- 1UNESP, College of Agricultural Science, Dept. of Crop Science, Botucatu, Brazil
- 2Netherlands Institute of Ecology (NIOO-KNAW), Department of Microbial Ecology, Wageningen, Netherlands
- 3UNESP, College of Engineering, Department of Soil Science, Ilha Solteira, Brazil
- 4UNESP, College of Veterinary Medicine and Animal Science, Department of Animal Nutrition and Breeding, Botucatu, Brazil
- 5UNESP, College of Engineering, Department of Civil Engineering, Ilha Solteira, Brazil
- 6UNESP, College of Agronomic and Technological Sciences, Dracena, Brazil
- 7Institute of Environmental Biology, Ecology and Biodiversity, Utrecht University, Utrecht, Netherlands
In tropical regions, intercropping systems under no-tillage improve biomass quantity, soil conservation, and cash crop productivity. However, the optimal sowing time for forage species in these cropping systems is unknown. The objective of this study was to evaluate the effects of two sowing times of palisade and guinea grass on forage production and quality, soybean yield and soil chemical properties. Palisade and guinea grasses were sown for intercropping with maize or after maize silage harvest (hereafter succession) in an experiment carried out over three crop seasons. We evaluated forage dry matter production, pasture nutritive values, straw nutrient content, soybean leaf nutrients, yield, and soil fertility. The highest dry matter production was 8.1 Mg ha−1 for guinea grass in the intercropping system (sum of 3 cuts). Sowing forage after maize silage harvest provided 4% more crude protein compared with intercropping, regardless of grass species. Soybean yield was over 1.0 Mg ha−1 higher when soybean was cropped in succession compared with intercropping; however, the effects of the two forage grasses on soybean production were similar. Soil pH, calcium and magnesium content, cation exchange capacity, and base saturation were higher in the intercropping systems than in the succession systems, particularly when guinea grass was cultivated. Sowing guinea grass after maize harvest provided better forage quality, nutrient cycling, soybean yields, and soil chemical properties in tropical conditions.
Introduction
Leaving the straw and roots of successive crops on agricultural fields, such as under no-tillage systems (NTS), improves the physical, chemical, and biological properties of soil (Castro et al., 2015; Calonego et al., 2017). In tropical regions, soil quality can be improved by adopting integrated crop-livestock systems, intercropping, and crop rotation management under NTS (Costa et al., 2015; Moraes et al., 2019). These conservationist management practices preserve natural tropical resources (Crusciol et al., 2015), provide high yields of most grain crops (Pariz et al., 2017; Mateus et al., 2020) and are good options to increase food production during irregular periods of rain (Borghi et al., 2013). Worldwide, these crop systems can reduce poverty, allowing farmers to achieve better productivity and increase profits with minimum environmental impact (FAO – Food Agriculture Organization of the United Nations, 2017).
In tropical and sub-tropical regions, intercropping crops under NTS may be one of the best alternatives for farmers to gain income and simultaneously achieve sustainability and nutrient cycling (Carvalho et al., 2010; Mateus et al., 2020). Intercropping systems promote grain crop yields during the growing season, pasture production during the off-season, and remaining straw to maintain the NTS (Mateus et al., 2020). One of the key factors determining the adoption of these crop systems is their relative profitability compared with other land-use practices (Telles et al., 2018), as well as their perceived environmental benefits, which are recognized as a potential means of improving socioeconomic, biodiversity and environmental sustainability in many regions of the world (Franzluebbers and Stuedemann, 2014; FAO – Food Agriculture Organization of the United Nations, 2017). However, in regions with dry winters, high temperatures and water deficits, such as the Brazilian Cerrado and African Savannah, it is very difficult to establish and maintain effective NTS due to the high rate of straw decomposition on soil (Costa et al., 2014). In these environments, the selection of an appropriate plant species for use as cover crop is crucial to achieve the benefits of NTS.
For tropical soils, forage species such as palisade grass (Urochloa brizantha cv. Marandu; syn. Brachiaria brizantha cv. Marandu) and guinea grass (Megathyrsus maximum cv. Tanzania; syn. Panicum maximum cv. Tanzania) are recommended as cover crops (Crusciol et al., 2012; Pariz et al., 2017; Mateus et al., 2020). These grass species are drought tolerant, have deep root systems, produce high biomass, cycle nutrients, and maintain soil moisture through the cash crop cycle. In general, palisade and guinea grass provide adequate nutritional quality in intercropping systems and exhibit good potential for regrowth and pasture production during the off-season, thus increasing animal carrying capacity (Costa et al., 2016a; Pariz et al., 2017; Moraes et al., 2019). However, compared with grasses grown in succession, the physiological maturation of grasses intercropped with maize is more advanced, resulting in higher levels of cell wall components. The greater nutritional quality of intercropped forage grasses is of extreme importance for crop systems. Nutritional quality is measured by the balance between crude protein content and fiber digestibility, which affect the consumption of fodder by animals (van Soest et al., 1991).
Sowing these cover crop species in intercropping seems to be the best option for effective NTS establishment and maximum cover crop biomass production (Borghi et al., 2013; Mateus et al., 2020). Ceccon et al. (2013) reported higher soybean yield following maize intercropped with palisade and guinea grass compared with monocropped maize. In addition, Pacheco et al. (2017) observed that intercropping ruzigrass and maize improved nutrient cycling, and Pariz et al. (2016) found higher availability of P, K and Mg in the soil after intercropping of maize and palisade grass. Thus, intercropping grain crops with tropical perennial forage can increase food production (grain or silage + pasture) per unit area in tropical regions (Costa et al., 2016a).
Although the interspecific competition between intercropped plants is small (Borghi et al., 2013; Crusciol et al., 2015; Mateus et al., 2016), appropriate plant management and lower co-existence times can favor the development of both crops (cash crop and tropical perennial forage) by further reducing inter-specific competition (Crusciol et al., 2014). The time of grass sowing is an important factor for minimizing competition for water, light, and nutrients between the grass and cash crop (Crusciol et al., 2012). Environmental conditions also affect grass cover crop development; for example, the initial development of palisade and guinea grass is limited by low temperatures and insufficient water (Costa et al., 2005). Depending on the climatic conditions, sowing tropical fodder after harvesting commercial crops may not provide enough soil cover during the off-season (Costa et al., 2016a; Mateus et al., 2016). However, the best sowing period of these two forage species in tropical regions is not yet known.
The aim of this study was to evaluate the effects of two sowing times of palisade and guinea grass on forage production and quality, soybean yield, and soil chemical properties. We hypothesized that the sowing times (intercropped or in succession with maize silage) of palisade and guinea grass affect forage characteristics, remaining straw (mulch), soybean yield in succession, and soil fertility. To test this hypothesis, we evaluated the effects of different crop systems of maize silage with tropical perennial grasses (cultivated during the summer of the first two crop seasons) on (i) forage yield and chemical composition during winter, (ii) surface mulch (production, nutrient content, lignin/N ratio and C/N ratio) during spring, (iii) soybean cultivated during the summer of the third crop season (plant nutrition, production components and yield), and (iv) soil improvement in an experiment over three consecutive crop growing seasons in a tropical region with dry winters (Brazilian Cerrado).
Materials and Methods
Site Description
This experiment was carried out in Central-West Brazil (20°18'S, 51°22'W, 370 m above sea level). The climate in this region is classified as Aw, characterized by a tropical and humid climate with a rainy summer season and a dry winter, according Köppen (Unicamp – Centro de Pesquisas Meteorológicas e Climáticas Aplicadas a Agricultura., 2016). The long-term (1956–2013) average annual maximum and minimum temperatures are 31.3 and 18.4°C, respectively The precipitation rate, and maximum and minimum temperatures of the area of this study were measured (Table 1).
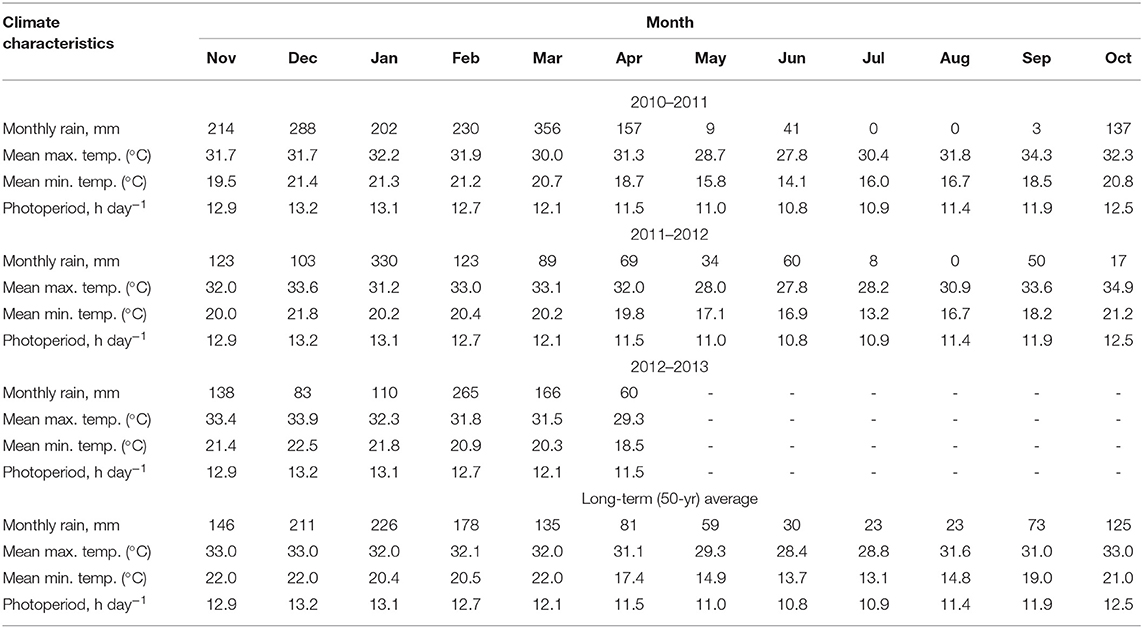
Table 1. Rainfall, maximum and minimum temperatures, and photoperiod at Selvíria, Mato Grosso do Sul State, Brazil, during the study period.
The soil of the experimental area is a Ferralsol (FAO – Food Agriculture Organization of the United Nations., 2006), clayey, with 482, 140, and 378 g kg−1 of clay, silt, and sand, respectively. Before October 2010, annual crops and semi-evergreen crops (maize, soybeans, sorghum, dwarf pigeon peas, palisade grass, beans, rice, and maize) were grown for 8 years in NTS. The chemical characteristics of the soil (0–0.20 m) were determined according to the methods described by van Raij et al. (2001). Before initiating the experiment, soil analyses indicated pH = 5.1, total soil organic matter = 25 g dm−3, P (resin) = 33 mg kg−1, exchangeable K, Ca, Mg, and total acidity at pH 7.0 (H + Al) = 4.1, 28, 16, and 29 mmolc kg−1, respectively, and base saturation = 48.1%. The soil pH was determined in a 0.01-mol L−1 CaCl2 suspension (1:2.5 soil/solution).
Experimental Design and Treatments
The experimental design was a randomized block, arranged in a 2 × 2 factorial scheme, with four replications. Treatments consisted of two different forages, palisade grass [Urochloa brizantha (A. Rich.) Stapf Marandu] and guinea grass [Megathyrsus maximus (Jacq.) B.K. Simon & S.W.L. Jacobs Tanzania] and two sowing times, i.e., the tropical grasses were sowing intercropped with maize at the same time, in alternative rows, according each treatment and sowed after maize silage harvest in the monocropped maize plots. The soybean (Glycine max (L.) Merrill) crop was sown in succession in crop rotation. The experiment was performed in the same location for three growing seasons. During the second growing season, the plots were established in the same location as the first growing season. The maize intercropped with palisade or guinea grass and forage in the off-season was carried out in 2010–2011, 2011–2012 and soybean in 2012–2013. The third growing season was a residual effect of intercropping systems from previous growing seasons with the same soybean crop system applied to all plots. Each plot consisted of four 20-m long maize rows that were spaced 0.90 m apart. The usable area was the two central rows; the 0.5 m at the end of each plot and the two external rows constituted the edge.
Tillage and Crop Management
Maize and Pasture
On November 22, 2010, the plants and weeds in the area were eliminated by applying glyphosate [isopropylamine salt of N-(phosphonomethyl) glycine] (1.44 g acid-equivalent ha−1) at a spray volume of 200 L ha−1. On November 26, 2010, the plants were cut using a plant residue crusher.
Triple hybrid BG 7049 maize [Zea mays (L.)] was sown on December 2, 2010–2011 for silage production. For the second growing season (2011–2012), simple hybrid AG 8088 YG was sown on December 10, 2011–2012. The same management plan was adopted in both growing seasons. The maize was sown at a depth of 0.05 m using a no-till drill, at a density of 60,000 seeds ha−1. For all treatments and during both growing seasons, the basic fertilization in the sowing furrows consisted of 20 kg ha−1 of N, 70 kg ha−1 of P2O5, and 40 kg ha−1 of K2O. Topdressing fertilization was conducted between maize rows without incorporation (90 kg ha−1 of N as urea) during both growing seasons when the maize plants had four expanded leaves. Subsequently, the experimental area was irrigated with 15 mm of water to minimize the N losses due of volatilization.
In the intercropping treatments, the grasses were sown at densities of 7 kg ha−1. The forage (palisade grass or guinea grasses) seeds were sown simultaneously alternating rows with the maize, on the same day, at a depth of 0.08 m below the soil surface, using a no-till drill with a row spacing of 0.34 m. When the maize grains reached the ¼-milk grain stage (grains with 34–35% moisture), the crops in each plot were harvested using a mechanical silage forage harvester (Model JF-90 with 12 knives). The cutting height of the species for silage was ~0.30 m above the soil surface.
On April 15, 2011 and April 12, 2012, the palisade grass and guinea grass were sown in the plots where the monocropped maize was cultivated. The sowing management of grasses in the off-season was the same as described previously in the crop season in intercropping systems.
Soybean in Crop Rotation
Soybean (Glycine max (L.) Merrill) “BRS Valiosa RR” was sown on October 30, 2012, at 4-cm depth, 0.45-m row spacing, and 260,000 seeds ha−1 density using a no-till seeding under palisade grass or guinea grass implanted during the intercropping or in succession after maize. Soybean seeds were inoculated with Bradyrhizobium japonicum (SEMIA 5079-CPAC 15 and SEMIA 5080-CPAC 7) at 5 g inoculant kg−1 seed. All soybean crop systems were fertilized in furrows with 90 kg ha−1 of P2O5 and 60 kg ha−1 of K2O. The soybean plants were harvested 127 days after emergence (at grain physiological maturity).
Sampling and Analyses
Forage Dry Matter Production (FDMP) and Pasture Nutritive Values
Forage dry matter production (FDMP) was determined in both treatments at 50 (first cut), 100 (second cut), and 150 days (third cut) after sowing the forage species in the plots in monocropping systems during the off-season. All leaves (0.30 m from the soil surface) within a 2 m2 area per plot were cut using a mechanical rotary mower. After cutting, all forage was removed from the plots, which was also performed using a mechanical rotary mower. This cutting height was used to provide faster forage regrowth. The collected material was dried by forced air circulation at 65°C for 72 h. The dry matter was weighed, and the data were extrapolated to kg ha−1 (FDMP). Samples were collected on June 4, 2011, July 24, 2011, and September 12, 2011 in the first growing season (2010–2011) and on June 1, 2012, July 21, 2012, and September 9, 2012 in the second growing season (2011–2012). In all cuts, the forage nutritional quality was determined. The crude protein (CP), neutral detergent fiber (NDF), and acid detergent fiber (ADF) content was determined according to the methods described by Silva and Queiroz (2002) and Association of Official Analytical Chemists (2005).
Remaining Straw and Nutrient Content
On October 19, 2011, and October 14, 2012, following pasture and weed desiccation with herbicide, estimates of plant dessicated material (i.e., remaining straw) was evaluated. All leaves (0.05 m above the soil surface) within a 2-m2 area per plot were cut using a mechanical rotary mower. After this management, the grasses in the plots were sprayed with glyphosate [isopropylamine salt of N-(phosphonomethyl) glycine] (1.44 g acid-equivalent ha−1), using a spray volume of 200 L ha−1, on October 25, 2011 and October 19, 2012.
Content of N, P, K, Ca, Mg and S were determined (Malavolta et al., 1997) in the accumulated straw. Lignin content was determined according to the method described by Silva and Queiroz (2002) and used to calculate the total lignin/N ratio. The C content (Tedesco et al., 1995) was determined to calculate the total C/N ratio, indicative of the durability of produced straw.
Soybean Agronomic Characteristics and Yield
Soybean leaf samples were collected from the upper third trifoliate at the R2 growth stage, full bloom (Fehr and Caviness, 1977). Petioles from 30 plants per plot were collected as proposed by Ambrosano et al. (1996). Leaf samples were washed with demi water and then dried under forced air circulation at 65°C for 72 h before grinding and analyzing for chemical composition. Contents of N, P, K, Ca, Mg, and S were determined using methods described by Malavolta et al. (1997).
The soybean plants were harvested 127 days after emergence, i.e., at physiological maturity. The plants contained in the four central rows were harvested to determine the soybean grain yield per plot, on March 12, 2013. The grains were weighed and corrected to a moisture content of 130 g kg−1. The calculated agronomic characteristics were the final plant population (PP, calculated from the number of plants in the four central rows, excluding 1 m from the end of each side of the row in each plot), plant height (PH), height of the first pod insertion (HFPI), number of pods per plant (NPP), number of grains per pod (NGP), and 100-seed weight (W100, calculated from eight random samples per plot).
Soil Fertility
Soil chemical attributes were determined after soybean harvest, on April 03, 2013. Five single soil samples from 0.0 to 0.20-m depth were collected per plot in the soybean crop interlines and subjected to soil analysis according to methods described by van Raij et al. (2001).
Statistical Analyses
All data were normally distributed (W > 0.90) according the Shapiro-Wilk Test using UNIVARIATE procedure (version 9.3; SAS Inst. Inc., Cary, NC, USA) (SAS Institute, 2015), with the results indicating that all data were distributed normally (W C 0.90). The homogeneity of variances was tested by Levene's test for residual errors. The data for all variables were analyzed using the PROC MIXED procedure of SAS and the Satterthwaite approximation to determine the denominator's degrees of freedom for the tests of the fixed effects. The sowing times and forage species were considered fixed effect. Data were analyzed using the replication (block), year, and block (sowing times × forage species) as random variables. The growing seasons and their interactions between sowing times and forage species were not significant at P <0.05 for any of the dependent variables. Thus, the data were combined for the growing seasons. The model statement that was employed to analyze the forage production encompassed the effects of the sowing times, forage species, cuts, and their resultant interactions. The specified term for the repeated statement was cuts, for the subject was replications (sowing times × forage species), and for the covariance structure utilized for all repeated statements was autoregressive, which provided the best fit for these analyses according to the Akaike information criterion (AIC). The results were reported as least square means (LSMEANS) and separated using the probability of differences (PDIFF) option. Mean separations were conducted using an LSD test. The effects were considered statistically significant at p ≤ 0.05.
Results
Forage Dry Matter Production (FDMP) and Pasture Nutritive Values
There was a significant effect of the sowing time × forage species interaction (p < 0.030) on forage dry matter production (FDMP) (sum of 3 cuts) (Table 2). The FDMP obtained during the off-season was highest when guinea grass was intercropped with maize compared to other treatments, reflection of this effect in the first cut (Figure 1).
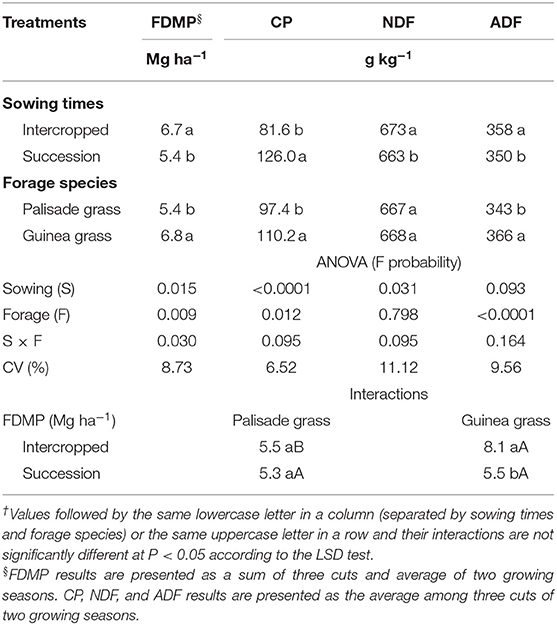
Table 2. Forage dry matter production (FDMP), crude protein (CP), neutral detergent fiber (NDF), and acid detergent fiber (ADF) as a function of sowing times and forage species.
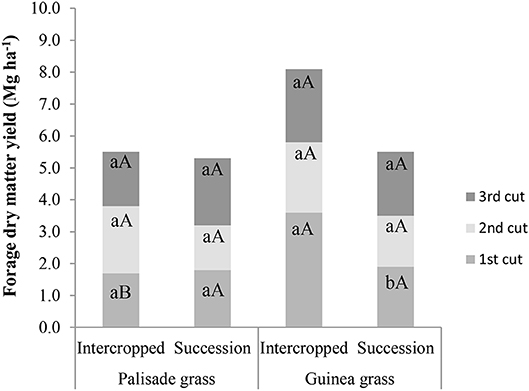
Figure 1. Forage dry matter production (FDMP) of three cuts as a function of sowing times and forage species (average of two growing seasons). Values followed by the same lowercase letter (comparison of sowing times for the same forage specie) or the same uppercase letter (comparison of forage species for the same sowing time) for each cut are not significantly different at P < 0.05 according to the LSD test.
Crude protein (CP) content was significantly influenced by sowing time (p < 0.0001) and forage species (p = 0.012) (Table 2). Guinea grass provided the highest CP content, and both species had the highest values when sown in succession to maize. Neutral detergent fiber (NDF) content differed based on sowing time (p = 0.031), and acid detergent fiber (ADF) differed (p < 0.0001) between forage species. For both species, NDF was lowest for sowing in succession, whereas ADF was lowest for palisade grass sown in succession to maize (Table 2).
Remaining Straw and Nutrient Content
There was a significant effect of the sowing time × forage species interaction on remaining straw and straw macronutrient accumulation (N, K, Ca, Mg, and S) (Table 3). Remaining straw production was highest for guinea grass sown in succession to maize (9.8 Mg ha−1) and was similar in the other treatments (~5.2–5.4 Mg ha−1). Nutrient accumulation was greatest in guinea grass sown in succession to maize due to the highest remaining straw production of this species. The straw production and nutrient accumulation of palisade grass were similar at the two sowing times. P accumulation (p < 0.001; p < 0.0001) and the lignin/nitrogen (LIG/N) (p = 0.032; p = 0.038) and carbon/nitrogen (C/N) (p = 0.009; p = 0.003) ratios differed significantly as a function of sowing time and forage species (Table 3). P accumulation was highest for guinea grass, regardless of sowing time. The highest LIG/N and C/N ratios were obtained in the grasses intercropped with maize in the summer. Guinea grass provided the highest LIG/N ratio, whereas palisade grass resulted in the highest C/N ratio (Table 3).
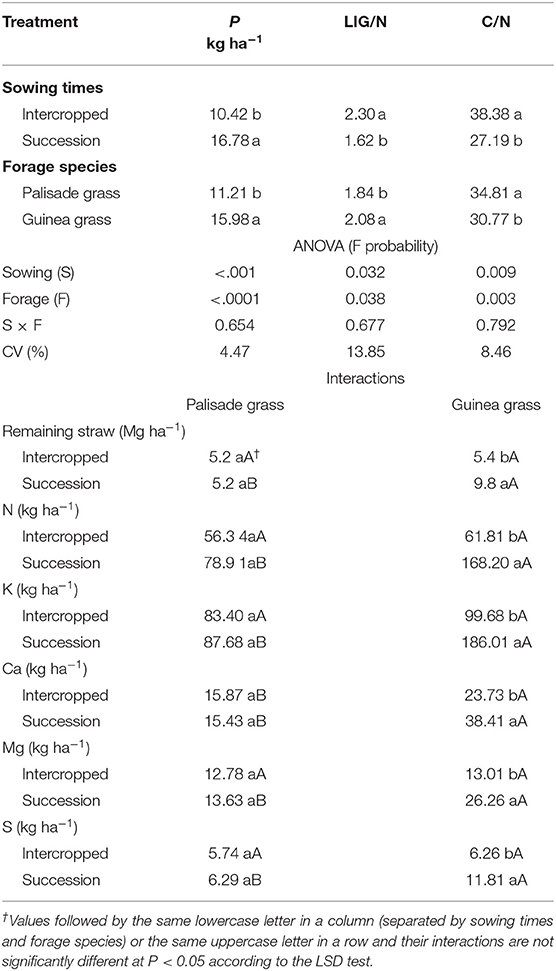
Table 3. Remaining straw production, nutrient content, lignin/N (LIG/N) and C/N ratio in forage species sown at different times over two growing seasons.
Soybean Agronomic Characteristics and Yield
The soybean leaf contents of K, Ca and S were significantly influenced by the sowing time × forage species interaction (Table 4). In general, K and S content were higher when soybean was sown after guinea grass in succession to maize, whereas Ca content was higher when soybean was sown after palisade grass intercropped with maize. By contrast, soybean leaf N, P, and Mg content were influenced by forage species and sowing time (Table 4). N content differed significantly based on the sowing time (p = 0.049) and forage species (p < 0.0001). Leaf N and P content were highest in soybean sown under guinea grass straw or after maize harvest. On the other hand, leaf Mg content was higher (p < 0.0001) when soybean was sown after intercropped maize or under palisade grass straw, similar to leaf Ca content (Table 4).
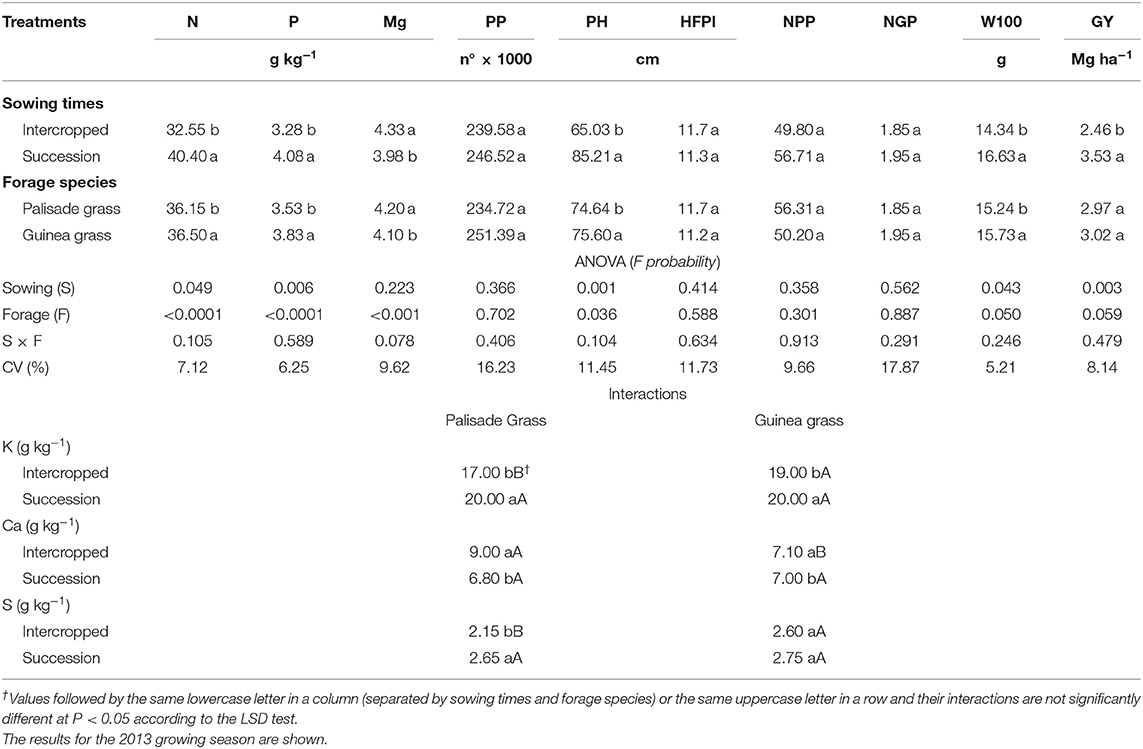
Table 4. Leaf nutrient content, plant population (PP), plant height (PH), height of the first pod insertion (HFPI), number of pods per plant (NPP), number of grains per pod (NGP), 100-seed weight (W100), and grain yield (GY) for soybean cultivated in crop rotation systems under forage species sown at different times as a cover crop.
With respect to agronomic parameters, none was influenced by the sowing time × forage species interaction (Table 4). The plant population (PP), height of the first pod insertion (HFPI), number of pods per plant (NPP), and number of grains per pod (NGP) were not significantly influenced by the sowing time or forage species. The plant height (PH), 100-seed weight (W100), were significantly influenced by the sowing time and forage species and grain yield (GY) by sowing time. Higher values were obtained when soybean was sown on straw produced by forage in succession to maize compared to the sown on straw produced by forage intercropped with maize (sowing times).
Soil Fertility
The soil chemical attributes were evaluated at the end of the experimental period. The changes in soil chemical properties (soil pH, exchangeable Ca and Mg, H + Al, CEC and BS) were greatest when the forage species were sown in succession to maize, particularly in the plots where guinea grass was cultivated (Table 5). These treatments provided soil with less acidity (pH ~ 5.0), higher levels of exchangeable Ca (78–81 mmolc dm−3) and Mg (13.6–14.6 mmolc dm−3), and lower values of H + Al (34.7–36.0 mmolc dm−3), resulting in higher CEC (78–81 mmolc dm−3), and BS (42–46%).
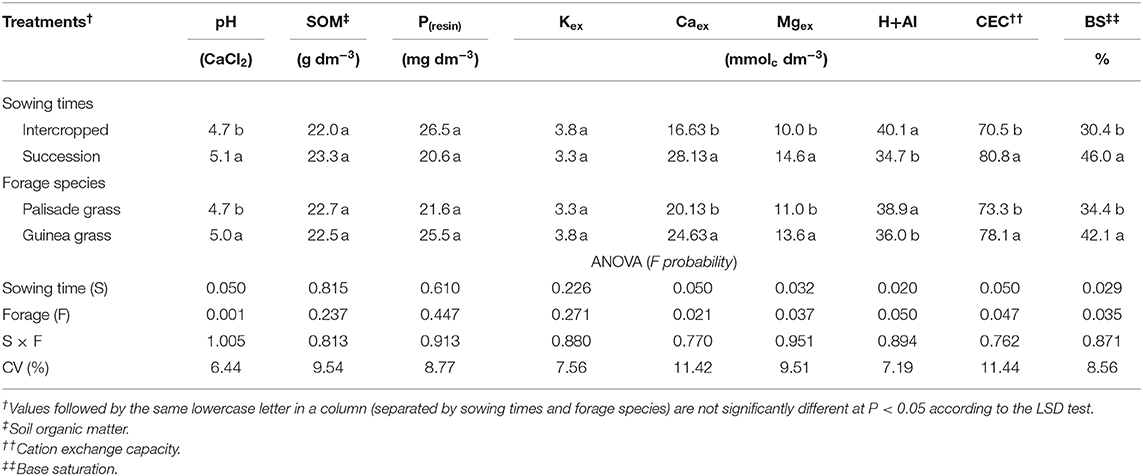
Table 5. Soil chemical characteristics of the experimental site at 0–0.20 m depth after soybean harvest.
Discussion
Forage Dry Matter Production (FDMP) and Pasture Nutritive Values
In the present study, the different sowing times of forage grasses in this specific tropical production systems differently improves soil quality and the soybean yield response, consequently increasing the FDMP provided by forage grasses, an important objective. Here was showed just guinea grass intercropped with maize strongly influenced the FDMP in the first cut (3.6 Mg ha−1) compared to other treatments (1.7–1.9 Mg ha−1) (Figure 1), reflecting in the sum of three cuts (8.1 Mg ha−1) compared to other treatments (5.3–5.5 Mg ha−1) (Table 2). These positive results are attributable to the climatic conditions after maize harvest in both agricultural years (15.5 and 28°C) (Table 1), which were suitable for the development of forage (Costa et al., 2005). Additionally, the three-month precipitation totals of 200 and 160 mm (growing seasons 2012 and 2013, respectively), guaranteed plant establishment, even when sown in succession to maize. The combination of adequate luminosity incidence and photosynthetic rates during forage development promoted FDMP. Guinea grass is competitive and has a higher N demand than palisade grass; therefore, guinea grass has a higher potential for biomass production (Mateus et al., 2016). Moreover, the FDMP values obtained in the current study are superior to previously reported values for tropical soils under similar management systems (Mateus et al., 2020). FDMP is an important index for farmers who need to provide food to livestock through mechanical cutting or for grazing (Pariz et al., 2011).
Moreover, crude protein is an important parameter of forage quality, which decreased in the palisade grass and intercropped (Table 2). The nutritional quality of pasture is an extremely important factor for animal weight gain, economic viability and greater agricultural sustainability in tropical regions. In the present study, crude protein exceeded 70 g kg−1 in all treatments, and the NDF and ADF values were within the necessary ranges for maintaining the population of microorganisms in the animal rumen and for good digestibility (van Soest et al., 1991). Therefore, both forage grasses are great options for Cerrado regions since both provided adequate FDMP and nutritional composition in the off-season.
Remaining Straw and Nutrient Content
The remaining straw (mulch) in NTS contributes to soil quality and protection and to nutrient cycling (Crusciol et al., 2015; Costa et al., 2016b; Pariz et al., 2017). For successful implementation of NTS in the tropics, sufficient remaining straw production is key for maintaining favorable conditions for successive planting, as straw is a slow-release source of nutrients for cash crops (Costa et al., 2016b). In contrast to the FDMP results, the amount of remaining straw was highest for guinea grass sown in succession to maize (9.8 Mg ha−1). In general, guinea grass has a higher biomass production capacity than palisade grass; however, this higher biomass productivity (the total for the three cuts) also increases the export of nutrients from the area, which can limit subsequent straw production. Guinea grass sown in succession to maize was best able to convert residual nutrients in the soil into biomass.
In the present study, forages sown in succession to maize showed lower lignin content and C/N ratios due to the younger age of the grasses, a strong indication that this plant material is capable of decomposing more quickly, cycling nutrients and improving soil quality (Costa et al., 2016b). The high production of remaining straw from guinea grass sown in succession to maize enabled greater accumulation of nutrients that can potentially return to the soil. Forage grasses with high remaining straw and nutrient accumulation (mainly N) provide a lower C/N straw ratio, accelerate the decomposition process and nutrient cycling, and increase the organic matter content of the soil (Mendonça et al., 2015), which are extremely important in tropical conditions.
Soybean Agronomic Characteristics and Yield
Soybean sown in the third agricultural year was positively influenced by sowing after forage species planted in succession to maize (regardless of forage species) or sowing after guinea grass (regardless of sowing time) (Table 4). Leaf N and P content and grain yield increased under these conditions. In addition, the leaf contents of K and S were highest when soybean was sown under the straw of both forage species planted in succession to maize. Ca content was highest when soybean was sown under palisade grass intercropped with maize. The grain yield of soybean was 1.07 Mg ha−1 higher when sown on straw produced by forage in succession to maize than that sown on straw produced by forage intercropped with maize (sowing times). The effects of the two forage grasses on soybean production were similar, only 0.05 Mg ha−1 higher when sown on straw produced by guinea grass than that sown on straw produced by palisade grass. Soybean benefits from the residual effect of nutrient cycling from predecessor crops, especially N and K (Crusciol et al., 2012; Pariz et al., 2016, 2017; Bossolani et al., 2018). The combination of both forage and maize residues provides soil cover for most of the soybean crop and results in lower soil temperature variation and higher moisture and greater soil decompaction and nutrient release as the plant and root residues decompose (Kliemann et al., 2006; Costa et al., 2014; Calonego et al., 2017). Furthermore, tropical grasses produce greater dry matter yield when following soybean in the rotation system because of the increase in N availability (Filizadeh et al., 2007; Pereira et al., 2016; Pariz et al., 2017). Thus, our results demonstrate positive effects of intercropping systems under NTS, as nutrient cycling due to straw decomposition and mineralization of the predecessor crops favors succeeding crops (Pereira et al., 2016; Pacheco et al., 2017; Pariz et al., 2017; Franzluebbers and Gastal, 2019).
Soil Fertility
Two years of maize cropping with forage species during or after maize cultivation and diversification with soybean altered soil chemical properties and macronutrient content. Specifically, the forage species in succession to maize (regardless of forage species) and guinea grass (regardless of sowing time) were associated with increased soil pH, exchangeable Ca and Mg, CEC and BS and lower H + Al content, probably due to the nutrient content in the crop residues (Brandan et al., 2017). The lower C/N ratio of remaining straw for forage sown in succession to maize (lower age) suggests that compared with intercropping, sowing forage in succession to maize could have greater potential to transform organic material into mineral nutrients, particularly in tropical regions with higher temperature and rainfall in the spring/summer season.
The results of this research will promote the sustainability of tropical soils because improving remaining straw (surface mulch) production and soil fertility in intercropping systems could enable a constant input of organic matter into the soil (Costa et al., 2015) to improve soil quality. Cropping systems that incorporate plant diversification through associations and rotations are sustainable and innovative (Moraes et al., 2019; Mateus et al., 2020). Species with high biomass production in the same area, such as maize, palisade grass, and guinea grass, result in a higher concentration of roots in the soil than under monocropping, and root exudates can reduce soil pH (Calonego et al., 2017). In the present study, the observed reduction in pH was not followed by a reduction in macronutrient content because NTS reduce pH values while increasing nutrient accumulation at the soil surface and soil organic matter (Castro et al., 2015).
Conclusions
Intercropping crops under NTS is very promising, but studies of the effectiveness of different evaluation parameters for achieving multiple objectives in tropical regions are lacking, particularly analyses of the maintenance of these crop systems for greater sustainability. In the current study, forage biomass production was highest for guinea grass intercropped with maize. Guinea grass had similar nutritive quality but higher crude protein levels than palisade grass. Sowing guinea grass after maize harvest increased soybean nutrient content and yield and improved soil properties after three consecutive growing seasons in tropical conditions. These results indicate that guinea grass can improve the productivity of crop systems and the long-term sustainability of tropical agriculture in the Brazilian Cerrado.
Data Availability Statement
The datasets generated for this study are available on request to the corresponding author.
Author Contributions
NC and MA: Design the experiment. NC, MA, CC, CP, JB, CL, and CB: Obtain and process the data. NC, AC, and JB: Analyze the data. NC, JB, and EK: Wrote the paper with contribution of all co-authors. All authors confirm being contributor of this work and has approved it for publication.
Funding
The authors would like to thank the São Paulo Research Foundation (FAPESP) for financial support (Grant No. 2011/01057-0) to the first author. In addition, the second and third authors would like to thank the National Council for Scientific and Technological Development (CNPq) for an award for excellence in research. Publication number 6969 of the Netherlands Institute of Ecology (NIOO-KNAW).
Conflict of Interest
The authors declare that the research was conducted in the absence of any commercial or financial relationships that could be construed as a potential conflict of interest.
References
Ambrosano, E. J., Tanaka, R. T., Mascarenhas, H. A. A., van Raij, B., Quaggio, J. A., and Cantarella, H. (1996). “Leguminosas e oleaginosas,” in Recomendações de adubação e calagem para o Estado de São Paulo, 2nd ed. Bol. Tec. 100. Inst. eds van B. Raij, J. A. Quaggio, H. Cantarella (Campinas, SP, Brazil: Agronômico) (IAC) 187–202. (In Portuguese)
Association of Official Analytical Chemists (2005). Official Method of Analysis, 18th edition. Gaithersburg, MD: AOAC Internatinal.
Borghi, E., Crusciol, C. A. C., Nascente, A. S., Souza, V. V., Martins, P. O., Mateus, G. P., et al. (2013). Sorghum grain yield, forage biomass production and revenue as affected by intercropping time. Eur. J. Agron. 51, 130–139. doi: 10.1016/j.eja.2013.08.006
Bossolani, J. W., Lazarini, E., Souza, L. G., Parente, T. D. L., Caioni, S., and Biazi, N. Q. D. (2018). Potassium doses in previous crops and effect on soybean in succession. Revista Brasileira de Engenharia Agrícola e Ambiental. 22, 90–94. doi: 10.1590/1807-1929/agriambi.v22n2p90-94
Brandan, C. P., Chavarría, D., Huidobro, J., Meriles, J. M., Brandan, C. P., and Gil, S. V. (2017). Influence of a tropical grass (Brachiaria brizantha cv. mulato) as cover crop on soil biochemical properties in a degraded agricultural soil. Eur. J. Soil Biol. 83, 84–90. doi: 10.1016/j.ejsobi.2017.10.009
Calonego, J. C., Raphael, J. P. A., Rigon, J. P. G., Oliveira Neto, L., and Rosolem, C. A. (2017). Soil compaction management and soybean yields with cover crops under no-till and occasional chiseling. Eur. J. Agron. 85, 31–37. doi: 10.1016/j.eja.2017.02.001
Carvalho, P. C. F., Anghinoni, I., Moraes, A., Souza, E. D., Sulc, R. M., Lang, C. R., et al. (2010). Managing grazing animals to achieve nutrient cycling and soil improvement in no-till integrated systems. Nutr. Cycl. Agroecosyst. 88, 259–273. doi: 10.1007/s10705-010-9360-x
Castro, G. S. A., Crusciol, C. A. C., Calonego, J. C., and Rosolem, C. A. (2015). Management impacts on soil organic matter of tropical soils. Vadose Zone J. 14:vzj2014.07.0093. doi: 10.2136/vzj2014.07.0093
Ceccon, G., Staut, L. A., Sagrilo, E., Machado, L. A. Z., Nunes, D. P., and Alves, V. B. (2013). Legumes and forage species sole or intercropped with corn in soybean-corn succession in Midwestern Brazil. Rev. Bras. Ci Solo. 37, 204–212. doi: 10.1590/S0100-06832013000100021
Costa, C. H. M., Crusciol, C. A. C., Soratto, R. P., and Ferrari Neto, J. (2016b). Phytomass decomposition and nutrients release from pearl millet, guinea grass and palisade grass. Biosci. J. 32, 1191–1203. doi: 10.14393/BJ-v32n5a2016-32982
Costa, K. A. P., Rosa, B., Oliveira, I. P., Custódio, D. P., and Silva, D. C. (2005). Effect of seasonal climate condition on the dry matter production and bromatological composition of Brachiaria brizantha cv. Marandu. Ci Anim Bras. 6, 187–193.
Costa, N. R., Andreotti, M., Buzetti, S., Lopes, K. S. M., Santos, F. G., and Pariz, C. M. (2014). Macronutrient accumulation and decomposition of brachiaria species as a function of nitrogen fertilization during and after intercropping with maize. Rev Bras Ci Solo. 38, 1223–1233. doi: 10.1590/S0100-06832014000400019
Costa, N. R., Andreotti, M., Crusciol, C. A. C., Pariz, C. M., Lopes, K. S. M., Yokobatake, K. L. A., et al. (2016a). Effect of intercropped tropical perennial grasses on the production of sorghum-based silage. Agron J. 108, 2379–2390. doi: 10.2134/agronj2016.07.0385
Costa, N. R., Andreotti, M., Ulian, N. A., Costa, B. S., Pariz, C. M., Cavasano, F. A., et al. (2015). Soybean yield on straw forages sown at different times and changes in chemical soil. Rev Bras Ci Agrárias. 10, 8–16. doi: 10.5039/agraria.v10i1a3842
Crusciol, C. A. C., Mateus, G. P., Nascente, A. S., Martins, P. O., Borghi, E., and Pariz, C. M. (2012). An innovative crop-forage intercrop system: early cycle soybean cultivars and palisadegrass. Agron J 104:1085–1095. doi: 10.2134/agronj2012.0002
Crusciol, C. A. C., Nascente, A. S., Borghi, E., Soratto, R. P., and Martins, P. O. (2015). Improving soil fertility and crop yield in a tropical region with palisadegrass cover crops. Agron J. 107:2271–2280. doi: 10.2134/agronj14.0603
Crusciol, C. A. C., Nascente, A. S., Mateus, G. P., Pariz, C. M., Martins, P. O., and Borghi, E. (2014). Intercropping soybean and palisadegrass for enhanced land use efficiency and revenue in a no till system. Eur. J. Agron. 58, 53–62. doi: 10.1016/j.eja.2014.05.001
FAO – Food and Agriculture Organization of the United Nations (2017). Integrated Crop-Livestock Systems (ICLS). Available online at: http://www.fao.org/agriculture/crops/core-themes/theme/spi/scpi-home/managing-ecosystems/integrated-crop-livestock-systems/en/ (accessed November 01, 2017).
FAO – Food and Agriculture Organization of the United Nations. (2006). World Reference Base for Soil Resources 2006: A Framework for International Classification, Correlation and Communication. WorldSoil Resources Rep. no. 103. Rome: FAO. Available online at: http://www.fao.org/nr/land/sols/soil/en/ (accessed June 6, 2017).
Fehr, W. R., and Caviness, C. E. (1977). Stages of Soybean Development. Ames: Iowa State University.
Filizadeh, Y., Rezzadeh, A., and Younessi, Z. (2007). Effects of crop rotation and tillage depth on weed competition and yield of rice in the paddy fields of Northern Iran. J. Agric. Sci. Technol. 10, 99–105.
Franzluebbers, A. J., and Gastal, F. (2019). Building agricultural resilience with conservation pasture-crop rotations. Agroecosyst. Divers. 1, 109–121. doi: 10.1016/B978-0-12-811050-8.00007-8
Franzluebbers, A. J., and Stuedemann, J. A. (2014). Crop and cattle production responses to tillage and cover crop management in an integrated crop–livestock system in the southeastern USA. Eur. J. Agron. 57, 62–70. doi: 10.1016/j.eja.2013.05.009
Kliemann, H. J., Braz, A. J. B. P., and Silveira, P. M. (2006). Decomposition rates of cover crop residues on a dystrophic oxisoL. Pesq. Agropec. Trop. 36, 21–28.
Malavolta, E., Vitti, G. C., and Oliveira, A. S. (1997). Avaliação do estado nutricional das plantas: princípios e aplicações. 2nd Edn. Piracicaba, SP: Associação Brasileira para Pesquisa da Potassa e do Fosfato, 319.
Mateus, G. P., Crusciol, C. A. C., Pariz, C. M., Borghi, E., Costa, C., Martello, J. M., et al. (2016). Sidedress nitrogen application rates to sorghum intercropped with tropical perennial grasses. Agron. J. 108, 433–447. doi: 10.2134/agronj2015.0236
Mateus, G. P., Crusciol, C. A. C., Pariz, C. M., Costa, N. R., Borghi, E., Costa, C., et al. (2020). Corn intercropped with tropical perennial grasses as affected by sidedress nitrogen application rates. Nut. Cycl. Agroecosyst. 116, 223–244. doi: 10.1007/s10705-019-10040-1
Mendonça, V. Z., Mello, L. M. M., Andreotti, M., Pariz, C. M., Yano, E. H., and Pereira, F. C. B. L. (2015). Nutrient release from forage straw intercropped with maize and followed by soybean. Rev. Bras. Ci Solo. 39, 183–193. doi: 10.1590/01000683rbcs20150666
Moraes, A., Carvalho, P. C. F., Crusciol, C. A. C., Lang, C. R., Pariz, C. M., Deiss, L., et al. (2019). Integrated crop-livestock systems as a solution facing the destruction of pampa and cerrado biomes in South America by intensive monoculture systems. Agroecosyst. Divers. 1, 257–273. doi: 10.1016/B978-0-12-811050-8.00016-9
Pacheco, L. P., São Miguel, A. S. D. C., Silva, R. G., Souza, E. D., Petter, F. A., and Kappes, C. (2017). Biomass yield in production systems of soybean sown in succession to annual crops and cover crops. Pesq. Agropec. Bras. 52, 582–591. doi: 10.1590/s0100-204x2017000800003
Pariz, C. M., Andreotti, M., Bergamaschine, A. F., Buzetti, S., Costa, N. R., Cavallini, M. C., et al. (2011). Yield, chemical composition and chlorophyll relative content of Tanzania and Mombaca grasses irrigated and fertilized with nitrogen after corn intercropping. Rev. Bras. Zootecn. 40, 728–738. doi: 10.1590/S1516-35982011000400005
Pariz, C. M., Costa, C., Crusciol, C. A. C., Meirelles, P. R. L., Castilhos, A. M., Andreotti, M., et al. (2016). Production and soil responses to intercropping of forage grasses with corn and soybean silage. Agron. J. 108:2541. doi: 10.2134/agronj2016.02.0082
Pariz, C. M., Costa, C., Crusciol, C. A. C., Meirelles, P. R. L., Castilhos, A. M., Andreotti, M., et al. (2017). Production, nutrient cycling and soil compaction to grazing of grass companion cropping with maize and soybean. Nutr. Cycl. Agroecosyst. 108, 35–54. doi: 10.1007/s10705-016-9821-y
Pereira, F. C. B. L., Mello, L. M. M., Pariz, C. M., Mendonça, V. Z., Yano, E. H., Miranda, E. E. V., et al. (2016). Autumn maize intercropped with tropical forages: crop residues, nutrient cycling, subsequent soybean and soil quality. Rev. Bras. Ci Solo. 40:e0150003. doi: 10.1590/18069657rbcs20150003
Silva, D. J., and Queiroz, A. C. (2002). Análise de alimentos: métodos químicos e biológicos. 3. ed. Viçosa: UFV, 235p.
Tedesco, M. J., Gianello, C., Bissani, C. A., Bohnen, H., and Volkweiss, S. J. (1995). Análises de Solo, Plantas e Outros Materiais. 2 Edn. Porto Alegre: Departamento de Solos UFRGS. 174.
Telles, T. S., Reydon, B. P., and Maia, A. G. (2018). Effects of no-tillage on agricultural land values in Brazil. Land Use Policy 76, 124–129. doi: 10.1016/j.landusepol.2018.04.053
Unicamp – Centro de Pesquisas Meteorológicas e Climáticas Aplicadas a Agricultura. (2016). Clima dos Municípios Paulistas, Botucatu. Cepagri Meteorologia-UNICAMP. Available online at: http://www.cpa.unicamp.br/outras-informacoes/clima_muni_086.html# (accessed 06 Jun. 2017).
van Raij, B., Andrade, J. C., Cantarella, H., and Quaggio, J. A. (2001). Análise Química Para Avaliação da Fertilidade de Solos Tropicais. Campinas: IAC, 284.
Keywords: intercropping crops, Glycine max, Megathyrsus maximus, Urochloa brizantha, sustainability, tropical agriculture
Citation: Costa NR, Andreotti M, Crusciol CAC, Pariz CM, Bossolani JW, Castilhos AM, Nascimento CAC, Lima CGR, Bonini CSB and Kuramae EE (2020) Can Palisade and Guinea Grass Sowing Time in Intercropping Systems Affect Soybean Yield and Soil Chemical Properties? Front. Sustain. Food Syst. 4:81. doi: 10.3389/fsufs.2020.00081
Received: 07 February 2020; Accepted: 05 May 2020;
Published: 04 June 2020.
Edited by:
Paulo Mazzafera, Campinas State University, BrazilReviewed by:
Andre Froes Borja Reis, Kansas State University, United StatesAgnieszka Barbara Najda, University of Life Sciences of Lublin, Poland
Copyright © 2020 Costa, Andreotti, Crusciol, Pariz, Bossolani, Castilhos, Nascimento, Lima, Bonini and Kuramae. This is an open-access article distributed under the terms of the Creative Commons Attribution License (CC BY). The use, distribution or reproduction in other forums is permitted, provided the original author(s) and the copyright owner(s) are credited and that the original publication in this journal is cited, in accordance with accepted academic practice. No use, distribution or reproduction is permitted which does not comply with these terms.
*Correspondence: Nídia Raquel Costa, nidiarcosta@gmail.com; Marcelo Andreotti, dreotti@agr.feis.unesp.br