- 1Division of Animal and Nutritional Sciences, Davis College, West Virginia University, Morgantown, WV, United States
- 2Division of Plant and Soil Sciences, Davis College, West Virginia University, Morgantown, WV, United States
- 3Division of Resource Economics and Management, Davis College, West Virginia University, Morgantown, WV, United States
This study was designed to evaluate two triple-wash procedures with commercial antimicrobials to inactivate foodborne pathogens and surrogate bacteria on cucumbers and tomatoes. Fresh, West Virginia locally grown cucumbers and tomatoes were dip-inoculated with Salmonella Typhimurium and Tennessee, Listeria monocytogenes (3-strain), and Enterococcus faecium. Produce was washed through two triple-wash steps (10 s each) including water dip, antimicrobial dip, and water dip (WAW), or water dip, water dip, and antimicrobial dip (WWA), followed by draining (2 min) on aluminum foil. A triple-water (WWW) process was also included as a water-only control. Tested treatments were (1) water; (2) sodium hypochlorite (SH, 100 ppm, pH 8.2); (3) acidified sodium hypochlorite (ASH, 100 ppm, pH 6.8 adjusted by citric acid); (4) lactic and citric acid blend (LCA, 2.5%); and (5) a H2O2-peroxyacetic acid mix [SaniDate-5.0 (SD) 0.0064, 0.25, and 0.50%]. Surviving bacteria were recovered on xylose lysine tergitol-4 (XLT-4, Salmonella), Modified Oxford (MOX, L. monocytogenes), and bile esculin agar (E. faecium). Data (two replicates, four samples/replicate) were analyzed using the mixed model procedure of SAS (P = 0.05). Counts of Salmonella, L. monocytogenes, and E. faecium on unwashed cucumbers and tomatoes were 5.42–6.23, 6.31–6.92, and 6.05 log colony-forming units (CFU)/produce, respectively. Triple-wash with water only reduced all three tested bacteria by 0.45–1.36 log CFU/fruit. Triple-wash by WWA with antimicrobials achieved additional reductions [least squares means (LsMeans)] of 0.38 log CFU/cucumber (Salmonella), 0.56 log CFU/cucumber (E. faecium), 1.48 log CFU/tomato (Salmonella), 1.09 log CFU/tomato (L. monocytogenes), and 0.71 log CFU/tomato more than the WAW procedure. Applying SD-0.25 and SD-0.50% solutions in triple-washing cucumbers and tomatoes resulted in reductions (P > 0.05) similar to ASH and greater reductions (P < 0.05) than SH and LCA. E. faecium was less susceptible (P < 0.05) or there was no difference (P > 0.05) in comparison with Salmonella in most cases, except for tomatoes treated with WWA. The results of this study indicate that SD could be used as an alternative antimicrobial agent for chlorine water in triple-wash processing at local small produce plants. Future pilot plant validation studies and cost-effectiveness analyses are needed for applying SD solutions in triple-wash by WV local small produce growers.
Introduction
Among foodborne pathogens, Salmonella is the second most common cause of foodborne illnesses (Dewey-Mattia et al., 2018). A multi-state outbreak of Salmonella Poona on sliced cucumber from 2015 to 2016 caused more than 900 cases of infection, and six deaths were recorded according to the Centers for Disease Control and Prevention (CDC) (CDC, 2019). In 2006, an outbreak across 21 states in the United States caused 183 illnesses, of which 22 patients were hospitalized (CDC, 2006). In 2017, Denmark, Finland, Germany, Ireland, and the United Kingdom were affected by an outbreak of Salmonella on cucumbers (EFSA and ECDC, 2018). Another popular fresh produce commodity, tomatoes, has been associated with Salmonella outbreaks as well. Tomatoes were also inked to a recent Salmonella outbreak in Sweden, with 71 identified infections/illnesses (Colombe et al., 2019). In 2015, tomatoes served at Chipotle restaurants in Minnesota were reported to be contaminated with Salmonella (Minnesota Department of Health, 2015).
Listeria monocytogenes is another pathogen of concern reported by the United Fresh Produce Association (UFPA) due to the higher fatality rate of listeriosis (United Fresh Produce Association, 2018). In Iran, the prevalence of L. monocytogenes in sampled cucumbers was reported to be 18% (Hossein et al., 2013). Depending on the area, the prevalence of L. monocytogenes ranged from 3.8% (Li et al., 2017) to 17.5% (Strawn et al., 2013) on cucumbers sampled from a farmers market. Although outbreaks caused by L. monocytogenes on tomatoes are uncommon, tomatoes were recognized as a common food crop susceptible to foodborne pathogens (Honjoh et al., 2016).
The consumption of fresh produce (fresh vegetables and fruits) has increased to 345 pounds (per capita availability) in 2017 [(USDAERS, 2019)]. In the United Sates; however, there has been increasing concern regarding the microbial safety of farmers market–sold produce (Scheinberg et al., 2017). Fresh produce accounted for 46% of foodborne illnesses according to a comprehensive analysis by the CDC (Painter et al., 2013). In a recent study in West Virginia and Kentucky farmers markets, 18.6% of spinach, 10.9% of tomatoes, 18.5% of peppers, and 56.3% of cantaloupes tested positive for Salmonella, and 3.8% of the produce samples were positive for Listeria spp. (Li et al., 2017). Fresh produce may be eaten without further processing; therefore, cross-contamination during transportation, or handling becomes a serious issue to ensure produce safety. As the demand for locally produced foods has increased nationwide, with an estimated $20 billion target by 2019 (USDA, 2015), produce safety becomes a recurring problem, especially in regions where raw consumption of fresh produce is common practice.
In 1998, the United States Food and Drug Administration (FDA) published the “Guide to Minimize Microbial Food Safety Hazards for Fresh Fruits and Vegetables” as the main guidelines for Good Agricultural Practices (GAP), which identified that antimicrobial chemicals in processing water may reduce the microbial load on the surface of produce (United States Food and Drug Administration, 1998). The West Virginia University (WVU) Extension Service Small Farm Center encourages small produce growers to apply a triple-wash process during their post-harvest processing if their produce is eaten raw or grown on the ground (Strohbehn et al., 2013). Although more evidence suggests that washing is more a cross-contamination preventative than a pathogen reduction step, the triple-wash process (water rinse, water rinse, and final antimicrobial dip) is still recommended for removing pathogens from food surfaces and for improving on-farm food safety with the assumption of clean water being used in each wash step (Strohbehn et al., 2013). The effectiveness of the triple-wash critically depends on the antimicrobial solutions used. Sodium hypochlorite (SH, referred to as chlorinated water) has been well-recognized and used extensively as an effective and low-cost sanitizer during the post-harvest washing process (Shen et al., 2013). The antimicrobial effect of chlorinated water against Salmonella was observed when spraying 200 ppm chlorinated water onto tomato surfaces (Bari et al., 2003). However, chlorinated water has obvious disadvantages including being easily degraded by organic matter and generating chlorine byproducts (Shen et al., 2016). Therefore, local produce growers are interested in learning the efficacy of new antimicrobial solutions. For example, Preston County Workshop Inc., a local small produce processor, is currently using SaniDate-5.0 [SD, a mix of peroxyacetic acid (PAA) and H2O2] in their triple-wash tanks to control foodborne pathogens on their produce. According to our recent internal survey from local small produce growers at the 2018 West Virginia Small Farm Conference produce safety training workshop, approximately half of the participants (9/20) currently choose water dip–antimicrobial dip–water dip (WAW), and the other half (10/20) use the water dip–water dip–antimicrobial dip (WWA) procedure. Recently, there is growing recognition that post-harvest washing reduces cross-contamination with no expectation of achieving log count reductions of pathogens on produce (Gombas et al., 2017). Therefore, the concentration level to be used and the antimicrobial efficacy of the triple-wash procedures need to be investigated.
The efficacy of antimicrobial solutions during triple-washing should be tested in real local small produce commercial settings. This is because the dynamics of processing conditions applied by local produce growers could be controlled to a lesser extent than under laboratory conditions. To validate washing procedures, local small produce plants typically have a great aversion to using an actual microbial test pathogen in their processing lines; instead, they usually apply alternative methods such as ensuring a sufficient active sanitizer within the wash tank. The use of a pathogen surrogate is a possible valid approach, and the target surrogate needs to be validated in laboratory conditions first (Hu and Gurtler, 2017). Enterococcus faecium, a Gram-positive chain-shaped cocci, has been studied on our WVU poultry farm as a safer alternative for Salmonella during steam conditioning, antimicrobial inclusion, and standard/thermal aggressive pelleting during broiler feed manufacturing (Boney et al., 2018; Boltz et al., 2019). Enterococcus faecium has also been validated as a surrogate for Salmonella in almond pasteurization (Jeong et al., 2011). However, this Salmonella surrogate has not been validated on fresh produce, and no publications identified the ideal surrogate for Salmonella during the post-harvest produce washing process.
As outbreaks associated with Salmonella and L. monocytogenes from fresh produce are of concern, a more comprehensive evaluation of washing procedures should be carried out. Therefore, the objectives of this study were to evaluate two triple-wash procedures with three commercial antimicrobials to inactivate Salmonella, L. monocytogenes, and the surrogate E. faecium on WV locally grown cucumbers and tomatoes.
Materials and Methods
Fresh Produce Sample Preparation and Background Microflora Elimination
Fresh cucumbers and tomatoes were purchased from WV Morgantown Farmers Market and stored overnight in a refrigerated cooler. Before each experiment, the population of natural microflora on produce surfaces was determined by adding one cucumber or tomato into 200 ml of buffered peptone water (BPW, Alpha Biosciences, Baltimore, MD, USA) with shaking for 30 s, followed by spread-plating onto tryptic soy agar (TSA, Alpha Biosciences, Baltimore, MD, USA) after 10-fold serial dilution and incubating at 35°C for 48 h. Results indicated that there were ~5–6 log colony-forming units (CFU)/produce of background microbiota on cucumber and tomato surfaces. It was noticed that the microflora on cucumbers interfered with the results of the E. faecium experiment, as the selective medium used for the E. faecium experiment was not selective enough to preclude background microbiota. Therefore, cucumber samples were decontaminated before being subjected to E. faecium inoculation. To reduce microbiota on cucumbers in the E. faecium experiment, a pre-wash procedure was conducted. Fresh cucumbers were first rinsed with tap water and then submerged in a chlorinated solution (40 ml bleach in 2 L water) for a minute, followed by submerging in boiling water for 5 s. This surface disinfection method was verified by showing growth of no colonies on bile esculin agar (BEA) after shaking cucumbers with 200 ml BPW following by spread-plating. The decontaminated cucumber samples were air-dried in a biosafety cabinet before inoculation.
Preparation of Inoculum
Salmonella Typhimurium ATCC 14028, Salmonella Tennessee ATCC 10722, L. monocytogenes strains L2624 and L2625 (cantaloupe outbreak serotype 1/2b, donated by Dr. Joshua Gurtler, USDA-ARS, Wyndmoor, PA), and surrogate E. faecium ATCC 8459 were used in this study. Both Salmonella and L. monocytogenes strains were used in the previously reported farmers market produce safety projects (Li et al., 2017, 2018), and this strain of E. faecium has also been studied in WVU poultry meat projects (Lemonakis et al., 2017; Boltz et al., 2019). Salmonella, L. monocytogenes, and E. faecium retrieved from frozen stock cultures were streak-plated onto xylose lysine tergitol-4 agar (XLT-4, Hardy Diagnostics, Santa Maria, CA, USA), Modified Oxford agar (MOX, Hardy Diagnostics, Santa Maria, CA, USA), and BEA (Hardy Diagnostics, Santa Maria, CA, USA), respectively, and then incubated at 35°C for 48 h to generate single colonies. Before each experiment, a single colony was picked from XLT-4 (Salmonella), MOX (L. monocytogenes), and BEA (E. faecium) of each strain and was enriched in 10 ml tryptic soy broth (TSB; Alpha Biosciences, Baltimore, MD, USA) at 35°C for 24 h. Then, each individual bacterial suspension was centrifuged (5,000 × g) for 15 min (VWR Symphony 4417, VWR International, Radnor, PA). Each suspension was then centrifuged and washed in triplicate in 0.1% BPW followed by re-suspending in 10 ml of 0.1% BPW. The Salmonella and L. monocytogenes inoculum was made by combining the two strains of Salmonella with the two L. monocytogenes strains (Li et al., 2017, 2018). After creating the four-strain cocktail, the inoculum was diluted to ca. 6 log CFU/ml by adding the 40 ml cocktail into 3 L of 0.1% BPW solution for the dip inoculation process. The inoculum level of E. faecium was adjusted to 6.5 log CFU/ml by adding 40 ml of triplicate-washed strains into 3 L of 0.1% BPW.
Inoculation of Fresh Produce Samples
Tomatoes and cucumbers were inoculated by placing the product in a metal bowl containing 3 L of Salmonella or Listeria inoculum with gentle stirring for 5 min, followed by placement in a biosafety cabinet for 15 min to allow for pathogen attachment. According to our preliminary studies, E. faecium was inoculated onto tomato and cucumber samples by pipetting 1 ml of inoculum onto the samples, fully covering the surface using food-grade plastic wrap for 30 s, and then air-drying in a biosafety cabinet for 15 min.
Triple-Wash Produce With Antimicrobials
Before the triple-washing process, the inoculated produce (cucumbers and tomatoes) was tested for temperature using a scan thermometer (Exergen Corporation, Watertown, MA, USA), and the surface temperatures of both products were 46.76 ± 0.6°F (8.2°C). Inoculated samples were left unwashed (control) or triple-washed in three metal containers with 3 L of solution each. Each treatment contained six samples randomly and evenly split into two groups; each sample contained either one tomato or one cucumber. Two triple-wash procedures were applied to the samples, including WAW or WWA. Each step in the triple-wash procedure was completed by dipping the samples into the solutions with manual rotation for 10 s with agitation at ca. 200 rpm (Li et al., 2017). Treatments tested include: (i) tap water only (pH = 6.9, 15.4°C); (ii) SH [free available chlorine 100 ± 0.6 ppm, pH = 8.2 (SH), or pH = 6.8 (adjusted by 10% citric acid: acidified SH, ASH), 14.4°C; Birko, Henderson, CO, USA]; (iii) a lactic/citric acid blend (LCA, Veggiexide®, 2.5%, pH = 5.1, 15.4°C, Birko); and (iv) a H2O2-PAA mix (SD, 15.2°C; Arbico Organics, Tucson, AZ, USA) with concentrations of 0.0064% (pH 6.25), 0.25% (pH 5.52), and 0.50% (pH 3.75). Free chlorine concentration was measured using the N, N diethyl-1,4 phenylenediamine sulfate method with a chlorine photometer (CP-15, HF Scientific, Inc., Ft. Myers, FL). Temperatures of all wash solutions ranged from 57.9 to 59.7°F (14.4–15.4°C), which meets the U.S. FDA advisory recommendation that the wash should be 10°F higher than the produce (46.8°F) being washed (United States Food and Drug Administration, 2018). After triple-wash procedures, samples were drained and dried on aluminum foil for 2 min.
Microbiological Analysis
Each unwashed and washed produce sample was placed into a sterile sample bag (Nasco, Fort Atkinson, WI, USA) and rinsed in 200 ml of BPW, followed by vigorously shaking for 60 s to detach bacteria from the surface. Sample rinse solutions were then 10 or 100-fold serially diluted in 0.1% BPW and spread-plated (0.1 ml onto one plate or 1.0 ml equally divided onto three plates) on XLT-4, MOX, and BEA agar to enumerate Salmonella, L. monocytogenes, and E. faecium cells, respectively. All agar plates were than incubated at 35°C for 24 h (XLT-4) or 48 h (MOX and BEA) and were manually counted for CFU after the incubation period. Three different dilutions were used for spread-plating of each sample including “0” dilution by adding 1 ml of 200 ml BPW diluent equally split onto three agar plates (0.33 ml each). Numbers of colonies from each of the three plates were then combined following incubation; therefore, the detection limit was 2.3 log CFU/fruit (=log10 200 CFU/fruit). The presumptive positive colonies of Salmonella and L. monocytogenes were confirmed by using an Oxoid latex agglutination test kit (Oxoid Ltd, Basingstoke, Hampshire, UK).
Data Analysis
The triple-wash studies were duplicated with four cucumbers and four tomatoes per treatment per repetition, with a total of eight samples per treatment for each bacterium. The experimental design was a randomized 2 × 6 factorial design with two triple-wash procedures (WAW or WWA) and six antimicrobial treatments. The survival and reduction of Salmonella, L. monocytogenes, and E. faecium were analyzed using the mixed model procedure of SAS (version 9.2, SAS Institute, Cary, NC), including individual factors of triple-wash procedures, antimicrobial treatments, and their interactions. The comparison of reductions between Salmonella and the surrogate E. faecium was also analyzed using the mixed model procedure. The reduction data were determined by a reduction ratio of log10 (N0/N), which includes N0, the average control plate counts, and N, the plate count of each individual antimicrobial treated sample (Adler et al., 2016). Means were separated by Tukey Honestly Significant Difference (HSD) at an α = 0.05 significance level.
Results
Comparison of WAW and WWA Procedures
In general, the least squares mean (LsMean) values across the six tested antimicrobial treatments (water-only wash was not included) indicated that triple-washing with WWA is more effective (P < 0.05) than WAW in reducing Salmonella (2.39 vs. 2.01 log CFU/cucumber) and E. faecium (2.16 vs. 1.60 log CFU/cucumber) on cucumbers, and reducing Salmonella (2.82 vs. 1.34 log CFU/tomato), L. monocytogenes (2.35 vs. 1.26 log CFU/tomato), and E. faecium (2.81 vs. 2.10 log CFU/tomato) on tomatoes. Although there is a statistical difference between the WWA and WAW processes, the differences in log reductions ranged from 0.38 to 1.44 log CFU/fruit, which are still relatively low. Applying WWA or WAW procedures on cucumbers showed no difference in reduction (1.39 vs. 1.35 log CFU/cucumber) for L. monocytogenes.
Efficacy of Triple-Wash With Antimicrobials Against Salmonella
Survival and reductions of Salmonella on cucumbers are shown in Table 1. All six antimicrobial treatments were more effective (P < 0.05) in reducing Salmonella than the water-only wash (1.26–1.36 log CFU/cucumber greater inactivation, Table 1). Compared to SH, ASH increased reductions of the pathogen by 0.41 (P < 0.05, WAW) and 0.32 log CFU/cucumber (P = 0.06, WWA). As expected, antimicrobials applied in the WAW procedure for cucumbers significantly (P < 0.05) reduced the Salmonella population (survivals of 3.36–4.17 log CFU/cucumber) more than the untreated control (5.80 log CFU/cucumber), with the reductions ranging from 1.63 (SD-0.0064%) to 2.44 (SD-0.50%) log CFU/cucumber (Table 1). Compared to WAW, SH, SD-0.0064%, and SD-0.25% applied in the WWA process achieved an additional (P < 0.05) reduction of Salmonella by 0.40 to 0.50 log CFU/cucumber (Table 1). However, there was no significant (P > 0.05) difference in reductions between WAW and WWA in ASH, LCA, and SD-0.50% washed samples (Table 1). Compared to SH and LCA, adding SD (0.0064, 0.25, 0.50%) into the triple-wash process showed similar or greater (P < 0.05) reductions (1.63–2.44 vs. 1.82–2.00 log CFU/cucumber for WAW, 2.09–2.66 vs. 2.14–2.43 log CFU/cucumber for WWA, Table 1). Compared to ASH, SD-0.25% and SD-0.50% showed similar (P > 0.05) reductions ranging from 2.18 to 2.44 log CFU/cucumber (WAW) and 2.43–2.75 log CFU/cucumber (WWA).
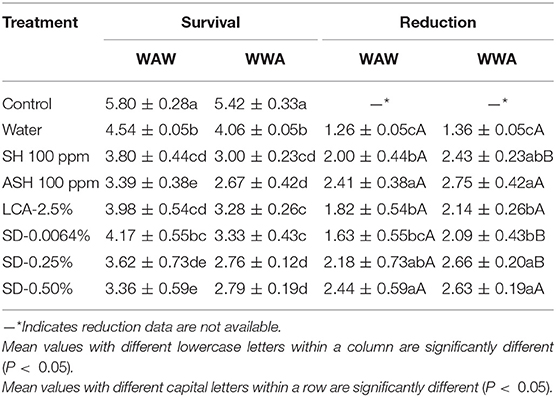
Table 1. Survival and reduction of Salmonella Typhimurium and Tennessee on cucumbers [log colony-forming units (CFU)/cucumber] by triple-wash procedure water dip–antimicrobial dip–water dip (WAW) or water dip–water dip–antimicrobial dip (WWA) in water, sodium hypochlorite (SH, 100 ppm, pH 8.2), acidified SH (ASH; SH, 100 ppm, pH 6.8 adjusted by citric acid), lactic and citric acid blend (LCA, Veggiexide®, 2.5%), and a peroxyacetic acid (PAA) and hydrogen peroxide mix [SaniDate-5.0 (SD), 0.0064, 0.25, and 0.50%].
As shown in Table 2, triple-washing tomatoes in antimicrobials by the WAW process significantly (P < 0.05) reduced Salmonella, with the survival populations ranging from 3.72 to 4.80 log CFU/tomato compared to 5.70 log CFU/tomato (unwashed control). All six tested antimicrobials were more effective (P < 0.05) at reducing the pathogen than the water-only wash, except for LCA. Again, ASH achieved additional (P < 0.05) reduction of 0.66 log CFU/tomato compared with the SH washed samples. SD-0.25% and SD-0.50% treated samples showed similar (P > 0.05) reductions (1.63–1.98 log CFU/tomato) compared to ASH (1.98 log CFU/tomato), and greater (P < 0.05) reductions than SH (1.32 log CFU/tomato) and LCA (0.90 log CFU/tomato). The reduction by SD-0.0064% was similar (P > 0.05) to SH and LCA but less (P < 0.05) than ASH. Compared to WAW, applying the WWA process increased (P < 0.05) the reduction levels from 1.32 to 3.14, 1.98 to 3.30, 0.90 to 3.28, 1.13–1.72, 1.98–3.35, and 1.63–3.26 log CFU/tomato for SH, ASH, LCA, SD-0.0064, SD-0.25, and SD-0.50%, respectively (Table 2).
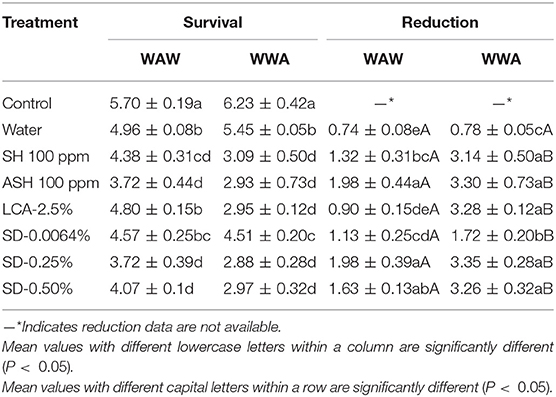
Table 2. Survival and reduction of Salmonella Typhimurium and Tennessee on tomatoes (log CFU/tomato) by triple-wash procedure WAW or WWA in water, SH (100 ppm, pH 8.2), ASH (SH, 100 ppm, pH 6.8 adjusted by citric acid), LCA (Veggiexide®, 2.5%), and a PAA and hydrogen peroxide mix (SD, 0.00645, 0.25, and 0.50%).
Efficacy of Triple-Wash With Antimicrobials Against L. monocytogenes
Survival and reductions of L. monocytogenes on cucumbers and tomatoes are shown in Tables 3, 4, respectively. As shown in Table 3, triple-washing cucumbers in the six antimicrobials by the WAW process significantly (P < 0.05) reduced L. monocytogenes, with the survival populations ranging from 4.44 to 5.55 log CFU/cucumber compared to 6.31 log CFU/cucumber for the control (Table 3). For the WAW process, reductions of SH, ASH, LCA, SD-0.25%, and SD-50% were greater than the water control (0.59 log CFU/cucumber) except for SD-0.0064%, showing similar reduction (0.76 log CFU/cucumber). Among the tested antimicrobial treatments, no difference (P > 0.05) was found between the reductions (1.56–1.87 log CFU/cucumber) caused by SH, ASH, SD-0.25%, and SD-0.50%, which were greater (P < 0.05) than LCA (1.23 log CFU/cucumber) and SD-0.0064% (0.76 log CFU/cucumber) treatments. Applying the WWA process increased (P < 0.05) reductions from 1.64 to 2.25, 1.87 to 2.41, and 0.76 to 1.30 log CFU/cucumber for SH, ASH, and SD-0.0064% washed samples, respectively (Table 3). However, the WWA process did not increase reductions of the pathogen on cucumbers washed in LCA, SD-0.25%, and SD-0.50% as compared to the WAW process (Table 3).
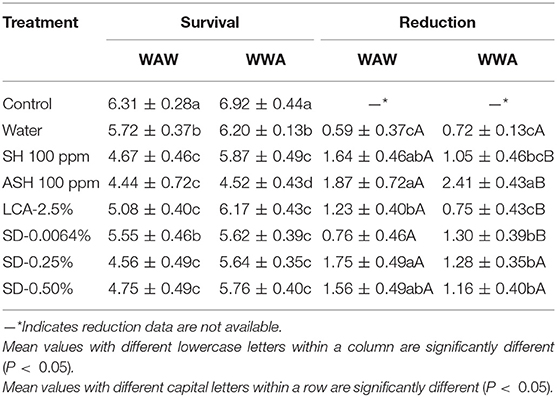
Table 3. Survival and reduction of Listeria monocytogenes on cucumbers (log CFU/cucumber) by triple-wash procedure WAW or WWA in water, SH (100 ppm, pH 8.2), ASH (SH, 100 ppm, pH 6.8 adjusted by citric acid), LCA (Veggiexide®, 2.5%), and a PAA and hydrogen peroxide mix (SD, 0.0064, 0.25, and 0.50%).
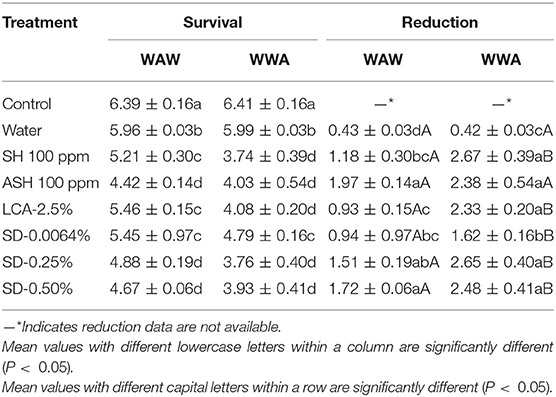
Table 4. Survival and reduction of L. monocytogenes on tomatoes (log CFU/tomato) by triple-wash procedure WAW or WWA in water, SH (100 ppm, pH 8.2), ASH (SH, 100 ppm, pH 6.8 adjusted by citric acid), LCA (Veggiexide®, 2.5%), and a PAA and hydrogen peroxide mix (SD, 0.0064, 0.25, and 0.50%).
The efficacy of antimicrobials in inactivating L. monocytogenes on tomatoes has not been widely studied. As shown in Table 4, significantly lower survival (4.42–5.46 log CFU/tomato) was observed on tomatoes washed in the six antimicrobials using the WAW process compared with the untreated control (6.39 log CFU/g) and water wash control (5.96 log CFU/g). SD-0.25% and SD-0.50% reduced the pathogen counts by 1.51–1.72 log CFU/tomato, which were slightly (P > 0.05) lower than ASH (1.97 log CFU/tomato) and greater (P < 0.05) than the reductions of SH (0.93 log CFU/tomato), LCA (1.18 log CFU/tomato), and SD-0.0064% (0.94 log CFU/tomato, Table 4). Compared to the WAW process, applying WWA procedures (P < 0.05) increased reductions of all six tested antimicrobial treatments by 0.49–1.40 log CFU/tomato (Table 4).
Comparison of Salmonella vs. Surrogate E. faecium
The response of surrogate E. faecium to antimicrobials on cucumbers and tomatoes is shown in Tables 5, 6, respectively. Triple-wash with only water reduced (P < 0.05) the surrogate by 0.56 log CFU/cucumber and 0.45 log CFU/tomato compared to the unwashed control. ASH showed greater (P < 0.05) and similar (P ≥ 0.05) reductions compared to SH for cucumbers and tomatoes, respectively. For the WAW process, reductions obtained after washing in SH, ASH, LCA, SD-0.0064, SD-0.25, and SD-0.50% ranged from 0.80 to 2.27 log CFU/cucumber (Table 5) and from 1.34 to 2.90 log CFU/tomato (Table 6). Like Salmonella, the application of the WWA process with the six tested antimicrobials resulted in additional (P < 0.05) reductions of 0.25–0.83 log CFU/cucumber and 0.49–1.22 log CFU/tomato, respectively, compared to the WAW process. For cucumbers, the application of SD-0.0064, SD-0.25, and SD-0.50% solutions showed similar (P > 0.05) reductions (1.82–2.27 log CFU/cucumber for WAW, 2.48–2.96 log CFU/cucumber for WWA) compared to ASH (2.08 log CFU/cucumber for WAW, 2.39 log CFU/cucumber for WWA), but better (P < 0.05) reductions than SH and LCA treatments in both the WAW (0.80–0.92 log CFU/cucumber) and WWA (1.05–1.63 log CFU/cucumber) processes (Table 5). For tomatoes, SD-0.25% and SD-0.50% created greater (P < 0.05) reductions (2.48–2.90 log CFU/tomato) than SH (1.88 log CFU/tomato), ASH (1.37 log CFU/tomato), and LCA (1.34 log CFU/tomato) treated samples using the WAW process. Applying the WWA process, SD-0.50% was the most effective (P < 0.05) in reducing the pathogen surrogate from tomatoes (3.53 log CFU/tomato, Table 6), followed by SD-0.25%, SH, LCA, and ASH, which showed similar reductions of 2.50–2.97 log CFU/tomato.
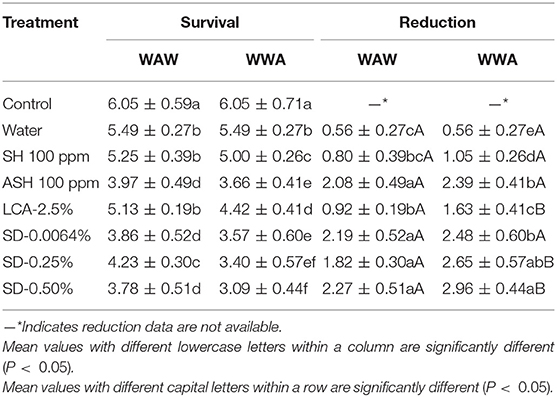
Table 5. Survival and reduction of Enterococcus faecium on cucumbers (log CFU/cucumber) by triple-wash procedure WAW or WWA in water, SH (100 ppm, pH 8.2), ASH (SH, 100 ppm, pH 6.8 adjusted by citric acid), LCA (Veggiexide®, 2.5%), and a PAA and hydrogen peroxide mix (SD, 0.0064, 0.25, and 0.50%).
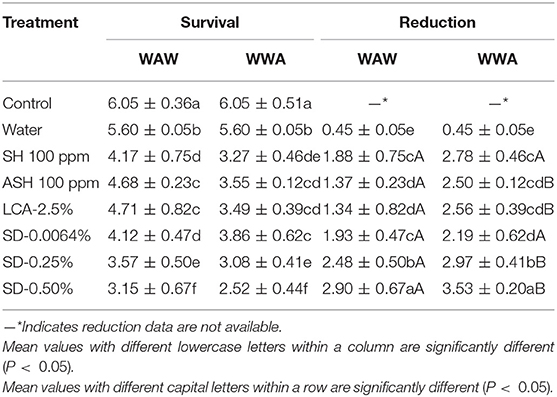
Table 6. Survival and reduction of E. faecium on tomatoes (log CFU/tomato) by triple-wash procedure WAW or WWA in water, SH (100 ppm, pH 8.2), ASH (SH, 100 ppm, pH 6.8 adjusted by citric acid), LCA (Veggiexide®, 2.5%), and a PAA and hydrogen peroxide mix (SD, 0.0064, 0.25, and 0.50%).
In this study, the mixed model procedure was applied to compare the reductions of Salmonella vs. the surrogate E. faecium, as shown in Table 7 (cucumbers) and 8 (tomatoes). For cucumbers, the reductions of E. faecium after the application of the WAW or WWA process with the six tested antimicrobial treatments were less (LsMeans 1.60 vs. 2.01 log CFU/cucumber for WAW, P < 0.05) or not different (LsMeans 2.16 vs. 2.39 log CFU/cucumber for WWA, P > 0.05) compared to Salmonella. Specifically, application of SH, ASH, and LCA indicated lower (P < 0.05) reductions, which ranged from 0.80 to 2.08 log CFU/cucumber (WAW) and 1.05–2.39 log CFU/cucumber (WWA) for E. faecium compared to Salmonella reduced by WAW (1.82–2.41 log CFU/cucumber) and WWA (2.14–2.75 log CFU/cucumber) (Table 7). SD-0.25 and SD-50% reduced E. faecium by 1.82–2.27 log CFU/cucumber (WAW) and 2.65–2.96 log CFU/cucumber (WWA), which were similar (P > 0.05) to the reductions of Salmonella (Table 7).
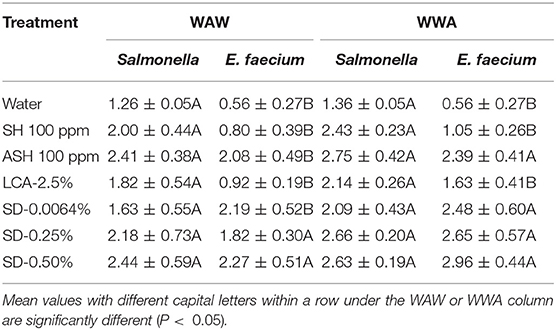
Table 7. A comparison of the reduction of Salmonella and surrogate E. faecium on cucumbers (log CFU/cucumber) by triple-wash procedure WAW) or WWA in water, SH (100 ppm, pH 8.2), ASH (SH, 100 ppm, pH 6.8 adjusted by citric acid), LCA (Veggiexide®, 2.5%), and a PAA and hydrogen peroxide mix (SD, 0.0064, 0.25, and 0.50%).
For tomatoes, the reduction of E. faecium from WAW with antimicrobials was greater (P < 0.05) than the reduction of Salmonella (LsMeans 2.10 vs. 1.39 log CFU/tomato) but similar (P > 0.05) to the reduction of Salmonella in WWA processed samples (LsMeans 2.80 vs. 2.81 log CFU/tomato). Specifically, E. faecium on tomatoes washed through WWA with SD-0.0064, SD-0.25, and SD-0.50% showed no significant difference (P > 0.05) compared to Salmonella, with reductions of 2.19–3.53 log CFU/tomato (E. faecium) compared to reductions of 1.72–3.35 log CFU/tomato (Salmonella) (Table 8).
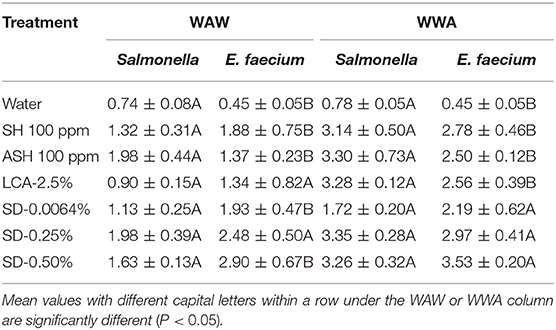
Table 8. A comparison of the reduction of Salmonella and surrogate E. faecium on tomatoes (log CFU/tomato) by triple-wash procedure WAW or WWA in water, SH (100 ppm, pH 8.2), ASH (SH, 100 ppm, pH 6.8 adjusted by citric acid), LCA (Veggiexide®, 2.5%), and a PAA and hydrogen peroxide mix (SD, 0.0064, 0.25, and 0.50%).
Discussion
Results from this study suggest that applying the WWA process during triple-wash is a better approach than WAW for reducing foodborne pathogens. This conclusion could possibly be explained by the fact that the residual sanitizers after the WWA process further inactivate pathogens on produce samples, since a neutralization step was absent from this study. The WWW control showed reductions of 0.5–1.2 log CFU/fruit across all tested pathogens on cucumbers and tomatoes in this study. These results are similar to those of Wang and Ryser (2014), who reported a 1.0 log CFU/g reduction of Salmonella from plain water wash for 15 s in a pilot-scale processing line.
As the physiochemical properties of cucumber and tomato surfaces are greatly different, it is plausible that the same sanitizer may not result in the same level of antimicrobial effect. Our results suggest that it is critical to consider the types of fresh produce when choosing a sanitizer to reduce foodborne pathogens, as the antimicrobial effect of one sanitizer may vary. Furthermore, the amount of organic load created by dust, soil, and debris from produce surfaces could impact the washing process.
Chlorine is a common sanitizer used in fresh produce processing due to the economic feasibility and the strong antimicrobial effect preventing cross-contamination (Shen et al., 2013). When chlorinated water is used as a sanitizer for fresh produce, the maximum concentration regulated by the U.S. FDA is 200 ppm. However, even 100 ppm chlorinated water demonstrates strong antimicrobial activity. An earlier study reported that tomatoes dipped in 100 ppm chlorinated water for 30 s showed significant reduction of Salmonella, and the level of reduction was not different between 30 s, 1, and 2 min treatments (Wei et al., 1995). A recent study by Sreedharan et al. (2017) showed that 100 ppm of free chlorine reduced Salmonella by > 4.5 log CFU/tomato in a model flume water for 30 s. It suggested that longer treatment time with chlorinated water was not necessary for fresh produce against Salmonella, which benefits actual produce processing plants. When immersing tomatoes into 200 ppm chlorinated water, its antimicrobial activity against Salmonella was significantly higher than 1 or 2 ppm ozonated water under different levels of turbidity of the water (Chaidez et al., 2007). Currently, there are no small produce growers (we contacted eight local growers in WV) that acidify the chlorine wash water before triple-washing their produce in WV (personal communication with Dr. Tom McConnell, Program Leader of the WV Small Farm Center). However, during the large-industry-scale produce washing process, adjusting the pH of chlorine solutions to 6.8 with citric acid is often conducted to ensure that the protonated form of hypochlorite predominates in the wash solution (Luo et al., 2012). Therefore, chlorine solutions with near-neutral pH at 6.8 adjusted by citric acid were included as a test treatment in this study. The results of this study suggest that the antimicrobial efficacy of chlorinated water in the concentration of 100 ppm was significant against Salmonella, and the reductions were improved when the pH of SH was adjusted to 6.8 in most cases, except for E. faecium on tomatoes. A previous study by Wang and Ryser (2014) also reported that chlorine plus citric acid (pH 6.0) yielded a greater reduction (3.1 log CFU/g) of Salmonella on tomatoes than chlorine at alkaline status (2.1 log CFU/g). This is because hypochlorous acid, the most effective antimicrobial component, predominates in chlorine water at near-neutral pH, whereas the hypochlorite would be in the ionic form rather than the antimicrobial protonated state in an alkaline-pH solution (White, 2010). The antimicrobial effect of 100 ppm SH or ASH can also be maximized when the WWA triple-wash procedure is used on cucumbers and tomatoes, as the reduction of Salmonella on cucumbers and tomatoes was significantly higher in the WWA than the WAW process.
The antimicrobial activity of chlorinated water is not limited to Salmonella. L. monocytogenes., another common food pathogen, was also sensitive to chlorinated water treatment on the surface of fresh produce. On cucumbers, 1 or 2 min washing with 200 ppm chlorinated water demonstrated the same level of Salmonella reduction (Yuk et al., 2006). Spraying 200 ppm chlorinated water on tomatoes significantly reduced L. monocytogenes on tomatoes (Beuchat et al., 1998; Bari et al., 2003). Surprisingly, although a L. monocytogenes outbreak associated with cucumber has been reported (Meldrum et al., 2009; Ponniah et al., 2012; Hossein et al., 2013), the antimicrobial effect of chlorine water against L. monocytogenes is relatively lacking in the current literature. Considering the accessibility of chlorinated water, chlorinated water without, and with neutralizing pH was included in this study to better contribute to the current database of antimicrobial effects against L. monocytogenes on cucumbers (Table 3). Our results suggest that 100 ppm chlorinated water (SH) is an effective antimicrobial against L. monocytogenes on cucumbers, and neutralizing pH to 6.8 by citric acid and the WWA procedure maximize the antimicrobial effect of chlorinated water, as significantly higher reduction was observed. On tomatoes, although 100 ppm SH is still an effective sanitizer (Table 4), the antimicrobial efficacy of SH against L. monocytogenes was significantly increased when the pH was adjusted to 6.8 (ASH), which was similar to SD-0.25% and SD-0.50% and >SD-0.0064% and LCA.
Recently, there is growing interest for produce processors to apply antimicrobial chemicals rather than chlorine during produce washing, as chlorine water easily reacts with water constituents and generates chlorine byproducts after repeated replenishing with new chlorine solutions (López-Gálvez et al., 2012; Shen et al., 2016). Local small produce growers in WV are also losing interest in chlorine use due to the increased marketability of natural and organic fresh produce (personal communication with Dr. Tom McConnell, Program Leader of the WV Small Farm Center). LCA, a buffered mixture of lactic and citric acid solution, was introduced by the food chemical industry about a decade ago and was reported to be effective in reducing Salmonella on poultry carcasses, avoiding the discoloration of chicken meat caused by lactic acid solutions (Laury et al., 2009). The only study of LCA on produce demonstrated that spraying 2.5% LCA onto jalapeno peppers through a commercial cabinet reduced the natural flora, Salmonella, and the surrogate generic Escherichia coli by 1.3, 1.1, and 0.8 log CFU/g, respectively (Adler et al., 2016). The mechanism of LCA to inhibit bacterial survival is the combination effect of lactic and citric acids. Lactic acid decreases the ionic concentration within the bacterial cell membrane of the exterior cell wall, and citric acid can diffuse through the cell membrane, being a weak non-dissociated acid. The combination of both acids leads to an accumulation of the acid within the cell cytoplasm, acidification of the cytoplasm, disruption of the proton motive force, and inhibition of substrate transport (Vasseur et al., 1999). Results showed that similar reductions (< 0.5 log CFU/g) were achieved by LCA compared to SH against Salmonella, L. monocytogenes, and the surrogate E. faecium for most tests in the current study. However, LCA was less effective than ASH for inactivating Salmonella on tomatoes and E. faecium on cucumbers.
SD is a mixed antimicrobial solution composed of 23% H2O2, 5.3% PAA, and 70% unknown ingredients, which has been recommended by the WV Small Farm Center to wash fresh produce processed from local small farms (Li et al., 2017), since the major wholesale buyer in WV Appalachian Harvest requires the use of SD as part of the post-harvest protocol for growers selling to their business, especially for the organic farming process (personal communication with Dr. Tom McConnell, Program Leader of the WV Small Farm Center). Like other oxidizing chemicals, SD oxidizes bacterial cells, denatures protein, and further disrupts the cell wall structure to kill or inhibit bacteria (Block, 2011). A previous study by Briñez et al. (2006) reported that a mix of H2O2 and PAA reduced nonpathogenic strains of Staphylococcus, Listeria spp., and E. coli by more than 5 log CFU/ml after 10 min contact even with organic matter present in solutions (Briñez et al., 2006). The results of the present study suggested similar (P > 0.05) reductions of Salmonella, L. monocytogenes, and E. faecium on cucumbers and tomatoes achieved by SD-0.25 and SD-50% when compared to ASH, which were greater (P < 0.05) than the reductions with SH and LCA solutions. The market price of a 5-gallon pallet of SD is $330 compared to $12 for SH and $108.5 for LCA. Therefore, agricultural economic cost-effectiveness analysis is needed to verify that SD is economically feasible for local small produce growers as an alternative antimicrobial solution to chlorine water.
Enterococcus faecium has been previously studied and validated as a potential Salmonella surrogate in almonds (Jeong et al., 2011), a balanced carbohydrate–protein meal (Bianchini et al., 2014), and pet foods (Ceylan and Bautista, 2015) during thermal activation processing. Our recent study also confirmed that E. faecium could be a non-pathogenic surrogate of Salmonella for in-plant antimicrobial validation studies on broiler carcasses (Lemonakis et al., 2017). To evaluate the suitability of choosing surrogate microorganisms for foodborne pathogens when exposed to antimicrobials, the surrogate should behave equally well or better (resistant to interventions) compared to the target pathogen in challenge studies (Adler et al., 2016). Therefore, side-by-side comparisons of reduction levels of Salmonella and E. faecium after triple-washing through WAW or WWA with antimicrobials on cucumbers and tomatoes are presented in Tables 7, 8. Results indicated that the E. faecium strain used in this study could potentially be a surrogate of Salmonella for validating triple-wash with commercial antimicrobials on cucumbers in local small produce processing settings; however, more studies are still needed to confirm its use on tomatoes as a Salmonella surrogate since opposite results were generated from the WAW compared with the WWA process. Other non-pathogenic bacteria such as generic E. coli (ATCC BAA-1427, ATCC BAA-1428, ATCC BAA-1429, ATCC BAA-1430, and ATCC BAA-1431) could be surrogates for Salmonella on different produce commodities including tomatoes (Adler et al., 2016). This may be surmised from our previous pilot plant trial, which showed that spraying 50 ppm SH or 1.0% LCA reduced the generic E. coli on jalapeno peppers by 0.8–1.0 log CFU/g, which was not different from the reductions of Salmonella (0.5–1.1 log CFU/g) (Adler et al., 2016).
Conclusions
Under the conditions of this study, the triple-wash WWA procedure was better than WAW at inactivating Salmonella, L. monocytogenes, and E. faecium on cucumbers and tomatoes. SD at concentrations of 0.25 and 0.50% was similar or better in antimicrobial efficacy compared to chlorine water without or with pH adjustment. Enterococcus faecium could be a potential Salmonella surrogate used for validation studies of antimicrobial treatments during post-harvest produce washing. The results from this study provide important information for local small produce growers who are interested in adopting the triple-wash procedure during post-harvest processing. Future studies are needed to validate the same procedure in commercial pilot plant settings, and cost-effectiveness analyses are necessary to evaluate whether SD is economically feasible for local small produce processors.
Limitations of This Study
The authors recognize the following limitations of this study. First, this extension validation study is valuable for local, very small produce growers in WV, which do not represent large commercial-scale industry produce processing. Second, the extent of cross-contamination in the different wash regimes of the triple-wash process was not reported in this study. It is well-established that preventing cross-contamination is more critical than reduction of pathogens during the produce washing process (Gombas et al., 2017). A cross-contamination study of triple-wash in three washing tanks with or without antimicrobials should be included in future studies. Third, the cucumbers tested for E. faecium were pre-treated to remove background microbiota, which may not well represent the cucumbers' surface characteristics. An antibiotic marker should be introduced into E. faecium to solve this issue in our future related studies. Fourth, the absence of a neutralization step from the WWA process may promote further pathogen reduction by the residual sanitizer on produce samples.
Data Availability Statement
The raw data of the whole study are recorded by hand in our lab notebooks and stored electronically in multiple electronic devices, which will be available from the corresponding author to any researchers who are interested in our results.
Author Contributions
LJ created the idea of this study. KL, Y-CC, and WJ designed and conducted the experiments. KL and CS performed statistical analysis. KL, Y-CC, and CS drafted this manuscript. XE revised this manuscript.
Funding
This research was funded by the United States Department of Agriculture, the National Institute of Food and Agriculture, and the Agriculture and Food Research Initiative (AFRI) Critical Agricultural Research and Extension (CARE) (Grant # 2019-68008-29828).
Conflict of Interest
The authors declare that the research was conducted in the absence of any commercial or financial relationships that could be construed as a potential conflict of interest.
References
Adler, J. M., Cain-Helfrich, E. D., and Shen, C. (2016). Reductions in natural microbial flora, nonpathogenic Escherichia coli, and pathogenic Salmonella on Jalapeno peppers processed in a commercial antimicrobial cabinet: a pilot plant trial. J. Food Prot. 79, 1854–1859. doi: 10.4315/0362-028X.JFP-16-222
Bari, M. L., Sabina, Y., Isobe, S., Uemura, T., and Isshiki, K. (2003). Effectiveness of electrolyzed acidic water in killing Escherichia coli O157:H7, Salmonella enteritidis, and listeria monocytogenes on the Surfaces of tomatoes. J. Food Prot. 66, 542–548. doi: 10.4315/0362-028X-66.4.542
Beuchat, L. R., Nail, B. V., Adler, B. B., and Clavero, M. R. S. (1998). Efficacy of spray application of chlorinated water in killing pathogenic bacteria on raw apples, tomatoes, and lettuce. J. Food Prot. 61, 1305–1311. doi: 10.4315/0362-028X-61.10.1305
Bianchini, A., Stratton, J., Weier, S., Hartter, T., Plattner, B., Rokey, G., et al. (2014). Use of Enterococcus faecium as a surrogate for Salmonella enterica during extrusion of a balanced carbohydrate-protein meal. J. Food Prot. 77, 75–82. doi: 10.4315/0362-028X.JFP-13-220
Block, S. S. (2011). Disinfection, Sterilization, and Preservation. Philadelphia, PA: Lippincott Williams & Wilkins.
Boltz, T. P., Boney, J. W., Shen, C., Jaczynski, J., and Moritz, J. S. (2019). The effect of standard pelleting and more thermally aggressive pelleting utilizing a hygieniser on feed manufacture and reduction of Enterococcus faecium, a Salmonella surrogate. J. Appl. Poult. Res. 28, 1226–1233. doi: 10.3382/japr/pfz088
Boney, J. W., Jaczynski, J., Weidhaas, J. L., Bergeron, A. N., and Moritz, J. S. (2018). The effects of steam conditioning and antimicrobial inclusion on feed manufacturing and inactivation of Enterococcus faecium, a Salmonella surrogate. J. Appl. Poult. Res. 27, 472–482. doi: 10.3382/japr/pfy052
Briñez, W. J., Roig-Sagués, A. X., Hernández Herrero, M. M., López-Pedemonte, T., and Guamis, B. (2006). Bactericidal efficacy of peracetic acid in combination with hydrogen peroxide against pathogenic and non pathogenic strains of Staphylococcus spp., Listeria spp. and Escherichia coli. Food Control 17, 516–521. doi: 10.1016/j.foodcont.2005.02.014
CDC (2006). Salmonella Typhimurium Infections Linked to Tomatoes - Salmonella. Centers for Disease Control and Prevention. Available online at: https://www.cdc.gov/salmonella/2006/tomatoes-11-2006.html (accessed December 11, 2019).
CDC (2019). Multistate Outbreak of Salmonella Poona Infections Linked to Imported Cucumbers (Final Update)|Multistate Outbreak of Salmonella Poona Infections Linked to Imported Cucumbers|September 2015|Salmonella|CDC. Centers for Disease Control and Prevention. Available online at: https://www.cdc.gov/salmonella/poona-09-15/index.html (accessed December 11, 2019).
Ceylan, E., and Bautista, D. A. (2015). Evaluating Pediococcus acidilactici and Enterococcus faecium NRRL B-2354 as thermal surrogate microorganisms for Salmonella for in-plant validation studies of low-moisture pet food products. J. Food Prot. 78, 934–939. doi: 10.4315/0362-028X.JFP-14-271
Chaidez, C., Lopez, J., Vidales, J., and Campo, N. C.-D. (2007). Efficacy of chlorinated and ozonated water in reducing Salmonella typhimurium attached to tomato surfaces. Int J Environ Health Res. 17, 311–318. doi: 10.1080/09603120701417063
Colombe, S., Jernberg, C., Löf, E., Angervall, A. L., Mellström-Dahlgren, H., Dotevall, L., et al. (2019). Outbreak of unusual H2S-negative monophasic Salmonella typhimurium strain likely associated with small tomatoes, Sweden, august to october 2019. Euro Surveill. 24:1900643. doi: 10.2807/1560-7917.ES.2019.24.47.1900643
Dewey-Mattia, D., Manikonda, K., Hall, A. J., Wise, M. E., and Crowe, S. J. (2018). Surveillance for Foodborne Disease Outbreaks — United States 2009–2015. Surveill. Summ. 67, 1–11. doi: 10.15585/mmwr.ss6710a1
EFSA and ECDC (2018). EFSA (European Food Safety Authority) and ECDC(European Centre for Disease Prevention and Control). (2018). Multi-Country Outbreak of Salmonella Agona Infections Possibly Linked to Ready-to-Eat Food. EFSA supporting publication.
Gombas, D., Luo, Y., Brennan, J., Shergill, G., Petran, R., Walsh, R., et al. (2017). Guidelines to validate control of cross-contamination during washing of fresh-cut leafy vegetables. J. Food Prot. 80, 312–330. doi: 10.4315/0362-028X.JFP-16-258
Honjoh, K., Iwaizako, Y., Lin, Y., Kijima, N., and Miyamoto, T. (2016). Possibilities for contamination of tomato fruit by Listeria monocytogenes during cultivation. Food Sci. Technol. Res. 22, 349–357. doi: 10.3136/fstr.22.349
Hossein, J., Mohammadjavad, P., Chung, Y. L., and Won, F. W. (2013). Prevalence of Listeria species and Listeria monocytogenes serotypes in ready mayonnaise salads and salad vegetables in Iran. Afr. J. Microbiol. Res. 7, 1903–1906. doi: 10.5897/AJMR2013.5658
Hu, M., and Gurtler, J. B. (2017). Selection of surrogate bacteria for use in food safety challenge studies: a review. J. Food Prot. 80, 1506–1536. doi: 10.4315/0362-028X.JFP-16-536
Jeong, S., Marks, B. P., and Ryser, E. T. (2011). Quantifying the performance of Pediococcus sp. (NRRL B-2354: Enterococcus faecium) as a nonpathogenic surrogate for Salmonella enteritidis PT30 during moist-air convection heating of almonds. J. Food Prot. 74, 603–609. doi: 10.4315/0362-028X.JFP-10-416
Laury, A. M., Alvarado, M. V., Nace, G., Alvarado, C. Z., Brooks, J. C., Echeverry, A., et al. (2009). Validation of a lactic acid– and citric acid–based antimicrobial product for the reduction of Escherichia coli O157:H7 and Salmonella on beef tips and whole chicken carcasses. J. Food Prot. 72, 2208–2211. doi: 10.4315/0362-028X-72.10.2208
Lemonakis, L., Li, K., Adler, J. M., and Shen, C. (2017). Microbiological quality assessment and validation of antimicrobials against unstressed or cold-stress adapted Salmonella and surrogate Enterococcus faecium on broiler carcasses and wings. Poult. Sci. 96, 4038–4045. doi: 10.3382/ps/pex195
Li, K., Khouryieh, H., Jones, L., Etienne, X., and Shen, C. (2018). Assessing farmers market produce vendors' handling of containers and evaluation of the survival of Salmonella and Listeria monocytogenes on plastic, pressed-card, and wood container surfaces at refrigerated and room temperature. Food Control 94, 116–122. doi: 10.1016/j.foodcont.2018.06.036
Li, K., Weidhaas, J., Lemonakis, L., Khouryieh, H., Stone, M., Jones, L., et al. (2017). Microbiological quality and safety of fresh produce in West Virginia and Kentucky farmers' markets and validation of a post-harvest washing practice with antimicrobials to inactivate Salmonella and Listeria monocytogenes. Food Control 79, 101–108. doi: 10.1016/j.foodcont.2017.03.031
López-Gálvez, F., Posada-Izquierdo, G. D., Selma, M. V., Pérez-Rodríguez, F., Gobet, J., Gil, M. I., et al. (2012). Electrochemical disinfection: an efficient treatment to inactivate Escherichia coli O157:H7 in process wash water containing organic matter. Food Microbiol. 30, 146–156. doi: 10.1016/j.fm.2011.09.010
Luo, Y., Nou, X., Millner, P., Zhou, B., Shen, C., Yang, Y., et al. (2012). A pilot plant scale evaluation of a new process aid for enhancing chlorine efficacy against pathogen survival and cross-contamination during produce wash. Int. J. Food Microbiol. 158, 133–139. doi: 10.1016/j.ijfoodmicro.2012.07.008
Meldrum, R. J., Little, C. L., Sagoo, S., Mithani, V., McLauchlin, J., and de Pinna, E. (2009). Assessment of the microbiological safety of salad vegetables and sauces from kebab take-away restaurants in the United Kingdom. Food Microbiol. 26, 573–577. doi: 10.1016/j.fm.2009.03.013
Minnesota Department of Health (2015). UPDATE: Tomatoes Identified as Source of Salmonella Outbreak in Restaurant Chain. Available online at: https://www.health.state.mn.us/news/pressrel/2015/salmonella091615.html (accessed January 20, 2020).
Painter, J. A., Hoekstra, R. M., Ayers, T., Tauxe, R. V., Braden, C. R., Angulo, F. J., et al. (2013). Attribution of foodborne illnesses, hospitalizations, and deaths to food commodities by using outbreak data, United States, 1998–2008. Emerg. Infect. Dis. 19, 407–415. doi: 10.3201/eid1903.111866
Ponniah, J., Robin, T. D., Paie, M. S., Chai, L. C., Radu, S., Ghazali, F. M., et al. (2012). Assessment of listeria monocytogenes in salad vegetables through kitchen simulation study. J. Trop. Agric. Food Sci. 40, 55–62.
Scheinberg, J., Dudley, E., Campbell, J., Roberts, B., DiMarzio, M., DebRoy, C., et al. (2017). Prevalence and phylogenetic characterization of Escherichia coli and hygiene indicator bacteria isolated from leafy green produce, beef, and pork obtained from farmers' markets in Pennsylvania. J. Food Prot. 80, 237–244. doi: 10.4315/0362-028X.JFP-16-282
Shen, C., Luo, Y., Nou, X., Wang, Q., and Millner, P. (2013). Dynamic effects of free chlorine concentration, organic load, and exposure time on the inactivation of Salmonella, Escherichia coli O157:H7, and Non-O157 shiga toxin–producing E. coli. J. Food Prot. 76, 386–393. doi: 10.4315/0362-028X.JFP-12-320
Shen, C., Norris, P., Williams, O., Hagan, S., and Li, K. (2016). Generation of chlorine by-products in simulated wash water. Food Chem. 190, 97–102. doi: 10.1016/j.foodchem.2015.04.146
Sreedharan, A., Li, Y., De, J., Gutierrez, A., Silverberg, R., and Schneider, K. R. (2017). Determination of optimum sanitizer levels for prevention of Salmonella cross-contamination of mature round tomatoes in a laboratory model flume system. J. Food Protect. 80, 1436–1442. doi: 10.4315/0362-028X.JFP-17-032
Strawn, L. K., Gröhn, Y. T., Warchocki, S., Worobo, R. W., Bihn, E. A., and Wiedmann, M. (2013). Risk factors associated with Salmonella and Listeria monocytogenes contamination of Produce fields. Appl. Environ. Microbiol. 79, 7618–7627. doi: 10.1128/AEM.02831-13
Strohbehn, C., Mendonca, A., Wilson, L., Domoto, P., and Smith, M. (2013). On-farm Food Safety: Cleaning and Sanitizing Guide. Human Sciences Extension and Outreach Publications. Available online at: https://lib.dr.iastate.edu/extension_families_pubs/102 (accessed December 9, 2019).
United Fresh Produce Association (2018). Guidance on Environmental Monitoring and Control of Listeria for the Fresh Produce Industry. Available online at: https://www.unitedfresh.org/content/uploads/2019/03/FINAL-UFPA-Listeria-Guidance.pdf (accessed December 9, 2019).
United States Food Drug Administration (1998). Guidance for Industry: Guide to Minimize Microbial Food Safety Hazards for Fresh Fruits and Vegetables. Available online at: https://www.fda.gov/regulatory-information/search-fda-guidance-documents/guidance-industry-guide-minimize-microbial-food-safety-hazards-fresh-fruits-and-vegetables (accessed January, 24, 2020).
United States Food Drug Administration (2018). Program Information Manual: Retail Food Protection Storage and Handling of Tomatoes. Available online at: https://www.fda.gov/food/retail-food-industryregulatory-assistance-training/program-information-manual-retail-food-protection-storage-and-handling-tomatoes (accessed January 24, 2020).
USDA (2015). Trends in U.S. Local and Regional Food Systems: A Report to Congress. Available online at: https://www.ers.usda.gov/webdocs/publications/42805/51173_ap068.pdf (accessed December, 9, 2019).
USDAERS (2019). Food Availability (Per Capita) Data System. Available online at: https://www.ers.usda.gov/data-products/food-availability-per-capita-data-system/ (accessed December, 9, 2019).
Vasseur, C., Beverel, L., Hebraud, M., and Labadie, J. (1999). Effect of osmotic, alkaline, acid, or thermal stresses on the growth and inhibition of Listeria monocytogenes. J. Appl. Microbiol. 86, 469–476. doi: 10.1046/j.1365-2672.1999.00686.x
Wang, H., and Ryser, E. (2014). Efficacy of various sanitizers against Salmonella during simulated commercial packing of tomatoes. J. Food Prot. 77, 1868–1875. doi: 10.4315/0362-028X.JFP-14-213
Wei, C. I., Huang, T. S., Kim, J. M., Lin, W. F., Tamplin, M. L., Bartz, J. A., et al. (1995). Growth and survival of Salmonella montevideo on tomatoes and disinfection with chlorinated water. J. Food Prot. 58, 829–836. doi: 10.4315/0362-028X-58.8.829
White, G. C. (2010). White's Handbook of Chlorination and Alternative Disinfectants, 5th Edn. Hoboken, NJ: Black and Veach Corporation. John Wiley and Sons, Inc.
Keywords: post-harvest wash, triple-wash, antimicrobials, pathogens, surrogate, cucumbers, tomatoes
Citation: Li K, Chiu Y-C, Jiang W, Jones L, Etienne X and Shen C (2020) Comparing the Efficacy of Two Triple-Wash Procedures With Sodium Hypochlorite, a Lactic–Citric Acid Blend, and a Mix of Peroxyacetic Acid and Hydrogen Peroxide to Inactivate Salmonella, Listeria monocytogenes, and Surrogate Enterococcus faecium on Cucumbers and Tomatoes. Front. Sustain. Food Syst. 4:19. doi: 10.3389/fsufs.2020.00019
Received: 12 December 2019; Accepted: 17 February 2020;
Published: 25 March 2020.
Edited by:
Joshua B. Gurtler, Agricultural Research Service, United StatesReviewed by:
Keith Warriner, University of Guelph, CanadaJitendra Patel, Beltsville Agricultural Research Center, United States
Copyright © 2020 Li, Chiu, Jiang, Jones, Etienne and Shen. This is an open-access article distributed under the terms of the Creative Commons Attribution License (CC BY). The use, distribution or reproduction in other forums is permitted, provided the original author(s) and the copyright owner(s) are credited and that the original publication in this journal is cited, in accordance with accepted academic practice. No use, distribution or reproduction is permitted which does not comply with these terms.
*Correspondence: Cangliang Shen, cangliang.shen@mail.wvu.edu
†These authors have contributed equally to this work