- Sustainability Research Institute, School of Earth and Environment, University of Leeds, Leeds, United Kingdom
The severity and uneven distribution of the expected climate change impacts across climate-sensitive agricultural areas, and the cropping systems operated within, call for identification, and effective management of these impacts. The climate services have the potential to help identifying and adequately addressing the expected changes in climate and their impacts on agricultural production systems. However, the development of effective climate services is conditioned by the need to clearly understand the critical decisions that underpin end-users' activities and how climate information can support those decision-making processes. This paper reviews and identifies the main decisions linked to key climate change impacts on the cropping systems of interest—olive, grape and durum wheat—in order to inform the development of climate services for these crops in the future. Our review results indicate two types of key findings: (i) the most common types of decisions across the three cropping systems address the increase in temperature, variability, and uneven distribution of rainfall, occurrence of extreme events, and increased solar radiation; (ii) the most common decisions are likely to be affected by an increase in temperature above the maximum supported by the three crops, or in combination with other impacting climate changes. These decisions mainly relate to irrigation and other water stress reducing measures (olive, durum wheat), choice of varieties (grape, durum wheat), clones and rootstocks (grape), training system and vine load (olive, grape), canopy management (olive, grape), change in planting/sowing and harvest date (olive, durum wheat), pest and disease management (grape), allocation/choice of cultivation area (grape, durum wheat), use of decision support tools (grape), and crop insurance (durum wheat). In these decision-making contexts, the timely availability of climate information on temperature increase and rainfall variability can be used for developing climate services to effectively support the affected decisions. Although this paper does not provide an exhaustive analysis, the entry points identified can be considered as starting points for informing the development of climate services to further support the adjustment of decision making for the identified olive, grape, and durum wheat cropping systems, as well as similar decision-making contexts beyond those identified here.
Introduction
The impacts of climate change on the agricultural sector are intrinsically complex due not only to physical aspects, (e.g., climate conditions) but also socio-economic factors (e.g., cropping decisions; Iglesias et al., 2012). The dependence of agricultural practices as well as the production and quality of crops on weather and climate conditions make the agriculture sector particularly susceptible to climate change impacts (Ciscar et al., 2011; Lorencová et al., 2013; Giannakis and Bruggeman, 2015). Among the most impacting weather and climate conditions, the temperature is expected to register disparate increases across south-western (up to 5.1°C) and northern European areas (2.1°C) with large seasonal temperature differences across eastern European areas (between 0.5 and 2.5°C) (Malheiro et al., 2010; Iglesias et al., 2012; Salazar-Parra et al., 2018). The predicted pattern of annual mean rainfall, is expected to vary largely across the northern and southern European areas (Mechler et al., 2010), with differing increases and uneven distribution during the year across most of the northern European regions (Peltonen-Sainio et al., 2016) and differing decrease, across the lower latitudes, in most of the central (Heumesser et al., 2012) and the southern regions (Malheiro et al., 2010; Iglesias et al., 2012) The increase in frequency and severity of extreme weather events, such as drought, is expected in parts of the Mediterranean area (Iberian Peninsula, France, Italy, and Albania) as well as in parts of south-eastern Europe and central Europe and even in some humid areas, such as Northwest France and Southeast England, (Giannakis and Bruggeman, 2015; Carrão et al., 2016; European Environment Agency, 2017).
The Mediterranean Basin is considered as a hot spot for climate change (and associated socio-economic) impacts in Europe with cumulative and unevenly distributed impacts becoming increasingly severe (Giorgi, 2006; Guiot and Cramer, 2016). These impacts include increased frequency of extreme meteorological events (particularly drought), increased inter-annual climate variation, sea level rise, increased soil salinity and coastal erosion (Grasso and Feola, 2012; Cramer et al., 2019). Among these, a particularly severe impact is the increase in water stress (i.e., the ratio between actual and maximum plant transpiration; Fraga et al., 2016) due to increasingly frequent water shortages (Københavns Universitet, 2013; Dubrovský et al., 2014). Additionally, a temperature increase in the last 100 years and an expected increase of up to 2°C in the coming years together with a predominantly negative trend in precipitation in the last 50 years across the region; a decrease and variation of rainfall in the coming years; and limited ground water resources will have a direct impact on the occurrence of more frequent and severe droughts and overall accelerated drier conditions (Dubrovský et al., 2014; Ponti et al., 2014; Mereu et al., 2016; European Environment Agency, 2017). Furthermore, the coupled effect of warming and drought is expected to lead to a general increase in aridity and subsequent desertification of many Mediterranean areas (Cramer et al., 2019).
Of particular importance in the Mediterranean region are three key staple crops—olive, grape and durum wheat—which are of utmost cultural, economic and ecological importance, not only as they are critical to the Mediterranean diet, but also critical food commodities in the global market (Ponti et al., 2014; Eurostat, 2020).
The most common climate information required to inform agricultural planning and decision-making relates to temperature and water availability due to the strong influence that these two factors have on crop growth and development (World Meteorological Organization, 2012; Food Agriculture Organization, 2019). However, for effective planning and farming decisions making, it is critical that the necessary climate information is made available in a timely manner (Table 1).
In this context, the potential of climate services to provide “(…) people and organizations with timely, tailored climate-related knowledge and information that they can use to reduce climate-related losses” (Vaughan and Dessai, 2014, p. 588) can be critical to help identifying and adequately addressing the expected changes in climate and their impacts on European agriculture. Effective climate services are based on a clear understanding of the decision-making context and the key decisions whose services are expected to help inform and enhance (Vaughan and Dessai, 2014; Bruno Soares et al., 2018a; Vincent et al., 2018). As such, it is critical to understand the range of aspects affecting the critical decisions that underpin end-users activities and how climate information can be provided to support and enhance those decision-making processes. In the agricultural sector, these aspects often include the level of risk aversion (e.g., the perception of the influence of the climatic conditions on crop production), the production and profit objectives, and the level of knowledge and access to technical advice, all of which influence, to different extents, the decisions made to manage the expected impacts of climate change on various aspects of crop production (Bert et al., 2006).
To date, there is limited understanding of the linkages between expected climate change impacts and key agricultural-related decisions for the olive, grape, and durum wheat cropping systems. Such knowledge can help enhance those key decisions by supporting the development of climate services that are able to adequately provide the climate information required to address future climate change.
The aim of this paper is therefore to review and identify the main decisions linked (either explicit or implicit) to key climate change impacts on the cropping systems of interest—olive, grape and durum wheat—in order to inform the development of climate services and other decision-support tools for these crops in the future.
Methods
To pursue the analysis of the climate change impacts and key agricultural decisions in the three crops—olive, grape and durum wheat—we conducted a systematic literature review. This allowed us to review relevant literature systematically according to clearly formulated objectives and specific eligibility criteria (Ford et al., 2011). Our review followed the protocol for systematic reviews developed for social (Maki et al., 2018) and health sciences (Li et al., 2015; Singh, 2017) which included the following stages: search eligibility criteria, search results screening and data abstraction, risk bias assessment, and data synthesis and analysis. A detailed description of the protocol, as well as the eligibility criteria used to screen the literature, are covered in Appendix A.
The initial search retrieved 1,753 peer-reviewed articles and gray literature documents. The titles, abstracts and keywords of the search results were screened according to the eligibility criteria developed (see Appendix A). Following from this initial screening process, 80 documents (including both peer-reviewed and gray literature) were selected for the data abstraction phase. The overall limited number of retained studies can be understood as room for more research linking expected climate change impacts and key agricultural-related decisions for the olive, grape, and durum wheat cropping systems.
The literature was coded using NVIVO (New QSR International Pty Ltd, 2014) and a cluster analysis was performed on the NVIVO nodes to help us identify common climate change impacts and climate-related decisions across the three cropping systems of interest to this study.
It is important to clarify that the key decisions identified within the three crops under analysis were, in some instances, retrieved from different sources from those identifying the climate change impacts since in general, climate change impacts tend to be examined and reported separately from the key decisions that are affected by such impacts. In such instances, we made certain assumptions based on both the literature on agricultural-related decisions and activities as well as based on our expertise in the field in order to implicitly link the potential climate change impacts and how that may affect existing decisions on the ground.
To identify decision areas which could be supported/enhanced by climate services across similar climatic conditions, we identified locations situated at very similar latitudes to the ones in the reviewed studies and prone to similar expected impacts of climate change. The similar latitudes have been associated with annual temperature analogs, which can be used to better understand the challenges and opportunities that agricultural decision makers may face in the future by comparing the existing crop choices, cropping techniques, and procedures with those currently employed to manage the crops cultivated in climate analog locations. These climate analogs can be contextualized to provide practical, usable information about decision makers in different land use sectors managed within the context of their climate (Dunn et al., 2019). However, there were no climate analogs identified for annual rainfall, which limits the identification of types of decisions that can be informed by tailored climate services across similar latitudes.
For the identification of temperature analog locations, the latitude values for the reviewed specific study locations are indicated in the tables including the findings for the olive, grape, and durum wheat cropping systems. Moreover, because topography affects the meso-climate of each location (Dunn et al., 2019), the reviewed study locations have been divided into higher and lower areas.
Therefore, across the three cropping systems, the study locations were separated between “southern areas and lower latitudes of northern areas” and “northern areas and higher latitudes of southern areas.” The extended tables, including all study locations for all the three cropping systems, are presented in Appendix B and illustrated in Figure 1.
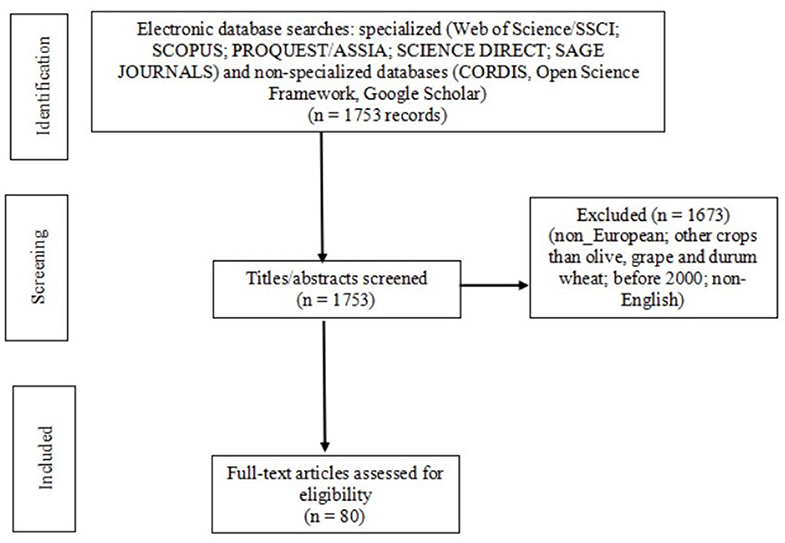
Figure 1. PRISMA flow diagram systematic review detailing the database searches, the number of abstracts screened and the full texts retrieved.
Results and Discussion
Linking Climate Change Impacts to Agricultural Decisions: Olive, Grape and Durum Wheat Cropping Systems
Changes in Temperature
Among the identified impacts, the most affecting one relates to the uneven increase of temperature during the cropping season. This affects the identified decisions across the olive, grape and durum wheat cropping systems, as determined by the expected impacts on the corresponding crops.
Table 2 presents the main expected changes in climate, their potential impacts and decisions that are expected to be affected across the olive, grape and durum wheat cropping systems (Appendix B includes the expected climatic changes, their impacts, affected decisions and study locations, for the three cropping systems).
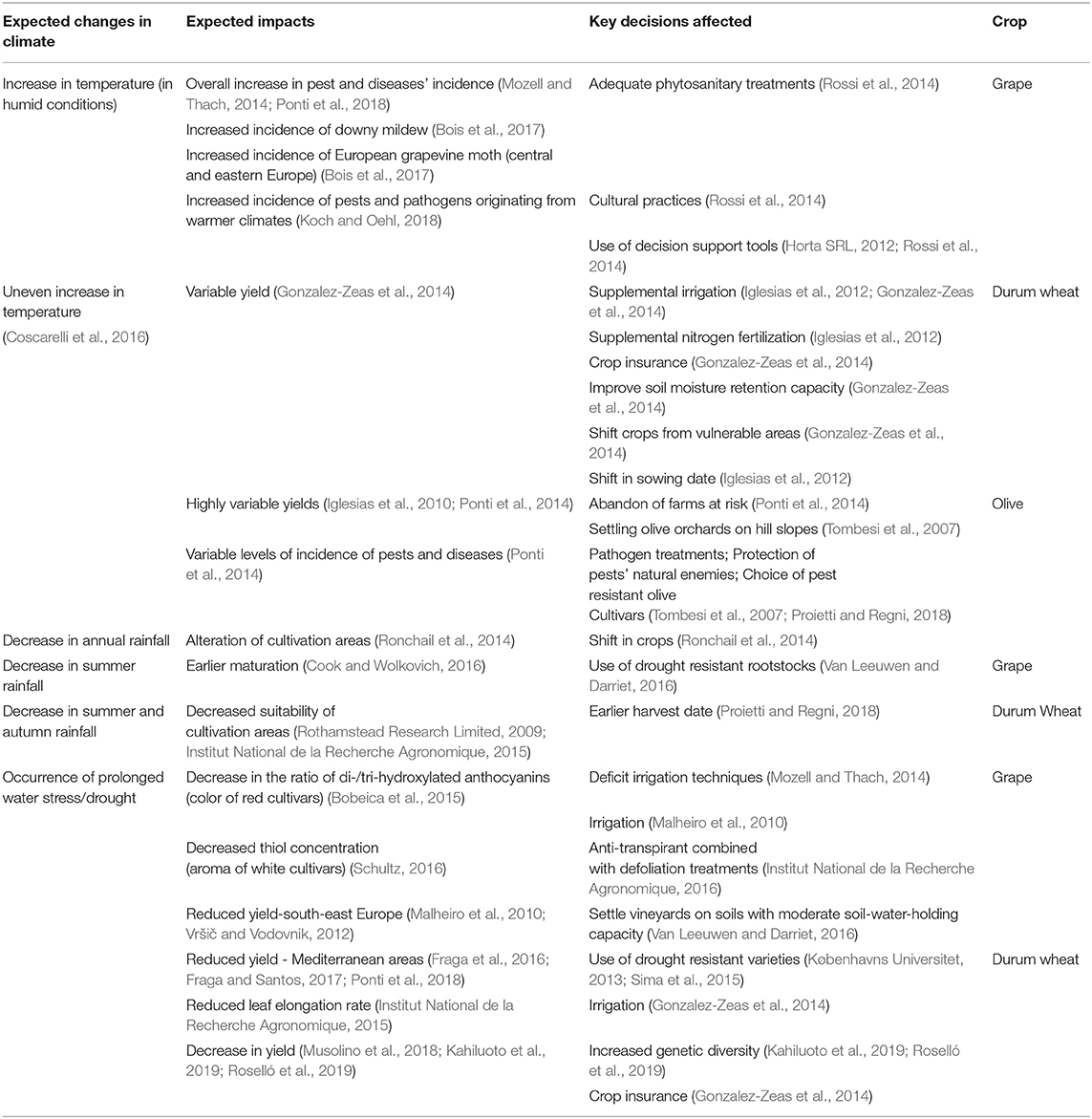
Table 2. Expected changes in climate, its impacts, and key decisions affecting olive, grape, and durum wheat crops.
For the olive and grape cropping systems, the identified types of decisions follow the southward and northward trends in the manifestation of the identified impacts of expected changes in climate.
For the olive cropping systems located in the southern areas/lower latitudes, the uneven increase in temperature affects the decisions addressing the water stress, altered development and phenology, yield, cultivation areas, variable incidence of pests and diseases, and occurrence of extreme events. In northern areas/higher latitudes, the decrease in temperature affects the decisions addressing the altered suitability of cultivation areas (Appendix B). However, these decisions are influenced by other factors besides the impacts of climate change on crops. For example, the decisions regarding the expected changes in olive yield are largely influenced at the local scale by aspects such as olive tree varieties, age structure, agronomic practices, and occurrence of pest and diseases, as they can affect the levels and variability of olive yields (Ponti et al., 2014).
The grape and wine producing areas have been delimited by the 12–13°C (lower threshold) and the 22–24°C (upper threshold) isotherm limits of the average growing season temperature which corresponds to latitudes between 30 to 50°N and 30° to 40°S. Geographically speaking, for Europe, the lower limit is the south of London, United Kingdom, and the upper limit includes the Euro-Mediterranean countries (Schultz and Jones, 2010). However, both of these limits can highly vary in the context of uneven increases of temperature across the grape and wine producing areas (Schultz, 2016).
For the grape cropping systems located in the southern areas/lower latitudes, the increase in temperature affects the decisions mainly addressing the altered phenology, berry development, and grape biochemistry of the red varieties, increased incidence of pests and diseases, altered suitability of grape varieties and of cultivation areas. In northern areas/higher latitudes, the increase in temperature affects the decisions mainly addressing the altered biochemistry and suitability of the white grape varieties (Appendix B). However, some of these decisions are influenced by other factors besides the impacts of climate change on grapes. For example, the changes in, and decisions regarding the, grape biochemistry are also affected by other factors such as increased solar radiation, adjusted viticultural techniques, and longer “hangtime” (Van Leeuwen and Darriet, 2016).
Due to the different crop (olive, grape) responses to the expected impacts of climate change, there have been identified very few common decisions including: canopy management, training techniques, and phytosanitary treatments, for the southern areas/lower latitudes, and choice of plantation settlement for the northern areas/higher latitudes (Appendix B).
The different decisions for the olive and grape cropping systems, mainly for the southern areas/lower latitudes, include irrigation and water stress reducing measures (careful control of weeds, low plant density, adequate intensity of pruning) (olive), rescheduled planting dates (olive), development of skills to quantify climate impacts (olive), abandon of farms at risk (olive), use of late ripening and warm climate clones (grape) and of decision support tools (grape) (Appendix B). However, the effectiveness of irrigation—as one of the main affected decisions for the olive cropping system—has been debated. Some authors stress that under increasing temperature conditions, an increased evapotranspiration rate requires a substantial increase in the amount of water needed for irrigation (Iglesias et al., 2010) which may make the olive production sector compete with other local water demanding sectors. One way to prevent this to happen would be to considerably reduce the volume of irrigation water during the stone hardening stage, when the olive fruit is less sensitive to water stress, and when a reduction in the seasonal volume of irrigation water does not negatively affect the yield levels (Tombesi et al., 2007).
Other decisions have been influenced by other factors. For example, the decision relating to abandon of farms at risk was valid in cases of severe pest infestation and loss of production (Ponti et al., 2014). The decision regarding the use of clones of traditional cultivars is largely affected by the compliance with existing regulations (Resco et al., 2016) for each grape and wine producing country.
For the durum wheat cropping systems, the identified decisions do not follow as clear regional trends as for the other 2 cropping systems, because the production of durum wheat in Europe is mainly concentrated in a few Mediterranean countries (Italy, Spain, France, Macedonia, Greece, Portugal, Cyprus, and Malta), all of which have similar climatic conditions (Ventrella et al., 2012; Ranieri, 2015; Moriondo et al., 2016). In these areas, the yield response indicates declining resilience to increasing temperatures, potentially due to continuously homogenizing and declining cultivars or genetic pool (Kahiluoto et al., 2019; Roselló et al., 2019).
The common decisions for durum wheat along with olive and grape cropping systems include irrigation (olive), use of drought resistant varieties (grape), and shifting the sowing date (olive). Different decisions for durum wheat cropping systems include supplemental nitrogen fertilization and crop insurance, increased genetic diversity. The fertilization is required because, under increasing temperature conditions, the general decrease in durum wheat productivity is caused by the shortening of the growing period with subsequent negative effects on the grain filling (Iglesias et al., 2012). The crop insurance is mainly chosen by bigger farmers (Sima et al., 2015).
To sum up, the common decisions for the southern areas (and south-eastern, for grape cropping system)/lower latitudes mainly address the impacts of water and heat stress, for all the three cropping systems. For the northern areas/higher latitudes, the affected decisions mainly address the impacts of extreme events (olive), and other climate-related impacts such as the shifts in traditional varieties, the reduction of white grape and wine quality, and the “import” of pests and diseases from the warmer climates, for grape cropping systems.
Decrease and Variability of Rainfall
Another identified impact of climate change is the uneven decrease and rainfall distribution pattern during the cropping year, which affects differently the operationality of the three cropping systems.
For the olive cropping systems in the southern areas/lower latitudes, the affected decisions are similar to some of the decisions for the impacts of increased temperature in the same areas (irrigation and water stress reducing measures, shift in crops). However, as olive tree has reduced water requirements, it can be used as an alternative to crops which exhibit a higher sensitivity to climate change, as is the case for grapevine (Migliore et al., 2019). The decision regarding the shift in crops was also influenced by a drop in the cereal market price determining a shift from cereals (less resistant to water stress) to olive trees (known as being tolerant to dry conditions) (Ronchail et al., 2014) (Appendix B).
For the grape cropping systems in the southern areas/lower latitudes, the affected decisions are more variate than for the olive cropping system, including irrigation, training techniques, management of soil conditions, canopy management, use of drought resistant rootstocks (Appendix B). These decisions are critical where increasingly severe dry conditions are expected, such as across the wine regions in south-eastern Europe (Vršič and Vodovnik, 2012), across some of the Mediterranean areas (e.g., southern Iberia, Emilia Romagna and Lombardy in Italy and along the Aegean Sea; Malheiro et al., 2010; Fraga et al., 2016; Fraga and Santos, 2017), as well as in central Europe, particularly during summer (Heumesser et al., 2012). Differently, in the northern areas/higher latitudes, the reduced rainfall coupled with increased temperature trigger the “import” of some diseases (fan leaf and leaf roll-associated viruses) from warmer climate. In these areas, the most common decisions identified relate to adequate phytosanitary treatments, cultural practices (pruning, burning of infested organs, tillage and hoeing control soil-borne insects), and the use of decision support tools (vite.net; Rossi et al., 2014 and MODEM_IVM DSS; Horta SRL, 2012) (Appendix B).
The decisions for the durum wheat cropping systems are similar to the ones addressing the impacts of increased temperature. These decisions are critical in the durum wheat cultivation areas at increasing risk in terms of their climatic suitability due to: (i) increasingly frequent water shortages (Københavns Universitet, 2013; Dubrovský et al., 2014) and (ii) a decrease and variation of rainfall and limited ground water resources (Fraga et al., 2016). Additional to the increasingly dry conditions, the decreasing genetic potential of durum wheat varieties is being emphasized as another important contributor to decreasing and variable durum wheat yields. Therefore, measures like increasing the genetic diversity of durum wheat varieties are gaining increasing attention (Moriondo et al., 2016; Kahiluoto et al., 2019; Roselló et al., 2019).
To sum up, the only common decisions for the three cropping systems relate to different irrigation techniques and water stress reducing measures adjusted to the specificity of each cropping system and local context.
Occurrence of Prolonged and More Frequent Extreme Events
For the olive cropping systems located in the northern areas/higher latitudes, the identified extreme events include frequent late spring and early autumn frost, severe winds, increase in the hail amount and frequency. The affected decisions include changing the harvest date, choice of the plantation settlement, and phytosanitary treatments (Appendix B). In the southern areas/lower latitudes, the impacts of the occurrence of prolonged/repeated water deficit, the affected decisions relate to specific irrigation techniques (Appendix B).
For the grape cropping systems in the southern areas/lower latitudes and the durum wheat systems, the main expected extreme event is the occurrence of prolonged water stress/drought. The affected decisions are similar to the ones discussed for the decrease in rainfall (Appendix B).
For the grape cropping systems in the northern areas/higher latitudes, the increased solar insolation may contribute to increased suitability of the northern grape cultivation areas, on one hand. On the other hand, for the plantations located at high altitudes, the increased solar insolation (and UV-B radiation, in particular) has been identified as contributing, for example, to the induction of off-flavors in white grapes (Van Leeuwen and Darriet, 2016). In these plantations, the detrimental impacts of high solar radiation can be limited by canopy management (late leaf removal), adapted training system, use of more adaptable grape varieties, and the use of special nets that filter UV-B radiation which protect the grape bunch zone (Appendix B).
To sum up, due to the variety of the expected extreme events, there were very few common affected decisions across the three cropping systems, including irrigation techniques (all three cropping systems) and use of drought resistant varieties (grape and durum wheat cropping systems) (Appendix B).
Overall, there is no common decision suiting the responses of all the three crops to the identified impacts of climate change, due to agronomic differences, as well as other factors, such as the decision makers' level of risk aversion, their production and profit objectives, and their level of knowledge and access to climate information.
Identifying Entry-Points for Climate Services
Climate services can help those operating in the agricultural sector with identifying expected changes in climate and its impacts on their activities as well as taking advantage of opportunities to enhance their operations through the use of tailored climate information (Vaughan and Dessai, 2014). Such services can help to better prepare for the occurrence of extreme events, better understanding trends for the next growing season as well as supporting other decisions related to planning activities, production and workforce (Hansen, 2002; Vaughan and Dessai, 2014; Bruno Soares, 2017). However, the use of climate information—both at seasonal and longer-term timescales—to support agricultural decisions and activities is still somewhat limited in Europe (Bruno Soares et al., 2018b).
An example of recent efforts to develop tailored climate information for the agriculture sector is the Global Agriculture Project. This initiative, funded by the European Commission as part of the Copernicus Climate Change Service (C3S), provides different products including crop related indicators (e.g., crop productivity, harvest indices and crop water use) as well as agricultural water resource indicators (e.g., soil moisture, surface- and groundwater availability, river discharge and reservoir status) (European Commission, 2019a). Also pursued in the context of the C3S is the “Agricultural Climate Advisory Services” (AgriCLASS) project which developed tools aimed at specific Mediterranean crops such as grape and olive. These tools enable the tracking of growing degree days across Europe (for the past, present, and future), monitoring of grape growth (Huglin Index), determining the onset, duration and magnitude of drought condition (Standardized Precipitation Evapotranspiration Index), and calculating the day of first annual appearance of olive fruit fly in spring (spring flight) (European Commission, 2019b). Another example is the “Vineyards' Integrated Smart Climate Application” (VISCA) project whose decision-support tool integrated climatic and phenological data to supply climate-informed decisions to the wine industry. Such decisions included those related to crop forcing, irrigation techniques, pruning and canopy management using short-term and mid-term forecasts for temperature, wind speed, accumulated precipitation, and relative humidity (Porras et al., 2017; Rossi et al., 2018).
Table 2 provides an overview of the information regarding the range of expected changes in climate conditions, expected impacts from such changes, and the key decisions that will be affecting olives, grapes and durum wheat crops. These decisions can be considered as entry points for the development of effective climate services, tailored for specific users' needs.
For example, an increase in temperature above the maximum threshold supported by the three crops (or in combination with other climate changes e.g., rainfall variation, drought occurrence, elevated CO2 levels, soil moisture deficit) is likely to affect decisions relating to irrigation and other water stress reducing measures (olive and durum wheat), the choice of varieties (grape and durum wheat), the type of clones and rootstocks (grape), the training system and vine load (olive and grape), canopy management (olive and grape), change in planting/sowing and harvest date (olive and durum wheat), pest and disease management (grape), allocation/choice of cultivation area (grape and durum wheat), use of decision support tools (grape), and crop insurance (durum wheat).
The occurrence of severe and prolonged dry conditions, associated with prolonged water stress, is likely to affect decisions relating to irrigation and other water stress reducing measures (all 3 cropping systems), use of drought resistant varieties (grape, durum wheat), crop insurance (durum wheat), adjusted trellis' height (grape). The variation and decrease in rainfall below the minimum water requirements or at critical development stages (fruit setting and development) are likely to affect decisions associated with irrigation and other water stress reducing measures (olive), shift in crops (olive, durum wheat), and use of drought resistant rootstocks (grape).
Other changes in climate and related conditions such as frost, hail, severe winds, solar radiation and salinity are also likely to affect these cropping systems and, as such, will require the implementation of adequate measures to address those challenges (Appendix B).
As depicted from Table 2, many of the decisions around the production and management of the olive, grape and durum wheat crops will be affected by changes in seasonal and long-term climate change patterns. For example, an increase in seasonal temperature can inform the decision to change for grape varieties more resistant to warmer climates. Similarly, a decrease in expected annual rainfall, particularly spring and summer rainfall, below water requirements required by olive trees can help support key decisions for implementing water-stress reduction measures or even the decision to shift to alternatives crops. Curiously, an expected decrease in summer rainfall can potentially affect all three crops—olive, grape and durum wheat—although the decisions associated with these changes would differ depending on the crops' responses. An increase in the occurrence of other climate-related phenomena, such as, for example droughts, can also help support a number of key decisions such as the use of drought-resistant durum wheat varieties, improving irrigation systems and/or take on crop insurance in order to cover any potential risks associated to the occurrence of drought conditions.
Understanding the type of climate information required (in terms of specific variables, temporal scale and prediction lead time) to support key decisions for these staple Mediterranean crops is a first step to help us understand the type of climate services that may be critical to support and inform the production and management of these crops in the future (Bruno Soares, 2017; Bruno Soares et al., 2018a; Falloon et al., 2018). Such climate services will need to be developed to fit the specific decision-making contexts of the various crops but also shaped by the decision-makers' level of risk aversion, their production and profit objectives, and their level of knowledge and access to climate information (Lemos et al., 2012).
This paper does not provide an exhaustive analysis but rather a synthesis of the entry points identified which can be regarded as a starting point for developing tailored climate services for the olive, grape, and durum wheat cropping systems, as well as for similar decision-making contexts to the ones identified in this study.
One remaining gap is that no climate analogs for annual rainfall were identified, which limits the identification of types of decisions that can be informed by tailored climate services. As explained in section Methods, the climate analogs can be contextualized to provide practical, usable information about decision makers in different land use sectors managed within the context of their climate (Dunn et al., 2019).
Conclusions
The aim of this paper is to review and identify the main decisions linked (either explicit or implicit) to key climate change impacts on the cropping systems of interest—olive, grape and durum wheat—in order to inform the development of climate services and other decision-support tools for these crops in the future.
The type of decisions identified as being linked to the most common impacts of climate change on olive and grape cropping systems mainly address the distinct regional trends (north, south, south-east) in the manifestation of these impacts. For the southern areas and lower latitudes of northern areas, the most common identified impacts related to: (i) reduced suitability of olive cultivation areas, decreases and variability of olive yields, limited olive fruit development and reduced olive oil quality (olive cropping system); and (ii) reduced suitability of cultivation areas, limited grape development, altered phenology and grape biochemistry, and reduced yields (grape cropping system). For the northern areas and higher latitudes of southern areas, the most common identified impacts related to: (i) reduced suitability of cultivation areas and damages of olive trees and fruits (olive cropping system) and (ii) shift in grape cultivars and altered grape biochemistry, (grape cropping system). For the durum wheat cropping system, the identified impacts did not follow distinct regional trends and were related to reduced suitability of cultivation areas, impaired plant development and phenology, and yield variability.
The most common decisions are likely to be affected by an increase in temperature above the maximum supported by the three crops, or in combination with other climate changes (rainfall variation, drought occurrence, elevated CO2 levels, soil moisture deficit). These decisions mainly relate to irrigation and other water stress reducing measures (olive and durum wheat), choice of varieties (grape and durum wheat), clones and rootstocks (grape), training system and vine load (olive and grape), canopy management (olive and grape), change in planting/sowing and harvest date (olive and durum wheat), pest and disease management (grape), allocation/choice of cultivation area (grape and durum wheat), use of decision support tools (grape), and crop insurance (durum wheat).
In these decision-making contexts, the timely availability of climate information on temperature increase can be used for developing climate services to effectively support the affected decisions.
Although this paper does not provide an exhaustive analysis, the entry points identified can be considered as a starting point for informing the development of climate services which can further support the adjustment of decision making for the olive, grape, and durum wheat cropping systems in the study locations, as well as for similar decision-making contexts beyond those identified in this study. Currently, such services are limited for these three cropping systems in Europe, most likely due to the fact that such developments are still evolving in Europe.
Author Contributions
All the stages of the protocol of the systematic review, as described in Appendix A, were carried out by EM. Writing of the abstract, introduction, methods, and Results and Discussion sections was done by EM. Writing of the Conclusions and Identifying entry-points for climate services sections was done by MB. MB also revised all the other sections of the manuscript.
Funding
This research was funded by the MED-GOLD (Turning climate-related information into added value for traditional MEDiterranean Grape, OLive and Durum wheat food systems) project through the European Union's Horizon 2020 research and innovation programme under grant agreement No. 776467.
Conflict of Interest
The authors declare that the research was conducted in the absence of any commercial or financial relationships that could be construed as a potential conflict of interest.
Acknowledgments
The authors wish to thank the MED-GOLD team, including: Luigi Ponti, Sandro Calmanti, Alessandro Dell'Aquila, Maurizio Calvitti, Sergio Musmeci, Adolfo Rosati, Chiara Monotti, Marco Silvestri, Cesare Ronchi, Luca Ruini, Federico Caboni, Luca Pinna, Michela Secci, Nube Gonzalez-Reviriego, Albert Soret, Marta Terrado, Raül Marcos, Ilaria Vigo, Marco Turco, Massimiliano Pasqui, Piero Toscano, Edmondo Di Giuseppe, Javier Lopez, Silvia Lopez, Rafael Sanchez de Puerta, Ricardo Arjona, Pedro Carrillo, Almudena Sanchez, Antonio Tabasco, Freddy Wilmer Rivas González, Pierluigi Meriggi, Matteo Ruggeri, Valentina Manstretta, Tiziano Bettati, Andrea Toreti, Matteo Zampieri, Michael Sanderson, Erika Palin, Christos Giannakopoulos, Anna Karali, Maria Hatzaki, Ioannis Lemesios, Konstantinos V. Varotsos, Aris Dadoukis, Antonio Graça, Natasha Fontes, Marta Teixeira, Suraje Dessai, Stavroula Maglavera, Athanasios Korakis, José Ricardo Cure, Daniel Rodríguez.
Supplementary Material
The Supplementary Material for this article can be found online at: https://www.frontiersin.org/articles/10.3389/fsufs.2020.00064/full#supplementary-material
References
Bert, F. E., Satorre, E. H., Toranzo, F. R., and Podesta, G. P. (2006). Climatic information and decision-making in maize crop production systems of the Argentinean Pampas. Agric. Syst. 88, 180–204. doi: 10.1016/j.agsy.2005.03.007
Bobeica, N., Poni, S., Hilbert, G., Renaud, C., Gomès, E., Delrot, S., et al. (2015). Differential responses of sugar, organic acids and anthocyanins to source-sink modulation in Cabernet Sauvignon and Sangiovese grapevines. Front. Plant Sci. 6:382. doi: 10.3389/fpls.2015.00382
Bois, B., Zito, S., and Calonnec, A. (2017). Climate vs grapevine pests and diseases worldwide: the first results of a global survey. Oeno One 51, 133–139. doi: 10.20870/oeno-one.2017.51.2.1780
Bruno Soares, M. (2017). Assessing the usability and potential value of seasonal climate forecasts in land management decisions in the southwest UK: challenges and reflections. Adv. Sci. Res. 14, 175–180. doi: 10.5194/asr-14-175-2017
Bruno Soares, M., Alexander, M., and Dessai, S. (2018a). Sectoral use of climate information in Europe: a synoptic overview. Clim. Serv. 9, 5–20. doi: 10.1016/j.cliser.2017.06.001
Bruno Soares, M., Daly, M., and Dessai, S. (2018b). Assessing the value of seasonal climate forecasts for decision-making. WIREs Clim. Change 9:e523. doi: 10.1002/wcc.523
Carrão, H., Naumann, G., and Barbosa, P. (2016). Mapping global patterns of drought risk: an empirical framework based on sub-national estimates of hazard, exposure and vulnerability. Glob. Environ. Chang. 39, 108–124. doi: 10.1016/j.gloenvcha.2016.04.012
Ciscar, J. C., Iglesias, A., Feyenc, L., Szabóa, L., Van Regemortera, D., Amelunge, A., et al. (2011). Physical and economic consequences of climate change in Europe. Proc. Natl. Acad. Sci. U.S.A. 108, 2678–2683. doi: 10.1073/pnas.1011612108
Cook, B. I., and Wolkovich, E. M. (2016). Climate change decouples drought from early wine grape harvests in France. Nat. Clim. 6, 715–719. doi: 10.1038/nclimate2960
Coscarelli, R., Caloiero, T., Minervino, I., and Sorriso-Valvo, M. (2016). Sensitivity to desertification of a high productivity area in Southern Italy. J. Maps 12, 573–581. doi: 10.1080/17445647.2015.1054904
Cramer, W., Guiot, J., and Marini, K. (2019). Risks Associated To Climate And Environmental Changes in the Mediterranean Region. A Preliminary Assessment by the MedECC Network Science-Policy Interface – 2019. Available online at: https://www.medecc.org/wp-content/uploads/2018/12/MedECC-Booklet_EN_WEB.pdf (accessed March 19 2020).
Dubrovský, M., Hayes, M., Duce, P., Trnka, M., Svoboda, M., and Zara, P. (2014). Multi-GCM projections of future drought and climate variability indicators for the Mediterranean region. Reg. Environ. Change 14, 1907–1919. doi: 10.1007/s10113-013-0562-z
Dunn, M. R., Rounsevell, M. D. A., Boberg, F., Clarke, E., Christensen, J., and Madsen, M. S. (2019). The future potential for wine production in Scotland under high-end climate change. Reg. Environ. Change 19, 723–732. doi: 10.1007/s10113-017-1240-3
European Commission (2019a). Global Agriculture Project. Available online at: https://climate.copernicus.eu/global-agriculture-project (accessed June 7, 2019).
European Commission (2019b). AGRICLASS - Climate Advisory Services for Agriculture. Available online at: https://climate.copernicus.eu/climate-advisory-services-agriculture (accessed June 7, 2019).
European Environment Agency (2017). Climate Change Adaptation and Disaster Risk Reduction in Europe Report. Denmark: European Environment Agency.
Eurostat (2020). EU Trade in Olive Oil. Available online at : https://ec.europa.eu/eurostat/en/web/products-eurostat-news/-/DDN-20191108-1 (accessed April 12, 2020).
Falloon, P., Bruno Soares, M., Manzanas, R., San-Martin, D., Liggins, F., Taylor, A, et al. (2018). The land management tool: developing a climate service in Southwest, UK. Clim. Serv. 9, 86–100. doi: 10.1016/j.cliser.2017.08.002
Food Agriculture Organization (2019). Handbook on Climate Information for Farming Communities – What Farmers Need and What Is Available. Rome: Food and Agriculture Organization. Available online at: http://www.fao.org/3/ca4059en/CA4059EN.pdf (accessed June 7, 2019).
Ford, J. D., Berrang-Ford, L., and Paterson, J. (2011). A systematic review of observed climate change adaptation in developed nations. Clim. Change 106, 327–336. doi: 10.1007/s10584-011-0045-5
Fraga, H., de Cortazar Atauri, I. G., Malheiro, A. C., and Santos, J. A. (2016). Modelling climate change impacts on viticultural yield, phenology and stress conditions in Europe. Glob. Change Biol. 22, 3774–3378. doi: 10.1111/gcb.13382
Fraga, H., and Santos, J. A. (2017). Daily prediction of seasonal grapevine production in the Douro wine region based on favourable meteorological conditions. Aust. J. Grape Wine Res. 23, 296–304. doi: 10.1111/ajgw.12278
Giannakis, E., and Bruggeman, A. (2015). The highly variable economic performance of European agriculture. Land Use Policy 45, 26–35. doi: 10.1016/j.landusepol.2014.12.009
Giorgi, F. (2006). Climate change hot-spots. Geophys. Res. Lett. 33:L08707. doi: 10.1029/2006GL025734
Gonzalez-Zeas, D., Quiroga, S., Iglesias, A., and Garrote, L. (2014). Looking beyond the average agricultural impacts in defining adaptation needs in Europe. Reg. Environ. Change 14, 1983–1993. doi: 10.1007/s10113-012-0388-0
Grasso, M., and Feola, G. (2012). Mediterranean agriculture under climate change: adaptive capacity, adaptation, and ethics. Reg. Environ. Change 12, 607–618. doi: 10.1007/s10113-011-0274-1
Guiot, J., and Cramer, W. (2016). Climate change: the 2015 Paris agreement thresholds and Mediterranean basin ecosystems. Science 354, 465–468. doi: 10.1126/science.aah5015
Hansen, J. W. (2002). Realizing the potential benefits of climate prediction to agriculture: issues, approaches, challenges. Agric. Syst. 74, 309–330. doi: 10.1016/S0308-521X(02)00043-4
Heumesser, C., Fuss, S., Szolgayová, J., Strauss, F., and Schmid, E. (2012). Investment in irrigation systems under precipitation uncertainty. Water Resour. Manag. 26, 3113–3137. doi: 10.1007/s11269-012-0053-x
Horta SRL (2012). Final Report Summary - MODEM_IVM (A Web-Based System for Real-time Monitoring and Decision Making for Integrated Vineyard Management), MODEM-IVM Project. Available online at: https://cordis.europa.eu/project/rcn/97134/reporting/en (accessed December 5, 2019).
Iglesias, A., Garrote, L., Quiroga, S., and Moneo, M. (2012). A regional comparison of the effects of climate change on agricultural crops in Europe. Clim. Change 112, 29–46. doi: 10.1007/s10584-011-0338-8
Iglesias, A., Quiroga, S., and Schlickenrieder, J. (2010). Climate change and agricultural adaptation: assessing management uncertainty for four crop types in Spain. Clim. Res. 44, 83–94. doi: 10.3354/cr00921
Institut National de la Recherche Agronomique (2015). DROPS (Drought-Tolerant Yielding Plants) Final Report. DROPS project. Avaiable online at: https://cordis.europa.eu/project/rcn/95052/reporting/en (accessed December 5, 2019).
Institut National de la Recherche Agronomique (2016). Final Report Summary - INNOVINE (Combining Innovation in Vineyard Management and Genetic Diversity for a Sustainable European viticulture). INNOVINE project. Available online at: https://cordis.europa.eu/project/rcn/104501/reporting/es (accessed December 5, 2019).
Kahiluoto, H., Kaseva, J., Balek, J., Olesen, J. E., Ruiz-Ramos, M., Gobin, A., et al. (2019). Decline in climate resilience of European wheat. Proc. Natl. Acad. Sci. U.S.A. 116, 123–128. doi: 10.1073/pnas.1804387115
Københavns Universitet (2013) Final Report Summary - SWUP-MED (Sustainable Water Use Securing Food Production in Dry Areas of the Mediterranean Region). SWUP-MED project. Available online at: https://cordis.europa.eu/project/rcn/88475/reporting/en (accessed December 5, 2019).
Koch, B., and Oehl, F. (2018). Climate change favors grapevine production in temperate zones. J. Agric. Sci. 9, 247–263. doi: 10.4236/as.2018.93019
Lemos, M. C., Kirchhoff, C. J., and Ramprasad, V. (2012). Narrowing the climate information usability gap. Nat. Clim. Change, 2, 789–794. doi: 10.1038/nclimate1614
Li, T., Vedula, S. S., Hadar, N., Parkin, C., Lau, J., and Dickersin, K. (2015). Innovations in data collection, management, and archiving for systematic reviews. Ann. Inten. Med. 162, 287–294. doi: 10.7326/M14-1603
Lorencová, E., Frélichová, J., Nelsonc, E., and Vackár, D. (2013). Past and future impacts of land use and climate change on agricultural ecosystem services in the Czech Republic. Land Use Policy 33, 183–194. doi: 10.1016/j.landusepol.2012.12.012
Maki, A., Cohen, M. A., and Vandenbergh, M. P. (2018). “Using meta-analysis in the social sciences to improve environmental policy,” in Handbook of Sustainability and Social Science Research, eds W. Leal Filho, R. Marans, and J. Callewaert (Cham: Springer International Publishing AG), 27–43. doi: 10.1007/978-3-319-67122-2_2
Malheiro, A. C., Santos, J. A., Fraga, H., and Pinto, J. G. (2010). Climate change scenarios applied to viticultural zoning in Europe. Clim. Res. 43, 163–177. doi: 10.3354/cr00918
Mechler, R., Hochrainer, S., Aaheim, A., Salen, H., and Wreford, A. (2010). Modelling economic impacts and adaptation to extreme events: insights from European case studies. Mitig. Adapt. Strat. Glob. 15, 737–762. doi: 10.1007/s11027-010-9249-7
Mereu, S., Sušnik, J., Trabucco, A., Daccache, A., Vamvakeridou-Lyroudia, L., Renoldi, S., et al. (2016). Operational resilience of reservoirs to climate change, agricultural demand, and tourism: a case study from Sardinia. Sci. Total Environ. 543 (Part B), 1028–1038. doi: 10.1016/j.scitotenv.2015.04.066
Migliore, G., Zinnanti, C., Schimmenti, E., Borsellino, V., Schifani, G., Di Franco, C. P., et al. (2019). A Ricardian analysis of the impact of climate change on permanent crops in a Mediterranean region. New Medit. 18, 41–52. doi: 10.30682/nm1901d
Moriondo, M., Argenti, G., Ferrise, R., Dibari, C., Trombi, G., and Bindi, M. (2016). Heat stress and crop yields in the Mediterranean basin: impact on expected insurance payouts. Reg. Environ. Change 16, 1877–1890. doi: 10.1007/s10113-015-0837-7
Mozell, M. R., and Thach, L. (2014). The impact of climate change on the global wine industry: challenges & solutions. Wine Econ. Policy 3, 81–89. doi: 10.1016/j.wep.2014.08.001
Musolino, D. A., Massarutto, A., and de Carli, A. (2018). Does drought always cause economic losses in agriculture? An empirical investigation on the distributive effects of drought events in some areas of Southern Europe. Sci. Total Environ. 633, 1560–1570. doi: 10.1016/j.scitotenv.2018.02.308
New QSR International Pty Ltd. (2014). NVivo Qualitative Data Analysis Software. Tulsa, OK: QSR International Pty Ltd.
Peltonen-Sainio, P., Jauhiainen, L., Palosuo, T., Hakala, K., and Ruosteenoja, K. (2016). Rainfed crop production challenges under European high-latitude conditions. Reg. Environ. Change 16, 1521–1533. doi: 10.1007/s10113-015-0875-1
Ponti, L., Gutierrez, A. P., Boggia, A., and Neteler, M. (2018). Analysis of grape production in the face of climate change. Climate 6:20. doi: 10.3390/cli6020020
Ponti, L., Gutierrez, A. P., Ruti, P. M., and Dell'Aquila, A. (2014). Fine-scale ecological and economic assessment of climate change on olive in the Mediterranean Basin reveals winners and losers. Proc. Natl. Acad. Sci. U.S.A. 111, 5598–5603. doi: 10.1073/pnas.1314437111
Porras, I., Marcos, R., and Navarro, M. (2017). Visca Project. Deliverable 2.2: Report on Weather Forecast Extreme Events. Available online at: http://visca.eu/index.php/the-project-4/deliverables-and-scientific-articles/send/4-deliverables-and-scientific-articles/26-viscadeliverable-2-2-report-on-weather-forecastextreme-events (accessed May 1, 2020).
Proietti, P., and Regni, L. (2018). Climate Change Mitigation Through a Sustainable Supply Chain for the Olive Oil Sector. Handbook Published Within Olive4Climate Project. Available online at: https://olive4climate.eu/wp-content/uploads/Olive4Climate-Handbook-_ENG.pdf (accessed December 6, 2019).
Ranieri, R. (2015). Geography of the Durum Wheat Crop. Pastaria International 6. Open fields. Available online at: http://www.openfields.it/sito/wp-content/uploads/2016/01/PASTARIA2015_N06_en-artOF.pdf (accessed February 27, 2019).
Resco, P., Iglesias, A., Bardaji, I., and Sotes, V. (2016). Exploring adaptation choices for grapevine regions in Spain. Reg. Environ. Change 16, 979–993. doi: 10.1007/s10113-015-0811-4
Ronchail, J., Cohen, M., Alonso-Roldán, M., Garcin, H., Sultan, B., and Angles, S. (2014). Adaptability of mediterranean agricultural systems to climate change: the example of the Sierra Mágina Olive-Growing Region (Andalusia, Spain). Part II: the future. Weather Clim. Soc. 6, 451–467. doi: 10.1175/WCAS-D-12-00045.1
Roselló, M., Villegas, D., Álvaro, F., Soriano, J. M., Lopes, M. S., Nazco, R., et al. (2019). Unravelling the relationship between adaptation pattern and yield formation strategies in Mediterranean durum wheat landraces. Eur. J. Agron. 107, 43–52. doi: 10.1016/j.eja.2019.04.003
Rossi, C., Girona, J., Exteve, J., and Navarro, M. (2018). VISCA Project. Deliverable D3.1: End-User Requirements. Available online at: http://visca.eu/index.php/the-project-4/publications-and-scientific-articles/send/4-publications-and-scientific-articles/4-visca_deliverable-3-1-end-user-requirements (accessed June 7, 2019).
Rossi, V., Salinari, F., Poni, S., Caffi, T., and Bettati, T. (2014). Addressing the implementation problem in agricultural decision support systems: the example of vite.net. Comput. Electron. Agric. 100, 88–99. doi: 10.1016/j.compag.2013.10.011
Rothamstead Research Limited (2009) TRITIMED - Exploiting the Wheat Genome to Optimise Water Use in Mediterranean Ecosystems. TRITIMED project final report. Available online at: https://cordis.europa.eu/project/rcn/74213/reporting/en (accessed December 6, 2019).
Salazar-Parra, C., Aranjuelo, I., Pascual, I., Aguirreolea, J., Sánchez-Díaz, M., Irigoyen, et al. (2018). Is vegetative area, photosynthesis, or grape C uploading involved in the climate change-related grape sugar/anthocyanin decoupling in Tempranillo? Photosynth. Res. 138, 115–128. doi: 10.1007/s11120-018-0552-6
Schultz, H. R. (2016). Global climate change, sustainability, and some challenges for grape and wine production. J. Wine Econ. 11, 181–200. doi: 10.1017/jwe.2015.31
Schultz, H. R., and Jones, G. V. (2010). Climate induced historic and future changes in viticulture. J. Wine Res. 21, 137–145. doi: 10.1080/09571264.2010.530098
Sima, M., Popovici, E.-A., Bălteanu, D., Micu, D. M., Kucsicsa, G., Dragotă, C., et al. (2015). A farmer-based analysis of climate change adaptation options of agriculture in the Bărăgan Plain, Romania. Earth Persp. 2:5. doi: 10.1186/s40322-015-0031-6
Singh, S. (2017). How to Conduct and interpret systematic reviews and meta-analyses. Clin. Transl. Gastroenterol. 5:e93. doi: 10.1038/ctg.2017.20
Tombesi, A., Tombesi, S., d'Andria, R., Lavini, A., Saavedra, M., Jardak, T., et al. (2007). Production Techniques in Olive Growing. Artegraf.
Van Leeuwen, C., and Darriet, P. (2016). The impact of climate change on viticulture and wine quality. J. Wine Econ. 11, 150–167. doi: 10.1017/jwe.2015.21
Vaughan, C., and Dessai, S. (2014). Climate services for society: origins, institutional arrangements, and design elements for an evaluation framework. WIRES Clim. Change 5, 587–603. doi: 10.1002/wcc.290
Ventrella, D., Charfeddine, M., Moriondo, M., Rinaldi, M., and Bindi, M. (2012). Agronomic adaptation strategies under climate change for winter durum wheat and tomato in southern Italy: irrigation and nitrogen fertilization. Reg. Environ. Change 12, 407–419. doi: 10.1007/s10113-011-0256-3
Vincent, K., Daly, M., Scannell, C., and Leathes, B. (2018). What can climate services learn from theory and practice of co-production? Clim. Serv. 12, 48–58. doi: 10.1016/j.cliser.2018.11.001
Vršič, S., and Vodovnik, T. (2012). Reactions of grape varieties to climate changes in North East Slovenia. Plant Soil Environ. 58, 34–41. doi: 10.17221/352/2011-PSE
World Meteorological Organization (2012). Climate Exchange. World Meteorological Organization. Available online at: https://library.wmo.int/pmb_ged/tudor-rose/files/assets/basic-html/toc.html (accessed June 7, 2019).
Keywords: agricultural decisions, olive, durum wheat, grape, Europe, climate impacts
Citation: Mihailescu E and Bruno Soares M (2020) The Influence of Climate on Agricultural Decisions for Three European Crops: A Systematic Review. Front. Sustain. Food Syst. 4:64. doi: 10.3389/fsufs.2020.00064
Received: 09 December 2019; Accepted: 17 April 2020;
Published: 15 May 2020.
Edited by:
Alberto Sanz Cobeña, Centro de Estudios e Investigación para la Gestión de Riesgos Agrarios y Medioambientales (CEIGRAM), SpainReviewed by:
Benjamin Sanchez Gimeno, Instituto Nacional de Investigación y Tecnología Agraria y Alimentaria (INIA), SpainLibère Nkurunziza, Swedish University of Agricultural Sciences, Sweden
Copyright © 2020 Mihailescu and Bruno Soares. This is an open-access article distributed under the terms of the Creative Commons Attribution License (CC BY). The use, distribution or reproduction in other forums is permitted, provided the original author(s) and the copyright owner(s) are credited and that the original publication in this journal is cited, in accordance with accepted academic practice. No use, distribution or reproduction is permitted which does not comply with these terms.
*Correspondence: Elena Mihailescu, elenacopenh@gmail.com