- 1Plant Stress Biology Laboratory, Institute of Environment and Sustainable Development (IESD), Banaras Hindu University (BHU), Varanasi, India
- 2Department of Earth Sciences, Indian Institute of Science Education and Research (IISER), Kolkata, India
Arsenic (As) is a toxic metalloid classified as group 1 carcinogen. The presence of As in high concentrations in paddy soil and irrigation water results into high As accumulation in rice grains posing a threat to the health of millions of people worldwide. The main reason for As contamination is the biogeochemical weathering of rocks and the release of bound As into groundwater. Human interventions through intensive agricultural practices and excessive groundwater consumption have contributed greatly to the prevailing As contamination. The flooded cultivation practice of rice favors the accumulation of As in rice grains. The formation of iron (Fe) plaque on paddy root surfaces, changes in the level of Fe and manganese (Mn) hydro(oxides), soil organic matter, soil pH, soil redox potential, and microbial activities under flooding conditions influence concentrations of various As species in the water–soil–paddy agroecosystem and favor the predominance of highly mobile arsenite [As(III)]. Once inside the rice plant, the concentration of As is regulated by arsenate reduction, arsenite efflux, root-to-shoot translocation, and vacuolar sequestration of As. The detailed understanding gained about the factors affecting As dynamics in soil and transport in rice plants may be helpful in developing feasible methods for sustainable cultivation of rice plants with low grain As. There is also need to ensure high production yields as well as grain quality to achieve the goals of sustainable development. This article discusses the aspects of As in the water–soil–paddy agroecosystem and presents suitable strategies to reduce the As load in rice grains.
Introduction
Arsenic (As) is an important geogenic contaminant found ubiquitously in the earth. Arsenic contamination of food and water is considered a global menace, threatening the health of ~150 million people worldwide (Majumder and Banik, 2019; Shikawa et al., 2019). The major sources of As exposure to humans include water and food items, especially rice. Arsenic exposure is associated with various chronic and acute health problems to humans that include skin lesions, cardiovascular diseases, diabetes, cancer, and so on (Shikawa et al., 2019). The As bound to Fe hydroxides, oxyhydroxides, and oxides has released in the recent past into groundwater through biogeochemical processes (Majumdar and Bose, 2017) and has resulted in widespread As contamination in India and Bangladesh (Raessler, 2018). In the Indian context, the region of As contamination, the Gangetic basin, is also renowned for extensive rice cultivation. The most common rice cultivation practice is flooded irrigation, and in absence of rains, groundwater is used. The repeated use of As-laden groundwater has resulted in As build-up in soil through the years (Upadhyay et al., 2019a). Rice can accumulate As in several-fold higher levels than other cereal crops such as wheat and maize (Williams et al., 2007; Shikawa et al., 2019; Upadhyay et al., 2019a). In reducing conditions of flooded paddy fields, arsenite [As(III)] is present in higher concentrations than arsenate [As(V)] (Meharg and Jardine, 2003). Other As forms also exist, which include organic methylated forms such as methylarsenate [MAs(V)], methylarsenite [MAs(III)], dimethylarsenate [DMAs(V)], dimethylarsenite [DMAs(III)], trimethylarsine [TMAs(III)] trimethylarsineoxide [TMAs(V)O] (Upadhyay et al., 2019b), and thio-arsenates (Kerl et al., 2019). Various factors such as pH, redox potential, dissolved organic carbon, organic matter, and biotic factors play a significant role in determining the bioavailability of various As species in the soil system (Majumdar et al., 2018). Rice roots release oxygen, which causes oxidation of Fe2+ to Fe3+and leads to the formation of iron plaque at the rice root surface. The adsorption of As by iron plaque increases the rhizospheric concentration of As around rice roots (Zhao et al., 2010; Hu et al., 2019). Further, As itself affects iron plaque formation, and there are also varietal influences on iron plaque formation due to differences in root oxidation abilities of different varieties (Lee et al., 2013).
The transporters involved in the uptake of As and translocation from root to shoot and grains play a crucial role in As build-up in plants. The extensive research conducted to date has resulted in identification of several transporters (Awasthi et al., 2017), such as aquaglyceroporins for As(III) and organic As species (Ma et al., 2008; Mosa et al., 2012; Lindsay and Maathuis, 2017), phosphate transporters for As(V) (Catarecha et al., 2007; Wang et al., 2016), ATP-binding cassette-type transporters for As-thiol complexes (Song et al., 2010, 2014), and inositol transporters and peptide transporter for grain/seed As accumulation (Duan et al., 2015). Furthermore, metabolites and genes/proteins involved in internal As metabolism in plants have also been discovered. To this end, the role of enzymes involved in As(V) to As(III) reduction is important, and these include high As content 1;1/1;2/4 (HAC1;1/1;2/4) (Shi et al., 2016; Xu et al., 2017) in rice. Significant progress has been made in understanding holistic biochemical, proteomic, and transcriptomic changes in response to As stress in plants (Chakrabarty et al., 2009; Srivastava et al., 2015). However, the development of low-As accumulating rice varieties through the use of genes/proteins by employing molecular techniques is not yet feasible. The As accumulation in rice grains varies significantly in different rice varieties (Norton et al., 2009). In West Bengal, India, the As concentration in five rice varieties (Satabdi, Gosai, Banskathi, Kunti, Ranjit) was recorded to range between 0.29 and 0.95 mg kg−1 (Upadhyay et al., 2019a). However, the identification of a suitable low As accumulating rice variety and its use in breeding programs for the development of a suitable rice variety is also time consuming (Dave et al., 2013). To tackle the problem, the use of sustainable, feasible, and easily applicable agronomic management practices can be an effective strategy.
Agronomic Strategies for as Reduction in Rice
Various agronomic practices have been examined for reducing grain As concentration in rice plants. These include water management [alternate wetting–drying (AWD), aerobic irrigation, sprinkler irrigation], nutrient management (phosphate, nitrogen, iron, selenium, silica, etc.), biological approaches (microbial, algal, and fungal inoculation), soil inversion, biochar, and nanoparticle amendments, and so on (Li et al., 2009, 2019; Norton et al., 2009; Moreno-Jiménez et al., 2014; Chauhan et al., 2017; Yu et al., 2017; Awasthi et al., 2018; Huhmann et al., 2019; Seyfferth et al., 2019; Srivastava et al., 2019) (Table 1). Recently, Upadhyay et al. (2018) discussed about the feasible utilization of plant growth-promoting microorganisms for the amelioration of As toxicity in plants. A recently utilized practical approach of Huhmann et al. (2019) was to excavate soil in three layers (top 20 cm and two layers of 10 cm each) and to simply invert the soil with the lowermost layer placed on top and uppermost layer at bottom. This led to a reduction in soil As around the rice root zone and also resulted in a significant increase in rice yields. However, the sustainability of the approach was questioned by the authors themselves. The benefits of inversion would not last a few decades until the upper layers again become laden with As brought from As-containing groundwater (Huhmann et al., 2019). Similarly, there are pros and cons of other management approaches also and in each of these approaches; the water supply itself plays a role in regulating eventual As levels in rice grains. Because water being used for irrigation is laden with As in contaminated areas, it appears worthwhile to develop an agronomic strategy based on water management either solely or in combination with other appropriate strategies. The following discussion is focused on water management–based approaches targeted at As reduction in rice grains.
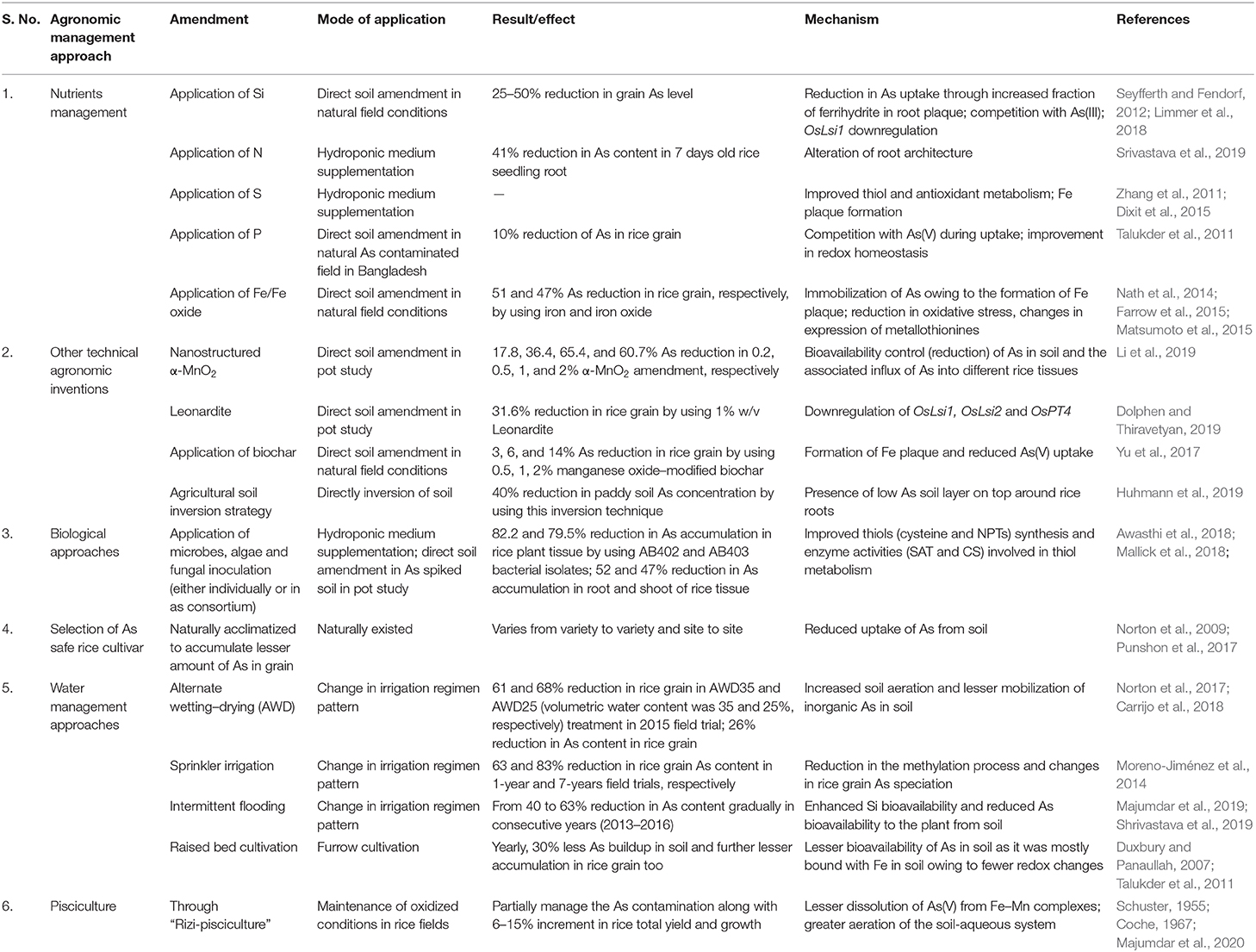
Table 1. Different agronomic management approaches studied through which As accumulation in rice grains can be reduced.
Rice–Water Interactions: Radial Oxygen Loss and Iron Plaque Formation
Groundwater used for irrigation is not only the source of As for rice fields but also an important regulator of As chemistry and bioavailability in rice fields. In the conventional method of rice irrigation, the fields are flooded throughout the growth period of rice plants (Shrivastava et al., 2019). Rice is a semiaquatic plant, and sufficient water availability plays a key role in achieving proper rice growth and productivity (Islam et al., 2019). The As cycle is a complex phenomenon and is influenced by various factors (Figure 1). Owing to continued water stagnancy in rice fields, anaerobic conditions are generated, and As release from the dissolution of Fe oxyhydroxides is promoted (Majumdar and Bose, 2018). As(III) tends to become attached to Fe oxides more frequently than As(V) and its concomitant release by microbial reduction, alteration in pH and redox coupling, and changes in organic matter results in increased bioavailability of more soluble As(III) (Majumdar et al., 2019). Water management must be practiced in such a way so as to reduce As loading in rice grains without affecting rice yields. Rice plants release oxygen through their roots, and this leads to iron plaque formation on the root surface (Mei et al., 2012; Majumdar et al., 2020). Iron plaque can act as a major sink or source of As to rice plants (Tripathi et al., 2014). Hence, water management of rice fields can also modify As bioavailability to rice plants through changes in oxygen release from rice plants and subsequent iron plaque formation on rice roots.
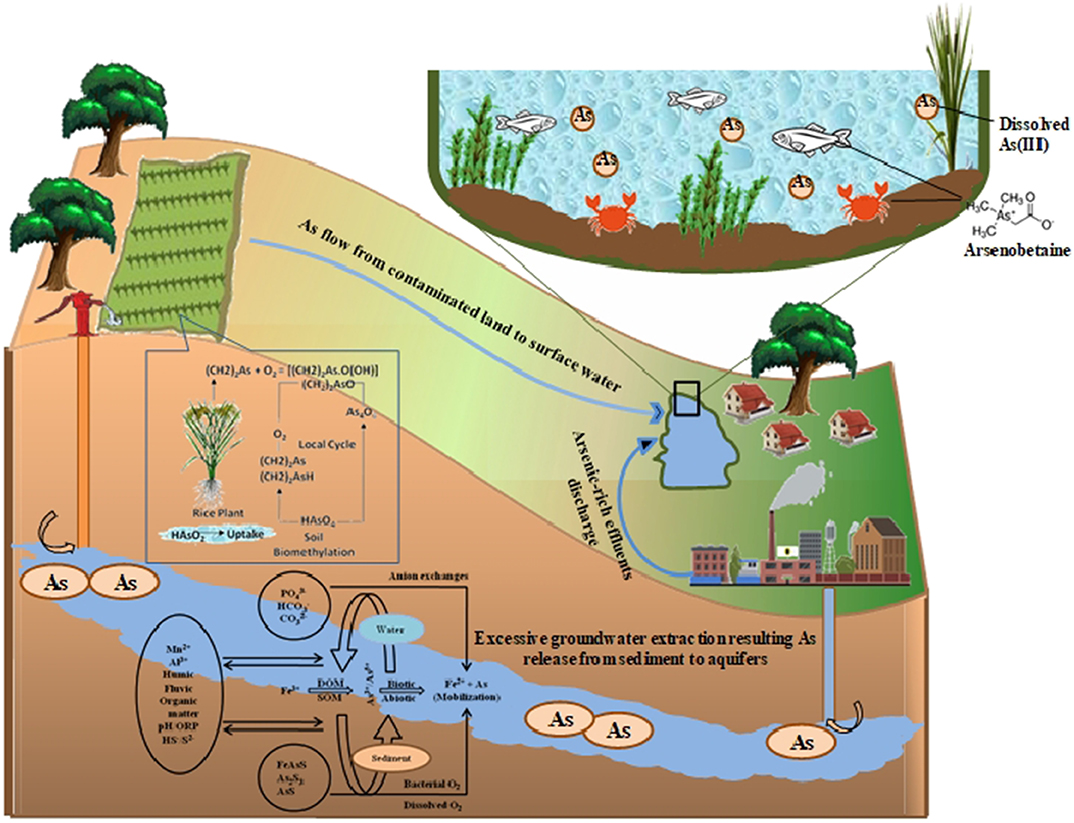
Figure 1. The figure depicts the arsenic (As) cycle in various arenas such as groundwater to agricultural rice crops. The extraction and application cycle of arsenic has been depicted here showing the potential impacts of excessive groundwater extraction enriched in dissolved As from Fe-oxyhydroxides. The practice results in gradual deposition of As on agricultural top soil and contamination to crops cultivated on it. Industrial effluent discharge contaminated with As to the water bodies together with agricultural surface runoff leads to the water pollution and accumulation of different forms of As in aquatic animals.
Reduced Flooding/Semiaerobic and Aerobic Cultivation
The practice of intermittent irrigation/semiaerobic irrigation has been found to result in optimum rice yields (Norton et al., 2017). This involves the periodical application of water to moisten the rice field followed by a drying phase. The whole Southeast Asia is an agricultural rich belt including the Indian subcontinent, which depends on intensive agriculture and consumes large amounts of groundwater for irrigation purpose in rice paddy fields. The reduced flooding cultivation such as sprinkler irrigation or AWD not only would indeed save groundwater but would also increase the organic content in the soil, which is known to be a key driver to agricultural production. The cycle of dry–wet phases also allows lesser translocation of As from soil to rice plants (Carrijo et al., 2018). During the growth cycle of rice plants, inflorescence- and grain-filling stages are crucial because most of the As is translocated to the grains during these stages (Majumdar and Bose, 2018). Semiaerobic cultivation generates less reducing conditions during the dry phase, leading to decreases in As bioavailability to the plants (Moreno-Jiménez et al., 2014; Majumdar et al., 2020). The continuous application of the dry phase for longer time periods (aerobic cultivation) reduces the soil As bioavailability to a greater extent and also causes less As accumulation in rice grains. However, it decreases total yield and productivity. Aerobic cultivation has also been found to increase cadmium (Cd) content in rice grain (Yuan et al., 2019).
Other Methods of Water Management
The other alternative methods of water application in rice cultivation include sprinkler irrigation (Table 1). Sprinkler irrigation can be used in fields to spread water from the top, keeping both the plants and soil moist without flooding the soil and hence reducing As loading to the rice grains (Majumdar et al., 2020). Moreno-Jiménez et al. (2014) tested the efficiency of sprinkler irrigation in reducing rice grain As. They found that sprinkler irrigation was able to reduce grain total As by one-third in only one application as compared to traditional irrigation. With continuous use of sprinkler irrigation, a significantly high reduction in grain total As was noticed in comparison to traditional irrigation. Further, the level of organic As was also lower in sprinkler irrigation. However, they did notice an increase in Cd concentration in rice grains as a consequence of sprinkler irrigation (Moreno-Jiménez et al., 2014). The reduction in mobility of soil As and reduction in the bioavailability of As to plants were noticed with the use of sprinkler irrigation by Spanu et al. (2012).
Another alternative is to simply avoid irrigation with groundwater and rely only on rain-fed rice cultivation. This is a simple and effective method. However, this would preclude summer season rice cultivation. Nevertheless, if proper rainwater storage could be achieved, and furrows were constructed for irrigation, rainwater-mediated irrigation could be practiced even during the summer season. In rainwater, presence of As is minimal. The application of rainwater in a furrow-like channel not only would provide necessary water to rice plants but also would avoid mobilization of soil As in high amounts as compared with conventional waterlogged cultivation (Sharma et al., 2014). During rain-fed irrigation in alkaline soils of paddy fields, the oxidative status of As and soil pH change and consequently the mobilization pattern of As are changed (Sultan and Dowling, 2006), leading to decreases in As accumulation in the top soil layer during the Aman season (monsoonal period) compared to Boro season (dry winter season) (Dittmar et al., 2010).
The stagnant water in the rice field resulting from flooded irrigation practices can also be used for the coculture of fishes. This is known as rizi-pisciculture. This method can also modulate the As concentration and its bioavailability in rice fields (Majumdar et al., 2020). The application of pisciculture has been established in China (Renkui et al., 1996), Thailand (Little et al., 1996), Vietnam (Berg, 2002), Bangladesh (Haroon and Pittman, 1997), and India (Das, 2002). Fish can also absorb As from the aqueous phase through their gills and convert it to less toxic organic forms such as arsenobetaine (Eisler, 1988; Majumdar et al., 2020). Therefore, the use of fish farming could be a sustainable solution to tackle As contamination in rice plants up to a certain extent.
In conclusion, As contamination of rice grains can be mitigated through effective water management strategies. This area needs more research and standardization to provide a sustainable option to reduce rice grain As for years to come. There is also a need to develop policies in this respect to restrict the use of highly As-contaminated bore wells and to lessen the extraction of excessive amounts of groundwater (Sekar and Randhir, 2009). This also entails that community-level participation is necessary to manage the As problem sustainably. The study conducted by Upadhyay et al. (2019b) in two remote villages of West Bengal demonstrated the yet prevalent lack of awareness among people and suggested the need for providing basic knowledge to tackle the As problem. Additionally, attention and coordination between stakeholders and government bodies are crucial to tackle the problem (Bhatia et al., 2014). There is also a need to integrate various potential agronomic practices in future research to achieve the desirable reduction in rice grain As.
Author Contributions
SS conceptualized the manuscript background and also revised and wrote the manuscript. MU and AM wrote the manuscript, prepared Table. JS partial write up and Figure preparation. Lastly, all the authors approves the manuscript.
Funding
This work was supported by a grant from Board of Research in Nuclear Sciences (BRNS), Department of Atomic Energy (DAE), Government of India (35/14/15/2018-BRNS/10393).
Conflict of Interest
The authors declare that the research was conducted in the absence of any commercial or financial relationships that could be construed as a potential conflict of interest.
Acknowledgments
MU thankfully acknowledges the SRF fellowship (09/013(0853)/2018 EMR-I) from CSIR-New Delhi, India. AM is thankful to MHRD-GOI for JRF fellowship.
References
Awasthi, S., Chauhan, R., Dwivedi, S., Srivastava, S., Srivastava, S., and Tripathi, R. D. (2018). A consortium of alga (Chlorella vulgaris) and bacterium (Pseudomonas putida) for amelioration of arsenic toxicity in rice: a promising and feasible approach. Environ. Exp. Bot. 150, 115–126. doi: 10.1016/j.envexpbot.2018.03.001
Awasthi, S., Chauhan, R., Srivastava, S., and Tripathi, R. D. (2017). The journey of arsenic from soil to grain in rice. Front. Plant Sci. 8:1007. doi: 10.3389/fpls.2017.01007
Berg, H. (2002). Rice monoculture and integrated rice-fish farming in the Mekong Delta, Vietnam-economic and ecological considerations. Ecol. Econ. 41, 95–107. doi: 10.1016/S0921-8009(02)00027-7
Bhatia, S., Balamurugan, G., and Baranwal, A. (2014). High arsenic contamination in drinking water hand-pumps in Khap Tola, West Champaran, Bihar, India. Front. Environ. Sci. 2:49. doi: 10.3389/fenvs.2014.00049
Carrijo, D. R., Akbar, N., Reis, A. F., Li, C., Gaudin, A. C., Parikh, S. J., et al. (2018). Impacts of variable soil drying in alternate wetting and drying rice systems on yields, grain arsenic concentration and soil moisture dynamics. Field Crop Res. 222, 101–110. doi: 10.1016/j.fcr.2018.02.026
Catarecha, P., Segura, M. D., Franco-Zorrilla, J. M., García-Ponce, B., Lanza, M., Solano, R., et al. (2007). A mutant of the Arabidopsis phosphate transporter PHT1;1 displays enhanced arsenic accumulation. Plant Cell 19, 1123–1133. doi: 10.1105/tpc.106.041871
Chakrabarty, D., Trivedi, P. K., Misra, P., Tiwari, M., Shri, M., Shukla, D., et al. (2009). Comparative transcriptomic analysis of arsenate and arsenite stresses in rice seedlings. Chemosphere 74, 688–702. doi: 10.1016/j.chemosphere.2008.09.082
Chauhan, R., Awasthi, S., Tripathi, P., Mishra, S., Dwivedi, S., Niranjan, A., et al. (2017). Selenite modulates the level of phenolics and nutrient element to alleviate the toxicity of arsenite in rice (Oryza sativa L.). Ecotoxicol. Environ. Saf. 38, 47–55. doi: 10.1016/j.ecoenv.2016.11.015
Coche, A. G. (1967). Fish culture in rice fields a world-wide synthesis. Hydrobiologia 30, 1–44. doi: 10.1007/BF00135009
Das, D. N. (2002). Fish farming in rice environments of North Eastern India. Aquac. Asia 7, 43–47. Available online at: http://library.enaca.org/AquacultureAsia/Articles/April-June-2002/FishFarmingInRiceEnvironments.pdf
Dave, R., Tripathi, R. D., Dwivedi, S., Tripathi, P., Dixit, G., Sharma, Y. K., et al. (2013). Arsenate and arsenite exposure modulate antioxidants and amino acids in contrasting arsenic accumulating rice (Oryza sativa L.) genotypes. J. Hazard. Mater. 262, 1123–1131. doi: 10.1016/j.jhazmat.2012.06.049
Dittmar, J., Voegelin, A., Roberts, L. C., Hug, S. J., Saha, G. C., and Ali, M. A. (2010). Arsenic accumulation in a paddy field in Bangladesh: seasonal dynamics and trends over a three-year monitoring period. Environ. Sci. Technol. 44, 2925–2931. doi: 10.1021/es903117r
Dixit, G., Singh, A. P., Kumar, A., Singh, P. K., Kumar, S., Dwivedi, S., et al. (2015). Sulfur mediated reduction of arsenic toxicity involves efficient thiol metabolism and the antioxidant defence system in rice. J. Hazard. Mater. 298, 241–251. doi: 10.1016/j.jhazmat.2015.06.008
Dolphen, R., and Thiravetyan, P. (2019). Reducing arsenic in rice grains by leonardite and arsenic–resistant endophytic bacteria. Chemosphere 223, 448–454. doi: 10.1016/j.chemosphere.2019.02.054
Duan, G. L., Hu, Y., Schneider, S., McDermott, J., Chen, J., Sauer, N., et al. (2015). Inositol transporters AtINT2 and AtINT4 regulate arsenic accumulation in Arabidopsis seeds. Nat. Plants 2:15202. doi: 10.1038/nplants.2015.202
Duxbury, J. M., and Panaullah, G. M. (2007). Remediation of arsenic for agriculture sustainability. Food Security Health Bangladesh (Rome: FAO, 1–28. Available online at: http://www.fao.org/land-water/home/en/ (accessed May 6, 2020).
Eisler, R. (1988). Arsenic Hazards to Fish, Wildlife, and Invertebrates: A Synoptic Review (No. 12). Fish and Wildlife Service. Laurel, MS: US Department of the Interior.
Farrow, E. M., Wang, J., Burken, J. G., Shi, H., Yan, W., Yang, J., et al. (2015). Reducing arsenic accumulation in rice grain through iron oxide amendment. Ecotoxicol. Environ. Saf. 118, 55–61. doi: 10.1016/j.ecoenv.2015.04.014
Haroon, A. K. Y., and Pittman, K. A. (1997). Rice-fish culture: feeding, growth and yield of two size classes of Puntius gonionotus Bleeker and Oreochromis spp. in Bangladesh. Aquaculture 154, 261–281. doi: 10.1016/S0044-8486(97)00061-6
Hu, M., Sun, W., Krumins, V., and Li, F. (2019). Arsenic contamination influences microbial community structure and putative arsenic metabolism gene abundance in iron plaque on paddy rice root. Sci. Total Environ. 649, 405–412. doi: 10.1016/j.scitotenv.2018.08.388
Huhmann, B., Harvey, C. F., Uddin, A., Choudhury, I., Ahmed, K. M., Duxbury, J. M., et al. (2019). Inversion of high-arsenic soil for improved rice yield in Bangladesh. Environmen. Sci. Technol. 53, 3410–3418. doi: 10.1021/acs.est.8b06064
Islam, S., Rahman, M. M., and Naidu, R. (2019). Impact of water and fertilizer management on arsenic bioaccumulation and speciation in rice plants grown under greenhouse conditions. Chemosphere 214, 606–613. doi: 10.1016/j.chemosphere.2018.09.158
Kerl, C. F., Rafferty, C., Clemens, S., and Planer-Friedrich, B. (2019). Monothioarsenate uptake, transformation, and translocation in rice plants. Environ. Sci. Technol. 52, 9154–9161. doi: 10.1021/acs.est.8b02202
Lee, C. H., Hsieh, Y. C., Lin, T. H., and Lee, D. Y. (2013). Iron plaque formation and its effect on arsenic uptake by different genotypes of paddy rice. Plant Soil 363, 231–241. doi: 10.1007/s11104-012-1308-2
Li, B., Zhou, S., Wei, D., Long, J., Peng, L., Tie, B., et al. (2019). Mitigating arsenic accumulation in rice (Oryza sativa L.) from typical arsenic contaminated paddy soil of southern China using nanostructured α-MnO2: pot experiment and field application. Sci. Total Environ. 650, 546–556. doi: 10.1016/j.scitotenv.2018.08.436
Li, R. Y., Stroud, J. L., Ma, J. F., McGrath, S. P., and Zhao, F. J. (2009). Mitigation of arsenic accumulation in rice with water management and silicon fertilization. Environ. Sci. Technol. 43, 3778–3783. doi: 10.1021/es803643v
Limmer, M. A., Mann, J., Amaral, D. C., Vargas, R., and Seyfferth, A. L. (2018). Silicon-rich amendments in rice paddies: effects on arsenic uptake and biogeochemistry. Sci. Total Environ. 624, 1360–1368. doi: 10.1016/j.scitotenv.2017.12.207
Lindsay, E. R., and Maathuis, F. J. M. (2017). New molecular mechanisms to reduce arsenic in crops. Trends Plant Sci. 22, 1016–1026. doi: 10.1016/j.tplants.2017.09.015
Little, D. C., Surintaraseree, P., and Innes-Taylor, N. (1996). Fish culture in rainfed rice fields of northeast Thailand. Aquaculture 140, 295–321. doi: 10.1016/0044-8486(95)01208-7
Ma, J. F., Yamaji, N., Mitani, N., Xu, X. Y., Su, Y. H., McGrath, S. P., et al. (2008). Transporters of arsenite in rice and their role in arsenic accumulation in rice grain. Proc. Nat. Acad. Sci. U. S. A. 105, 9931–9935. doi: 10.1073/pnas.0802361105
Majumdar, A., Barla, A., Upadhyay, M. K., Ghosh, D., Chaudhuri, P., Srivastava, S., et al. (2018). Vermiremediation of metal(loid)s via Eichornia crassipes phytomass extraction: a sustainable technique for plant amelioration. J. Environ. Manage. 220, 118–125. doi: 10.1016/j.jenvman.2018.05.017
Majumdar, A., and Bose, S. (2017). “Toxicogenesis and metabolism of arsenic in rice and wheat plants with probable mitigation strategies,”in Arsenic: Risks of Exposure, Behavior in the Environment and Toxicology, ed R. Kneževi (Hauppauge, NY: Nova Science Publisher, 149–166.
Majumdar, A., and Bose, S. (2018). “A glimpse on uptake kinetics and molecular responses of arsenic tolerance in rice plants,” in Mechanisms of Arsenic Toxicity and Tolerance in Plants, eds M. Hasanuzzaman, K. Nahar, and M. Fujita (Singapore: Springer), 299–315. doi: 10.1007/978-981-13-1292-2_13
Majumdar, A., Kumar, J. S., and Sheena Bose, S. (2020). “Agricultural water management practices and environmental influences on arsenic dynamics in rice field,” in Arsenic in Drinking Water and Food, ed S. Srivastava (Singapore: Springer), 425–443. doi: 10.1007/978-981-13-8587-2_17
Majumdar, A., Upadhyay, M. K., Kumar, J. S., Barla, A., Srivastava, S., Jaiswal, M. K., et al. (2019). Ultra-structure alteration via enhanced silicon uptake in arsenic stressed rice cultivars under intermittent irrigation practices in Bengal delta basin. Ecotoxicol. Environ. Saf. 180, 770–779. doi: 10.1016/j.ecoenv.2019.05.028
Majumder, S., and Banik, P. (2019). Geographical variation of arsenic distribution in paddy soil, rice and rice-based products: a meta-analytic approach and implications to human health. J. Environ. Manage. 233, 184–199. doi: 10.1016/j.jenvman.2018.12.034
Mallick, I., Bhattacharyya, C., Mukherji, S., Dey, D., Sarkar, S. C., Mukhopadhyay, U. K., et al. (2018). Effective rhizoinoculation and biofilm formation by arsenic immobilizing halophilic plant growth promoting bacteria (PGPB) isolated from mangrove rhizosphere: a step towards arsenic rhizoremediation. Sci. Total Environ. 610, 1239–1250. doi: 10.1016/j.scitotenv.2017.07.234
Matsumoto, S., Kasuga, J., Taiki, N., Makino, T., and Arao, T. (2015). Inhibition of arsenic accumulation in Japanese rice by the application of iron and silicate materials. Catena 135, 328–335. doi: 10.1016/j.catena.2015.07.004
Meharg, A. A., and Jardine, L. (2003). Arsenite transport into paddy rice (Oryza sativa L.) roots. New phytol. 157, 39–44. doi: 10.1046/j.1469-8137.2003.00655.x
Mei, X. Q., Wong, M. H., Yang, Y., Dong, H. Y., Qiu, R. L., and Ye, Z. H. (2012). The effects of radial oxygen loss on arsenic tolerance and uptake in rice and on its rhizosphere. Environ. Pollut.165, 109–117. doi: 10.1016/j.envpol.2012.02.018
Moreno-Jiménez, E., Meharg, A. A., Smolders, E., Manzano, R., Becerra, D., Sánchez-Llerena, J., et al. (2014). Sprinkler irrigation of rice fields reduces grain arsenic but enhances cadmium. Sci. Total Environ. 485, 468–473. doi: 10.1016/j.scitotenv.2014.03.106
Mosa, K. A., Kumar, K., Chhikara, S., Mcdermott, J., Liu, Z., Musante, C, et al. (2012). Members of rice plasma membrane intrinsic proteins subfamily are involved in arsenite permeability and tolerance in plants. Transgenic Res. 21, 1265–1277. doi: 10.1007/s11248-012-9600-8
Nath, S., Panda, P., Mishra, S., Dey, M., Choudhury, S., Sahoo, L., et al. (2014). Arsenic stress in rice: redox consequences and regulation by iron. Plant Physiol. Biochem. 80, 203–210. doi: 10.1016/j.plaphy.2014.04.013
Norton, G. J., Islam, M. R., Deacon, C. M., Zhao, F. J., Stroud, J. L., McGrath, S. P., et al. (2009). Identification of low inorganic and total grain arsenic rice cultivars from Bangladesh. Environ. Sci. Technol. 43, 6070–6075. doi: 10.1021/es901121j
Norton, G. J., Shafaei, M., Travis, A. J., Deacon, C. M., Danku, J., Pond, D., et al. (2017). Impact of alternate wetting and drying on rice physiology, grain production, and grain quality. Field Crop. Res. 205, 1–13. doi: 10.1016/j.fcr.2017.01.016
Punshon, T., Jackson, B. P., Meharg, A. A., Warczack, T., Scheckel, K., and Guerinot, M. L. (2017). Understanding arsenic dynamics in agronomic systems to predict and prevent uptake by crop plants. Sci. Total Environ. 581, 209–220. doi: 10.1016/j.scitotenv.2016.12.111
Raessler, M. (2018). The arsenic contamination of drinking and groundwaters in Bangladesh: featuring biogeochemical aspects and implications on public health. Arch. Environ. Contam. Toxicol. 75, 1–7. doi: 10.1007/s00244-018-0511-4
Renkui, C., Dashu, N., and Jianguo, W. (1996). “Rice fish culture in China: the past, present, and future,” in Rice Fish Culture in China, ed K. T. Mackay (Ottawa: IDRC, 3–14.
Sekar, I., and Randhir, T. (2009). Arsenic contamination in water resources: mitigation and policy options. Water Policy 11, 67–78. doi: 10.2166/wp.2009.005
Seyfferth, A. L., Amaral, D., Limmer, M. A., and Guilherme, L. R. (2019). Combined impacts of Si-rich rice residues and flooding extent on grain As and Cd in rice. Environ. Int. 128, 301–309. doi: 10.1016/j.envint.2019.04.060
Seyfferth, A. L., and Fendorf, S. (2012). Silicate mineral impacts on the uptake and storage of arsenic and plant nutrients in rice (Oryza sativa L.). Environ. Sci. Technol. 46, 13176–13183. doi: 10.1021/es3025337
Sharma, A. K., Tjell, J. C., Sloth, J. J., and Holm, P. E. (2014). Review of arsenic contamination, exposure through water and food and low cost mitigation options for rural areas. Appl. Geochem. 41, 11–33. doi: 10.1016/j.apgeochem.2013.11.012
Shi, S., Wang, T., Chen, Z., Tang, Z., Wu, Z., Salt, D. E., et al. (2016). OsHAC1; 1 and OsHAC1; 2 function as arsenate reductases and regulate arsenic accumulation. Plant Physiol. 172, 1708–1719. doi: 10.1104/pp.16.01332
Shikawa, S., Arao, T., and Makino, T. (2019). “Agronomic strategies for reducing arsenic risk in rice,” in Arsenic Contamination in Asia, eds H. Yamauchi and G. Sun (Singapore: Springer), 181–198. doi: 10.1007/978-981-13-2565-6_11
Shrivastava, A., Barla, A., Majumdar, A., Singh, S., and Bose, S. (2019). Arsenic mitigation in rice grain loading via alternative irrigation by proposed water management practices. Chemosphere 238:124988. doi: 10.1016/j.chemosphere.2019.124988
Song, W. Y., Park, J., Mendoza-Cózatl, D. G., Suter-Grotemeyer, M., Shim, D., Hörtensteiner, S., et al. (2010). Arsenic tolerance in Arabidopsis is mediated by two ABCC-type phytochelatin transporters. Proc. Nat. Acad. Sci. U. S. A. 107, 21187–21192. doi: 10.1073/pnas.1013964107
Song, W. Y., Yamaki, T., Yamaji, N., Ko, D., Jung, K. H., Fujii-Kashino, M., et al. (2014). A rice ABC transporter, OsABCC1, reduces arsenic accumulation in the grain. Proc. Nat. Acad. Sci. U. S. A. 111,15699–15704. doi: 10.1073/pnas.1414968111
Spanu, A., Daga, L., Orlandoni, A. M., and Sanna, G. (2012). The role of irrigation techniques in arsenic bioaccumulation in rice (Oryza sativa L.). Environ. Sci. Technol. 46, 8333–8340. doi: 10.1021/es300636d
Srivastava, S., Pathare, V. S., Sounderajan, S., and Suprasanna, P. (2019). Nitrogen supply influences arsenic accumulation and stress responses of rice (Oryza sativa L.) seedlings. J. Hazard. Mater. 367, 599–606. doi: 10.1016/j.jhazmat.2018.12.121
Srivastava, S., Srivastava, A. K., Sablok, G., Deshpande, T., and Suprasanna, P. (2015). Transcriptomics profiling of Indian mustard (Brassica juncea) under arsenate stress identifies key candidate genes and regulatory pathways. Front. Plant Sci. 6:646. doi: 10.3389/fpls.2015.00646
Sultan, K., and Dowling, K. (2006). Seasonal changes in arsenic concentrations and hydrogeochemistry of Canadian Creek, Ballarat (Victoria, Australia). Water Air Soil Pollut. 169, 355–374. doi: 10.1007/s11270-006-2813-9
Talukder, A. S. M. H. M., Meisner, C. A., Sarkar, M. A. R., and Islam, M. S. (2011). Effect of water management, tillage options and phosphorus status on arsenic uptake in rice. Ecotoxicol. Environ. Saf. 74, 834–839. doi: 10.1016/j.ecoenv.2010.11.004
Tripathi, R. D., Tripathi, P., Dwivedi, S., Kumar, A., Mishra, A., Chauhan, P. S., et al. (2014). Roles for root iron plaque in sequestration and uptake of heavy metals and metalloids in aquatic and wetland plants. Metallomics 6, 1789–1800. doi: 10.1039/C4MT00111G
Upadhyay, M. K., Majumdar, A., Barla, A., Bose, S., and Srivastava, S. (2019b). An assessment of arsenic hazard in groundwater–soil–rice system in two villages of Nadia district, West Bengal, India. Environ. Geochem. Health 41, 2381–2395. doi: 10.1007/s10653-019-00289-4
Upadhyay, M. K., Shukla, A., Yadav, P., and Srivastava, S. (2019a). A review of arsenic in crops, vegetables, animals and food products. Food Chem. 276, 608–618. doi: 10.1016/j.foodchem.2018.10.069
Upadhyay, M. K., Yadav, P., Shukla, A., and Srivastava, S. (2018). Utilizing the potential of microorganisms for managing arsenic contamination: a feasible and sustainable approach. Front. Environ. Sci. 6:24. doi: 10.3389/fenvs.2018.00024
Wang, P., Zhang, W., Mao, C., Xu, G., and Zhao, F. J. (2016). The role of OsPT8 in arsenate uptake and varietal difference in arsenate tolerance in rice. J. Exp. Bot. 67, 6051–6059. doi: 10.1093/jxb/erw362
Williams, P. N., Villada, A., Deacon, C., Raab, A., Figuerola, J., Green, A. J., et al. (2007). Greatly enhanced arsenic shoot assimilation in rice leads to elevated grain levels compared to wheat and barley. Environ. Sci. Technol. 41, 6854–6859. doi: 10.1021/es070627i
Xu, J., Shi, S., Wang, L., Tang, Z., Lv, T., Zhu, X., et al. (2017). OsHAC4 is critical for arsenate tolerance and regulates arsenic accumulation in rice. New Phytol. 215, 1090–1101. doi: 10.1111/nph.14572
Yu, Z., Qiu, W., Wang, F., Lei, M., Wang, D., and Song, Z. (2017). Effects of manganese oxide-modified biochar composites on arsenic speciation and accumulation in an indica rice (Oryza sativa L.) cultivar. Chemosphere 168, 341–349. doi: 10.1016/j.chemosphere.2016.10.069
Yuan, C., Li, F., Cao, W., Yang, Z., Hu, M., and Sun, W. (2019). Cadmium solubility in paddy soil amended with organic matter, sulfate, and iron oxide in alternative watering conditions. J. Hazard. Mater. 378:120672. doi: 10.1016/j.jhazmat.2019.05.065
Zhang, J., Zhao, Q. Z., Duan, G. L., and Huang, Y. C. (2011). Influence of sulphur on arsenic accumulation and metabolism in rice seedlings. Environ. Exp. Bot. 72, 34–40. doi: 10.1016/j.envexpbot.2010.05.007
Keywords: aerobic irrigation, arsenic, iron plaque, rice, sprinkler irrigation
Citation: Upadhyay MK, Majumdar A, Suresh Kumar J and Srivastava S (2020) Arsenic in Rice Agro-Ecosystem: Solutions for Safe and Sustainable Rice Production. Front. Sustain. Food Syst. 4:53. doi: 10.3389/fsufs.2020.00053
Received: 15 November 2019; Accepted: 08 April 2020;
Published: 21 May 2020.
Edited by:
Joshua B. Gurtler, Agricultural Research Service (USDA), United StatesCopyright © 2020 Upadhyay, Majumdar, Suresh Kumar and Srivastava. This is an open-access article distributed under the terms of the Creative Commons Attribution License (CC BY). The use, distribution or reproduction in other forums is permitted, provided the original author(s) and the copyright owner(s) are credited and that the original publication in this journal is cited, in accordance with accepted academic practice. No use, distribution or reproduction is permitted which does not comply with these terms.
*Correspondence: Sudhakar Srivastava, c3VkaGFrYXIuc3JpdmFzdGF2YUBnbWFpbC5jb20=; c3VkaGFrYXIuaWVzZEBiaHUuYWMuaW4=