- 1Facultad de Ciencias Químicas, Universidad Autónoma de Chihuahua, Chihuahua, Mexico
- 2Facultad de Ciencias Químicas, Benemérita Universidad Autónoma de Puebla, Ciudad Univ. Puebla, Puebla, Mexico
- 3Escuela de Ingeniería, Universidad De Las Américas Puebla, Cholula, Mexico
Hurdle technologies are among the novel food processing techniques for food preservation, where there is a combination of mild antimicrobial factors, which can interact on the inhibition of microbial spoilage. Spices essential oils are widely known as antimicrobial compounds that can have a preservation effect on food, but their effect can be affected by food components. In order to evaluate the effect of pH (4.5–7.0) and protein content (2–8%) on the antifungal effect of Mexican oregano essential oil (150–600 mg/L), a Box-Benkhen design was used to test the effect of the treatments on the radial growth of two food-related fungi, Penicillium expansum (NRRL 2304) and Aspergillus ochraceus (ATCC 22947). Three essential oils were used, these with a different chemical composition. The first chemotype of Lippia berlandieri Schauer, presented a higher proportion of carvacrol (58.23%). The second Lippia berlandieri Schauer essential oil presented a higher concentration of thymol (60.15%). Essential oil from Poliomintha longiflora Gray contained a 43.02% of thymol. Kinetic growth parameters were obtained using the modified Gompertz equation. The maximum mold growth (A) of the molds was affected in a lineal concentration-dependent effect by the three Mexican oregano essential oils and protein concentration. This phenomenon is independent of the chemistry variability in the composition of the three OEO. The higher concentration of protein (8%) was also related to an increase in lag time (λ). These two growth parameters were mainly statistically significant of all treatments evaluated, when considering as intrinsic parameters in food such as pH and protein content. The action of OEO was higher against A. ochraceus, positioning itself as the most sensitive microorganism. The use of predictive modeling can provide valuable information on the interaction of food components to enhance or decrease the antifungal capacity of essential oils.
Introduction
Food processing and preservation faces challenges such as nutrient conservation or the search for alternatives to chemical synthetic preservatives, which can preserve food quality, prevent microbial deterioration, and control enzymatic and chemical degradation. These new alternatives have focused on ensuring food safety but also providing a product with excellent sensory and nutritional quality (Hossain et al., 2016; Ngnitcho et al., 2017). On the other hand, traditional conservation products and sanitizers used in the food industry have been used for a long time, and have gradually acquired resistance (Davidson and Harrison, 2002). The combination of factors that affect microbial growth in foods can lead to less severe treatments that have a lesser effect on the nutrimental and sensory properties of food products. The combination of preservation factors is known as hurdle or barrier technologies, and have been successfully used in food processing to increase the product's shelf life (Khan et al., 2017; Ngnitcho et al., 2017).
Among the antimicrobial compounds suggested for use in hurdle technologies, spices are recognized for their biological properties, including antimicrobial, and antioxidant activities. These biological properties have been attributed to the complexity of their chemical or structural composition, and the functional groups present in their major active compounds (Rivera-Calo et al., 2015). Among species, essential oils used in food processing are thyme, basil, cinnamon, mint, tea tree, and eucalyptus, which are recognized for their functional groups and lipophilic properties (Hossain et al., 2016). The addition of these secondary metabolites has been evaluated in meat food, seafood, daily food, and fruits, vegetables, cereals whit promising results (Perricone et al., 2015; Possas et al., 2017).
One of the most studied essential oil is Oregano's that include carvacrol and thymol as bioactive components, as well as minor concentration of other terpenes and aromatic compounds, recognized as GRAS, while not exceeding 50 mg/kg (Marinelli et al., 2018; Navarro-Cruz et al., 2018). Extraction of Oregano essential oil (OEO) can be done using different parts of the plant, including leaf, stem, or root (Rivera-Calo et al., 2015). Worldwide, there are close to 40 different plant species known as oregano, with differences in the proportion of thymol and carvacrol in the essential oil; also, there are differences in the composition of a particular oregano species. In Mexico, Lippia berlandieri (also identified as Lippia graveolens) is the most common oregano spice found, but other species such as Poliomintha longiflora or Lippia palmeri have also been studied (Ortega-Nieblas et al., 2011; Cid-Pérez et al., 2016).
OEO has been reported with antifungal and antimicrobial effects under several conditions, both in the laboratory in vitro experiments and while added to food products (Witkowska et al., 2014; Hossain et al., 2016; Cid-Pérez et al., 2019). When added to food products, is necessary to consider that the antimicrobial effect of essential oils can be modified by intrinsic factors such as the presence of lipid, proteins, carbohydrates, salt content or the pH of the food matrix, regardless of the deteriorative of pathogenic microorganism that is intended to be controlled or eliminated (García-Díez et al., 2017). Also, the extrinsic factors such as storage temperature and relative humidity can affect the food safety of the stored products (Gutierrez et al., 2008).
The understanding of the microbial growth in food is essential to evaluate the efficacy of preservation methods and can provide a base for the preparation of high-quality products (McMeekin et al., 2002). The use of experimental designs and prediction models can provide a preliminary assessment of a food product processing or formulation, by giving a quantitative measure of the interaction of hurdle factors on the microbiota present in a food system (Gutierrez et al., 2009). Predictive microbiology studies the microbial responses to environmental factors, expressed in mathematical equations that can predict the behavior of microorganisms under new conditions (Akkermans et al., 2018; Ochoa-Velasco et al., 2018).
In order to evaluate the effect of food composition on the antifungal activity of Mexican oregano, this work was aimed to use predictive models to evaluate the combined effect of pH, percentage of protein, and concentration of Mexican OEO (Lippia berlandieri Schauer and Poliomintha longiflora Gray), on the growth of food-related fungi, Penicillium expansum and Aspergillus ochraceus.
Materials and Methods
Biological Material
Mexican oregano essential oils were provided by CIReNA (Center of Research for Natural Resources, Salaices, municipality of López Chihuahua-Mexico). Essential oils were obtained by steam distillation and were maintained in refrigerated conditions and protected from light. Three different essential oils were tested, two of L. berlandieri Schauer and one of P. longiflora. Fungal strains of P. expansum (NRRL 2304) and A. ochraceus (ATCC 22947) were provided by the laboratory of Microbiology of the UDLAP-Mexico.
Chemical Characterization of Mexican Oregano Essential Oils
Essential oils were characterized by Gas Chromatography coupled to Mass Spectrometry, using a GC Perkin Elmer Turbo Mass Gold MS-Auto system XLTM (Perkin- Elmer, Norwalk, CT) with a splitless injector and 70 eV electronic fragmentation detector, equipped with an AT-1 capillary column (30 m X 0.25 I.D. X 0.25 μm). The injection temperature was 220°C, with a flow rate of 1 mL/min, and helium was used as a carrier gas. Oven initial temperature was 120°C and was increased at a 10°C x min, with an isotherm of 180°C for 7 min. An aliquot of 200 μL was diluted in 1 mL of acetone, and 1 μL of this dilution was injected into the equipment. The identification of major components of the essential oils was based on a comparison of the mass spectra with the mass profile available at NIST (National Institute of Science & Technology) library of chemical compounds and samples (Cid-Pérez et al., 2019).
Kinetic Models From the Combined Effect of pH, % Protein, and Concentration of Essential Oil
The effect of pH, percentage of proteins, and concentration of OEO on fungal growth was determined by agar dilution method with modifications on the formulation. PDA agar (MCD LAB, Tlalnepantla, Mexico) was enriched with meat extract (BD BIOXON, Mexico City, Mexico); pH was adjusted with citric acid (0.1 N) or sodium hydroxide (0.1 N) (CTR Scientific, Monterrey, Mexico). Essential oils were previously diluted in alcohol (5% w/v) and sterilized by filtration through a 0.22 μm membrane (Millipore, Burlington, Mass, USA), to then adjust the concentrations to 150, 375, and 600 mg/L. The concentrations of the oregano EO tested were selected for sublethal concentrations, according to Cid-Pérez et al. (2016), that reports an MIC value of 1,200 mg/L. A Box-Behnken experimental design was used, with three factors and three levels of each factor; three replicates were done in the central point and one replicate in each axial point (Minitab 18 Statistical Software, 2017), as shown in Table 2.
For media inoculation, a spore suspension was prepared by pouring 10 mL of a sterile Tween 80 (0.1% in water) solution in a PDA slant tube with mold growth; the tube was agitated for spore detachment. In a PDA petri plate, 5 μL of the spore suspension was inoculated in the center of the plate and left to dry. Plates were incubated at room temperature (25°C) in separate containers for each concentration of essential oil. Radial growth (cm) was obtained as the average diameter of perpendicular measurements; data were recorded every 24 h during 21 days. Three replicates were used for each treatment. Data were adjusted to Ln (D/D0), where D is the radial growth at a given day and D0 was the initial diameter of the inoculation. Data was adjusted by non-linear regression to obtain the growth parameters, as proposed by the modified Gompertz equation (Avila-Sosa et al., 2010), using KaleidaGraph 4.5.4 (Synergy Software, Reading, PA).
Where:
A = maximum mold growth at the stationary phase (cm),
Vm = maximum specific growth rate (cm/day),
λ = lag phase (day),
t = time (day).
In order to validate the results, the coefficient of determination was obtained for each growth curve. The kinetic growth parameters were analyzed considering the Box- Behnken design; the dependent variables used in the analysis were maximum mold growth at the stationary phase (A), the maximum specific growth rate (Vm), and the lag phase (λ). To determine the statistically significant models, a full quadratic design was considered, as stated in Equation (2), and the stepwise function was used, to include in the models only those parameters that were significant (Avila-Sosa et al., 2010).
Where
x1 = protein content
x2 = pH
x3 = Mexican oregano EO concentration
Model selection was based on the ANOVA of the regression model (p < 0.01). Statistical analysis was done using Minitab 18 software (Minitab, Inc., State College, PA, USA).
Results
Characterization of Essential Oils
Mexican oregano (L. berlandieri Schauer) essential oil has a stronger flavor, due to the higher content of carvacrol in its composition. There are, however, chemotypes with differences in the proportion of thymol and carvacrol (Cid-Pérez et al., 2016). On the other hand, P. longiflora Gray essential oil is more related to the composition of Origanum vulgare essential oil (Cid-Pérez et al., 2019). In order to characterize the composition of the essential oils used, particularly to the proportion of carvacrol and thymol, GC-MS analysis was carried out. Figure 1 show the differences between the chemotypes of L. berlandieri Schauer as well as a more balanced proportion in the P. longiflora essential oil. The essential oil included in Figure 1A will be referred to as L. berlandieri OEO high in carvacrol, and the one in Figure 1B as L. berlandieri OEO high in thymol. The complete chemical composition of the three Mexican oregano essential oils is described in Table 1.
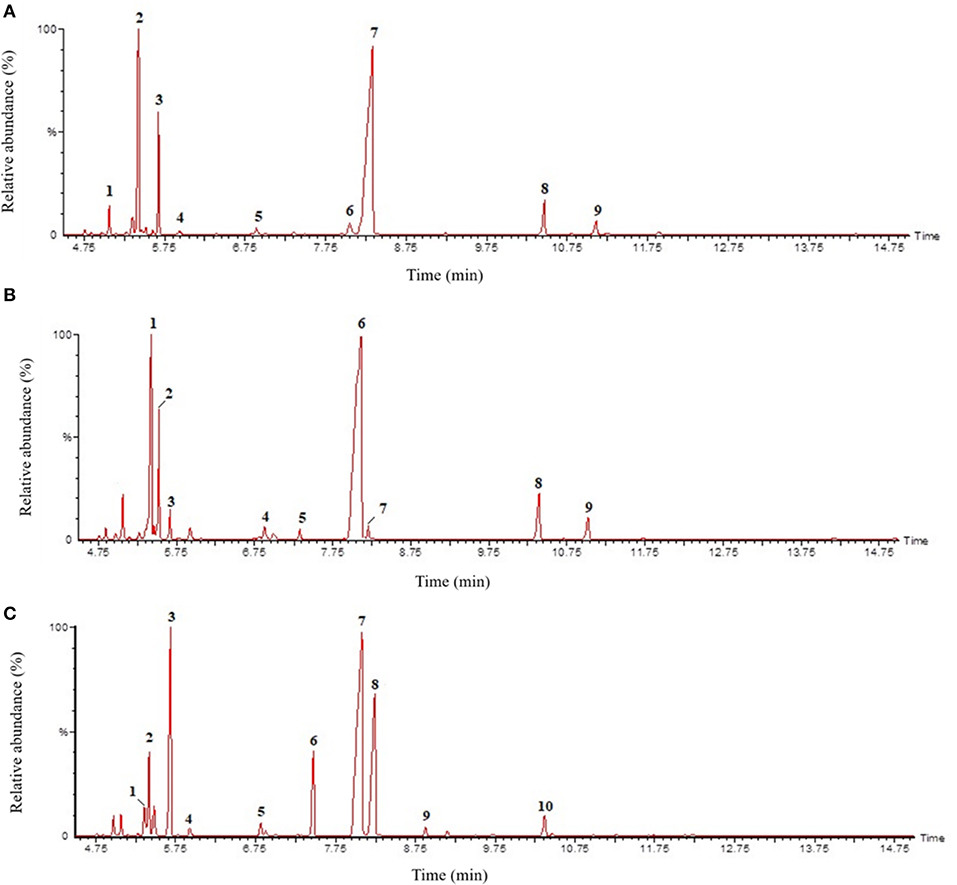
Figure 1. GC chromatogram of Mexican oregano essential oils. (A) Lippia berlandieri Schauer essential oil, showing a higher proportion of carvacrol. (B) Lippia berlandieri Schauer essential oil, chemotype with higher proportion of thymol. (C) Poliomintha longiflora Gray essential oil.
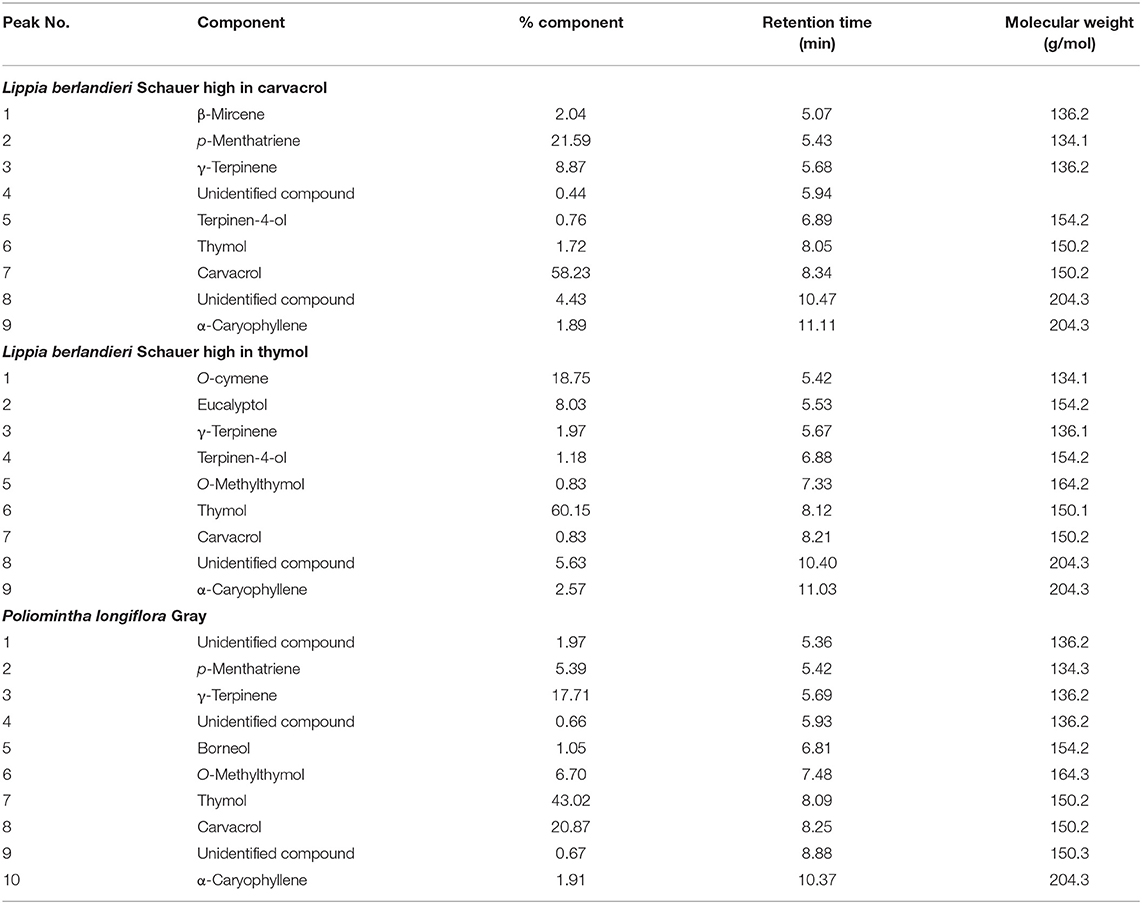
Table 1. Chemical components of Lippia berlandieri Schauer and Poliomintha longiflora Gray essential oils.
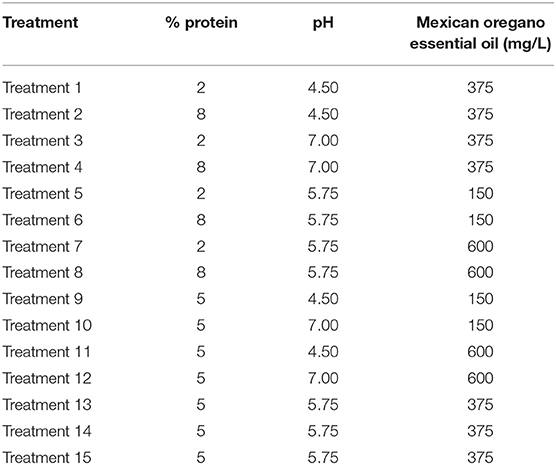
Table 2. Box-Behnken design for evaluation of pH, protein content, and Mexican oregano essential oil, on the growth of food-related fungi.
Kinetic Models From the Combined Effect of pH, Percentage of Protein and Concentration of Mexican Oregano Essential Oil
The data obtained from daily measurement of radial growth of both fungal strains in the different treatments was adjusted using the modified Gompertz equation, to obtain the kinetic parameters: A (maximum diameter of the fungal colony); Vmax, as the maximal growth velocity, given in cm/day, and the lag phase (λ). Data for both molds in all treatments are presented in Tables 3–5, for the different OEO tested. The intrinsic food factors included in the study were pH, protein content, and different concentrations of three different Mexican OEO, two chemotypes of L. berlandieri, and one sample from P. longiflora. Although the major difference among the essential oils is the concentration of thymol and carvacrol, there are many other components present in the essential oil.
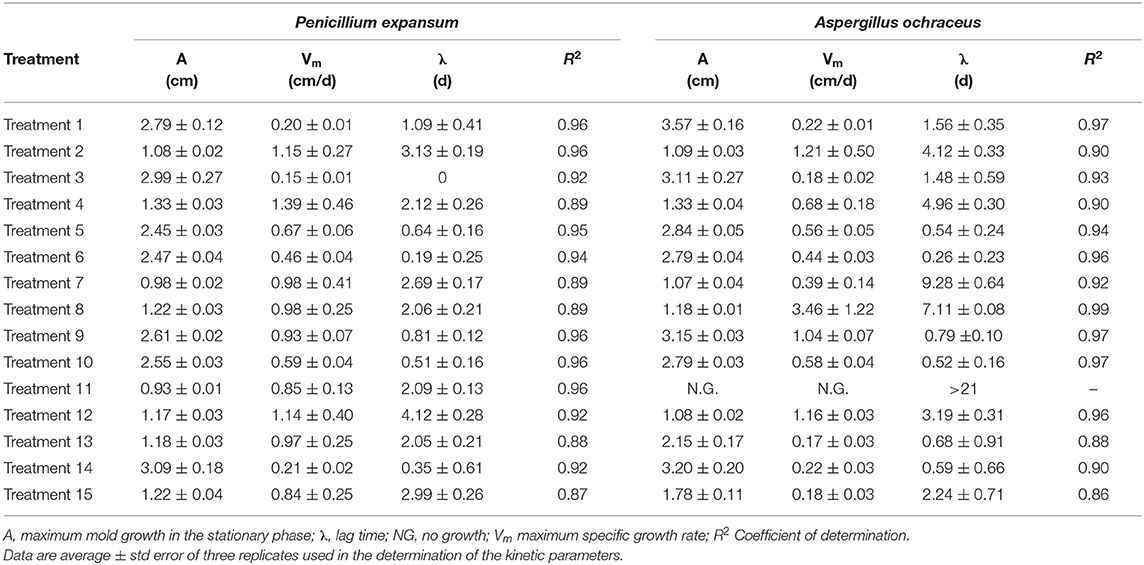
Table 3. Parameters of the modified Gompertz equation for radial growth of Penicillium expansum and Aspergillus ochraceus, using Lippia berlandieri Schauer essential oil with high carvacrol content in combination with pH and protein content.
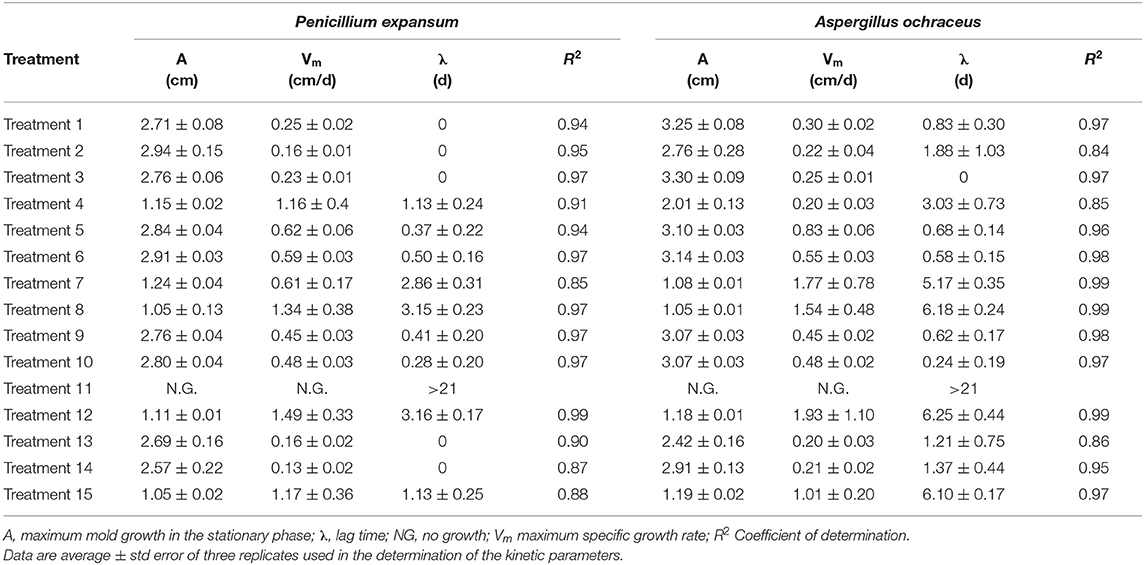
Table 4. Parameters of the modified Gompertz equation for radial growth of Penicillium expansum and Aspergillus ochraceus, using Lippia berlandieri Schauer essential oil with high thymol content in combination with pH and protein content.
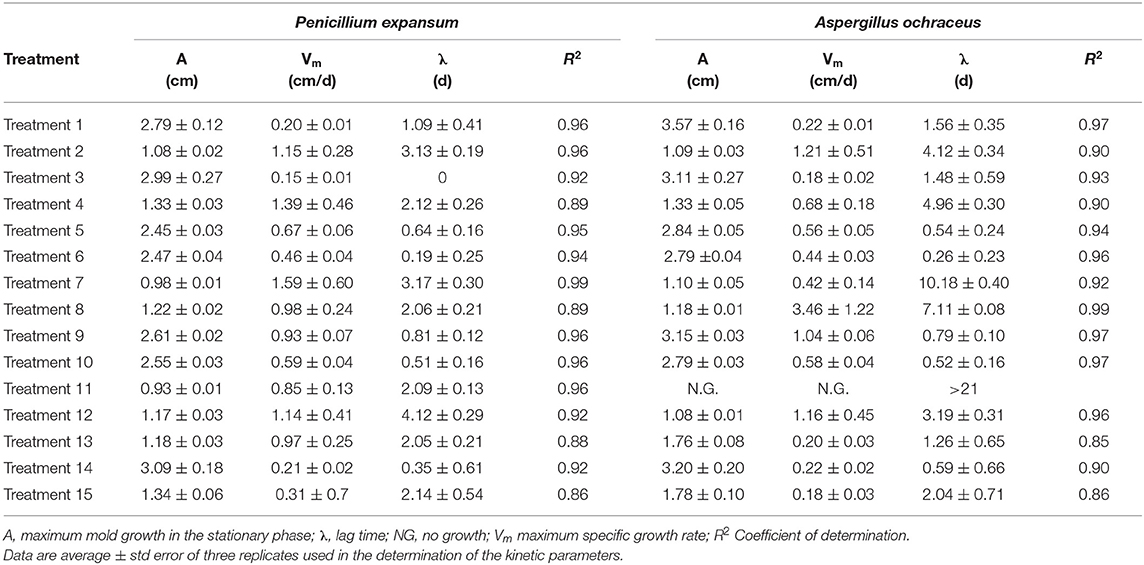
Table 5. Parameters of the modified Gompertz equation for radial growth of Penicillium expansum and Aspergillus ochraceus, using Poliomintha longiflora Gray essential oil in combination with pH and protein content.
One of the chemotypes of L. berlandieri with a high concentration of carvacrol (58.23%), in combination with pH and protein in the growth media, showed differences in the kinetic parameters for both molds (Table 3). An acidic pH and a high concentration of OEO inhibited the growth of A. ochraceus (treatment 11), and a high percentage of proteins leads to a lag phase of up to 4 days for the same mold (treatments 2 and 4). Regression analysis showed that the concentration of OEO had a significant effect on the maximum growth of the fungi, in a lineal concentration-dependent proportion (Equations 3 and 4). Neither the maximum growth rate nor the Lag time models were significant for both molds.
Regression model for P. expansum in the presence of Mexican OEO (L. berlandieri) with a high proportion of carvacrol
F = 7.54, p = 0.008, R2 = 55.68%
Regression model for A. ochraceus in the presence of Mexican OEO (L. berlandieri) with a high proportion of carvacrol
F = 11.94, p = 0.001, R2 = 66.56%
Regarding the OEO of L. berlandieri chemotype with a high concentration of thymol (60.15%), the effect on the lag phase in both molds was higher in many of the treatments, especially in those with a higher concentration of protein in the growth media (Table 4). Regression analysis showed that OEO was also significant for the explanation of the maximum growth rate in both P. expansum and A. ochraceus, but in the later fungi, the effect was not only linear but quadratic. Lag time was also affected in a quadratic effect by the concentration of OEO, and the maximum growth rate of A. ochraceus was affected by pH and OEO (Equations 5–8).
Regression model for P. expansum in the presence of Mexican OEO (L. berlandieri) with a high proportion of thymol
F = 18.40, p = 0.001, R2 = 58.59%
F = 7.38, p = 0.006, R2 = 66.81%
Regression model for A. ochraceus in the presence of Mexican OEO (L. berlandieri) with a high proportion of thymol
F = 16.10, p < 0.001, R2 = 72.86%
F = 6.51, p = 0.008, R2 = 78.35%
In the case of P. longiflora essential oil, high protein content was also related to larger lag times (treatments 2, 4); A. ochraceus was more susceptible than P. expansum (Table 5). Regression analysis demonstrated a lineal effect of OEO concentration on the lag time (λ) of P. expansum, as well as a lineal effect of protein and OEO on the maximum growth (A). In A. ochraceus, the lineal effect of protein in OEO concentration was also statistically significant. No effect was observed on the growth rate of both fungal strains (Equations 9–11). The fungicidal effect of OEO was not affected by the variability in chemical composition between essential oils. The natural product evaluated by Cid-Pérez in 2016, contained a lower amount of thymol (37.2%), compared to 43.0% in the essential oil used in this investigation. The biological activity of all compounds present in essential oils, as a mixture, is what determines their potential.
Regression model for P. expansum in the presence of Mexican OEO (P. longiflora)
F = 7.82, p = 0.007, R2 = 56.57%
F = 7.82, p = 0.007, R2 = 56.57%
Regression model for A. ochraceus in the presence of Mexican OEO (P. longiflora)
F = 11.78, p = 0.001, R2 = 66.25%
In general, when evaluating the effect of the combination of factors, high concentrations of the three essential oils (600 mg/L) limit the growth (A) of P. expansum and A. ochraceus. This behavior is represented in the response surface graphs of Mexican OEO (L. berlandieri) with a high proportion of carvacrol in Figure 2.
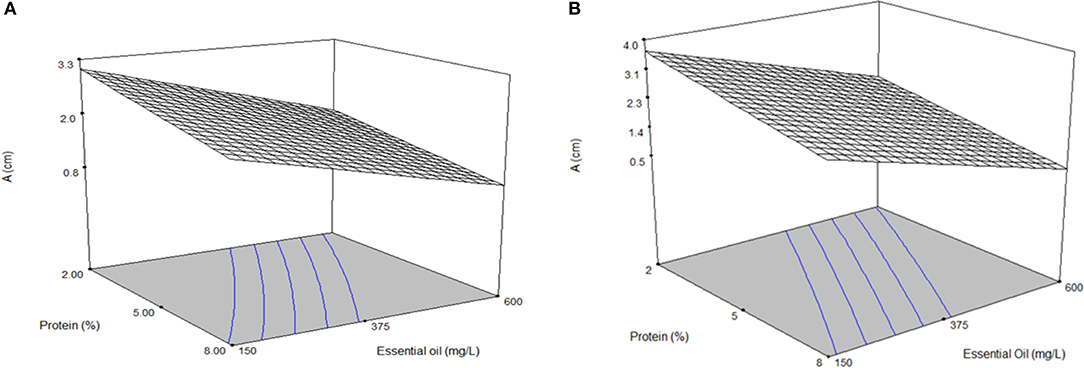
Figure 2. Response surface graphs of the kinetic parameter A, when evaluating protein content and essential oil high in carvacrol (Lippia berlandieri Schauer) against both fungal strains: (A) Penicillium expansum. (B) Aspergillus ochraceus.
Discussion
The contamination of molds in food represents a risk to the consumer since they can produce allergenic metabolites such as aflatoxins, ochratoxins A, fumonisins, trichothecenes, and zearalenones. Mycotoxins are the cause of autoimmune, teratogenic, cancerogenic, and mutagenic health problems (Hossain et al., 2016). OEO has demonstrated its fungicidal effect alone, as part of edible films or encapsulated to keep their biological properties, for food preservation, to extend the shelf life (Avila-Sosa et al., 2010; Cid-Pérez et al., 2016; Ribeiro-Santos et al., 2017). The general mechanism of action of essential oils, include morphological alterations in the cell membrane of pathogenic microorganisms (Tang et al., 2018). However, it is essential to understand the effect of food components or properties, such as pH, on the antimicrobial effect of the essential oil.
Predictive microbiology has been used to explain the antifungal mechanisms of natural antimicrobials. Weibull‘s distribution model has been used to study the viability of P. expansum spores places in contact with betel leaf essential oil. The authors noted that spore survival decreases while treatment times increased. Spore inactivation is due to the loss of cell membrane integrity, since the essential oil penetrates inside the cell, and interferes with the enzymatic activities of cell wall synthesis (Basak and Guha, 2017). The use of predictive models does not always provide information for the interaction of the variables studied. Possas et al. (2017) used the primary models of Baranyi and Weibull to evaluate the growth and survival of Salmonella on meat products, applying thyme essential oil with thymol as the main component (60%), and carvacrol in lower concentration (3.8%). Although the Weibull model has high flexibility to adapt to too many types of data, the results were not sufficient to model them.
Extrinsic food factors such as temperature and relative humidity, or intrinsic factors such as water activity, are among the most important factors that affect fungal growth in foods (Avila-Sosa Sanchez et al., 2015). However, the effect of other food properties or intrinsic factors are not extensively reported. The interaction of pH and protein content was tested on the fungicidal effect of Mexican oregano essential oils. We expected to have a synergistic effect, or that the pH and protein concentration were not negatively affecting the antifungal effect of the OEO. In most cases, the predictive models that were statistically significant, linearly included protein and OEO, with no effect of interaction among the two factors. The growth rate (Vm) was not affected by the different compositions of the media, except for A. ochraceous in the presence of OEO with high proportion of thymol, were a combination of linear and quadratic effects of the essential oil and pH, as well as the lineal interaction of both factors were included in the model (Equation 8). The complexity of the model does not allow for a maximum prediction response. P. expansum lag time was also explained by a regression model that included a quadratic effect of the essential oil and a lineal effect of pH (Equation 6). The non-linear effect of essential oil concentrations on fungal growth has been previously reported, where higher or lower concentrations of OEO promoted fungal growth (Portillo-Ruiz et al., 2005).
The effect of pH and food ingredients has also been monitored for the antilisterial effect of Origanum vulgare essential oil on the lag phase and maximum growth of Listeria monocytogenes. OEO was more effective at pH 5.0; lower pH values increased the lag phase and reduced maximum growth. An acidic pH increases the OEO hydrophobicity, also increasing the dissolution of the membrane lipids, with the consequent interference with cellular functionality (Albuquerque et al., 2006; Rivera-Calo et al., 2015; Navarro-Cruz et al., 2018). The effect of pH is also related to the type of acid used to adjust the media, since organic acids are also mild antimicrobials. Propionic acid is used as a food preservative due to its fungicidal ability. It has been reported that a concentration of 0.20%, propionic acid reduces the growth rate of Aspergillus spp, Geotrichum spp, Mucor spp, Penicillium spp, and Scopulariosis spp by up to 50% (Khan et al., 2017). The concentrations of pH used in this report were from mildly acidic to neutral, and two models, there was no interaction with the OEO fungicidal capacity. It can be considered that the citric acid that was used to adjust the pH values does not affect the fungicidal action of OEO.
The hydrophobic components of essential oils are absorbed in the protein matrix and the lipid fraction of a food product, leaving essential oils less available to act, as reported for Listeria monocytogenes, where the presence of these components promoted the bacterial growth (Gutierrez et al., 2008). On the other hand, food nutrients can be helpful for bacterial cell recovery. When different essential oils (oregano and thyme) were combined, an additive antimicrobial effect on the bacteria was observed. When NaCl (5 g/100 mL) was added to the media added with starch, water activity decreased, and microbial counts were also reduced (Perricone et al., 2015; Cattelan et al., 2018). When OEO (carvacrol 65.1%) and NaCl were evaluated separately on the growth of Escherichia coli, the effect observed suggested the use of the essential oil with salt reduction in the food products, considering the health problem caused by a high salt intake (Witkowska et al., 2014). This synergistic phenomenon has also been reported for oregano oil (Origanum vulgare) and thyme (Thymus vulgaris), when evaluated against Aspergillus flavus, Aspergillus parasiticus, and Penicillium chysogenus (Hossain et al., 2016). The authors found the lowest values of minimum fungicidal concentration in this system when compared with other oils derived from basil, cinnamon, eucalyptus, tangerine, mint, and tea tree. The combination of essential oils halted colonial growth and its maximum growth rate. The lag phase was extended by the antifungal effect, as obtained by the modified Gompertz model (Witkowska et al., 2014). Another alternative to potentiate the effect of essential oils, is the combination with peptides or proteins produced by specific lactic bacteria, including bacteriocins such as nisin V at 500–1,000 UI/g (derived from Lactococcus lactis), pediocin (derived from Pediococcus acidilactici), or enterocine A (Abdollahzadeh et al., 2014; Field et al., 2015; Ghrairi and Hani, 2015).
In order to increase the effectiveness of essential oils when incorporated into a food matrix, several strategies have been proposed. One strategy is the microencapsulation and nano emulsification of essential oils (Estrada-Cano et al., 2017; Dávila-Rodríguez et al., 2019). Another strategy is the preparation of packing materials, such as the edible coats and films formed with chitosan, carboxymethylcellulose, or starch and added with essential oils. Both strategies aim to distribute essential oils evenly and reduce interaction with food ingredients (Paparella et al., 2016; Basak and Guha, 2017; Navarro-Cruz et al., 2018; Ochoa-Velasco et al., 2018).
The composition of the three Mexican oregano essential oils varied on their proportion of thymol and carvacrol; the difference was also observed in the growth of the molds studied. A. ochraceus was more sensible to the presence of OEO and higher concentrations of protein than P. expansum. The predominant effect was the added effect of protein content and concentration of OEO on the maximum fungal growth, with a lineal effect and no interaction among the two variables. Lag time was also affected by protein content, although the predictive models did not have statistical significance. The growth rate was not affected by the three variables studied.
The development of predictive models can provide information on the growth or inactivation of microorganism present in food, under various conditions. Also, it can help on the determination of the detrimental or synergistic effect of a particular food additive, such as essential oils bioactivities, in interaction with food components or food properties. The interactions among components of a food matrix can be very complex, and quantitative measurements of these interactions are useful on the determination of the final preservation effect in the food product. On the other hand, the variable chemical composition of essential oils will also affect their antifungal capacity. Even more, the response of fungal isolates even from the same fungal genera and species can vary, and the isolates will be differentially affected by the antifungal compounds. Further studies are needed to propose general trends on the interaction of food components, food characteristics, and natural food antimicrobials. These mathematical tools are useful for food industries to determine the food safety risk of processed food products. Scientific research in predictive microbiology can finally enrich databases for the development of software (tertiary models) that supporting food safety from processing to the table.
Data Availability Statement
The datasets generated for this study are available on request to the corresponding author.
Author Contributions
Experimental design: AL-G, AL-M, and GN-M. Experimentation: AL-G. Methods: RÁ-S, AL-G, and AL-M. Supervision: RÁ-S, and NG-M. Statistical analysis: RÁ-S, NG-M, and GN-M. Manuscript draft: AL-G. Final manuscript: GN-M. All author: read and approved the final manuscript.
Funding
Partial funding was provided by Universidad Autónoma de Chihuahua (UACH) and Universidad de las Américas Puebla (UDALP). AL-G held a scholarship from Consejo Nacional de Ciencia y Tecnología for her graduate studies (Fellowship No. 238772).
Conflict of Interest
The authors declare that the research was conducted in the absence of any commercial or financial relationships that could be construed as a potential conflict of interest.
References
Abdollahzadeh, E., Rezaei, M., and Hosseini, H. (2014). Antibacterial activity of plant essential oils and extracts: the role of thyme essential oil, nisin, and their combination to control Listeria monocytogenes inoculated in minced fish meat. Food Control 35, 177–183. doi: 10.1016/j.foodcont.2013.07.004
Akkermans, S., Nimmegeers, P., and Van Impe, J. F. (2018). A tutorial on uncertainty propagation techniques for predictive microbiology models: a critical analysis of state-of-the-art techniques. Int. J. Food Microbiol. 282, 1–8. doi: 10.1016/j.ijfoodmicro.2018.05.027
Albuquerque, C. C. D., Camara, T. R., Mariano, R. D. L. R., Willadino, L., Marcelino Júnior, C., and Ulisses, C. (2006). Antimicrobial action of the essential oil of Lippia gracilis Schauer. Brazilian Arch. Biol. Technol. 49, 527–535. doi: 10.1590/S1516-89132006000500001
Avila-Sosa Sanchez, R., Portillo-Ruiz, M. C., Viramontes-Ramos, S., Muñoz-Castellanos, L. N., and Nevarez-Moorillón, G. V. (2015). Effect of Mexican oregano (Lippia berlandieri Schauer) essential oil fractions on the growth of Aspergillus spp. in a bread model system. J. Food Process. Preserv. 39, 776–783. doi: 10.1111/jfpp.12287
Avila-Sosa, R., Hernández-Zamoran, E., López-Mendoza, I., Palou, E., Munguía, M. T. J., Nevárez-Moorillón, G. V., et al. (2010). Fungal inactivation by Mexican oregano (Lippia berlandieri Schauer) essential oil added to amaranth, chitosan, or starch edible films. J. Food Sci. 75, M127–M133. doi: 10.1111/j.1750-3841.2010.01524.x
Basak, S., and Guha, P. (2017). Use of predictive model to describe sporicidal and cell viability efficacy of betel leaf (Piper betle L.) essential oil on Aspergillus flavus and Penicillium expansum and its antifungal activity in raw apple juice. LWT - Food Sci. Technol. 80, 510–516. doi: 10.1016/j.lwt.2017.03.024
Cattelan, M. G., de Oliveira Nishiyama, Y. P., Gonçalves, T. M. V., and Coelho, A. R. (2018). Combined effects of oregano essential oil and salt on the growth of Escherichia coli in salad dressing. Food Microbiol. 73, 305–310. doi: 10.1016/j.fm.2018.01.026
Cid-Pérez, T. S., Ávila-Sosa, R., Ochoa-Velasco, C. E., Rivera-Chavira, B. E., and Nevárez-Moorillón, G. V. (2019). Antioxidant and antimicrobial activity of Mexican oregano (Poliomintha longiflora) essential oil, hydrosol and extracts from waste solid residues. Plants 8:E22. doi: 10.3390/plants8010022
Cid-Pérez, T. S., Nevárez-Moorillón, G. V., Torres-Muñoz, J. V., Palou, E., and López-Malo, A. (2016). Mexican oregano (Lippia berlandieri and Poliomintha longiflora) oils,” in Essential Oils in Food Preservation, Flavor and Safety, ed V. R. Preddy (San Diego, CA: Academic Press), 551–560. doi: 10.1016/B978-0-12-416641-7.00063-8
Davidson, P. M., and Harrison, M. A. (2002). Resistance and adaptation to food antimicrobials, sanitizers, and other process controls. Food Technol. 56, 69–78.
Dávila-Rodríguez, M., López-Malo, A., Palou, E., Ramírez-Corona, N., and Jiménez-Munguía, M. T. (2019). Antimicrobial activity of nanoemulsions of cinnamon, rosemary, and oregano essential oils on fresh celery. LWT - Food Sci. Technol. 112:108247. doi: 10.1016/j.lwt.2019.06.014
Estrada-Cano, C., Anaya-Castro, M. A., Muñoz-Castellanos, L. N., Amaya-Olivas, N., García-Triana, A., and Hernandez-Ochoa, L. (2017). Antifungal activity of microcapsulated clove (Eugenia caryophyllata) and Mexican oregano (Lippia berlandieri) essential oils against Fusarium oxysporum. J. Microb. Biochem. Technol. 9, 567–571. doi: 10.4172/1948-5948.1000342
Field, D., Daly, K., O'Connor, P. M., Cotter, P. D., Hill, C., and Ross, R. P. (2015). Efficacies of nisin A and nisin V semipurified preparations alone and in combination with plant essential oils for controlling Listeria monocytogenes. Appl. Environ. Microbiol. 81, 2762–2769. doi: 10.1128/AEM.00070-15
García-Díez, J., Alheiro, J., Pinto, A. L., Soares, L., Falco, V., Fraqueza, M. J., et al. (2017). Influence of food characteristics and food additives on the antimicrobial effect of garlic and oregano essential oils. Foods 6:44. doi: 10.3390/foods6060044
Ghrairi, T., and Hani, K. (2015). Enhanced bactericidal effect of enterocin A in combination with thyme essential oils against L. monocytogenes and E. coli O157: H7. J. Food Sci. Technol. 52, 2148–2156. doi: 10.1007/s13197-013-1214-5
Gutierrez, J., Barry-Ryan, C., and Bourke, P. (2008). The antimicrobial efficacy of plant essential oil combinations and interactions with food ingredients. Int. J. Food Microbiol. 124, 91–97. doi: 10.1016/j.ijfoodmicro.2008.02.028
Gutierrez, J., Barry-Ryan, C., and Bourke, P. (2009). Antimicrobial activity of plant essential oils using food model media: efficacy, synergistic potential and interactions with food components. Food Microbiol. 26, 142–150. doi: 10.1016/j.fm.2008.10.008
Hossain, F., Follett, P., Vu, K. D., Harich, M., Salmieri, S., and Lacroix, M. (2016). Evidence for synergistic activity of plant-derived essential oils against fungal pathogens of food. Food Microbiol. 53, 24–30. doi: 10.1016/j.fm.2015.08.006
Khan, I., Tango, C. N., Miskeen, S., Lee, B. H., and Oh, D. H. (2017). Hurdle technology: a novel approach for enhanced food quality and safety–a review. Food Control 73, 1426–1444. doi: 10.1016/j.foodcont.2016.11.010
Marinelli, L., Di Stefano, A., and Cacciatore, I. (2018). Carvacrol and its derivatives as antibacterial agents. Phytochem. Rev. 17, 903–921. doi: 10.1007/s11101-018-9569-x
McMeekin, T. A., Olleyi, J., Ratkowsky, D. A., and Ross, T. (2002). Predictive microbiology: towards the interface and beyond. Int. J. Food Microbiol. 73, 395–407. doi: 10.1016/S0168-1605(01)00663-8
Minitab 18 Statistical Software (2017). [Computer Software]. State College, PA: Minitab, Inc. Available online at: www.minitab.com
Navarro-Cruz, A. R., Ochoa-Velasco, C. E., Caballero-Alvarez, F. J., Lazcano-Hernández, M. A., Vera-López, O., López-Malo, A., et al. (2018). Effect of pH and Mexican oregano (Lippia berlandieri Schauer) essential oil added to carboxymethyl cellulose and starch edible films on listeria monocytogenes and staphylococcus aureus. J. Food Qual. 2018:659394. doi: 10.1155/2018/1659394
Ngnitcho, P. F. K., Khan, I., Tango, C. N., Hussain, M. S., and Oh, D. H. (2017). Inactivation of bacterial pathogens on lettuce, sprouts, and spinach using hurdle technology. Innov. Food Sci. Emerg. Technol. 43, 68–76. doi: 10.1016/j.ifset.2017.07.033
Ochoa-Velasco, C. E., Navarro-Cruz, A. R., Vera-López, O., Palou, E., and Avila-Sosa, R. (2018). Growth modeling to control (in vitro) Fusarium verticillioides and Rhizopus stolonifer with thymol and carvacrol. Revista argentina de Microbiol. 50, 70–74. doi: 10.1016/j.ram.2016.11.010
Ortega-Nieblas, M. M., Robles-Burgueño, M. R., Acedo-Félix, E., González-León, A., Morales-Trejo, A., and Vázquez-Moreno, L. (2011). Chemical composition and antimicrobial activity of oregano (Lippia palmeri S. Wats) essential oil. Revista Fitotecnia Mexicana 34, 11–17.
Paparella, A., Mazzarrino, G., Chaves-López, C., Rossi, C., Sacchetti, G., Guerrieri, O., et al. (2016). Chitosan boosts the antimicrobial activity of Origanum vulgare essential oil in modified atmosphere packaged pork. Food Microbiol. 59, 23–31. doi: 10.1016/j.fm.2016.05.007
Perricone, M., Arace, E., Corbo, M. R., Sinigaglia, M., and Bevilacqua, A. (2015). Bioactivity of essential oils: a review on their interaction with food components. Front. Microbiol. 6:76. doi: 10.3389/fmicb.2015.00076
Portillo-Ruiz, M. C., Viramontes-Ramos, S., Muñoz-Castellanos, L. N., Gastelum-Franco, M. G., and Nevarez-Moorillon, G. V. (2005). Antifungal activity of Mexican oregano (Lippia berlandieri Shauer). J. Food Prot. 68, 2713–2717. doi: 10.4315/0362-028X-68.12.2713
Possas, A., Posada-Izquierdo, G. D., Pérez-Rodríguez, F., Valero, A., García-Gimeno, R. M., and Duarte, M. C. (2017). Application of predictive models to assess the influence of thyme essential oil on Salmonella enteritidis behaviour during shelf life of ready-to-eat turkey products. Int. J. Food Microbiol. 240, 40–46. doi: 10.1016/j.ijfoodmicro.2016.08.003
Ribeiro-Santos, R., Andrade, M., and Sanches-Silva, A. (2017). Application of encapsulated essential oils as antimicrobial agents in food packaging. Curr. Opin. Food Sci. 14, 78–84. doi: 10.1016/j.cofs.2017.01.012
Rivera-Calo, J. R., Crandall, P. G., O'Bryan, C. A., and Ricke, S. C. (2015). Essential oils as antimicrobials in food systems–a review. Food Control 54, 111–119. doi: 10.1016/j.foodcont.2014.12.040
Tang, X., Shao, Y.-L., Tang, Y.-J., and Zhou, W.-W. (2018). Antifungal activity of essential oil compounds (Geraniol and Citral) and inhibitory mechanisms on grain pathogens (Aspergillus flavus and Aspergillus ochraceus). Molecules 23:2108. doi: 10.3390/molecules23092108
Keywords: Mexican oregano essential oil, food components, predictive microbiology, Penicillium expansum, Aspergillus ochraceus
Citation: Levario-Gómez A, Ávila-Sosa R, Gutiérrez-Méndez N, López-Malo A and Nevárez-Moorillón GV (2020) Modeling the Combined Effect of pH, Protein Content, and Mexican Oregano Essential Oil Against Food Spoilage Molds. Front. Sustain. Food Syst. 4:34. doi: 10.3389/fsufs.2020.00034
Received: 10 December 2019; Accepted: 09 March 2020;
Published: 31 March 2020.
Edited by:
Cristobal N. Aguilar, Universidad Autónoma de Coahuila, MexicoReviewed by:
Gerardo Mendez-Zamora, Autonomous University of Nuevo León, MexicoMiriam Desiree Davila Medina, Universidad Autónoma de Coahuila, Mexico
Balaram Mohapatra, Indian Institute of Technology Bombay, India
Copyright © 2020 Levario-Gómez, Ávila-Sosa, Gutiérrez-Méndez, López-Malo and Nevárez-Moorillón. This is an open-access article distributed under the terms of the Creative Commons Attribution License (CC BY). The use, distribution or reproduction in other forums is permitted, provided the original author(s) and the copyright owner(s) are credited and that the original publication in this journal is cited, in accordance with accepted academic practice. No use, distribution or reproduction is permitted which does not comply with these terms.
*Correspondence: Guadalupe Virginia Nevárez-Moorillón, dm5ldmFyZSYjeDAwMDQwO3VhY2gubXg=