of soil erosion control practice on the agricultural land in Asia. Int. Soil Wat. Cons. Res. 8, 1–31. doi: 10.1016/j.iswcr.2020.04.002
Alvares, C. A., Stape, J. L., Sentelhas, P. C., De Moraes Gonçalves, J. L., and Sparovek, G. (2013). Köppen’s climate classification map for Brazil. Meteor. Z. 22, 711–728. doi: 10.1127/0941-2948/2013/0507
Assis, R. L., Lazarini, G. D., Lanças, K. P., and Cargnelutti Filho, A. (2009). Avaliação da resistência do solo à penetração em diferentes solos com a variação do teor de água. Eng. Agric. 29, 558–568. doi: 10.1590/S0100-69162009000400006
Auler, P. A. M., Fidalski, J., Pavan, M. A., and Neves, C. S. V. J. (2008). Produção de laranja “Pêra” em sistemas de preparo de solo e manejo nas entrelinhas. Rev. Bras. Ciênc. Solo 32, 363–374. doi: 10.1590/s0100-06832008000100034
Azevedo, F. A., de Pacheco, C. A., Schinor, E. H., de Carvalho, S. A., and da Conceição, P. M. (2015). Produtividade de laranjeira folha murcha enxertada em limoeiro cravo sob adensamento de plantio. Brag 74, 184–188. doi: 10.1590/1678-4499.0374
Azevedo, F. A., de Rossetto, M. P., Schinor, E. H., Martelli, I. B., and Pacheco, C. D. E. A. (2012). Influência do Manejo da Entrelinha do Pomar na Produtividade da Laranjeira ‘Pera’. Rev. Bras. Frutic. 34, 134–142. doi: 10.1590/S0100-29452012000100019
Bataglia, O. C., Furlani, A. M. C., Teixeira, J. P. F., Furlani, P. R., and Gallo, J. R. (1983). Métodos de Análise Química de Plantas. Campinas: IAC.
Boer, C. A., Assis, R. L. d., Silva, G. P., Braz, A. J. B. P., Barroso, A. L. d. L., Cargnelutti Filho, A., et al. (2008). Biomassa, decomposição e cobertura do solo ocasionada por res duos culturais de tr s esp cies vegetais na regi o Centro-Oeste do Brasil. Rev. Bras. Cienc. Solo 32, 843–851. doi: 10.1590/S0100-06832008000200038
Borghi, E., Crusciol, C. A. C., Mateus, G. P., Nascente, A. S., and Martins, P. O. (2013). Intercropping time of corn and palisadegrass or guineagrass affecting grain yield and forage production. Crop Sci. 53, 629–636. doi: 10.2135/cropsci2012.08.0469
Bremer Neto, H., Victoria Filho, R., Mourão Filho, F. D. A. A., de Menezes, G. M., and Canali, E. (2008). Estado nutricional e produção de laranjeira “Pêra” em função da vegetação intercalar e cobertura morta. Pesq. Agrop. Bras. 43, 29–35. doi: 10.1590/S0100-204X2008000100005
Bauer, M. d. O., Pacheco, L. P. A., Chichorro, J. F., Vasconcelos, L. V., and Pereira, D. F. C. (2011). Produção e características estruturais de cinco forrageiras do gênero Brachiaria sob intensidade de cortes intermitentes. Ci. Anim. Bras. 12, 17–25. doi: 10.5216/cab.v12i1.4817
Cantuarias-Avilés, T., Assis, F., De Mourão, A., Sanches, E., Bremer, H., Rodrigues, S., et al. (2012). Rootstocks for high fruit yield and quality of ‘Tahiti’ lime under rain-fed conditions. Sci. Hortic. 142, 105–111. doi: 10.1016/j.scienta.2012.05.008
Cavagnaro, T. R. (2016). Soil moisture legacy effects: impacts on soil nutrients, plants and mycorrhizal responsiveness. Soil Biol. Biochem. 95, 173–179. doi: 10.1016/j.soilbio.2015.12.016
Christoffoleti, P. J., Carvalho, S. J. P., de López-Ovejero, R. F., Nicolai, M., Hidalgo, E., and da Silva, J. E. (2007). Conservation of natural resources in Brazilian agriculture: implications on weed biology and management. Crop Protect. 26, 383–389. doi: 10.1016/j.cropro.2005.06.013
Corá, J. E., Mattos, D. Jr., Boaretto, R. M., Azevedo, F. A., Zambrosi, F. C. B., et al. (2019). “Manejo do solo em cultivo de citros,” in Manejo e Conservação do Solo e da Água, ed. M. G. Viçosa, (Viçosa: Sociedade Brasileira de Ciencia do Solo), 907–934.
Empresa Brasileira de Pesquisa Agropecuária [EMBRAPA], (2013). Sistema Brasileiro de Classificação de Solos. Embrapa Solos. Brasília: EMBRAPA.
Empresa Brasileira de Pesquisa Agropecuária [EMBRAPA] (2018). Determinação Simplificada da Umidade do Solo Visando o Manejo de Irrigação em Hortaliças. Embrapa Hortaliças. Brasília: EMBRAPA.
Espinoza-Núñez, E., Mourão Filho, F., de, A. A., Stuchi, E. S., Cantuarias-Avilés, T., Dias, C. T., et al. (2011). Performance of “Tahiti” lime on twelve rootstocks under irrigated and non-irrigated conditions. Sci. Hortic. 129, 227–231. doi: 10.1016/j.scienta.2011.03.032
Favarin, J. L., Tezotto, T., Pedrosa, A. W., and Paula Neto, A. (2015). Coffee-forage intercropping is a sustainable production system for Brazil. Bet. Crops Plan. Food 99, 16–17.
Fidalski, J., Auler, P. A. M., Beraldo, J. M. G., Marur, C. J., de Faria, R. T., Barbosa, G. M., et al. (2010). Availability of soil water under tillage systems, mulch management and citrus rootstocks. Rev. Bras. Ciênc. Solo 34, 917–924. doi: 10.1590/s0100-06832010000300033
Fidalski, J., Barbosa, G. M., de, C., Auler, P. A. M., Pavan, M. A., and Beraldo, J. M. G. (2009). Qualidade física do solo sob sistemas de preparo e cobertura morta em pomar de laranja. Pesq. Agrop. Bras. 44, 76–83. doi: 10.1590/S0100-204X2009000100011
Fidalski, J., and Stenzel, N. M. C. (2006). Nutrição e produção da laranjeira “Folha Murcha” em porta-enxertos e plantas de cobertura permanente na entrelinha. Ciênc. Rural 36, 807–813. doi: 10.1590/S0103-84782006000300013
Food and Agriculture Organization [FAO] (2017). Conservation Agriculture. Available at: http://www.fao.org/ag/ca/$>$ (acessed June 09, 2019).
Fundo de Defesa da Citricultura [Fundecitrus] (2019). Tree Inventory and Orange Production Forecast for São Paulo and West-Southwestminas Gerais Citrus Belt – 2018–2019. Available at: https://www.fundecitrus.com.br/pdf/pes_relatorios/2019_05_24_Tree_Inventory_and_Orange_Crop_Forecast_2019-2020.pdf (accessed March 1, 2020).
Garcia, R. A., Crusciol, C. A. C., Calonego, J. C., and Rosolem, C. A. (2008). Potassium cycling in a corn-brachiaria cropping system. Eur. J. Agron. 28, 579–585. doi: 10.1016/j.eja.2008.01.002
García-Tejero, I., Jiménez-Bocanegra, J. A., Martínez, G., Romero, R., Durán-Zuazo, V. H., and Muriel-Fernández, J. L. (2010). Positive impact of regulated deficit irrigation on yield and fruit quality in a commercial citrus orchard [Citrus sinensis (L.) Osbeck, cv. salustiano]. Agric. Water Manag. 97, 614–622. doi: 10.1016/j.agwat.2009.12.005
Gomes, F. G., and Christoffoleti, P. J. (2008). Weed biology and management in no-tillage areas. Planta Daninha 26, 789–798. doi: 10.1590/S0100-83582008000400010
Granatstein, D., Andrews, P., and Groff, A. (2014). Productivity, economics, and fruit and soil quality of weed management systems in commercial organic orchards in Washington State, USA. Org. Agric. 4, 197–207. doi: 10.1007/s13165-014-0068-0
Gregory, P. J., and George, T. S. (2011). Feeding nine billion: the challenge to sustainable crop production. J. Exp. Bot. 62, 5233–5239. doi: 10.1093/jxb/err232
Hamza, M. A., and Anderson, W. K. (2005). Soil compaction in cropping systems: a review of the nature, causes and possible solutions. Soil Til. Res. 82, 121–145. doi: 10.1016/j.still.2004.08.009
Hiltbrunner, J., Liedgens, M., Bloch, L., Stamp, P., and Streit, B. (2007). Legume cover crops as living mulches for winter wheat: components of biomass and the control of weeds. Eur. J. Agron. 26, 21–29. doi: 10.1016/j.eja.2006.08.002
IBGE, (2018). Levantamento Sistemático da Produção Agrícola. Available at: https://sidra.ibge.gov.br/tabela/1613#resultado (acessad February 20, 2020).
James, S. W., and Friel, S. (2015). An integrated approach to identifying and characterising resilient urban food systems to promote population health in a changing climate. Public Health Nutr. 18, 2498–2508. doi: 10.1017/S1368980015000610
Kauffmann, M. R. (1968). Evaluation of the pressure chamber technique for estimating plant water potential in forest tree species. For. Sci. 14, 369–374.
Lima, C. L. R., Reichert, J. M., Reinert, D. J., Suzuki, L. E. A. S., and Dalbianco, L. (2007). Densidade crítica ao crescimento de plantas considerando água disponível e resistência à penetração de um Argissolo Vermelho distrófico arênico. Cienc Rural 37, 1166–1169. doi: 10.1590/S0103-84782007000400042
Liu, Y., Gao, M., Wu, W., Tanveer, S. K., Wen, X., and Liao, Y. (2013). The effects of conservation tillage practices on the soil water-holding capacity of a non-irrigated apple orchard in the Loess Plateau, China. Soil Til. Res. 130, 7–12. doi: 10.1016/j.still.2013.01.012
Martinelli, R., Monquero, P. A., Fontanetti, A., Conceição, P. M., and Azevedo, F. A. (2017). Ecological mowing: an option for sustainable weed management in young citrus orchards. Weed Tech. 31, 260–268. doi: 10.1017/wet.2017.3
Martínez-Alcántara, B., Rodriguez-Gamir, J., Martínez-Cuenca, M. R., Iglesias, D. J., Primo-Millo, E., and Forner-Giner, M. A. (2013). Relationship between hydraulic conductance and citrus dwarfing by the Flying Dragon rootstock (Poncirus trifoliata L. Raft var. monstruosa). Trees Struct. Func. 27, 629–638. doi: 10.1007/s00468-012-0817-1
Matheis, H. A. S. M., de Azevedo, F. A., and Victoria Filho, R. (2006). Adubação Verde no Manejo de Plantas Daninhas na Cultura de Citros. Laranja 27, 101–110.
Mattos-Junior, D., Davie, M., Kadyampakeni, D. M., Oliverc, A. Q., Boaretto, R. M., Morgand, K. T., et al. (2020). “Soil and nutrition interactions,” in The Genus Citrus, eds M. Talon, M. Caruso, and F. G. Gmitter, (Sawston: Woodhead Publishing).
Mattos-Júnior, D., Quaggio, J. A., Cantarella, H., and Boaretto, R. M. (2009). Citros: manejo da fertilidade do solo para alta produtividade. Info. Agron. 128, 5–12.
Mattos-Junior, D., Quaggio, J. A., Cantarella, H., and de Carvalho, S. A. (2004). Superfícies de resposta do tangor “Murcott” à fertilização com N, P e K. Rev. Bras. Frut. 26, 164–167. doi: 10.1590/s0100-29452004000100043
Medeiros, J., dos, S., de Oliveira, F. H. T., de Santos, H. C., de Arruda, J. A., and da Silva, M. S. (2014). Formas de potássio em solos representativos do estado da Paraíba1. Rev. Ciênc. Agron. 45, 417–426. doi: 10.1590/s1806-66902014000200025
Melloni, R., Melloni, E. G. P., Alvarenga, M. I. N., and Vieira, F. B. M. (2008). Avaliação da qualidade de solos sob diferentes coberturas florestais e de pastagem no Sul de Minas Gerais. Rev. Bras. Ciênc. Solo 32, 2461–2470. doi: 10.1590/s0100-06832008000600023
Mengel, K., and Kirkby, E. A. (2001). Principles of Plant Nutrition. Dordrechth: Kluwer Academic Publishers.
Moraes, M. T., de Debiasi, H., Carlesso, R., Franchini, J. C., and da Silva, V. R. (2014). Critical limits of soil penetration resistance in a rhodic Eutrudox. Rev. Bras. Ciênc. Solo 38, 288–298. doi: 10.1590/s0100-06832014000100029
Moreira, A. S., Stuchi, E. S., Silva, P. R. B., Bassanezi, R. B., Girardi, E. A., and abd Laranjeira, F. F. (2019). Could tree density play a role in managing Citrus Huanglongbing epidemics? Trop. Plant Path. 44, 268–274. doi: 10.1007/s40858-019-00284-1
Nkakini, S. O., and Fubara-Manuel, I. (2012). The effects of tillage methods on soil penetration resistance, porosity and okra yield. Res. J. Appl. Sci. Eng. Tech. 4, 387–392.
Noce, M. A., Souza, I. F., Karam, D., França, A. C., and Maciel, G. M. (2008). Influência da palhada de gramíneas forrageiras sobre o desenvolvimento da planta de milho e das plantas daninhas. Rev. Bras. Milho Sorgo. 3, 265–278. doi: 10.18512/1980-6477/rbms.v7n3p265-278
Oliveira, B. S., Ambrosini, V. G., Trapp, T., dos Santos, M. A., Sete, P. B., Lovato, P. E., et al. (2016). Nutrition, productivity and soil chemical properties in an apple orchard under weed management. Nutr. Cycle Agroecos. 104, 247–258. doi: 10.1007/s10705-016-9769-y
Oliveira, F. A., de Carmello, Q. A., and Mascarenhas, H. A. A. (2001). Disponibilidade de potássio e suas relações com cálcio e magnésio em soja cultivada em casa de vegetação. Sci. Agric. 58, 329–335. doi: 10.1590/S0103-90162001000200016
Oliveira, S. M., de Almeida, R. E. M., de Pierozan Junior, C., de Reis, A. F. B., Souza, L. F. N., and Favarin, J. L. (2019). Contribution of corn intercropped with brachiaria species to nutrient cycling. Pesq. Agrop. Trop. 49, 1–9. doi: 10.1590/1983-40632019v4955018
Pedrosa, A. W., Favarin, J. L., Luisa, A., and de Vasconcelos, S. (2014). Brachiaria residues fertilized with nitrogen in coffee fertilization. Cof. Sci. 9, 366–373. doi: 10.25186/cs.v9i3.650
Quaggio, J. A., Mattos-Jr, D., and Boaretto, R. M. (2011). Sources and rates of potassium for sweet orange production. Sci. Agric. 68, 369–375. doi: 10.1590/s0103-90162011000300015
Raghothama, K. G., and Karthikeyan, A. S. (2005). “Phosphate acquisition,” in Root Physiology: From Gene to Function. Plant Ecophysiology, 4, eds H. Lambers and T. D. Colmer, (Dordrecht: Springer).
Raij, B., van Andrade, J. C., Cantarella, H., and Quaggio, J. A. (2001). Análise Química Para Avaliação da Fertilidade de Solos Tropicais. Campinas: Instituto Agronômico de Campinas.
Ribeiro, H., Jaime, P. C., and Ventura, D. (2017). Alimentação e sustentabilidade. Est. Avanç. 31, 185–198. doi: 10.1590/s0103-40142017.31890016
Salton, J. C., and Lamas, F. M. (2011). “Integração lavourapecuária e o cultivo do algodoeiro nos cerrados,” in Algodão no cerrado do Brasil. 2, ed. E. C. Freire, (Aparecida de Goiânia, GO: Mundial), 473–494.
Santos, M. G., Soares Filho, W., Girardi, E. A., Gesteira, A. S., Orlando, S. P., and Ferreira, C. F. (2016). Initial horticultural performance of nine ‘Persian’ lime selections grafted onto Swingle citrumelo. Sci. Agric. 73, 109–114. doi: 10.1590/0103-9016-2015-0058
Souza, L. S., Velini, E. D., Martins, D. E., and Rosolem, C. A. (2006). Allelopathic effects of Brachiaria decumbens on the initial development of seven crops. Planta Daninha 34, 657–668. doi: 10.1590/S0100-83582006000400006
Spera, S. T., Escosteguy, P. A. V., Denardin, J. E., Klein, V. A., and dos Santos, H. P. (2011). Atributos químicos restritivos de Latossolo Vermelho distrófico e tipos de manejo de solo e rotação de culturas. Rev. Agrar. 4, 324–334.
Stolf, R., Murakami, J. H., Brugnaro, C., Silva, L. G., Silva, L. C. C., and Margarido, L. A. F. (2014). Penetrômetro de impacto stolf – programa computacional de dados em Excel-VBA. Rev. Bras. Ciênc. Solo 38, 774–782. doi: 10.1590/S0100-06832014000300009
Teasdale, J. R., and Mohler, C. L. (2000). The quantitative relationship between weed emergence and the physical properties of mulches. Weed Sci. 48, 385–392. doi: 10.1614/0043-1745(2000)048[0385:tqrbwe]2.0.co;2
Torres, F. E., Borges, C., Lempp, B., and Teodoro, P. E. (2016). Notas Científicas Contribuição dos caracteres de qualidade da forragem ao teor de proteína bruta em Urochloa brizantha. Pesq. Agrop. Bras. 51, 284–287. doi: 10.1590/S0100-204X2016000300011
Turner, N. C. (1988). Measurement of plant water status by the pressure chamber technique. Irrig. Sci. 9, 289–308. doi: 10.1007/bf00296704
Ulén, B., Aronsson, H., Bechmann, M., Krogstad, T., Oygarden, L., and Stenberg, M. (2010). Soil tillage methods to control phosphorus loss and potential side-effects: a Scandinavian review. Soil Use Manag. 26, 94–107. doi: 10.1111/j.1475-2743.2010.00266.x
Wright, G. C., McCloskey, W. B., and Taylor, K. C. (2003). Managing orchard floor vegetation in flood-irrigated citrus groves. Hort Tech. 13, 668–677. doi: 10.21273/horttech.13.4.0668
Yoshida, F. D. A., and Stolf, R. (2019). Environmental Fragility Based On The Spatial Distribution Of Soil Resistance For The Environmental Protection Area (Epa) Of Botucatu, São Paulo, Brazil. Hortic. Envir. 19, 391–405. doi: 10.14295/holos.v19i3.12316
Keywords: Citrus latifolia, ecological mower, intercropping, soil protection, Urochloa ruziziensis, glyphosate management, yield
Citation: Azevedo FA, Almeida RF, Martinelli R, Próspero AG, Licerre R, Conceição PM, Arantes ACC, Dovis VL, Boaretto RM and Mattos D Jr (2020) No-Tillage and High-Density Planting for Tahiti Acid Lime Grafted Onto Flying Dragon Trifoliate Orange. Front. Sustain. Food Syst. 4:108. doi: 10.3389/fsufs.2020.00108
Received: 04 May 2020; Accepted: 16 June 2020;
Published: 16 July 2020.
Edited by:
José Laércio Favarin, University of São Paulo, BrazilReviewed by:
Agnieszka Barbara Najda, University of Life Sciences in Lublin, PolandIfigeneia Mellidou, Hellenic Agricultural Organisation (HAO), Greece
Copyright © 2020 Azevedo, Almeida, Martinelli, Próspero, Licerre, Conceição, Arantes, Dovis, Boaretto and Mattos. This is an open-access article distributed under the terms of the Creative Commons Attribution License (CC BY). The use, distribution or reproduction in other forums is permitted, provided the original author(s) and the copyright owner(s) are credited and that the original publication in this journal is cited, in accordance with accepted academic practice. No use, distribution or reproduction is permitted which does not comply with these terms.
*Correspondence: Fernando Alves de Azevedo, fernando@ccsm.br