- 1Department of Environment and Society, Utah State University, Logan, UT, United States
- 2Department of Environmental Sciences, Emory University, Atlanta, GA, United States
Over the past century, agricultural land use in the United States has seen drastic shifts to support increasing demand for food and commodities; in many regions, this has resulted in highly simplified agricultural landscapes. Surmounting evidence exhibits the negative impacts of this simplification on the long-term provisioning of necessary ecosystem services to and from agriculture. However, transitions toward alternative systems often occur at a small scale, rather than at a systemic level. Within the National Research Council's (NRC) sustainable agricultural systems framework, we utilize national open-source datasets spanning several decades to broadly assess past and current agricultural landscapes across the U.S. We integrate and analyze agricultural land use and land cover data with policy data to address two main objectives: (1) Document and visualize changes over recent decades in cropland conversion, agricultural productivity, and crop composition across the U.S.; and (2) identify broad policy changes of the U.S. Farm Bills from 1933 to 2018 associated with these land use trends. We show that U.S. agriculture has gradually trended toward an intensely regulated and specialized system. Crop production is heavily concentrated in certain areas, larger farms are getting larger, while the number of smaller operations is decreasing, and crop diversity is declining. Meanwhile, federal agricultural policy is increasing in scope and influence. Through these data-driven insights, we argue that incremental and transformative pathways of change are needed to support alternative production practices, incentivize diversified landscapes, and promote innovation toward more sustainable agricultural systems across multiple scales.
Introduction
Agriculture has drastically transformed Earth's surface over the last century. Concerns arise in the ability of the global agri-food system to meet current and future food demands while maintaining biological diversity and conservation needs. Globally, since the 1960s, the large-scale demand and movement of commercial crops grown in intensive management systems has increased, contributing to a narrowing of crop species and genetic diversity worldwide (Harlan, 1975; Heal et al., 2004; Khoury et al., 2014). Surmounting evidence illustrates the negative ecological impacts of this shift, largely due to intensive annual crop production and landscape simplification (Pimentel et al., 1995; Tilman, 1999; Horrigan et al., 2002; Robinson and Sutherland, 2002; Benton et al., 2003; Bianchi et al., 2006). Simplified agricultural landscapes are associated with the degradation of key ecosystem services (ES)—or the benefits humans receive freely from the environment—that are essential to agricultural production, such as soil fertility, nutrient cycling, and genetic biodiversity, as well as regulating services including soil retention, pollination, natural pest control, and water purification (Tscharntke et al., 2005, 2012; Hendrickx et al., 2007; Meehan et al., 2011; Bommarco et al., 2013; McDaniel et al., 2014; Landis, 2017). ES generated by agricultural systems are primarily acquired through provisioning services, i.e., food, fiber, and fuel production, but also through cultural services, such as enhancing landscape aesthetics, building social networks, and market participation, and other services, such as wildlife habitat preservation; these mechanisms feed back into supporting and regulating services. Ecological functions that disrupt agricultural production (referred to as disservices), such as competition for water or crop damage from natural predators and pests, may further contribute to disservices generated from agriculture, including nutrient runoff or habitat loss (Rabalais et al., 2002; Zhang et al., 2007; Hillier et al., 2009; Cardinale et al., 2012; Hooper et al., 2012). Managing agriculture to optimize ecosystem health and the provisioning of key ES for agriculture while minimizing disservices can increase the stability and quantity of production over time, decrease need for external inputs, and increase ES delivery to the broader ecosystem (Cassman, 1999; Tscharntke et al., 2005; Bommarco et al., 2013, 2018; Pywell et al., 2015; Burchfield et al., 2019).
Recent calls for transformations in our agricultural landscapes emphasize the importance of agricultural systems that boost ES for agriculture through practices that are environmentally, economically, and socially beneficial while also maintaining or increasing productivity (Reganold et al., 2011). The National Research Council's (NRC) Committee on Twenty First Century Systems Agriculture (NRC, 2010) defined several objectives for sustainable agricultural systems. First and foremost, agricultural sustainability is defined within four main themes: (1) Satisfy human food, feed, and fiber needs and contribute to biofuel needs; (2) enhance environmental quality and the resource base; (3) sustain the economic viability of agriculture; and (4) enhance the quality of life for farmers, farm workers, and society as a whole. These main objectives of sustainability align with NRC's “systems agriculture” approach to understanding the interactions among actors and components of the system as a whole, rather than the function of each component separately. The NRC further identified three main qualities of system's robustness to use as considerations for systems moving toward sustainability. Robustness encompasses resistance (ability to withstand shocks), resilience (capacity to absorb shocks and stressors over time), and adaptability (ability to make necessary systemic changes in response to long-term environmental changes).
Identifying pathways toward sustainable change cannot be viewed through a dichotomous conventional-sustainable lens but rather contextualized within social, political, economic, and ecological drivers. As the NRC states, “The committee's definition of sustainable farming does not accept a sharp dichotomy between conventional and sustainable farming systems, not only because farming enterprises reflect many combinations of farming practices, organization forms, and management strategies, but also because all types of systems can potentially contribute to achieving various sustainability goals and objectives” (2010, p. 37). Although poorly defined across disciplines, agroecology has long presented viable alternatives to industrial agricultural practices (Francis et al., 2003). Rather than focusing on certain agroecological on-farm practices, we ground this paper in the broad definition from Brym and Reeve (2016, p. 214): agroecology is a “field of study motivated to understand ecological, evolutionary, and socioeconomic principles and use them in an improvement process that sustains food production, conserves resources, and maintains social equality.” This definition aligns with calls from the NRC to move toward greater sustainability through several pathways of change, either incremental or transformative. Incremental change can gradually increase and support the adoption of current conservation practices to make them more widespread within conventional systems, as well as also support research for the economic viability of such practices. Transformative change would support broader, systemic shifts from conventional and agroecological approaches through establishing new markets and supporting ecologically based management (e.g., organic, mixed systems) (NRC, 2010).
We build upon prior research that has attempted to assess and interpret changes in U.S. agricultural systems over time. Several studies have focused on land use change within specific regions of the U.S., such as agricultural land cover loss due to competing development demands in the Eastern U.S. (Drummond and Loveland, 2010; Sayler et al., 2016) or cropland concentration due to high soil quality in the South (Hart, 1978). A large number of studies have shown how the Corn Belt has intensified agricultural land toward specialized commodity production over time due to favorable climatic conditions, high quality land, and political incentives (Hart, 1986, 1991, 2001, 2004; Hudson, 1994; Drummond et al., 2012; Auch and Karstensen, 2015; Laingen, 2017). Other studies discuss trends of fluctuating conversion from grassland and marginal cropland to intensive commodity and biofuel production in the Great Plains, driven by enrollment in federal conservation programs, technological advances, improved management practices, and increased precipitation (Drummond et al., 2012; Wright and Wimberly, 2013; Reitsma et al., 2015; Taylor et al., 2015; Auch et al., 2018). However, these studies are limited in geographic scope and do not contextualize such trends in the national aggregate. Research with a broad U.S. focus are either outdated (Hudson, 1994; Hart, 2001; Cozen, 2010) or fail to discuss political drivers and environmental implications within an agroecological framework (Sleeter et al., 2013; Sohl et al., 2016; Auch et al., 2018; Hudson and Laingen, 2018). Other recent research has attempted to project recent land cover datasets farther back in time to assess historical land use trends (e.g., Arora and Wolter, 2018) but do not extend past the 1980s and emphasize the need to understand current land use trends through historical processes. Given the trajectory of U.S. federal agricultural policy, land use changes prior to the 1970s and 1980s are important in understanding how current trends were established and are reinforced. Data-driven research can help identify trends within and across agricultural systems to better inform the prioritization of sustainability objectives.
This paper serves as a high-level overview of how agricultural land use and policy drivers have changed at a national level over the past half century. Rather than attempting to evaluate the current state of sustainability of the U.S. agricultural system, this data-driven narrative serves two main objectives: (1) to clarify the magnitude and extent of large-scale agricultural landscape transformations, as well as the changes in policy structure, and (2) provide a framework to interpret and assess sustainable pathways for future agricultural change at the national scale. After discussing the methods, we present data trends and figures and contextualize these findings in the discussion section. We conclude with recommendations of national-level factors to consider within transitions toward more sustainable agriculture systems.
Methods
We utilized open-source datasets and open-source programming software to visualize policy, land use, and agricultural production changes. The majority of these data are focused on the county scale, as it is the finest resolution at which farm-level data is aggregated in the U.S. Using county-level data enabled us to understand, visualize, and interpret the spatial and temporal complexities of national agricultural trends. Through such visualizations, we illustrated trends in cropland transitions, crop composition, and the policy structure of the Farm Bills.
Datasets
Various multiscale datasets were synthesized and merged into a panel dataset (Table 1). Crop acreage, farm size, and chemical inputs were obtained through the National Agricultural Statistics Service (NASS) (USDA NASS, 2019c), whereby the county-year scale is the highest resolution available. The NASS database presents data both from the U.S. Census of Agriculture and a variety of national agricultural surveys administered by the USDA. USDA surveys are administered at the county and state scale annually with foci such as crop/stocks to measure crop acreage and yield, farm labor, crop prices and markets, and more specific topics, such as milk or broiler production. For some surveys, data are available from the mid-1800s to present day. The NASS QuickStats interface provides all of this survey information but does not indicate which survey the data are from or clearly define the cutoff of who counts in the surveys; additionally, the sampling strategy is determined by each state. Openly available from 1997 onward, the Census is conducted every 5 years and is administered to all farms and ranches (in rural or urban settings) producing and potentially selling at least $1,000 of their products. The Census is the only source of detailed county-level agricultural data that is collected, tabulated, and published using a uniform set of definitions and methodology. Thus, the Census is considered the most complete count and measurement of U.S. farms, operators, and ranches in the U.S. Though the combination of these data is limited in its generalizability given its inconsistency of data collection measures, it provides the most comprehensive, open-source record of historical U.S. agricultural data.
There are few land cover datasets that cover the entire U.S. and also extend decades back in time. Given its moderate spatial and temporal resolution, we utilized the National Wall-to-Wall Anthropogenic Land Use Trends (NWALT) dataset created by the U.S. Geological Survey (USGS) (Falcone, 2015). It uses the 2011 National Land Cover Database (NLCD) (Homer et al., 2015) as a base grid and other USGS and USDA historical imagery and datasets to map land use farther back in time with similar accuracy. NWALT classifications agreed with NLCD land use classifications from 2001-2011 with at least 94% accuracy and agreed with over 99.5% of county-level cropland changes from the USDA Census of Agriculture (Falcone, 2015). This dataset contains five 60-meter (m) resolution raster datasets from the years 1974, 1982, 1992, 2002, and 2012 of land use across the coterminous U.S, extending farther back in time than most other land cover datasets. However, some of the underlying data may span several years rather than an exact snapshot in time (Falcone, 2015); therefore, NWALT can be used for assessing broad temporal trends. We computed agricultural land as a percentage of overall county land to match the spatial resolution of NASS data. Agricultural land pixels are differentiated in this dataset by cultivated crop production and pasture/hay production based on 2011 NLCD classifications. Agricultural infrastructure, such as farm roads, are not included in these classifications.
The USDA's Economic Research Service (ERS) Major Land Uses (MLU) series has been collected every 5 years beginning in 1945, coinciding with the Census of Agriculture. As such, the ERS MLU is the longest running, most comprehensive accounting of all major land uses in the U.S. The dataset provides acreage across six land use categories (cropland, grassland pasture and range, forest-use land, special-use areas, urban areas, and miscellaneous other land) at both regional (Pacific, Mountain, Southern Plains, etc.) and state scales, compiled by reconciling several data sources. Thus, despite the ERS's use of standardized procedures to measure land use (Barnard and Hexem, 1988), there is a degree of uncertainty introduced by making comparisons through time. For this dataset, cropland includes cropland used for crops (harvested, crop failure, and cultivated summer fallow), cropland used for pasture (considered to be in long term rotation), and cropland idled. Grassland, pasture and range includes grassland and other non-forested pasture and range in farms, as well as estimates for open and non-forested grazing lands not in farms. Special use areas include rural transportation, rural parks and wildlife, defense and industrial areas, and miscellaneous farmland (farmsteads, farm roads and lanes, and misc. farmland). Urban areas include densely populated urbanized areas of 2,500 to 50,000 people or more, and forested areas including forest cover of grazed (commercial use) and non-grazed forest. We utilized this dataset to track trends in cropland conversion in comparison to other ERS MLUs between 1945 and 2012 (Bigelow and Borchers, 2017).
Finally, the U.S. Farm Bill (FB) policy documents from 1933 to 2018 are openly available through the National Agricultural Law Center (2019). While not the only important agricultural policy in recent U.S. history, the FB has played a key role in how, where, why, and what type of food is produced at a national scale. Over time, it has grown in size to encompass nearly all aspects of food production. These policy documents have changed in structure, starting with a 25-page document in 1933 encompassing two main topics: (1) agricultural adjustment and (2) agricultural credit, and becoming a 529-page document in 2018, encompassing 12 specific “Titles” ranging from Commodities to Nutrition to Rural Development. Within these Titles are statutes and funding programs that largely define the broader policy structure within which agricultural land use decisions are made.
Data Exploration
Using exploratory mapping and data mining techniques in R (version 3.6.3) (R Core Team, 2020), we selected variables of interest and assessed their spatiotemporal consistency and availability. This included plotting variables over time at county, state, and national scales to determine data reliability and representativeness, noting when and how representation changed across scales We focused on county-level data whenever possible as the most interpretable scale of agricultural landscape change. Particularly for NASS data, availability is variable by county, state, and year based on changing federal data collection, reporting procedures, and data privacy concerns; there are noted inconsistencies across USDA datasets as well (Hart, 2001; Arora and Wolter, 2018). Nonetheless, land use science and spatial modeling communities have acknowledged and accepted the need to use data at multiple scales given a lack of other alternatives (Rindfuss et al., 2004; Auch et al., 2018). Ultimately, we focused on six main variables of interest: (1) acres planted (by crop, per county and nationally), (2) percent planted (by crop, per county), (3) average acres per farm operation (per county), (4) percent crop and pasture land (per county), (5) cropland acreage (as a proportion of national acreage), and (6) agricultural input use (per county).
Given the changing structure and purpose of federal FB policies, we conducted a broad content analysis of the FB documents as a systematic way of capturing the frequency and content of textual data of the FBs from 1933 to 2018 (Krippendorf, 2004). With the qualitative coding software ATLAS.ti, we utilized a predetermined coding scheme to identify two major themes in each FB: (1) the number of distinct crops and (2) the stated purpose. These codes aimed to operationalize the scope and purpose of the FB as it relates to commodity production. Coding was limited to Titles, programs, and definitions that directly defined commodity crops, stipulated support and subsidies for their production, and promoted commodity markets; these included commodity programs, trade, agricultural marketing, credit, and crop insurance but excluded nutrition, conservation, forestry, research, etc. While excluded Titles do play a role in commodity production and land use, we explicitly focused on those that drive and regulate the composition of crops produced. Further, commodity definitions in the FB are defined within the commodity programs, and other Titles, such as conservation, are based upon these prior definitions. We contextualized these results within academic and gray literature.
Results
The results of this data synthesis are organized by three main themes. The first theme is land use which includes cropland, farm size, and productivity by visualizing trends in location of agricultural land, regional farm size variation, and how these changes relate to increased productivity of U.S. agriculture. The second theme is crop composition, including the composition of crops and how their relative acreage varies across space and has changed through time. The third theme is policy, presenting data to contextualize the overarching FB policy structure, how it has changed, and how it affects the first and second themes.
Changes are referenced within the regional specifications of the USDA ERSs Farm Resource Regions (FRRs) (Figure 1). These regions portray the geographic distribution of, and specialization within, the production of U.S. farm commodities (ERS, 2000). FRRs aggregate areas with similar types of farms, commodities, soil, physiographic, and climate characteristics nationally to contrast with the state and county boundaries (that are often political rather than biophysical borders) used to visualize data trends. We utilized these regions to further understand and contextualize trends across themes.
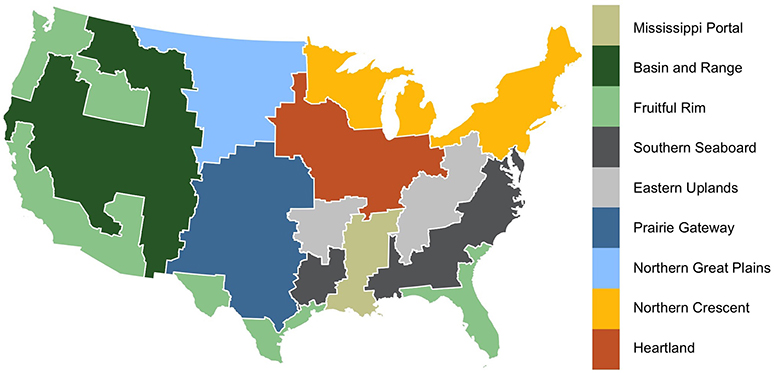
Figure 1. Farm Resource Regions (FRRs) across the U.S., determined by crop production type, amount, and value.
Cropland, Farm Size, and Productivity
U.S. cropland has changed in both amount and type over recent decades. From 1945 to 2012, cropland as a proportion of total land use decreased; meanwhile urban and special use areas increased (Figure 2). As seen in Figure 2, there was a slight decrease from 23.7% of the national share of land use in 1945 to 20.7% in 2012 (3% decrease). Comparatively, urban areas increased from 0.8% of the national share in 1945 to 3.7% in 2012. Special-use areas increased from 4.5% in 1945 to 8.9% in 2012. Grassland, pasture and range decreased by 0.03%. Forest-use decreased from 31.6 to 28.5%. Miscellaneous land uses decreased from 4.9 to 3.6%. However, both the ERS MLU and NWALT data confirm that cropland as a percentage of national land has decreased by 3% just since the 1970s. Therefore, this decline primarily occurred within the past four decades.
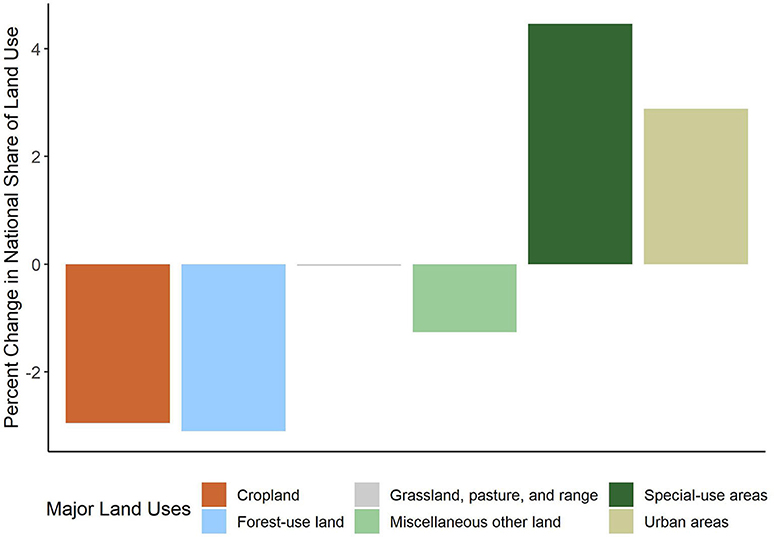
Figure 2. Percent change in the national share of land use across the ERS Major Land Use categories between 1945 and 2012. Data: ERS MLU.
Further, crops are grown in fairly concentrated regions, and there are no obvious changes in location of cropland. According to the NWALT data, counties where cropland is dominant have remained consistent over the past few decades without dramatic conversion of other land uses to cropland (see Supplementary Figure 1); by “dominant,” we mean that cropland accounts for most of the land use in a county. Though dominance does not tell the full story of a commodity (i.e., it does not demonstrate which counties are the most productive), it is an important metric in understanding the composition of U.S. agricultural landscapes. As Figure 3 illustrates, some counties, e.g., in the Heartland region, are almost entirely covered by cropland (nearly 100%), while others, e.g., in the Basin and Range region, produce few, if any, crops. Figure 3 also illustrates where cropland is most prevalent by county. The Southern Seaboard and the Fruitful Rim of California and the Pacific Northwest demonstrate clear intra-regional agricultural clustering, whereby crop production is concentrated in a select few counties. The midwestern Heartland and Mississippi Portal regions are dominated by cropland compared to the rest of the country; these areas of cropland dominance largely align with spatial trends in harvested acres for corn, soy, and wheat (see Supplementary Figures 2–4).
Pasture and land in hay production also demonstrate patterns of clustering. The proportion of land devoted to hay and pasture in the U.S. has decreased by 13.8% from the 1970s to 2012 (according to NWALT data), which is a larger change than the decrease in cropland (−2%). Furthermore, according to the ERS MLU data, grassland pasture and range have only lost 0.08% of its share of total land use between 1945 and 2012. Areas within the Heartland, Eastern Uplands, and Prairie Gateway regions exhibit high proportions of pasture and hay (Figure 4), whereby some counties are 50 to 70% covered by such production. However, these areas of landscape dominance do not necessarily produce the highest yields or relative yields (yield/harvested acre) in the U.S. For instance, clusters of counties in the West Coast portion of the Fruitful Rim harvest more hay per acre than any county in the Heartland (see Supplementary Figure 5). Pasture-dominant areas do not appear to overlap with crop-dominant areas, indicating divergent specialization in intensive crop and pastureland.
Farm size has been changing alongside the concentration of national agricultural land. The total number of farms has declined over time. In 2018, the USDA estimated 2 million farms nationally, which is 12,800 farms less than the estimate for 2017 (USDA NASS, 2019b). In 2011, the estimate was nearly 2.13 million; over 8 years, there was a 4.7% decrease in the number of farms nationally (USDA NASS, 2019b). The peak number of farms in the U.S. was in 1935 at 6.8 million farms, but this number has steadily decreased since then (Hoppe, 2014). Meanwhile, highly productive industrial farms have expanded in size while midsize farms continue to decrease in number. For example, of all agricultural land in the U.S. in 2018, 40.8% is operated by large-scale farms that earn sales of $500,000 or more, but these large operations comprise merely 7.5% of all total number of farms; farms that earn less than $100,000 comprise 30.1% of all farmland but comprise 81.5% of all farms (USDA NASS, 2019b). Thus, significantly fewer large-scale family and commercial farms operate a greater proportion of cropland.
Given this shift, total average farm size has not changed much in recent decades. According to the Census of Agriculture, the national average farm size changed from 440 acres in 1982, to 491 acres in 1992, to 433 acres in 2012, and 443 in 2019 (USDA, 1982, 1992; USDA NASS, 2019b). Therefore, average farm size has remained relatively stable due to a disproportionately greater number of smaller farms and larger farms increasing in size (Hoppe, 2014; MacDonald and Hoppe, 2017).
Regional differences of farm size further affect these averages. As seen in Figure 5, the largest farms are found in the Northern Great Plains [median = 1,505 acres, mean = 2,135 acres, standard deviation (SD) = 1,528 acres] and Basin and Range Regions (median = 783 acres, mean = 1,369 acres, SD = 1,516 acres), while the smallest farms are found in the Eastern Uplands (median = 148 acres, mean = 165 acres, SD = 77 acres) and Northern Crescent Regions (median = 161 acres, mean = 168 acres, SD = 80 acres). However, most regions have several outlier counties that exhibit average county farm sizes significantly beyond the regional mean. In particular, counties in the Basin and Range (median = 783 acres, mean = 1,368 acres, SD = 1,515 acres), Fruitful Rim (median = 271 acres, mean = 1,145 acres, SD = 3,756 acres), and Prairie Gateway (median = 817 acres, mean = 1,143 acres, SD = 1,186 acres) exhibit a wide range of average farm sizes; some counties in these regions average well over 5,000 acres per operation. Since most pasture and hay production occurs within the Prairie Gateway (Figure 4), these data show that such production in certain counties comprises much larger farms than the rest of the region. Contrastingly, regions such as the Eastern Uplands, Heartland, Northern Crescent, and Southern Seaboard exhibit outliers noticeably closer to the regional median. Given that the majority of cropland falls within the Heartland region (Figure 3), these data demonstrate that most of these farms are similar in size and are not the largest on average at a national scale (median = 319 acres, mean = 343 acres, SD = 155 acres).
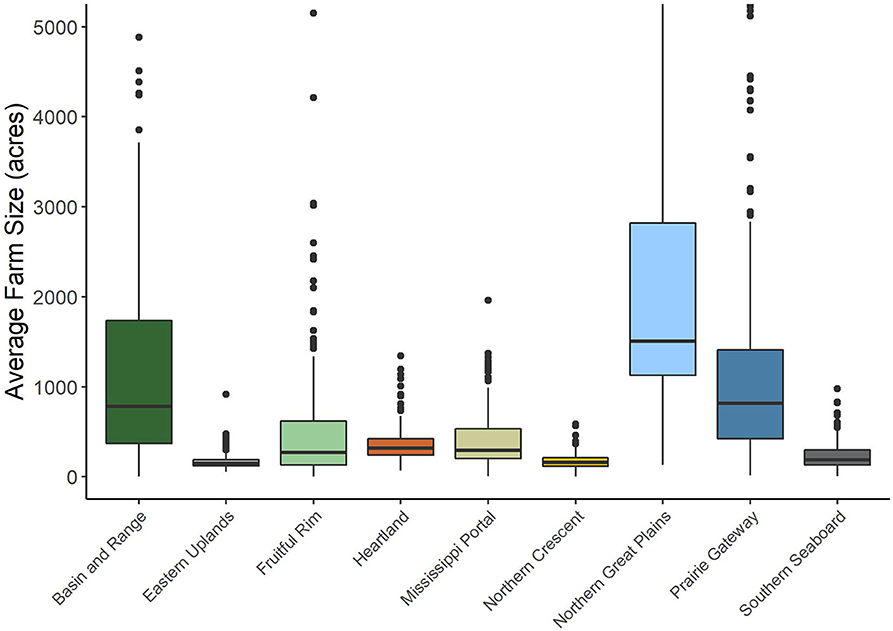
Figure 5. Average farm size (acres per operation) by FRR in 2012. Counties with an average farm size > 5,000 acres [n = 46, range = 5,119 to 37,952 acres] were removed from visualization for readability. Data: USDA NASS Survey.
Further, Figure 6 illustrates the variability in average farm size by county. The largest farms (in acres/operation per county) are found primarily in the western U.S. with a clear distinction between eastern and western counties. This also indicates where the largest farms in the Basin and Range, Prairie Gateway, and Texas portion of the Fruitful Rim regions are located. Farms that average over 10,000 acres are exclusively found in these regions and are clustered together. Most of the average farm sizes in these regions exceed 1,000 acres, if not 5,000 acres. In the Heartland, however, most farms do not exceed an average of 400 acres per operation.
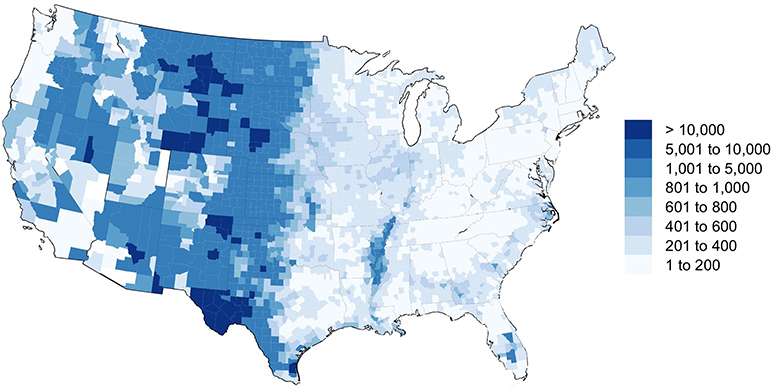
Figure 6. Average farm size (acres per operation) by county in 2012. Data: USDA NASS Census of Agriculture.
When directly comparing farm size and dominance of agricultural land (including both cropland and hay/pasture production) by county, certain areas exhibit large farm sizes but are not dominated by agricultural production at the county scale. By binning both average farm size by county and percentage agricultural land by county into thirds and pairing each tercile into distinct categories, we visualize the spatial relationship between farm size and agricultural dominance (Figure 7). Counties largely in the Heartland, Mississippi Portal, and Northern Great Plains exhibit, on average, medium and large farms with the highest percentage of agricultural land (in teal). Much of the counties in the Basin and Range and Prairie Gateway exhibit large average farm sizes (in acres/operation) and a low percentage of agricultural land (yellow). Counties with relatively small average farm size but a large percentage of the county as agricultural land (dark purple) are scattered throughout the rest of the Heartland, while both low percentage agricultural land and a relatively small average farm size per county (light blue-green) are almost exclusively found in the Southern Seaboard, Northern Crescent, and northwestern Fruitful Rim. These trends reflect the different landscape composition patterns across the country. Greater availability of land in the western U.S. may allow for much larger farms on average for grazing and pasture, but the concentration of these farms is relatively low compared to densely concentrated crop-producing farms across the midwestern U.S.
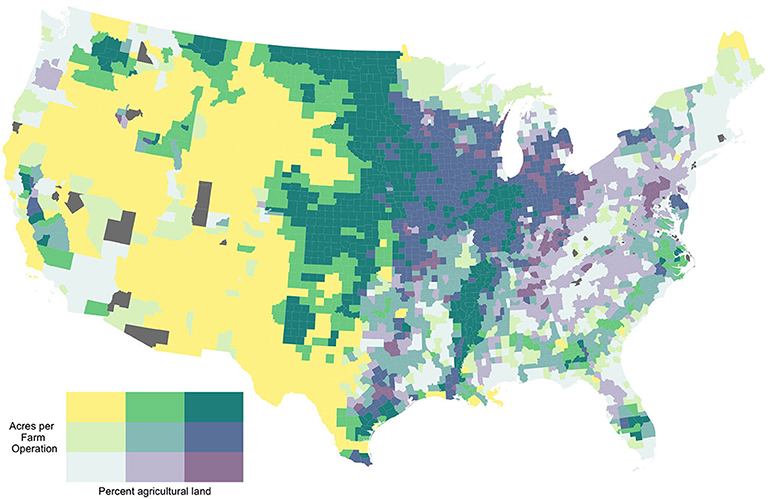
Figure 7. Bivariate choropleth constructed by binning county-level average farm size (by acre per operation per county) and percent agricultural land by county (both pasture and crop production) into thirds and pairing each tercile into distinct categories. Yellow indicates counties with large average farm sizes (in acres/operation) and a low percentage of agricultural land. Teal indicates counties with large average farm sizes and a high percentage of agricultural land. Purple indicates counties with a small average farm size but a large percentage of the county as agricultural land. Light blue is both low percentage agricultural land and a small average farm size per county. Dark gray counties indicate missing data. Data: NWALT and USDA NASS Census of Agriculture.
In conjunction with a decrease in national cropland and regional variations of farm size and type, U.S. agriculture has become more productive writ large since the 1970s. Total Factor Productivity (TFP) accounts for all of the land, labor, capital, and material resources employed in farm production and then compares them with the total amount of crop and livestock output. If, for instance, total output grows faster than total inputs, the total productivity of the factors of production (i.e., TFP) is increasing. TFP data is only publicly available at the state level from 1960 through 2004. Based on this data, since 1960, every state reflects an increase in TFP; no state or region has become less productive (ERS, 2019a). Farms in the Heartland and the Mississippi Portal have become over 100 to 150 percent more productive (see Supplementary Figure 6). Meanwhile, the Pacific Northwest portion of the Fruitful Rim and Basin and Range reflect TFP gains between 150 and 200 percent. Other areas in the Basin and Range, particularly throughout Colorado, Kansas, Montana, and Texas, have seen lesser gains but are still ~50 to 75 percent more productive than 1960. Productivity gains in the Southern Seaboard and the Northern Crescent reflect around a 100 to 125 percent increase on average. These increases are regionally concentrated to reflect the intensification of agricultural production in certain areas, particularly through increases in external inputs (Figure 8).
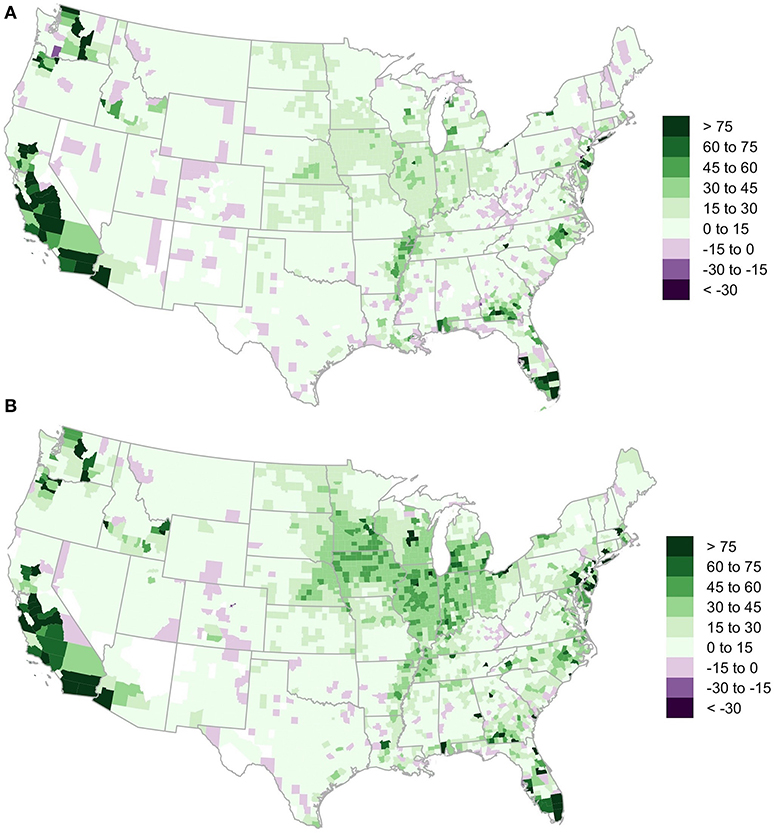
Figure 8. Change (in USD) in inputs per operated acre by county between 1997 and 2017 by county. (A) Change (in USD) in chemical expense per operated acre. (B) Change (in USD) in fertilizer expense per operated acre. 1997 USD values are adjusted for inflation using average consumer price indices (CPI) from January-December 1997 (avg. CPI ~ 160.52) and January-December 2017 (avg. CPI ~ 245.12). Data: USDA NASS Census of Agriculture.
Those same U.S. regions that have realized huge gains in TFP have, at the same time, become more reliant on off-farm inputs like synthetic fertilizers and chemicals. Certain counties in the West Coast portion of the Fruitful Rim and along the Southern Seaboard have increased expenditures on chemicals by, on average, $30 to over $75 per acre (Figure 8A) and on fertilizers by similar amounts (Figure 8B). Areas within the Heartland and Mississippi Portal have largely increased their chemical expenses by $0 to $30 per acre (Figure 8A) but have increased fertilizer expenses between an average of $15 to $45 per acre (Figure 8B). These large expenditure changes over the past two decades stand in contrast to places along the Southern Seaboard, within the Basin and Range, and the Prairie Gateway that have maintained spending, only shifting (increased or decreased) by $15 per acre. Again, these regional differences highlight the resource-intensive crop production practices of select U.S. agricultural regions. Overall, the majority (~80%) of counties show increasing use of, and expenditure on, synthetic inputs since 1997; few places (only within certain counties in the west and in the Eastern Uplands) have decreased spending per acre. However, since TFP has increased alongside external input use, this suggests that crop yield is rising faster than input use.
Crop Composition
Crop composition has seen drastic changes at a national level as agricultural production has become more productive and input intensive. Since 1963, harvested soybean and corn acreage (although complementary for crop rotation) has increased by 76 percent (74 million acres), while acreage for other feed crops such as oats, barley, sorghum, and hay have declined by a combined 50 million acres (Bigelow and Borchers, 2017). Wheat, once the dominant crop in the U.S., comprises the third largest acreage planted of U.S. crops at 46 million (Ash et al., 2018).
Since the 1970s (and preceding that), the composition of crop acreage (total acres planted per crop) across the U.S. has become increasingly specialized. Demonstrated in Figure 9, by 2019, total crop acreage of major crops is nearly dominated by corn, soy, and wheat (winter, spring, and durum). In 1925, corn and wheat comprise a majority of the acreage planted with cotton and oats following closely behind; however, the difference in acreage planted for these crops is comparatively small. From the mid-1920s to the 1970s, acreage for cotton, oats, barley, and peanuts gradually decreases; meanwhile, acreage for soybeans rapidly increases, and wheat and corn acreage remain consistently dominant. From the 1970s through 2019, acres planted for corn, soy, and wheat (particularly soy) increase at the same time other major commodities decrease. Steady declines of the planted acreage of sorghum, cotton, barley, and oats become evident as corn and wheat remain consistent, and soy continues to expand. Meanwhile, acreage of peanuts, canola, and rice remain negligible in a national context (see Supplemental Figure 7 for separated crop trends). Therefore, the 1970s era onward was characterized by observable specialization toward certain crops. As of 2019, these crops (corn, soy, and wheat) comprise a total of 210,958,000 planted acres; corn and soy alone cover nearly 166 million. According to the 2017 Census estimates of total cropland in the U.S., corn, soy, and wheat cover 64.7% of harvested cropland acres; corn and soy alone cover 56.6% (USDA NASS, 2019a).
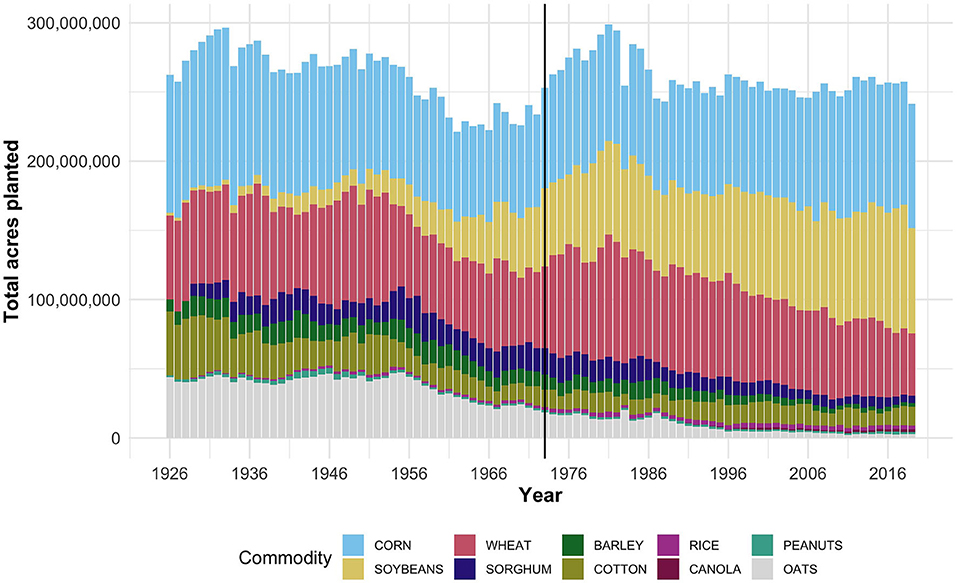
Figure 9. Total acres planted of 10 major U.S. crops between 1920 and 2019. Top 10 crops determined by acres planted in 2019. A vertical line at 1973 indicates the passing of the 1973 Farm Bill and marked transition toward crop specialization. Data: USDA NASS Survey.
Although the national trend in planted crop acres is dominated by corn, soybeans, and wheat, regional variability of agricultural land use diversity exists. The Shannon's Diversity Index (SDI) is a measure of evenness and abundance of different land use types as a way of measuring ecological diversity in a given area (Gustafson, 1998; Aguilar et al., 2015). Figure 10 illustrates the SDI per 20 km based on agricultural land use categories as defined by the USDA Cropland Data Layer (CDL) database (only available from 2008 to 2018 thus limiting its historical depth to interpret land use trends over time; Arora and Wolter, 2018) and computed by Burchfield et al. (2019). This index provides a measure of crop diversity for 20-kilometer (km) pixels within a given year. Areas of low diversity (light green) are concentrated in the Heartland and Basin and Range regions. Counties of high diversity (dark blue) are concentrated along the Southern Seaboard, Fruitful Rim of California and the Pacific Northwest, and the Northern Great Plans. Thus, certain agriculturally dominant regions, such as the Heartland, are highly specialized and non-diverse, while others, such as the Fruitful Rim of California, are highly diverse. Such variation in agricultural land use diversity emphasizes the different production systems and agroecological contexts in which crops are grown nationally.
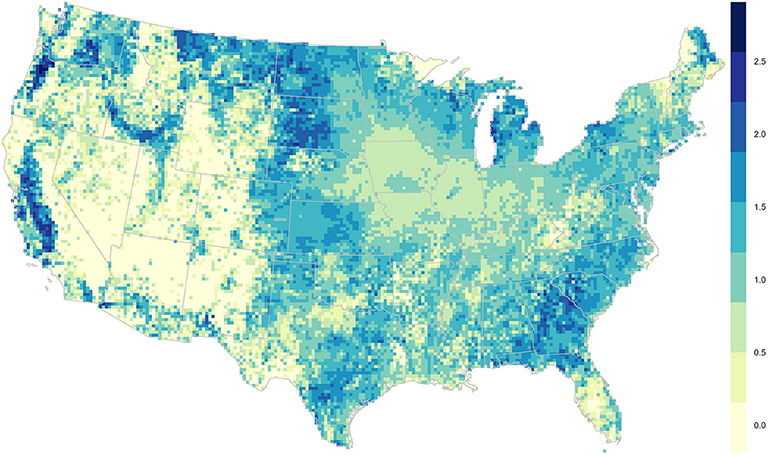
Figure 10. Shannon's Diversity Index (SDI) of agricultural land use categories for each 20-km pixel in the U.S. in 2017. Light green indicates counties with a low SDI and dark blue indicates counties with a high SDI. Reprinted from Burchfield et al. (2019). Copyright (2019), with permission from Elsevier.
These trends in crop diversity contextualize where the majority of crops that dominate U.S. crop production (as demonstrated from Figure 9) are concentrated. Figure 11 illustrates percent of a county cultivated for the two major crops: corn and soybeans. By visualizing the percent of each county cultivated by these crops in the U.S., regional dominance of this commodity production is evident.
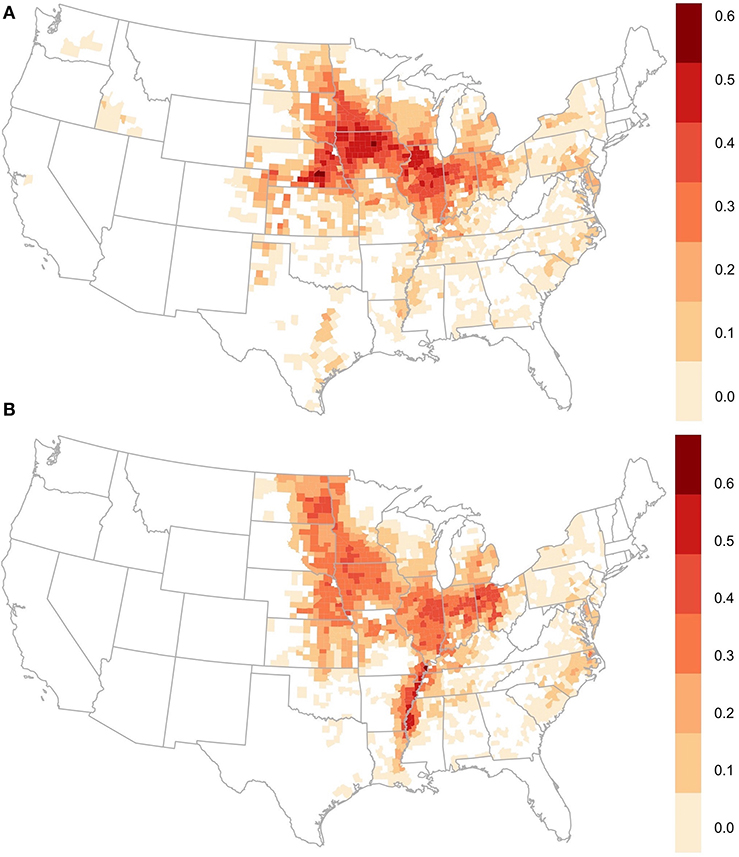
Figure 11. (A) Percent of total county land cultivated with corn in 2017. (B) Percent of total county land cultivated with soybeans in 2017. Data: USDA NASS Survey.
Dominant counties of 40% or higher of cultivated land for each crop largely fall within the midwestern Heartland region. Further, this region has a comparatively lower SDI value (Figure 10) than most other productive regions. Yet, areas along the Mississippi Portal and the Prairie Gateway demonstrate dominance of soybean cultivation and a comparatively high SDI value. The location of these dominant landscapes further illustrates how and where crop specialization has occurred and continues to occur.
Policy Structure
Agricultural land use changes in the U.S. take place within a policy structure that operates at multiple levels, from local zoning laws to national-level subsidy programs. The U.S. Farm Bill (FB) has become what is referred to as an omnibus (or all-encompassing) piece of legislation that largely influences how, where, and why food is produced and distributed; these policies cover an increasingly broad suite of programs and purposes. For example, the 1933 FB, titled the Agricultural Adjustment Act of 1933, aimed specifically to provide relief for farmers in debt and increase agricultural revenue. Its stated purpose is as follows:
“To relieve the existing national economic emergency by increasing agricultural purchasing power, to raise revenue for extraordinary expenses incurred by reason of such emergency, to provide emergency relief with respect to agricultural indebtedness, to provide for the orderly liquidation of joint-stock land banks, and for other purposes.” (Agricultural Adjustment Act, 1933)
Thus, it was a reactionary policy to an ongoing economic crisis. The most recent version of the FB passed in 2018, states its purpose as the following:
“To provide for the reform and continuation of agricultural and other programs of the Department of Agriculture through fiscal year 2023, and for other purposes.” (Agricultural Improvement Act, 2018)
This most recent FB reflects a broader purpose than 1933, maintaining and updating the status quo of the U.S. agricultural system. The goal for “reform and continuation of agricultural programs” emphasizes the growing importance of these programs that regulate how the U.S. agri-food system operates. FB programs currently cover a wide variety of “Titles” or topics in the 2018 policy document; these Titles include: (1) Commodities, (2) Conservation, (3) Trade, (4) Nutrition, (5) Credit, (6) Rural Development, (7) Research, Extension, and related matters, (8) Forestry, (9) Energy, (10) Horticulture, (11) Crop Insurance, and (12) Miscellaneous. This 2018 FB proposed a budget for $428 billion for its 5-year life span, of which 76% is dedicated to Nutrition programs such as the Supplemental Nutrition Assistance Program (SNAP), and a mere 9% is dedicated to crop insurance, 7% for commodities, and 7% for conservation (McMinimy et al., 2019). The importance and composition of these Titles has substantially changed over time, ultimately defining and reinforcing the political structure of agricultural production in the U.S (for a more complete list, see McFadden and Hoppe, 2017, Appendix A).
FB programs have historically aimed to improve agricultural productivity and markets by controlling the supply of commodities. The Emergency Feed Grains Act of 1961 replaced market-oriented policies with direct federal government regulation; this put the federal government in greater control over the driving forces of the production (McGranahan et al., 2013). Following that, the well-known era of “fencerow to fencerow” production of the 1970s was defined by increased supply of agricultural commodities that captured economies of scale to combat high production costs. The “Russian Grain Robbery” of the mid-1970s—in which the Soviet government purchased over one fourth of U.S. wheat harvests to increase their own livestock production—challenged domestic demand for commodities, tripled wheat prices, and doubled corn and soy prices. This market spike led to the export of 80% of wheat in the U.S. to the Soviet Union (Luttrell, 1973). The then Secretary of Agriculture, Earl Butz, supported this international trade market as a way of boosting exports to foreign markets. Therefore, to combat the rise in commodity prices for the U.S., he encouraged farmers to increase their production, aiming to create immediate surpluses of commodity crops, particularly corn and soybeans (McGranahan et al., 2013). Although overall cropland cultivated did not immediately increase during this era, corn, soy, and wheat production noticeably expanded while production of other crops (e.g., sorghum, barley, oats) declined (see Figure 9 above, whereby a vertical line at the year 1973 marks this transition). The Agricultural and Food Act of 1981 extended these federal support policies from the 1970s, leading to the 1980s Farm Crisis: the federal government made billions of dollars of payments to farmers growing commodity crops to reduce production, re-adjust commodity prices, and help farmers address rising debt (McGranahan et al., 2013). These federal regulations created incentives for specialized agricultural land use over the past 50 years currently still in effect.
Agricultural land reserve programs have played a role in influencing how and where commodities are produced. From the late 1950s through 1990, the federal government paid farmers to take productive cropland out of production as a means of supply control; this land had to be converted to grassland, trees, or other non-crop purposes (Olson, 2001). The Agricultural Act of 1956 established the Soil Bank Program to set aside 12 million hectares of land from commodity production to be used for wildlife habitat; however, the land enrolled in this early conservation reserve program was already low in productivity. Thus, this type of land reserve program helped regulate the amount of highly productive land used for commodity production by reducing the less productive land competing on the market with more productive land (McGranahan et al., 2013). Meanwhile, in conjunction with technological advances made during the Green Revolution of the 1950s and ‘60s, productivity of major crops increased on this high-quality land. In 1985, the Conservation Reserve Program (CRP) was established under the Food Security Act of 1985 with aims to reduce soil erosion on highly erodible cropland and reduce off-farm sedimentation, as well as decrease commodity surpluses and increase farm income. Further, the “swamp buster” provision was added for environmental protection by disincentivizing farmers from producing agricultural commodities on wetlands after 1985, as this conversion made them ineligible for federal support (Daniels, 1988). While the 1956 Soil Bank Act did not limit the amount of land that could be taken out of production, the 1985 CRP provision limited this amount of land to no more than 25% of a county's total cropland base; this helped minimize large-scale economic impacts on commodity prices and agri-businesses. However, ongoing commodity price support programs have continued to compete with CRP enrollment. Thus, while CRP enrollment has continued since 1985, it has not effectively targeted the most sensitive and erodible land or out-competed other financial incentives for farmers to produce subsidized commodity surpluses (Isik and Yang, 2004; Johnson et al., 2016).
In addition to incentivizing commodity production, FB programs have limited diversification on agricultural lands that are supported by federal subsidies. In the 1985 FB, acreage designated to commodity production was limited by the Acreage Limitation Program (ALP) and Paid Land Diversion Program (PLD); to receive subsidy payments, certain commodities could only be planted on a set amount of acreage. As of the 1996 FB, “production flexibility contracts” (a.k.a. “Freedom to Farm”) replaced ALP and PLD to allow farmers to plant different crops other than previously stipulated commodities to increase planting flexibility while still receiving federal support (Willis and O'Brien, 2002). Producers could plant 100% of their contract acreage to a different crop, including grazing or hay production. However, this flexibility was limited; fruit and vegetable production (other than lentils, mung beans, or dry peas) was prohibited, unless a history of double-cropping fruits or vegetables had been established (ERS, 1996). As of 2002, this planting flexibility was replaced with direct payments to farmers for specific crop types and payment rates, regardless of farmer need (Willis and O'Brien, 2002). By 2014, direct payment subsidies were cut from the FB, replaced by several risk management programs (discussed below), but these recent changes do not undo historical incentives for land use specialization.
Further, commodity support programs are only accessible to certain farmers and favor certain types of production. Historically and at present, these programs are only eligible for established base acres. Base acres are defined as farm-level acreage for certain commodities based on the historical average acreage of that commodity; these are the acres eligible for commodity program payments. Therefore, program payments are determined by what has been grown on these base acres rather than what is currently being grown. Base acres were established in the 2002 Farm Bill and reflect planted acreage from 1998 to 2001 until the recent opportunity from the 2014 Farm Bill to re-allocate acres based on 2009 to 2012 planting (Farm Bureau, 2016). However, this reallocation did not allow new base acres to be designated—only the adjustment of designated acres to different commodities. Since base acres are linked to the farm itself, not the farmer, this omits land recently converted to commodity production to be supported by FB commodity payments (Farm Bureau, 2016). This further incentivizes keeping land previously managed for intensive commodity production in the same type of production. Thus, farmers with certain acreage could receive payments for wheat production but not currently produce wheat; contrastingly, acreage under current wheat cultivation without base acreage designation could not receive program support. In fact, differences in base acres and actual average acreage planted for covered commodities are largely observed across the U.S., maligning the risk mitigation potential of Commodity Title programs with risk experienced by farmers (Newton, 2017). These base acreage designations have not been updated in the 2018 FB, but base acres out of commodity production in the past 10 years are now ineligible for program payments; instead, these base acres can be enrolled in conservation programs, such as the Conservation Stewardship Program (Newton, 2017).
Current Titles established under the 2018 FB reflect past influences of federal agricultural policies and reinforce federal support and influence over the U.S. food system. Although all Titles may influence farmer decision-making and agricultural land use in some way, the Commodity, Credit, Trade, and Crop Insurance Titles (designated as “commodity-focused” Titles hereafter) cover many of the programs that serve to directly mitigate risk through insurance, provide financial assistance and disaster relief through loans and subsidies, and influence market demand through international trade regulations. These Titles are major drivers of the types of commodities produced, as well as where, why, and how this production occurs in present day.
Of these commodity-focused titles, the Commodity Title is the arguably the most influential Title for regulating commodity production and influencing farmer decisions. Commodity programs effectively provide support for market fluctuations and risk associated with commodity production, comprising the majority of influence over agricultural land use. Two main programs under this title include the Price Loss Coverage (PLC) program and the Agricultural Risk Coverage (ARC) program and are administered through the Farm Service Agency (FSA). The PLC, based on a certain crop-year price, pays farmers with historical base acres eligible for covered commodities when the market-based effective price falls below the effective reference price—a price determined by the 2014 FB that allows for market fluctuations (ERS, 2019b). ARC pays farmers with historical base acres when the actual yield (distinguished between irrigated and non-irrigated acres) and prices for their county's average per-acre crop year revenue falls below the guaranteed level for each covered commodity. Commodities covered by both of these programs are defined as wheat, oats, barley, corn, grain sorghum, rice, soybeans, sunflower seed, rapeseed, canola, safflower, flaxseed, mustard seed, crambe and sesame seed, dry peas, lentils, small chickpeas, large chickpeas, and peanuts. As of the 2018 FB, farmers can switch between PLC and ARC programs with greater flexibility. Other programs include the Non-insured Crop Disaster Assistance Program (NAP), Non-recourse Marketing Assistance Loan Program (MAL), and the Dairy Margin Coverage Program (DMC). NAP provides risk protection for crops not covered under the Federal Crop Insurance Program. MAL offers farmers short-term loans when market prices are at their lowest (during harvest time) to allow them to wait and sell their commodity when prices improve. Eligible commodities for MAL include wheat, corn, sorghum, barley, oats, upland and extra-long-staple cotton, long- and medium-grain rice, soybeans/other oilseeds, certain pulses, peanuts, sugar, honey, wool, and mohair. DMC offers coverage for dairy producers when the margin between the price of all milk and the average feed price is below a producer-determined threshold to help manage the fluctuations of the dairy market (ERS, 2019b). These programs largely aim to mitigate risk for farmers, as opposed to control supply of commodities.
Other commodity-focused Titles serve different yet complementary purposes. The Crop Insurance Title updates, modifies, and enacts the Federal Crop Insurance Program (FCIP) whereby farmers can access subsidies to protect against yield, crop revenue, and whole-farm revenue (WFA) losses (Johnson and Monke, 2019). Yield and crop revenue insurance coverage is crop-specific, whereby WFA covers the expected income of an entire farm to support more diversified systems. These insurance products are administered through the Risk Management Agency (RMA) and coverage extends across row crops, livestock, dairy, organic production, other specialty crops, grazing land, etc. (ERS, 2019b). The Trade Title reinforces global markets for U.S. grown crops and largely influences international food prices for U.S. farmers (ERS, 2019b; Johnson and Monke, 2019). Finally, the Credit Title provides direct government loans to farmers and ranchers through the FSA to support beginning, socially disadvantaged, and veteran producers (ERS, 2019b; Johnson and Monke, 2019).
As the structure of each FB has changed over time, the number of crops and commodities included in commodity-focused Titles and programs has increased. Figure 12 illustrates the distinct number of crops and commodities in such Titles of each FB over time. This numeric measure helps illustrate both the broadening scope of the policy itself, as well as the diversity of crops included within FB programs that aim to support and regulate their production.
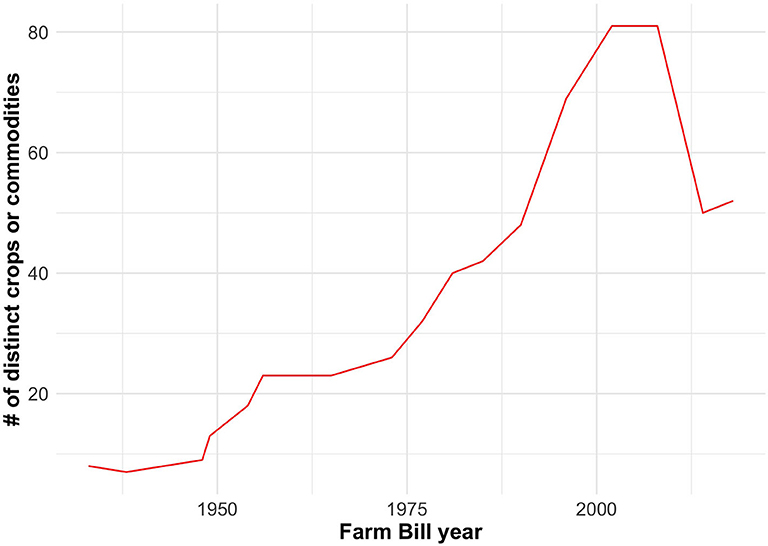
Figure 12. Number of distinct crops or commodities included in the Farm Bill Commodities, Trade, Credit, and Crop Insurance Titles (i.e., commodity-focused Titles). Data: U.S. Farm Bills.
The 1933 FB only mentions eight distinct crops and animal products (cotton, wheat, rice, corn, tobacco, hogs, milk, and fruit groves/orchards) in its entire 25 pages, demonstrating its limited and reactionary purpose. Contrastingly, the 2018 FB mentions 52 distinct crops across 529 pages—a product of a gradual expansion in scope and influence over time. The highest number of crops mentioned is 81 in both the 2002 and 2008 FBs. Crops classified as fruits or vegetables were not recognized or mentioned in the documents until the 1980s; crops for biofuel or organic production were not introduced until the late 1980s, as well. Further, while the number of crops and commodities within the FB increased from the 1970s onward, the composition of U.S. crop acreage became increasingly less diverse (as seen in Figure 9 above); these political and ecological changes occurred in tandem, suggesting that the increasing scope of the FB supported such specialization.
Discussion
We discuss the implications of these results in the context of recent literature and the concern for transitioning the U.S. agricultural system toward greater sustainability. The discussion is structured to mirror the results section and contextualize the above data trends. We conclude with recommendations within the broader framework of sustainable agricultural transitions and future research.
Cropland, Farm Size, and Productivity
In recent decades, U.S. agricultural production has reaped the benefits of industrialization and mechanization to support exponential increases in yield of major crops (Reganold et al., 2011; Aguilar et al., 2015; Pellegrini and Fernández, 2018). Although total land area devoted to agriculture is declining nationally (yet expanding globally, see Ramankutty et al., 2018), crop production is heavily concentrated in certain areas. Larger farms are consolidating, and competition for farmland among farmers is increasing (USDA NASS, 2019b). These large-scale farms are comprising more and more of U.S. cropland and are out-competing smaller operations (Paul et al., 2004; MacDonald and Hoppe, 2017); this consolidation is driven by historical patterns of land dispossession and predominantly White landownership (Dunbar-Ortiz, 2014; Ayazi and Elsheikh, 2015; Horst and Marion, 2019), as well as farmers expanding through part-ownership and operating rented land (Hart, 1991). At the same time, larger farms have brought economies of scale that boost productivity (Paul et al., 2004) and benefit from economies of size that make it profitable to expand farm size per unit of output (Duffy, 2009). Agglomeration of agricultural production around similar land uses and crop types reflects the pressure for farms to consolidate input investments, share information, and overcome the scalar thresholds of market competition.
While biophysical differences and political incentives influence regional specialization of crop production (Hart, 1978, 1986, 2001), county-level dominance of cropland in areas such as the Heartland, Basin and Range, and Mississippi Portal signifies the simplification and intensification of agricultural landscapes. The Corn Belt, originating from a landscape of mixed farming and agricultural experimentation, has become highly specialized for surplus commodity production (Hart, 1986; Hudson, 1994). The location of farms and cropland in the Heartland has remained relatively stable over the past several decades, indicating that the highest quality and most productive agricultural lands have stayed in agriculture throughout the region (Hart, 1986, 1991; Drummond et al., 2012). Other regions across the western U.S. have seen fluctuations in amount and location of cropland due to greater climatic, economic, and technological variability, as well as changing FB policies (Hart, 2001; Drummond et al., 2012). National evidence of productivity growth, particularly in the Midwest, indicate that farm consolidation is a substantial factor in the exponential increase of aggregate TFP, alongside technological innovation (Key, 2019).
Technological advances in seed genomics, fertilizers, chemicals, and mechanization have revolutionized agriculture in the U.S., but they have also introduced complicated ecological consequences. The introduction of herbicide-resistant (HR) genetically engineered crops in 1996 made the broad-spectrum application of glyphosate possible. Glyphosate-resistant HR crops have necessarily increased the application rates of herbicides and pesticides, introducing resistance in weed and insect populations; meanwhile, populations of beneficial species are decreasing (Benbrook, 2012; Pimentel and Burgess, 2014). Innovations in low-cost synthetic fertilizers in the 1950s and ‘60s made integrated crop-livestock farming and nutrient recycling biologically obsolete (Davis et al., 2012). Farmer reliance on synthetic fertilizers has increased due to soil fertility declines, yet evidence suggests that synthetic nitrogen depletes soil organic matter, a key indicator of soil health (Mulvaney et al., 2009). Labor efficiency increased with mechanization, and synthetic fertilizers and chemical inputs became increasingly available; meanwhile, specialization of crop and livestock production became more economically viable and efficient. Agricultural research has enabled corn, soy, and wheat to be highly productive per acre harvested. In the 2017/18 season, corn and soy provided $232 and $287 net returns per acre, respectively, and wheat provided $98 per acre (Ash et al., 2018). Yields of these crops and commodities have seen exponential increases prior to and following the Green Revolution in certain areas (e.g., the Corn Belt) yet have begun to plateau in others (e.g., fringes of the Corn Belt) (Hart, 1986; Ray et al., 2012; Pellegrini and Fernández, 2018). These advances led to increasing economies of scale, captured in the growth of farm size, shifts in farm infrastructure toward specialization, and a rapid decline in the number of farms across the U.S. (Hart, 1986; Dimitri et al., 2005).
Trends in national cropland reflect a “land-sparing” approach—less land used more intensively for increasing productivity and specialization—compared to a “land-sharing” approach—more land used more extensively to manage greater diversity of land use (Phalan et al., 2014). These different approaches to land management have been hotly debated regarding conservation and long-term sustainability (Fischer et al., 2008, 2014). As the U.S. trends toward greater specialization in agricultural production, this puts greater pressure on effective biodiversity conservation of non-agricultural land. Furthermore, this specialization holds implications for the sensitivity and resilience of agricultural production within an increasingly uncertain climate (Ortiz-Bobea et al., 2018) and increasing reliance on external mechanization (Rada and Fuglie, 2019). Such changes could increase farmer debt and put greater pressure on rural economies. These implications heighten concern over the long-term management of the ecological health of agricultural land within the context of increasing input use, machinery, and decreasing intra-crop and inter-crop species diversity within and across farms.
Crop Composition
In the U.S., the diversity of agricultural crops cultivated has decreased since the 1970s with wide regional differences. Regions that are most productive for dominant crops (i.e., corn and soybeans) maintain the least crop species diversity. Certain areas, such as Mississippi Portal Region, have maintained higher crop species diversity, whereby other areas, such as the Heartland region, have become largely optimized for a select few crops and commodities through decreasing diversity (Hart, 1986; Aguilar et al., 2015; Baines, 2015; Auch et al., 2018). Similarly, on a global scale, agricultural land has become dominated by a less diverse portfolio of crops (Martin et al., 2019).
Effects of declining crop species diversity raise concerns over the long-term health of agricultural ecosystems, as well as the stability of agricultural economies over time. Crop species diversity can be assessed at an on-farm and landscape level and holds different implications for land management. Increasing crop species diversity at a landscape level through compositional heterogeneity (i.e., the distinct number of crop types across a landscape) may have significant beneficial impacts on yield of major crops like corn and soy (Burchfield et al., 2019). Increasing configurational heterogeneity (i.e., the spatial arrangement of crop types and land uses) can boost pollinators and plant reproduction for small-scale farms (Hass et al., 2018). Further, increasing farm-scale diversity can improve the resilience and stability of agricultural production over time (Abson et al., 2013). Although some U.S. regions are much less diverse than others, maintaining crop diversity at local, national, and global scales is of great importance to achieve and maintain food security for the future (Massawe et al., 2016).
Managing on-farm and landscape-scale crop species diversity comes with a suite of considerations. Assuming that farmers aim to reduce risk in their operations, diverse cropping systems and practices have been positively linked to increased mean income and reduced income variance over time (Di Falco and Perrings, 2003). Crop diversity is known to enhance ecosystem services (ES) such as soil health, pest management, and water quality (Tscharntke et al., 2005, 2012; Hendrickx et al., 2007; Meehan et al., 2011; Bommarco et al., 2013; McDaniel et al., 2014; Landis, 2017), but these ecological benefits must also complement, if not enhance, other benefits for farmer livelihoods. Increasing crop diversity through practices such as crop rotation (over several seasons), intercropping (within one season), non-crop vegetation (such as filter strips or wildlife habitat), or integrated pest management pose challenges and barriers to their adoption; these include learning new management skills, balancing the potential risk on yield of major crops, or accessing appropriate machinery or technology to implement them effectively (Way and van Emden, 2000; Hooper et al., 2005; Pridham and Entz, 2008; NRC, 2010). Furthermore, these incentives and disincentives are filtered through federal agricultural policies that offer competing financial support. Biodiversity management on farms and across landscapes must be contextualized through such overlapping political, ecological, and social constraints.
Policy Structure
Federal agricultural policy has increased in scope since 1933 and maintains considerable influence. In fact, through this increase, the federal government is the primary source of supplemental income for farmers through subsidy payments (O'Connor, 2012). While the purpose of the FB has changed significantly since 1933, the incentive structure has not, prioritizing commodity production over both conservation practices (Lehner and Rosenberg, 2018) and agricultural diversification, even when the cost of production has exceeded farmer revenue (Hart, 1986). Even though the number of crops indicated in each commodity-focused FB Title has increased, the national crop portfolio has become increasingly less diverse. This misalignment between the diversity of crops regulated or supported by FB programs and the non-diversity of U.S. crop production highlights how policy ultimately promotes specialized commodity production. While environmental concerns arise over such land use trends, the implications of these federal policies are mixed.
Increasing federal control over and support of agricultural production has been debated in recent literature, particularly if and how it may promote or inhibit greater sustainability for both farmer livelihoods and ecological health. Evidence supports that U.S. agricultural subsidies are less accessible to smaller, organic, or diversified farming operations, fail to encourage conservation practices, promote commodity specialization (Bruckner, 2016), and systemically privilege White landowners over marginalized farmers and farmworkers (Dunbar-Ortiz, 2014; Ayazi and Elsheikh, 2015; Minkoff-Zern and Sloat, 2017). While subsidies and financial assistance may help mitigate risk associated with crop diversification for farmers, it has also been shown to discourage diversification and support specialized commodity production (Di Falco and Perrings, 2005). Since crop insurance helps mitigate the need for income variation, farmers may rely less on diversifying their farming systems to reduce this risk (O'Donoghue et al., 2009). Growing federal support for risk mitigation programs—such as ARC, PLC, and crop insurance programs—further decouples farmer decision-making from environmental risk. Although crop insurance enrollment does not lead to greater nutrient use through fertilizers and other chemicals (Weber et al., 2016), recent studies have shown that crop insurance increases irrigation withdrawals across the U.S. by motivating farmers to grow more water-intensive crops (Deryugina and Konar, 2017). Furthermore, farmers enrolled in crop insurance were found to experience greater yield sensitivity of corn and soy in extreme heat than those not insured; thus, crop insurance could provide a disincentive to take adaptive measures against climate-related impacts (Annan and Schlenker, 2015).
Despite these limitations, removing or decreasing federal agricultural assistance as an alternative is associated with several tradeoffs. In fact, this reduction may actually support farm consolidation. Large farms can more easily access crop insurance (due to access to greater capital) than small and medium size farms (Bruckner, 2016; Graddy-Lovelace and Diamond, 2017); this reinforces barriers for disadvantaged, small-scale, or aspiring farmers (Calo and De Master, 2016; Rosenberg and Stucki, 2017; Horst and Marion, 2019). Examples of subsidy reduction outside of the U.S. exhibit mixed results. Subsidy removal in Canada has been associated with increased specialization of production (Bradshaw, 2004), while New Zealand has seen increased farm diversification and off-farm income for farmers (Vitalis, 2007). Some argue that focusing the political debate around agricultural subsidies distracts policymakers from intervening in agricultural markets in necessary yet beneficial ways (Graddy-Lovelace and Diamond, 2017). Therefore, increased agricultural subsidies do not presume to move away from agricultural sustainability, but rather the type and incentive of such policies should be questioned.
Conclusion
Overarchingly, the U.S. agricultural system has gradually transitioned toward a regulated and specialized system, recognized through consolidation of U.S. farms and the homogenization of crop production. Fewer and fewer farms own more and more land, and these farms continue to produce a select few crops within highly mechanized processes. These changes emphasize productivity and efficiency, despite increasing concern for biodiversity loss. Further, even though the Farm Bill has increased in scope, the underlying structures incentivizing and reinforcing agricultural specialization have not changed.
While we do not attempt to assess the current sustainability of U.S. agriculture within the NRC's definition, historical data trends accentuate the priorities of the production system writ large. Through substantial gains in productivity and specialization of commodities across the U.S., past and current agricultural land use largely reflect two of the sustainability objectives: (1) satisfying human food, feed, fiber, and biofuel needs; and (2) sustaining the economic viability of agriculture. However, the prioritization of sufficient production and its economic viability has come at the cost of the other outlined objectives: (3) enhancing environmental quality and the resource base; and (4) enhancing the quality of life for farmers, farm workers, and society as a whole. Intensive commodity production has concentrated in space and contributes to biodiversity loss and declining agroecosystem health. These systems often fail to promote farming that harnesses and enhances ES provisioning and are increasing reliance on external inputs instead. Meanwhile agricultural policies are not equally as advantageous or accessible to all producers, exacerbating social inequities and disadvantaging new or diverse farmers. The imbalance of these objectives heightens concern over the robustness of the system. Decreasing trends in crop diversity may contribute to decreased resistance and resilience to shocks and stressors associated with a changing climate and changing environments, and the adaptability needed to address urgent changes may be limited by an increasingly regulatory policy structure.
Within the NRC framework of change, both incremental and transformative approaches to change are necessary to promote more sustainable agricultural systems. For large-scale landscape transformations to occur, agricultural research and technological innovation must focus on commercial grain producers; this is how the majority of the agricultural land is used. To implement transformative change without destabilizing crop markets would be difficult. However, given how large these agricultural landscapes are, any change in their compositional (increased complexity of different land cover types) and configurational (increased complexity of spatial patterning of cover types) heterogeneity can produce important changes in biodiversity for local or global conservation (Fahrig et al., 2011); changes outside of these markets will not have the largest transformative impact. Therefore, incremental approaches could best support technological advancements and innovations already available for land management by building off current research and enhancing adoption for existing conservation alternatives. Transformative change could target restrictive policies—such as updating base acreage designations or reducing barriers for non-White or small-scale farmers—to encourage more flexible and diverse programs that support commodity production. Federal agricultural policy at present fails to effectively promote diversification or conservation practices; whether increased or decreased federal support will do so is currently debated. Yet, a more diverse and socially inclusive suite of programs can help support more diverse systems in which these commodities are grown, promoting technological innovations that can reduce the impacts of agricultural landscape simplification. If large farms and corporate entities remain consistently advantaged over small farms and businesses, then alternative agricultural management schemes will be limited.
We have built upon the NRC (2010) report discussing the complicated nature of evaluating sustainability within agricultural systems. By utilizing national-level data to look at trends of land use and policy over time, we inform and update previous research to remain contextually relevant for policy decisions and assess U.S. trends writ large. Agricultural transformations toward sustainability do not fit within the dichotomy of conventional or sustainable systems. Rather, considering drivers and constraints across multiple scales helps identify realistic pathways of change. For a more sustainable future, both incremental and transformative changes are needed to address the proximate and ultimate conditions of the current state of agricultural landscapes. Although crop composition, productivity, and farm consolidation trends vary regionally, agricultural policy is regulated at a federal level. Therefore, we call for federal agricultural policies to more appropriately address the current drivers of on-farm and landscape simplification, as well as the overlapping factors of sustainability from the local to global scale to contextualize the feasibility of agricultural transitions.
Data Availability Statement
Publicly available datasets were analyzed in this study. These data can be found here: https://quickstats.nass.usda.gov/; https://nationalaglawcenter.org/farmbills/; https://www.ers.usda.gov/data-products/major-land-uses/; https://pubs.er.usgs.gov/publication/ds948; https://www.ers.usda.gov/data-products/agricultural-productivity-in-the-us/. All R scripts will be provided by the authors upon reasonable request.
Author Contributions
KS and EB contributed conception and design of the study. All authors organized and visualized data. KS led the writing of the manuscript, while all authors contributed to manuscript revision, and have read and approved the submitted version.
Funding
This research was supported by funds from EB's start-up funds from the Department of Environment and Society at Utah State University (USU), USU's Climate Adaptation Science Program through the National Science Foundation under Grant No. 1633756, and the Utah Agricultural Experiment Station under Grant No. UTA01422.
Conflict of Interest
The authors declare that the research was conducted in the absence of any commercial or financial relationships that could be construed as a potential conflict of interest.
The reviewer RA and handling editor declared their shared affiliation at the time of the review.
Acknowledgments
We would like to thank Stan Rhodes for his assistance with clarifying our policy analysis approach and Emma Jones for downloading and organizing data.
Supplementary Material
The Supplementary Material for this article can be found online at: https://www.frontiersin.org/articles/10.3389/fsufs.2020.00098/full#supplementary-material
References
Abson, D. J., Fraser, E., and Benton, T. G. (2013). Landscape diversity and the resilience of agricultural returns: a portfolio analysis of land-use patterns and economic returns from lowland agriculture. Agric. Food Secur. 2:2. doi: 10.1186/2048-7010-2-2
Aguilar, J., Greta, G. G., Hendrickson, J. R., Archer, D. W., Forcella, F., and Liebig, M. A. (2015). Crop species diversity changes in the United States: 1978-2012. PLoS ONE 10:e0136580. doi: 10.1371/journal.pone.0136580
Annan, F., and Schlenker, W. (2015). Federal crop insurance and the disincentive to adapt to extreme heat. Am. Econ. Rev. 105, 262–266. doi: 10.1257/aer.p20151031
Arora, G., and Wolter, P. (2018). Tracking land cover change along the western edge of the U.S. corn belt from 1984 through 2016 using satellite sensor data: observed trends and contributing factors. J. Land Use Sci. 13, 59–80. doi: 10.1080/1747423X.2018.1466001
Ash, M., Bond, J. K., Capehart, T., Childs, N. W., McConnell, M., Meyer, L., et al. (2018). An Exploration of Crop Markets: A Deeper Look Into the USDA Crop Baseline Projections. FDS-18J-01. U.S. Department of Agriculture, Economic Research Service.
Auch, R. F., and Karstensen, K. A. (2015). Status and Trends of Land Change in the Midwest-South Central United States-1973 to (2000). U.S. Geological Survey Professional Paper 1794-C. Land Cover Trends (Washington, DC: USGS).
Auch, R. F., Xian, G., Laingen, C. R., Sayler, K. L., and Reker, R. R. (2018). Human drivers, biophysical changes, and climatic variation affecting contemporary cropping proportions in the northern prairie of the U.S. J. Land Use Sci. 13, 32–58. doi: 10.1080/1747423X.2017.1413433
Ayazi, H., and Elsheikh, E. (2015). The Farm Bill: Corporate Power and Structural Racialization in the United States Food System. Berkeley, CA: Haas Institute for a Fair and Inclusive Society at the University of California Berkeley. Available online at: https://belonging.berkeley.edu/global-justice/glocal-food-systems/farm-bill-report-corporate-power-and-structural-racialization-us-food-system
Baines, J. (2015). Fuel, feed and the corporate restructuring of the food regime. J. Peasant Stud. 42, 295–321. doi: 10.1080/03066150.2014.970534
Barnard, C. H., and Hexem, R. (1988). Major Statistical Series of the U.S. Department of Agriculture: Land Values and Land Use, AH-671, Vol. 6. US Department of Agriculture, Economic Research Service.
Benbrook, C. M. (2012). Impacts of genetically engineered crops on pesticide use in the U.S. - The first sixteen years. Environ. Sci. Eur. 24:24. doi: 10.1186/2190-4715-24-24
Benton, T. G., Vickery, J. A., and Wilson, J. D. (2003). Farmland biodiversity: is habitat heterogeneity the key? Trends Ecol. Evol. 18, 182–188. doi: 10.1016/S0169-5347(03)00011-9
Bianchi, F. J. J. A., Booij, C. J. H., and Tscharntke, T. (2006). Sustainable pest regulation in agricultural landscapes: a review on landscape composition, biodiversity and natural pest control. Proc. R. Soc. B Biol. Sci. 273, 1715–1727. doi: 10.1098/rspb.2006.3530
Bigelow, D. P., and Borchers, A. (2017). Major Uses of Land in the United States (2012). EIB-178. U.S. Department of Agriculture, Economic Research Service.
Bommarco, R., Kleijn, D., and Potts, S. G. (2013). Ecological intensification: harnessing ecosystem services for food security. Trends Ecol. Evol. 28, 230–238. doi: 10.1016/j.tree.2012.10.012
Bommarco, R., Vico, G., and Hallin, S. (2018). Exploiting ecosystem services in agriculture for increased food security. Glob. Food Secur. 17, 57–63. doi: 10.1016/j.gfs.2018.04.001
Bradshaw, B. (2004). Plus c'est la même chose? questioning crop diversification as a response to agricultural deregulation in Saskatchewan, Canada. J. Rural Stud. 20, 35–48. doi: 10.1016/S0743-0167(03)00033-0
Bruckner, T. (2016). Agricultural subsidies and farm consolidation. Am. J. Econ. Sociol. 75, 623–648. doi: 10.1111/ajes.12151
Brym, Z. T., and Reeve, J. R. (2016). “Agroecological principles from a bibliographic analysis of the term agroecology,” in Sustainable Agriculture Reviews, Vol. 19, ed E. Lichtfouse (Cham: Springer International Publishing), 203–231.
Burchfield, E. K., Nelson, K. S., and Spangler, K. A. (2019). The impact of agricultural landscape diversification on U.S. crop production. Agric. Ecosyst. Environ. 285:106615. doi: 10.1016/j.agee.2019.106615
Calo, A., and De Master, K. (2016). After the incubator: factors impeding land access along the path from farmworker to proprietor. J. Agric. Food Syst. Commun. Dev. 6, 1–17. doi: 10.5304/jafscd.2016.062.018
Cardinale, B. J., Duffy, J. E., Gonzalez, A., Hooper, D. U., Perrings, C., Venail, P., et al. (2012). Biodiversity loss and its impact on humanity. Nature 486, 59–67. doi: 10.1038/nature11148
Cassman, K. G. (1999). Ecological intensification of cereal production systems: yield potential, soil quality, and precision agriculture. Proc. Natl. Acad. Sci. U.S.A. 96, 5952–5959. doi: 10.1073/pnas.96.11.5952
Cozen, M. (2010). The Making of the American Landscape. Routledge. Available online at: https://ebookcentral-proquest-com.dist.lib.usu.edu/lib/usu/detail.action?docID=1702299
Daniels, T. L. (1988). America's conservation reserve program: rural planning or just another subsidy? J. Rural Stud. 4, 405–411. doi: 10.1016/0743-0167(88)90008-3
Davis, A. S., Hill, J. D., Chase, C. A., Johanns, A. M., and Liebman, M. (2012). Increasing cropping system diversity balances productivity, profitability and environmental health. PLoS ONE 7:e47149. doi: 10.1371/journal.pone.0047149
Deryugina, T., and Konar, M. (2017). Impacts of crop insurance on water withdrawals for irrigation. Adv. Water Resour. 110, 437–444. doi: 10.1016/j.advwatres.2017.03.013
Di Falco, S., and Perrings, C. (2003). Crop genetic diversity, productivity and stability of agroecosystems. a theoretical and empirical investigation. Scott. J. Polit. Econ. 50, 207–216. doi: 10.1111/1467-9485.5002006
Di Falco, S., and Perrings, C. (2005). Crop biodiversity, risk management and the implications of agricultural assistance. Ecol. Econ. 55, 459–466. doi: 10.1016/j.ecolecon.2004.12.005
Dimitri, C., Effland, A. B. W., and Conklin, N. C. (2005). The 20th Century Transformation of U.S. Agriculture and Farm Policy. USDA ERS. Available online at: http://ageconsearch.umn.edu/bitstream/59390/2/eib3.pdf
Drummond, M. A., Auch, R., Karstensen, K. A., Sayler, K., Taylor, J., and Loveland, T. R. (2012). Land change variability and human-environment dynamics in the United States great plains. Land Use Policy 29, 710–723. doi: 10.1016/j.landusepol.2011.11.007
Drummond, M. A., and Loveland, T. R. (2010). Land-use pressure and a transition to forest-cover loss in the Eastern United States. Bioscience 60, 286–298. doi: 10.1525/bio.2010.60.4.7
Duffy, M. (2009). Economies of size in production agriculture. J. Hunger Environ. Nutr. 4, 375–392. doi: 10.1080/19320240903321292
Dunbar-Ortiz, R. (2014). An Indigenous Peoples' History of the United States. Boston, MA: Beacon Press.
ERS (1996). 1996 FAIR Act Frames Farm Policy for 7 Years. Agricultural Outlook Supplement April (1996). Washington, DC: ERS.
ERS (2000). Farm Resource Regions. Agricultural Information Bulletin 760. Washington, DC: USDA Economic Research Service. Available online at: https://www.ers.usda.gov/webdocs/publications/42298/32489_aib-760_002.pdf?v=42487
ERS (2019a). Agricultural Productivity in the U.S. USDA Economic Research Service. Available online at: https://www.ers.usda.gov/data-products/agricultural-productivity-in-the-us/
ERS (2019b). Agriculture Improvement Act of 2018: Highlights and Implications. Washington, DC: US Department of Agriculture, Economic Research Service. Avaliable online at: https://www.ers.usda.gov/agriculture-improvement-act-of-2018-highlights-and-implications/
Fahrig, L., Baudry, J., Brotons, L., Burel, F. G., Crist, T. O., Fuller, R. J., et al. (2011). Functional landscape eterogeneity and animal biodiversity in agricultural landscapes: heterogeneity and biodiversity. Ecol. Lett. 14, 101–112. doi: 10.1111/j.1461-0248.2010.01559.x
Falcone, J. A. (2015). U.S. Conterminous Wall-to-Wall Anthropogenic Land Use Trends (NWALT), 1974-2012. U.S. Geological Survey Data Series 948, 33 p. plus appendixes 3-6 as separate files. doi: 10.3133/ds948
Farm Bureau (2016). Options for Updating Bases and Yields. Farm Bureau: 2018 Farm Bill. Washington, DC: Farm Bureau. Avaliable online at: https://www.fb.org/files/2018FarmBill/Options_for_Updating_Bases_and_Yields.pdf
Fischer, J., Abson, D. J., Butsic, V., Chappell, M. J., Ekroos, J., Hanspach, J., et al. (2014). Land sparing versus land sharing: moving forward. Conserv. Lett. 7, 149–157. doi: 10.1111/conl.12084
Fischer, J., Brosi, B., Daily, G. C., Ehrlich, P. R., Goldman, R., Goldstein, J., et al. (2008). Should agricultural policies encourage land sparing or wildlife-friendly farming? Front. Ecol. Environ. 6, 380–385. doi: 10.1890/070019
Francis, C. A., Lieblein, G., Gliessman, S. R., Breland, T. A., Creamer, N., Harwood, R., et al. (2003). Agroecology: the ecology of food systems. J. Sustain. Agric. 22, 99–118. doi: 10.1300/J064v22n03_10
Graddy-Lovelace, G., and Diamond, A. (2017). From supply management to agricultural subsidies-and back again? the US farm bill & agrarian inviability. J. Rural Stud. 50, 70–83. doi: 10.1016/j.jrurstud.2016.12.007
Gustafson, E. J. (1998). Quantifying landscape spatial pattern: what is the state of the art? Ecosystems 1:15. doi: 10.1007/s100219900011
Harlan, J. R. (1975). Our vanishing genetic resources. Science 188, 617–621. doi: 10.1126/science.188.4188.617
Hart, J. F. (1978). Cropland concentrations in the South. Ann. Assoc. Am. Geogr. 68, 505–517. doi: 10.1111/j.1467-8306.1978.tb01213.x
Hart, J. F. (1991). Part-ownership and farm enlargement in the Midwest. Ann. Assoc. Am. Geogr. 81, 66–79. doi: 10.1111/j.1467-8306.1991.tb01679.x
Hart, J. F. (2004). The Changing Scale of American Agriculture. Charlottesville, VA: University of Virginia Press.
Hass, A. L., Kormann, U. G., Tscharntke, T., Clough, Y., Baillod, A. B., Sirami, C., et al. (2018). Landscape configurational heterogeneity by small-scale agriculture, not crop diversity, maintains pollinators and plant reproduction in Western Europe. Proc. R. Soc. B Biol. Sci. 285:20172242. doi: 10.1098/rspb.2017.2242
Heal, G., Walker, B., Levin, S., Arrow, K., Dasgupta, P., Daily, G., et al. (2004). Genetic diversity and interdependent crop choices in agriculture. Resour. Energ. Econ. 26, 175–184. doi: 10.1016/j.reseneeco.2003.11.006
Hendrickx, F., Maelfait, J. P., Van Wingerden, W., Schweiger, O., Speelmans, M., Aviron, S., et al. (2007). How landscape structure, land-use intensity and habitat diversity affect components of total arthropod diversity in agricultural landscapes: agricultural factors and arthropod biodiversity. J. Appl. Ecol. 44, 340–351. doi: 10.1111/j.1365-2664.2006.01270.x
Hillier, J., Hawes, C., Squire, G., Hilton, A., Wale, S., and Smith, P. (2009). The carbon footprint of food crop production. Int. J. Agric. Sustain. 7, 107–118. doi: 10.3763/ijas.2009.0419
Homer, C., Dewitz, J., Yang, L., Jin, S., Danielson, P., Coulston, J. W., et al. (2015). Completion of the 2011 National land cover database for the conterminous United States - representing a decade of land cover change information. Photogramm. Eng. Remote Sensing 81, 345–354. doi: 10.14358/PERS.81.5.345
Hooper, D. U., Adair, E. C., Cardinale, B. J., Byrnes, J. E. K., Hungate, B. A., Matulich, K. L., et al. (2012). A global synthesis reveals biodiversity loss as a major driver of ecosystem change. Nature 486, 105–108. doi: 10.1038/nature11118
Hooper, D. U., Chapin, F. S., Ewel, J. J, Hector, A., Inchausti, P., Lavorel, S., et al. (2005). Effects of biodiversity on ecosystem functioning: a consensus of current knowledge. Ecol. Monogr. 75, 3–35. doi: 10.1890/04-0922
Hoppe, R. A. (2014). Structure and Finances of US Farms: Family Farm Report, 2014 Edition. Economic Information Bulletin No. (EIB-132). Economic Research Service.
Horrigan, L., Lawrence, R. S., and Walker, P. (2002). How sustainable agriculture can address the environmental and human health harms of industrial agriculture. Environ. Health Perspect. 110, 445–456. doi: 10.1289/ehp.02110445
Horst, M., and Marion, A. (2019). Racial, ethnic and gender inequities in farmland ownership and farming in the US. Agric. Hum. Values 36, 1–16. doi: 10.1007/s10460-018-9883-3
Hudson, J. C. (1994). Making the Corn Belt: A Geographical History of Middle-Western Agriculture. Bloomington, IN: Indiana University Press.
Hudson, J. C., and Laingen, C. R. (2018). American Farms, American Food: A Geography of Agriculture and Food Production in the United States. Lanham, MD: Lexington Books.
Isik, M., and Yang, W. (2004). An analysis of the effects of uncertainty and irreversibility on farmer participation in the conservation reserve program. J. Agric. Resour. Econ. 29:18. doi: 10.22004/ag.econ.31113
Johnson, K. A., Dalzell, B. J., Donahue, M., Gourevitch, J., Johnson, D. L., Karlovits, G. S., et al. (2016). Conservation Reserve Program (CRP) lands provide ecosystem service benefits that exceed land rental payment costs. Ecosyst. Serv. 18, 175–185. doi: 10.1016/j.ecoser.2016.03.004
Johnson, R., and Monke, J. (2019). What Is the Farm Bill? CRS Report RS22131. Washington, DC: Congressional Research Service.
Key, N. (2019). Farm size and productivity growth in the United States corn belt. Food Policy 84, 186–195. doi: 10.1016/j.foodpol.2018.03.017
Khoury, C. K., Bjorkman, A. D, Dempewolf, H., Ramirez-Villegas, J., Guarino, L., Jarvis, A., et al. (2014). Increasing homogeneity in global food supplies and the implications for food security. Proc. Natl. Acad. Sci. U.S.A. 111, 4001–4006. doi: 10.1073/pnas.1313490111
Krippendorf, K. (2004). Content Analysis: An Introduction to Its Methodology, 2nd Edn. Thousand Oaks, CA: SAGE Publications, Inc. Available online at: https://www.jstor.org/stable/2288384?origin=crossref
Laingen, C. R. (2017). Creating a dynamic regional model of the US corn belt. Int. J. Appl. Geospat. Res. 8, 19–29. doi: 10.4018/IJAGR.2017100102
Landis, D. A. (2017). Designing agricultural landscapes for biodiversity-based ecosystem services. Basic Appl. Ecol. 18, 1–12. doi: 10.1016/j.baae.2016.07.005
Lehner, P. H., and Rosenberg, N. A. (2018). Farm bill to help farmers weather climate change. J. Food Law Policy 14, 39–54. Available online at: https://ssrn.com/abstract=3219464
Luttrell, C. B. (1973). The Russian Wheat Deal-Hindsight vs. Foresight. Federal Reserve Bank of St. Louis 55. doi: 10.20955/r.55.2-9.jiy
MacDonald, J. M., and Hoppe, R. A. (2017). Large Family Farms Continue to Dominate, US Agricultural Production. Statistic: Farm Economy, Amber Waves. Washington, DC: Economic Research Service.
Martin, A. R., Cadotte, M. W., Isaac, M. E., Milla, R., Vile, D., and Violle, C. (2019). Regional and global shifts in crop diversity through the anthropocene. PLoS ONE 14:e0209788. doi: 10.1371/journal.pone.0209788
Massawe, F., Mayes, S., and Cheng, A. (2016). Crop diversity: an unexploited treasure trove for food security. Trends Plant Sci. 21, 365–368. doi: 10.1016/j.tplants.2016.02.006
McDaniel, M. D., Tiemann, L. K., and Grandy, A. S. (2014). Does agricultural crop diversity enhance soil microbial biomass and organic matter dynamics? A meta-analysis. Ecol. Appl. 24, 560–570. doi: 10.1890/13-0616.1
McFadden, J. R., and Hoppe, R. A. (2017). The Evolving Distribution Of Payments From Commodity, Conservation, And Federal Crop Insurance Programs. Economic Information Bulletin Number 184. USDA Economic Research Service.
McGranahan, D. A., Brown, P. W., Schulte, L. A., and Tyndall, J. C. (2013). A historical primer on the US farm bill: supply management and conservation policy. J. Soil Water Conserv. 68, 67A−73A. doi: 10.2489/jswc.68.3.67A
McMinimy, M. A., Angadjivand, S., Aussenberg, R. A., Billings, K. C., Bracmort, K., Casey, A. R., et al. (2019). The 2018 Farm Bill (P.L. 115-334): Summary and Side-by-Side Comparison. Washington, DC: Congressional Research Service.
Meehan, T. D., Werling, B. P., Landis, D. A., and Gratton, C. (2011). Agricultural landscape simplification and insecticide use in the Midwestern United States. Proc. Natl. Acad. Sci. U.S.A. 108, 11500–11505. doi: 10.1073/pnas.1100751108
Minkoff-Zern, L. A., and Sloat, S. (2017). A new era of civil rights? latino immigrant farmers and exclusion at the United States department of agriculture. Agric. Hum.Values 34, 631–643. doi: 10.1007/s10460-016-9756-6
Mulvaney, R. L., Khan, S. A., and Ellsworth, T. R. (2009). Synthetic nitrogen fertilizers deplete soil nitrogen: a global dilemma for sustainable cereal production. J. Environ. Qual. 38, 2295–2314. doi: 10.2134/jeq2008.0527
National Agricultural Law Center (2019). United States Farm Bills. National Agricultural Law Center. Available online at: https://nationalaglawcenter.org/farmbills/
Newton, J. (2017). Modernizing Base Acres. Market Intel. Farm Bureau. Available online at: https://www.fb.org/market-intel/modernizing-base-acres
NRC (2010). Toward Sustainable Agricultural Systems in the 21st Century. National Research Council of the National Academies. Washington, DC: The National Academies Press.
O'Connor, A. (2012). Fence row to fence row: a examination of federal commodity subsidies. Kan. J. Law Pub. Policy. 21, 432–450.
O'Donoghue, E. J., Roberts, M. J., and Key, N. (2009). Did the federal crop insurance reform act alter farm enterprise diversification? J. Agric. Econ. 60, 80–104. doi: 10.1111/j.1477-9552.2008.00166.x
Olson, A. (2001). Federal farm programs - past, present and future-will we learn from our mistakes? Great Plains Nat. Resour. J. 1:6.
Ortiz-Bobea, A., Knippenberg, E., and Chambers, R. G. (2018). Growing climatic sensitivity of US agriculture linked to technological change and regional specialization. Sci. Adv. 4:eaat4343. doi: 10.1126/sciadv.aat4343
Paul, C. M., Nehring, R., Banker, D., and Somwaru, A. (2004). Scale economies and efficiency in US agriculture: are traditional farms history? J. Product. Anal. 22:22. doi: 10.1007/s11123-004-7573-1
Pellegrini, P., and Fernández, R. J. (2018). Crop intensification, land use, and on-farm energy-use efficiency during the worldwide spread of the green revolution. Proc. Natl. Acad. Sci. U.S.A. 115, 2335–2340. doi: 10.1073/pnas.1717072115
Phalan, B., Green, R., and Balmford, A. (2014). Closing yield gaps: perils and possibilities for biodiversity conservation. Philos. Trans. R. Soc. B Biol. Sci. 369:20120285. doi: 10.1098/rstb.2012.0285
Pimentel, D., and Burgess, M. (2014). “Chapter 2: environmental and economic costs of the application of pesticides primarily in the United States,” in Integrated Pest Management, eds D. Pimentel and R. Peshin (Dordecht: Springer), 47–71.
Pimentel, D., Harvey, C., Resosudarmo, P., Sinclair, K., Kurz, D., McNair, M., et al. (1995). Environmental and economic costs of soil erosion and conservation benefits. Science 267, 1117–1123. doi: 10.1126/science.267.5201.1117
Pridham, J. C., and Entz, M. (2008). Intercropping spring wheat with cereal grains, legumes, and oilseeds fails to improve productivity under organic management. Agron. J. 100:1436. doi: 10.2134/agronj2007.0227
Pywell, R. F., Heard, M. S., Woodcock, B. A., Hinsley, S., Ridding, L., Nowakowski, M., et al. (2015). Wildlife-friendly farming increases crop yield: evidence for ecological intensification. Proc. R. Soc. B Biol. Sci. 282:20151740. doi: 10.1098/rspb.2015.1740
R Core Team (2020). R: A Language and Environment for Statistical Computing. Vienna: R Foundation for Statistical Computing. Available online at: https://www.R-project.org/
Rabalais, N.N., Turner, R.E., and Wiseman, W.J. Jr. (2002). Gulf of Mexico Hypoxia, A.K.A “The Dead Zone.” Ann. Rev. Ecol. Syst. 33, 235–363. doi: 10.1146/annurev.ecolsys.33.010802.150513
Rada, N. E., and Fuglie, K. O. (2019). New perspectives on farm size and productivity. Food Policy 84, 147–152. doi: 10.1016/j.foodpol.2018.03.015
Ramankutty, N., Mehrabi, Z., Waha, K., Jarvis, L., Kremen, C., Herrero, M., et al. (2018). Trends in global agricultural land use: implications for environmental health and food security. Ann. Rev. Plant Biol. 69, 789–815. doi: 10.1146/annurev-arplant-042817-040256
Ray, D. K., Ramankutty, N., Mueller, N. D., West, P. C., and Foley, J. A. (2012). Recent patterns of crop yield growth and stagnation. Nat. Commun. 3:1293. doi: 10.1038/ncomms2296
Reganold, J. P., Jackson-Smith, D., Batie, S. S., Harwood, R. R., Kornegay, J. L., Bucks, D., et al. (2011). Transforming, US agriculture. Science 332, 670–671. doi: 10.1126/science.1202462
Reitsma, K. D., Dunn, B. H., Mishra, U., Clay, S. A., DeSutter, T., and Clay, D. E. (2015). Land-use change impact on soil sustainability in a climate and vegetation transition zone. Agron. J. 107, 2363–2372. doi: 10.2134/agronj15.0152
Rindfuss, R. R., Walsh, S. J., Turner, B. L., Fox, J., and Mishra, V. (2004). Developing a science of land change: challenges and methodological issues. Proc. Natl. Acad. Sci. U.S.A. 101, 13976–13981. doi: 10.1073/pnas.0401545101
Robinson, R. A., and Sutherland, W. J. (2002). Post-war changes in arable farming and biodiversity in Great Britain. J. Appl. Ecol. 39, 157–176. doi: 10.1046/j.1365-2664.2002.00695.x
Rosenberg, N., and Stucki, B. W. (2017). The butz stops here: why the food movement needs to rethink agricultural history. J. Food. Law. Policy. 13, 12–25.
Sayler, K. L., Acevedo, W., and Taylor, J. L. (2016). Status and Trends of Land Change in the Eastern United States-1973 to 2000. U.S. Geological survey professional paper 1794-D. Land Cover Trends. Washington, DC: USGS.
Sleeter, B. M., Sohl, T. L., Loveland, T. R., Auch, R. F., Acevedo, W., Drummond, M. A., et al. (2013). Land-cover change in the conterminous United States from 1973 to 2000. Glob. Environ. Change 23, 733–748. doi: 10.1016/j.gloenvcha.2013.03.006
Sohl, T., Reker, R., Bouchard, M. A., Sayler, K., Dornbierer, J., Wika, S., et al. (2016). Modeled historical land use and land cover for the conterminous United States. J. Land Use Sci. 11, 476–499. doi: 10.1080/1747423X.2016.1147619
Taylor, J. L., Acevedo, W., Auch, R. F., and Drummond, M. A. (2015). Status and Trends of Land Change in the Great Plains of the United States-1973 to (2000). U.S. Geological Survey Professional Paper 1794-B. Land Cover Trends. Washington, DC: USGS.
Tilman, D. (1999). Global environmental impacts of agricultural expansion: the need for sustainable and efficient practices. Proc. Natl. Acad. Sci. U.S.A. 96, 5995–6000. doi: 10.1073/pnas.96.11.5995
Tscharntke, T., Clough, Y., Wanger, T. C., Jackson, L., Motzke, I., Perfecto, I., et al. (2012). Global food security, biodiversity conservation and the future of agricultural intensification. Biol. Conserv. 151, 53–59. doi: 10.1016/j.biocon.2012.01.068
Tscharntke, T., Klein, A. M., Kruess, A., Steffan-Dewenter, I., and Thies, C. (2005). Landscape perspectives on agricultural intensification and biodiversity - ecosystem service management. Ecol. Lett. 8, 857–874. doi: 10.1111/j.1461-0248.2005.00782.x
USDA (1982). 1982 Census Publications. USDA Census of Agriculture Historical Archive. Avaliable online at: http://usda.mannlib.cornell.edu/usda/AgCensusImages/1982/01/51/121/Table-01.pdf
USDA (1992). 1992 Census Publications. USDA Census of Agriculture Historical Archive. Avaliable online at: http://usda.mannlib.cornell.edu/usda/AgCensusImages/1992/01/51/1575/Table-06.pdf
USDA NASS (2019a). 2017 Census of Agriculture Report: United States Summary and State Data. AC-17-A-51. Geography Area Series, Part 51. Washington, DC: United States Department of Agriculture.
USDA NASS (2019c). USDA National Agricultural Statistics Service Quick Stats. Available online at: https://quickstats.nass.usda.gov/
Vitalis, V. (2007). Agricultural subsidy reform and its implications for sustainable development: the New Zealand experience. Environ. Sci. 4, 21–40. doi: 10.1080/15693430601108086
Way, M. J., and van Emden, H. F. (2000). Integrated pest management in practice - pathways towards successful application. Crop Prot. 19, 81–103. doi: 10.1016/S0261-2194(99)00098-8
Weber, J. G., Key, N., and O'Donoghue, E. (2016). Does federal crop insurance make environmental externalities from agriculture worse? J. Assoc. Environ. Resour. Econ. 3, 707–742. doi: 10.1086/687549
Willis, B., and O'Brien, D. (2002). Summary and Evolution of U.S. Farm Bill Commodity Titles. Fayetteville, AK: National Ag Law Center.
Wright, C. K., and Wimberly, M. C. (2013). Recent land use change in the western corn belt threatens grasslands and wetlands. Proc. Natl. Acad. Sci. U.S.A. 110, 4134–4139. doi: 10.1073/pnas.1215404110
Keywords: land use, policy, sustainable agricultural systems, U.S., crop production
Citation: Spangler K, Burchfield EK and Schumacher B (2020) Past and Current Dynamics of U.S. Agricultural Land Use and Policy. Front. Sustain. Food Syst. 4:98. doi: 10.3389/fsufs.2020.00098
Received: 04 November 2019; Accepted: 09 June 2020;
Published: 21 July 2020.
Edited by:
Mark A. Drummond, United States Geological Survey (USGS), United StatesReviewed by:
Roger F. Auch, United States Geological Survey (USGS), United StatesJ. Arbuckle, Iowa State University, United States
Copyright © 2020 Spangler, Burchfield and Schumacher. This is an open-access article distributed under the terms of the Creative Commons Attribution License (CC BY). The use, distribution or reproduction in other forums is permitted, provided the original author(s) and the copyright owner(s) are credited and that the original publication in this journal is cited, in accordance with accepted academic practice. No use, distribution or reproduction is permitted which does not comply with these terms.
*Correspondence: Kaitlyn Spangler, kspangler@aggiemail.usu.edu