- 1Centre for Tropical Environmental Sustainability Science, James Cook University, Cairns, QLD, Australia
- 2Tree Fruit Research and Extension Center, Washington State University, Wenatchee, WA, United States
Entomopathogenic fungi are often relied on as important components of integrated pest management in tropical agriculture, either as biopesticides or as naturally occurring microbes conserved in the environment. Tropical abiotic conditions are often well-suited for microbial growth, and tropical habitats can be teeming with microbial biodiversity. However, competitive interactions with other fungi and the need to overcome defenses of hosts adapted to high fungal loads may inhibit the ability of insect pathogens to control tropical pests. Here, we review the current literature on Beauveria bassiana and Metarhizium anisopliae inhabiting tropical environments and their potential use as biological control agents. In some cases there is not a clear distinction between temperate and tropical agroecosystems, such as in the level of organic matter or soil texture in agricultural soils. Therefore, the effects of these soil characteristics in temperate agroecosystems are likely applicable to tropical systems as well. In contrast, factors such as microbial biodiversity and seasonal fluctuation in environmental conditions can differ dramatically between temperate and tropical systems. Therefore, we discuss literature that can be generalized to tropical systems. Where temperate and tropical systems are likely to differ we synthesize the literature specifically for tropical agroecosystems. We also provide hypotheses to stimulate future work on latitudinal gradients and the relative importance of biotic and abiotic factors in governing entomopathogen prevalence and community composition. These hypotheses provide a path forward to developing theory guiding the conservation and augmentation of entomopathogenic fungi to prevent pest outbreaks.
Introduction
Growing insecticide resistance and impacts on human and environmental health have encouraged entomopathogenic fungi (EPF) use for biocontrol (Inglis et al., 2001). Tropical environments support impressive microbial biodiversity (Thompson et al., 2017), including many parasites of arthropods (Mahe et al., 2017). However, by far the most common commercially available EPF in tropical and subtropical agroecosystems belong to two genera: Beauveria and Metarhizium (Ascomycota: Hypocreales) (Faria and Wraight, 2007; Li et al., 2010; Kumar et al., 2018; Mascarin et al., 2019). Here, we review literature focused on controlling arthropod pests with entomopathogens, with particular emphasis on these two genera.
Metarhizium and Beauveria have pan-global distributions revealing significant genetic diversification, with a wide insect host range and vast ecological niches (Driver et al., 2000; Rehner and Buckley, 2005; Zimmermann, 2007). Thus, many aspects of using these EPF to control pests span latitudinal gradients, and we discuss these generally. Environmental conditions and species compositions, however, can differ greatly between tropical and temperate regions, so we discuss these factors with specific reference to tropical studies and use general theory resulting from model systems to bridge gaps in the current literature and stimulate further studies.
EPF are generally formulated as biopesticides and applied in response to outbreaks. However, if the habitat is well-suited for the particular fungal strain to the environment it may be possible for fungal applications to serve as inoculative releases, where the EPF remain in the soil and prevent insect outbreaks. Here, we describe entomopathogenic fungal niche preferences to help inform EPF use to prevent pest outbreaks. We focus on two species Beauveria bassiana and Metarhizium anisopliae, but each most likely represents a broader range of species and strains that were previously grouped together. Therefore, the majority of cited papers regarding these fungal species refer to Beauveria bassiana and Metarhizium anisopliae sensu lato, due to recent taxonomic revisions (e.g., Driver et al., 2000; Bidochka et al., 2001; Inglis et al., 2019).
Beauveria and Metarhizium
Entomopathogenic fungal species, B. bassiana and M. anisopliae control a wide range of pests (Kassa et al., 2004; Castrillo et al., 2010; Migiro et al., 2010; Singha et al., 2010; Skinner et al., 2012; Akmal et al., 2013; Wraight et al., 2016). These fungi can also inhabit the leaf surface of variety of plant species and environments (Meyling and Eilenberg, 2006a; Garrido-Jurado et al., 2015), inhabit soil as saprophytes (Evans, 1982), or grow endophytically (Greenfield et al., 2016). The apparently wide distribution and diversity of pathogen hosts attacked by these fungi, and persistence in the environment when hosts are rare suggest potential value in integrated pest management programs across diverse locations and conditions (Lacey et al., 2015).
Fungal propagule persistence and efficacy on plants is directly influenced by temperature, humidity, sunlight, and microbial activity on the phylloplane (Jaronski, 2010). Much of our knowledge comes from in vitro studies (Fargues et al., 1997; Luz and Fargues, 1997; Fargues and Luz, 2000; Devi et al., 2005; Shin et al., 2017). However, it is unclear whether in vitro data be extrapolated to field conditions (Keyser et al., 2017), due to environmental influence on processes such as infection potential, conidial persistence and complex abiotic and biotic interactions that are rarely duplicated in laboratory environments (Inglis et al., 2001; Lacey et al., 2015).
Metarhizium and Beauveria readily colonize plant rhizospheres, forming endophytic associations (Vega et al., 2009; Behie et al., 2015). Beauveria species associate with several tropical or subtropical plant species, including cocoa (Posada and Vega, 2005), banana (Akello et al., 2008), and coffee (Vega, 2008). Post-endophytic colonization, Metarhizium robertsii can even transfer insect-derived nitrogen to plants (Behie et al., 2012). Beauveria and Metarhizium's plant host affiliations in agriculture and ecosystem services (i.e., beneficial symbiosis in plants and control of insect pest populations) make them promising candidates for their application as biopesticides in tropical agriculture. The use of EPF as biopesticides in a variety of agroecosystems provides exciting and sustainable farm management opportunities, but in depth knowledge of endemic fungal species is crucial to identifying scenarios and environments when the insect pathogen will be most effective (Meyling and Eilenberg, 2007; Meyling et al., 2009; Perez-Gonzalez et al., 2014).
Life in the Soil
Soil can act as a reservoir for fungal inoculates (Castrillo et al., 2010), dispersing above-ground by wind, rain-splash and insect activity, or via infection of soil-dwelling insects and radial hyphal growth (Meyling and Eilenberg, 2007). Hypocrealean fungi efficacy and persistence is influenced by soil type, moisture levels, and microbial interactions (Inglis et al., 2001). While tropical soils may contain very high organic matter and microbial diversity, the agricultural levels for each depend primarily on farm management practices (Moeskops et al., 2010; Bai et al., 2018), and texture varies widely amongst tropical soils without clear distinctions from temperate systems (Pulla et al., 2016). Therefore, temperate studies describing soil physical characteristics effects on EPF persistence and efficacy are likely directly applicable to tropical systems.
Soils high in organic matter often teem with microbes, potentially allowing antagonistic interactions between microbes (Inglis et al., 1998; Pal and Gardener, 2006). In temperate studies, antagonistic effects of increased microbial activity in the soil contributed to the inhibition of B. bassiana (Studdert and Kaya, 1990; Kessler et al., 2003; Quesada-Moraga et al., 2007), B. brongniartii (Kessler et al., 2004), and M. anisopliae (Jabbour and Barbercheck, 2009). For example, high soil moisture content promoted occurrence of antagonistic organisms, suggesting soil moisture could either directly or indirectly reduce conidia survival (Lingg and Donaldson, 1981; Jabbour and Barbercheck, 2009). However, this has been refuted by other studies in temperate regions finding little or no relationship between soil moisture and EPF occurrence, potentially due to limited variation in sampled soil moisture levels or oxygen deficiency (Griffin, 1963; Ali-Shtayeh et al., 2003; Kessler et al., 2003).
Soil oxygen levels during infection can promote mycelial growth, thermal tolerance, germination, and virulence against insects (Garza-López et al., 2012; Miranda-Hernández et al., 2014; Garcia-Ortiz et al., 2015; García-Ortiz et al., 2018; Oliveira and Rangel, 2018). In vitro studies reveal a positive correlation between enriched oxygen concentrations (26 and 30% O2) and conidial quality when compared to normal atmospheric oxygen levels (21% O2) (Miranda-Hernández et al., 2014; Garcia-Ortiz et al., 2015; García-Ortiz et al., 2018). Similarly, at deprived oxygen levels, the same contrast in conidia growth and virulence applies, reducing under ambient oxygen concentrations (Garza-López et al., 2012; Oliveira and Rangel, 2018). Germination under hypoxic conditions is lower than at normal atmospheric levels (Garza-López et al., 2012), increasing as oxygen conditions become enriched (Miranda-Hernández et al., 2014). Thus, aerating soil during mycelial growth may facilitate optimal entomopathogenic fungal development and pest control.
Soil texture influences fungal propagule transmission and retention. Increasing clay content can promote entomopathogenic fungal persistence, likely due to smaller pore size and/or the adsorption of conidia to clay and organic particles (Ignoffo et al., 1977; Storey and Gardner, 1988; Quesada-Moraga et al., 2007). However, high clay content can also inhibit the ability of a potential host to encounter another, due to reduced porosity for conidial penetration to deeper soil layers and potential transmission (Vänninen et al., 2000; Fuxa and Richter, 2004). Therefore, mechanical filtration of the soil structure can be a major determinant of entomopathogenic fungal persistence and effectiveness when applied to soil (Storey and Gardner, 1988). For example, the efficacy of B. bassiana against the red fire ants Solenopsis invicta improved when applied to wetter soils, promoting conidia transmission and infection rates (Fuxa and Richter, 2004).
Niche Preference
The Habitat Selection Hypothesis
Biotic interactions may alter tropical EPF persistence (Jaronski, 2010), particularly The habitat selection hypothesis for Metarhizium species suggests this is a key difference between temperate and tropical regions (Bidochka et al., 2002). Bidochka and Small (2005) suggested Metarhizium genotypes are associated with habitat types in temperate and polar regions and are more likely associated with certain host insects in (sub)tropical regions. The authors also suggested M. anisopliae originated in Southeast Asia but now comprises an assemblage of cryptic species, many of which traverse large geographical barriers. Temperate studies regarding Beauveria and Metarhizium have highlighted their preference for habitat selection over associations with insect hosts (Meyling and Eilenberg, 2006b; Meyling et al., 2009; Ormond et al., 2010). Any insect host associations of M. anisopliae at higher latitudes were attributed to the insect's habitat, suggesting that abiotic factors could potentially be driving the population genetic structure (Bidochka et al., 2001).
Takatsuka (2007) characterized Beauveria isolates from Japan using ISSR-PCR and found no evidence for long-term coevolution between the fungus and insect hosts, supporting the Bidochka et al. (2002) hypothesis that variation in persistence of the free-living, saprophytic stage of a facultative insect pathogen drives population genetic structure. In contrast, Bridge et al. (1997) suggested coevolution between tropical M. flavoviride var. minus isolates of a single genotype and those insects belonging to the superfamily Acridoidea. Tropical isolates of M. flavoviride var. minus with host-preference traits differed from those with a European derivation. Interestingly, the majority of isolates in the publications supporting the Bidochka and Small (2005) hypothesis regarding the association of Metarhizium spp. with insect host species have a tropical origin (Rombach et al., 1986; St. Leger et al., 1992; Bridge et al., 1993, 1997; Leal et al., 1994; Tigano-Milani et al., 1995). However, future analyses are needed to define these relationships.
Metarhizium strains have adapted to particular environments, supporting versatile life-history strategies (Lovett and St. Leger, 2015). Adjustments in environmental stress responses can arise from adaptation to environmental abiotic (e.g., temperature, UV radiation, and humidity) and biotic factors relating to infection of a host (e.g., antimicrobial and behavioral stressors) (Lovett and St. Leger, 2015; Ortiz-Urquiza and Keyhani, 2015). Conidia produced under abiotic and biotic stress can withstand a broader environmental range, and improve virulence against insects (Li et al., 2015; Rangel et al., 2015). For example, overcoming acridid host behavioral fevers during infection can produce more thermotolerant entomopathogenic fungal isolates (Fargues et al., 1997; Blanford and Thomas, 2000; Rangel et al., 2005). Behavioral defensive traits in grasshoppers can result in discrepancies between entomopathogenic fungal species and their effectiveness in controlling pest populations at different temperatures (Inglis et al., 1999). Grasshopper nymphs infected with B. bassiana and M. acridum experienced reduced levels of mortality when temperature was increased, and M. acridum substantially outcompeted B. bassiana in nymphal mortality at higher temperatures (Inglis et al., 1999).
From the evidence primarily presented for Metarhizium we propose two general hypotheses for EPF: (i) Abiotic factors are primary determinants of population genetic structure at higher latitudes, due to the insect pathogen's requirement to adapt seasonality and extreme environmental conditions. (ii) Conversely, biotic factors (interactions with other species and fungal-host associated infection pathways) are the primary regulators of EPF population genetic structure in lower latitudes. We evaluate these hypotheses in light of recent research below. To visually present our hypotheses, we constructed a conceptual model (Figure 1). This is meant to qualitatively describe our hypotheses to stimulate future research, rather than stand alone as a mathematical model.
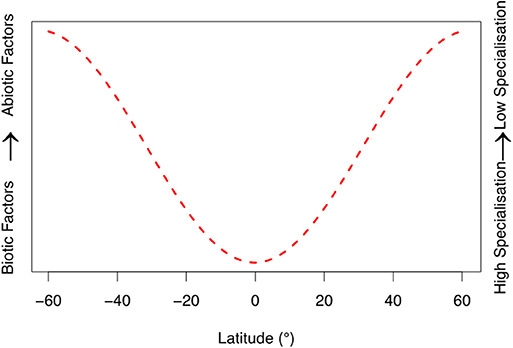
Figure 1. Qualitative conceptual model based of our reviews developed hypotheses. Specificity of fungal entomopathogenic communities' changes with latitude, corresponding to biotic and abiotic factors. Biotic factors are related to interactions with the host and other microbes (i.e., coevolutionary arms races and infection pathways). Abiotic factors include environmental variables such as temperature, humidity, UV radiation, and oxidative and osmotic stressors. The latitudinal range was capped at 60°N and 60°S, as this figure does not account for the climatic extremes beyond these thresholds on fungal communities. We hypothesize that at lower latitudes entomopathogenic fungi inhabit more ideal environmental conditions, despite increased pressure from other fungal species, and greater host defense (see “Abiotic conditions and adaptation” and “Biotic interactions and adaptation” sections of main text for more information). This corresponds to an increase in specialization of entomopathogenic fungi.
Abiotic Conditions and Adaptation
Fungi inhabiting higher latitudes experience a wider range of temperatures due to seasonality (Wielgolaski and Inouye, 2003). Thus, abiotic stressors (particularly temperature) at higher latitudes may predominantly drive population genetics and adaptability of EPF. In temperate regions, EPF must adapt to a broad range and greater levels of climatic intensities (Maggi et al., 2013; Wang et al., 2017), whereby abiotic factors primarily influence generalist pathogen's survival (Bidochka et al., 2001; Lennon et al., 2012). In contrast, we hypothesize that low latitude biotic factors such as species richness and pathogen-insect associations that drive coevolutionary arms races predominately influence EPF life history. Phylogenetic B. bassiana cluster by habitat type more at seasonally variable high latitudes (Ormond et al., 2010), although one study found no seasonal effect in regions of sub-tropical climates (Garrido-Jurado et al., 2015). Phylogenetically structured investigations suggest B. bassiana adapts gene regulation to environmental conditions, with habitat adaptation driving population dynamics (Bidochka et al., 2002; Xiao et al., 2012). Thus, differences in the magnitude of seasonal environmental conditions at different latitudes may contribute to the observed temporal dissimilarities in B. bassiana population dynamics between the studies.
Environmental conditions near entomopathogenic fungal survival limits can drive local adaptation when these limits are regularly experienced (Doberski, 1981; Vidal et al., 1997). The optimal temperature for growth and virulence against insect hosts of Metarhizium and Beauveria species is generally between 25 and 30°C (Luz and Fargues, 1997; Ekesi et al., 1999; Devi et al., 2005; Bugeme et al., 2009). However, significant variation exists in a fungal pathogen species' thermal preference and their effects on potential hosts, due to the environment in which the pathogens evolved (Fargues et al., 1997; Bugeme et al., 2009; Alali et al., 2019), and individual strains can differ in their thermal optima (Doberski, 1981; Thomas and Jenkins, 1997; Alali et al., 2019). M. acridum isolates obtained from a hot environment exhibited greater performance at higher temperatures than those derived from a much cooler climate (Thomas and Jenkins, 1997). Similarly, sub-tropical B. bassiana strains collected from hotter areas of Syria demonstrated greater thermotolerant ability than the outlier collected from a site experiencing lower temperatures (Alali et al., 2019). Regarding virulence against insects, temperate isolates of B. bassiana were significantly more effective against the elm bark beetle (Scolytus scolytus F.) at low temperatures (2 to 6°C) than isolates of M. anisopliae originating from tropical and sub-tropical latitudes (Doberski, 1981), although it is impossible to separate fungal species differences from differences arising from the geographical sources of the two fungal strains. B. bassiana and M. anisopliae are also sensitive to ultraviolet radiation, prompting UV protectant use in oil-based field sprays (Inglis et al., 1995; Shin et al., 2017; Kumar et al., 2018). UV tolerance often varies among isolates from different latitudes (Braga et al., 2001; Fernandes et al., 2008), and habitat types (Bidochka et al., 2001). Isolates of B. bassiana and M. anisopliae closer to the equator exhibit higher UV tolerance, and cold-adapted populations from higher latitudes generally experience optimal conditions in colder temperatures (Fernandes et al., 2008). In Canada Metarhizium isolates encountered in forested habitats are less tolerant to UV radiation and are more cold-active compared to agricultural landscapes (Bidochka et al., 2001). Thus, abiotic selection at high latitudes (e.g., UV exposure in a given habitat type) for specific genetic groups of fungal entomopathogens could influence their effectiveness in agriculture, particularly if isolates are sourced from forested or hedgerow habitats.
Biotic Interactions and Adaptation
Tropical forests support high entomopathogenic mycotaxa diversity, where the teleomorphs (sexual stages) of hypocrealean fungi are mostly found and are often more specialized in their host range than asexual morphotypes (Evans, 1982; Vega et al., 2012; Hu et al., 2014). In contrast, asexually developing EPF (anamorphs) inhabit both tropical and temperate climates (Vega et al., 2012). Lab studies in model systems that increased biodiversity observed corresponding intensification of evolutionary arms races between hosts and parasites (Betts et al., 2018). Similarly, genetic diversity and host specificity of some fungal species suggests that host insects can exert strong selective pressures on pathogens through a cascade of defense and counter-defense mechanisms (Maurer et al., 1997; Evans et al., 2011; Mukherjee and Vilcinskas, 2018). For example, Metarhizium often evolved from specialist to generalist insect pathogens; an expansion in host range coinciding with fungal occupation of an expanding latitudinal range (Bidochka and Small, 2005; Hu et al., 2014). However, in high species-density rainforest areas, high fungal tropical diversity may experience stronger pressure from hosts and competitors that can favor the occurrence of pathogens such as the teleomorph genus Cordyceps (Evans, 1982; Sung et al., 2007; Aung et al., 2008), and specialist fungal entomopathogens of the genus Ophiocordyceps (Aung et al., 2008; Evans et al., 2011; Araújo et al., 2015). Phylogenomic analyses suggest Beauveria spp. is the asexual life stage of the Cordyceps lineage (Xiao et al., 2012). Despite Beauveria having direct genetic links to Cordyceps, generalist Beauveria and Metarhizium are less common within tropical rainforest habitats, and are more frequently encountered in agriculture (Rehner, 2005; Aung et al., 2008). The contrast in life-histories between these specialists and generalist fungi could be attributed to the loss of the repeat-induced point mutations in B. bassiana and Metarhizium spp. (infers the sexual cycle to be rare in both fungi), which was a prerequisite of these fungal pathogens for the expansion of gene families (Xiao et al., 2012; Lovett and St. Leger, 2017).
Given the probable southeast Asian origin of Metarhizium (the continent with the highest genotypic diversity) (Bidochka and Small, 2005; Lovett and St. Leger, 2017), and the subsequent evolutionary changes in specificity (Hu et al., 2014), a geographical range expansion to temperate regions may have corresponded to a more generalist host range. We hypothesize that this could be due in part to lower host species richness in temperate regions (Thompson et al., 2017), and a need to adapt to greater variation in climatic conditions focusing adaptation on abiotic conditions. A remaining question is how greater potential host diversity in tropical environments alters these selection pressures. Future research into how insect-pathogen arms races alter community composition with changes in latitude would improve management of entomopathogens in different latitudes. Additionally, empirical evaluations of fungi collected at different latitudes and laboratory experiments will improve our knowledge of endemic fungal species and their relevance within a particular system, alongside their appropriate use in biocontrol regimes.
Improving Fungal Persistence and Insect Outbreak Prevention
The ability of some fungal species to cross large geographical barriers (i.e., cosmopolitan in nature) does not imply that the application of fungal pathogens as an agricultural biopesticide will ensure fungal persistence. Rather, researching the appropriateness of a fungal pathogen specific to the target environment is required, including interactive effects of individual biotic/abiotic factors. Efforts should be directed toward focusing on endemic fungal communities and applied within its derived system. Regional differences between suitable fungal application type, host range (i.e., generalist vs. specialist) and the dominant environmental factors (biotic/abiotic) on pathogen performance can better predict the long-term success of entomopathogenic biocontrol and help prevent insect outbreaks.
Author Contributions
AM wrote the first draft. AM and TN contributed significantly to subsequent drafts.
Funding
AM was supported by a AusIndustry Innovations Connections grant to TN.
Conflict of Interest
The authors declare that the research was conducted in the absence of any commercial or financial relationships that could be construed as a potential conflict of interest.
References
Akello, J., Dubois, T., Coyne, D., and Kyamanywa, S. (2008). Effect of endophytic Beauveria bassiana on populations of the banana weevil, Cosmopolites sordidus, and their damage in tissue-cultured banana plants. Entomol. Exp. Appl. 129, 157–165. doi: 10.1111/j.1570-7458.2008.00759.x
Akmal, M., Freed, S., Malik, M. N., and Gul, H. T. (2013). Efficacy of Beauveria bassiana (Deuteromycotina: Hypomycetes) against different aphid species under laboratory conditions. Pak. J. Zool. 45, 71–78.
Alali, S., Mereghetti, V., Faoro, F., Bocchi, S., Al Azmeh, F., and Montagna, M. (2019). Thermotolerant isolates of Beauveria bassiana as potential control agent of insect pest in subtropical climates. PLoS ONE 14:e0211457. doi: 10.1371/journal.pone.0211457
Ali-Shtayeh, M. S., Mara'i, A.-B. B. M., and Jamous, R. M. (2003). Distribution, occurrence and characterization of entomopathogenic fungi in agricultural soil in the Palestinian area. Mycopathologia 156, 235–244. doi: 10.1023/A:1023339103522
Araújo, J. P. M., Evans, H. C., Geiser, D. M., Mackay, W. P., and Hughes, D. P. (2015). Unravelling the diversity behind the Ophiocordyceps unilateralis complex: three new species of zombie-ant fungi from the Brazilian Amazon. Zootaxa 22, 224–238. doi: 10.11646/phytotaxa.220.3.2
Aung, O. M., Soytong, K., and Hyde, K. (2008). Diversity of entomopathogenic fungi in rainforests of Chiang Mai Province, Thailand. Fungal Divers. 30, 15–22.
Bai, Z., Caspari, T., Gonzalez, M. R., Batjes, N. H., Mäder, P., Bünemann, E. K., et al. (2018). Effects of agricultural management practices on soil quality: a review of long-term experiments for Europe and China. Agric. Ecosyst. Environ. 265, 1–7. doi: 10.1016/j.agee.2018.05.028
Behie, S. W., Jones, S. J., and Bidochka, M. J. (2015). Plant tissue localization of the endophytic insect pathogenic fungi Metarhizium and Beauveria. Fungal Ecol. 13, 112–119. doi: 10.1016/j.funeco.2014.08.001
Behie, S. W., Zelisko, P., and Bidochka, M. J. (2012). Endophytic insect-parasitic fungi translocate nitrogen directly from insects to plants. Science 336, 1576–1577. doi: 10.1126/science.1222289
Betts, A., Gray, C., Zelek, M., MacLean, R. C., and King, K. C. (2018). High parasite diversity accelerates host adaptation and diversification. Science 360, 907–911. doi: 10.1126/science.aam9974
Bidochka, M. J., Kamp, A. M., Lavender, T. M., Dekoning, J., and De Croos, J. N. (2001). Habitat association in two genetic groups of the insect-pathogenic fungus Metarhizium anisopliae: uncovering cryptic species? Appl. Environ. Microbiol. 67, 1335–1342. doi: 10.1128/AEM.67.3.1335-1342.2001
Bidochka, M. J., Menzies, F. V., and Kamp, A. M. (2002). Genetic groups of the insect-pathogenic fungus Beauveria bassiana are associated with habitat and thermal growth preferences. Arch. Microbiol. 178, 531–537. doi: 10.1007/s00203-002-0490-7
Bidochka, M. J., and Small, C. L. (2005). “Phylogeography of Metarhizium, an insect pathogenic fungus,” in Insect-Fungal Associations: Ecology and Evolution, eds F. E. Vega and M. Blackwell (New York, NY: Oxford University Press), 75–118.
Blanford, S., and Thomas, M. B. (2000). Thermal behavior of two acridid species: effects of habitat and season on body temperature and the potential impact on biocontrol with pathogens. Environ. Entomol. 29, 1060–1069. doi: 10.1603/0046-225X-29.5.1060
Braga, G., Flint, S., Miller, C., Anderson, A., and Roberts, D. (2001). Variability in response to UV-B among species and strains of Metarhizium isolated from sites at latitudes from 61°N to 54°S. J. Invertebr. Pathol. 78, 98–108. doi: 10.1006/jipa.2001.5048
Bridge, P. D., Prior, C., Sagbohan, J., Lomer, C. J., Carey, M., and Buddie, A. (1997). Molecular characterization of isolates of Metarhizium from locusts and grasshoppers. Biodivers. Conserv. 6, 177–189. doi: 10.1023/A:1018387918686
Bridge, P. D., Williams, M. A. J., Prior, C., and Paterson, R. R. M. (1993). Morphological, biochemical and molecular characteristics of Metarhizium anisopliae and M. flavoviride. J. Gen. Microbiol 139, 1163–1169. doi: 10.1099/00221287-139-6-1163
Bugeme, D. M., Knapp, M., Boga, H. I., Wanjoya, A. K., and Maniania, N. K. (2009). Influence of temperature on virulence of fungal isolates of Metarhizium anisopliae and Beauveria bassiana to the two-spotted spider mite Tetranychus urticae. Mycopathologia 167, 221–227. doi: 10.1007/s11046-008-9164-6
Castrillo, L. A., Bauer, L. S., Liu, H., Griggs, M. H., and Vandenberg, J. D. (2010). Characterization of Beauveria bassiana (Ascomycota: Hypocreales) isolates associated with Agrilus planipennis (Coleoptera: Buprestidae) populations in Michigan. Biol. Control 54, 135–140. doi: 10.1016/j.biocontrol.2010.04.005
Devi, K. U., Sridevi, V., Mohan, C. M., and Padmavathi, J. (2005). Effect of high temperature and water stress on in vitro germination and growth in isolates of the entomopathogenic fungus Beauveria bassiana (Bals.) Vuillemin. J. Invertebr. Pathol. 88, 181–189. doi: 10.1016/j.jip.2005.02.001
Doberski, J. W. (1981). Comparative laboratory studies on three fungal pathogens of the elm bark beetle Scolytus scolytus: effect of temperature and humidity on infection by Beauveria bassiana, Metarhizium anisopliae, and Paecilomyces farinosus. J. Invertebr. Pathol. 37, 195–200. doi: 10.1016/0022-2011(81)90075-6
Driver, F., Milner, R. J., and Trueman, J. W. H. (2000). A taxonomic revision of Metarhizium based on a phylogenetic analysis of rDNA sequence data. Mycol. Res. 104, 134–150. doi: 10.1017/S0953756299001756
Ekesi, S., Maniania, N. K., and Ampong-Nyarko, K. (1999). Effect of temperature on germination, radial growth and virulence of Metarhizium anisopliae and Beauveria bassiana on Megalurothrips sjostedti. Biocontrol Sci. Technol. 9, 177–185. doi: 10.1080/09583159929767
Evans, H. C. (1982). Entomogenous fungi in tropical forest ecosystems: an appraisal. Ecol. Entomol. 7, 47–60. doi: 10.1111/j.1365-2311.1982.tb00643.x
Evans, H. C., Elliot, S. L., and Hughes, D. P. (2011). Hidden diversity behind the zombie-ant fungus Ophiocordyceps unilateralis: Four new species described from carpenter ants in Minas Gerais, Brazil. PLoS ONE 6:e17024. doi: 10.1371/journal.pone.0017024
Fargues, J., Goettel, M. S., Smits, N., Ouedraogo, A., and Rougier, M. (1997). Effect of temperature on vegetative growth of Beauveria bassiana isolates from different origins. Mycologia 89, 383–892. doi: 10.1080/00275514.1997.12026797
Fargues, J., and Luz, C. (2000). Effects of fluctuating moisture and temperature regimes on the infection potential of Beauveria bassiana for Rhodnius prolixus. J. Invertebr. Pathol. 75, 202–211. doi: 10.1006/jipa.1999.4923
Faria, M. R. D., and Wraight, S. P. (2007). Mycoinsecticides and mycoacaricides: a comprehensive list with worldwide coverage and international classification of formulation types. Biol. Control 43, 237–256. doi: 10.1016/j.biocontrol.2007.08.001
Fernandes, E. K., Rangel, D. E. N., Moraes, Á. M. L., Bittencourt, V. R. E. P., and Roberts, D. W. (2008). Cold activity of Beauveria and Metarhizium, and thermotolerance of Beauveria. J. Invertebr. Pathol. 98, 69–78. doi: 10.1016/j.jip.2007.10.011
Fuxa, J. R., and Richter, A. R. (2004). Effects of soil moisture and composition and fungal isolate on prevalence of Beauveria bassiana in laboratory colonies of the red imported fire ant (Hymenoptera: Formicidae). Environ. Entomol. 33, 975–981. doi: 10.1603/0046-225X-33.4.975
García-Ortiz, N., Figueroa-Martínez, F. J., Carrasco-Navarro, U., Favela-Torres, E., and Loera, O. (2018). The oxygen concentration in cultures modulates protein expression and enzymatic antioxidant responses in Metarhizium lepidiotae conidia. Fungal Biol. 122, 487–496. doi: 10.1016/j.funbio.2017.10.013
Garcia-Ortiz, N., Tlecuitl-Beristain, S., Favela-Torres, E., and Loera, O. (2015). Production and quality of conidia by Metarhizium anisopliae var. lepidiotum: critical oxygen level and period of mycelium competence. Appl. Microbiol. Biotechnol. 99, 2783–2791. doi: 10.1007/s00253-014-6225-2
Garrido-Jurado, I., Fernandez-Bravo, M., Campos, C., and Quesada-Moraga, E. (2015). Diversity of entomopathogenic Hypocreales in soil and phylloplanes of five Mediterranean cropping systems. J. Invertebr. Pathol. 130, 97–106. doi: 10.1016/j.jip.2015.06.001
Garza-López, P. M., Konigsberg, M., Gómez-Quiroz, L. E., and Loera, O. (2012). Physiological and antioxidant response by Beauveria bassiana Bals (Vuill.) to different oxygen concentrations. World J. Microbiol. Biotechnol. 28, 353–359. doi: 10.1007/s11274-011-0827-y
Greenfield, M., Gomez-Jimenez, M. I., Ortiz, V., Vega, F. E., Kramer, M., and Parsa, S. (2016). Beauveria bassiana and Metarhizium anisopliae endophytically colonize cassava roots following soil drench inoculation. Biol. Control 95, 40–48. doi: 10.1016/j.biocontrol.2016.01.002
Griffin, D. M. (1963). Soil moisture and the ecology of soil fungi. Biol. Rev. 38, 141–166. doi: 10.1111/j.1469-185X.1963.tb00781.x
Hu, X., Xiao, G., Zheng, P., Shang, Y., Su, Y., Zhang, X., et al. (2014). Trajectory and genomic determinants of fungal-pathogen speciation and host adaptation. Proc. Natl. Acad. Sci. U.S.A. 111, 16796–16801. doi: 10.1073/pnas.1412662111
Ignoffo, C. M., Garcia, C., Hostetter, D. L., and Pinnell, R. E. (1977). Vertical movement of conidia of Nomuraea rileyi through sand and loam soils. J. Econ. Entomol. 70, 163–164. doi: 10.1093/jee/70.2.163
Inglis, G., Goettel, M. S., Butt, T., and Strasser, H. (2001). “Use of hyphomycetous fungi for managing insect pests,” in Fungi as Biocontrol Agents: Progress, Problems and Potential, eds T. M. Butt, C. Jackson, and N. Magan (Oxfordshire: CABI Publishing), 23–69. doi: 10.1079/9780851993560.0023
Inglis, G. D., Duke, G. M., Goettel, M. S., Kabaluk, J. T., and Ortega-Polo, R. (2019). Biogeography and genotypic diversity of Metarhizium brunneum and Metarhizium robertsii in northwestern North American soils. Can. J. Microbiol. 65, 261–281. doi: 10.1139/cjm-2018-0297
Inglis, G. D., Duke, G. M., Kawchuk, L. M., and Goettel, M. S. (1999). Influence of oscillating temperatures on the competitive infection and colonization of the migratory grasshopper by Beauveria bassiana and Metarhizium flavoviride. Biol. control 14, 111–120. doi: 10.1006/bcon.1998.0666
Inglis, G. D., Goettel, M. S., and Johnson, D. L. (1995). Influence of ultraviolet light protectants on persistence of the entomopathogenic fungus, Beauveria bassiana. Biol. Control 5, 581–590. doi: 10.1006/bcon.1995.1069
Inglis, G. D., Johnson, D. L., Kawchuk, L. M., and Goettel, M. S. (1998). Effect of soil texture and soil sterilization on susceptibility of ovipositing grasshoppers to Beauveria bassiana. J. Invertebr. 71, 73–81. doi: 10.1006/jipa.1997.4698
Jabbour, R., and Barbercheck, M. E. (2009). Soil management effects on entomopathogenic fungi during the transition to organic agriculture in a feed grain rotation. Biol. Control 51, 435–443. doi: 10.1016/j.biocontrol.2009.08.004
Jaronski, S. T. (2010). Ecological factors in the inundative use of fungal entomopathogens. Biocontrol 55, 159–185. doi: 10.1007/s10526-009-9248-3
Kassa, A., Stephan, D., Vidal, S., and Zimmermann, G. (2004). Laboratory and field evaluation of different formulations of Metarhizium anisopliae var. acridum submerged spores and aerial conidia for the control of locusts and grasshoppers. Biocontrol 49, 63–81. doi: 10.1023/B:BICO.0000009384.46858.aa
Kessler, P., Enkerl, J., Schweize, C., and Keller, S. (2004). Survival of Beauveria brongniartii in the soil after application as a biocontrol agent against the European cockchafer Melolontha melolontha. Biocontrol 49, 563–581. doi: 10.1023/B:BICO.0000036441.40227.ed
Kessler, P., Matzke, H., and Keller, S. (2003). The effect of application time and soil factors on the occurrence of Beauveria brongniartii applied as a biological control agent in soil. J. Invertebr. Pathol. 84, 15–23. doi: 10.1016/j.jip.2003.08.003
Keyser, C. A., Fernandes, É. K. K., Rangel, D. E. N., Foster, R. N., Jech, L. E., Reuter, K. C., et al. (2017). Laboratory bioassays and field-cage trials of Metarhizium spp. isolates with field-collected Mormon crickets (Anabrus simplex). Biocontrol 62, 257–268. doi: 10.1007/s10526-016-9782-8
Kumar, K. K., Sridhar, J., Murali-Baskaran, R. K., Senthil-Nathan, S., Kaushal, P., Dara, S. K., et al. (2018). Microbial biopesticides for insect pest management in India: current status and future prospects. J. Invertebr. Pathol. 165, 74–81. doi: 10.1016/j.jip.2018.10.008
Lacey, L. A., Grzywacz, D., Shapiro-Ilan, D. I., Frutos, R., Brownbridge, M., and Goettel, M. S. (2015). Insect pathogens as biological control agents: back to the future. J. Invertebr. Pathol. 132, 1–41. doi: 10.1016/j.jip.2015.07.009
Leal, S. C. M., Bertioli, T. M., Butt, T. M., and Peberdy, J. F. (1994). Characterization of isolates of the entomopathogenic fungus Metarhizium anisopliae by RAPD-PCR. Mycol. Res. 98, 1077–1081. doi: 10.1016/S0953-7562(09)80436-X
Lennon, J. T., Aanderud, Z. T., Lehmkuhl, B. K., and Schoolmaster, D. R. Jr. (2012). Mapping the niche space of soil microorganisms using taxonomy and traits. Ecology 93, 1867–1879. doi: 10.1890/11-1745.1
Li, F., Shi, H.-Q., Ying, S.-H., and Feng, M.-G. (2015). Distinct contributions of one Fe- and two Cu/Zn-cofactored superoxide dismutases to antioxidation, UV tolerance and virulence of Beauveria bassiana. Fungal Genet. Biol. 81, 160–171. doi: 10.1016/j.fgb.2014.09.006
Li, Z. B., Alves, S., Roberts, D., Fan, M., Delalibera, I., Tang, J., et al. (2010). Biological control of insects in Brazil and China: history, current programs and reasons for their successes using entomopathogenic fungi. Biocontrol Sci. Technol. 20, 117–136. doi: 10.1080/09583150903431665
Lingg, A. J., and Donaldson, M. D. (1981). Biotic and abiotic factors affecting stability of Beauveria bassiana conidia in soil. J. Invertebr. Pathol. 38, 191–200. doi: 10.1016/0022-2011(81)90122-1
Lovett, B., and St. Leger, R. J. (2015). Stress is the rule rather than the exception for Metarhizium. Curr. Genet. 61, 253–261. doi: 10.1007/s00294-014-0447-9
Lovett, B., and St. Leger, R. J. (2017). The insect pathogens. Microbiol. Spectr. 5, 1–19. doi: 10.1128/microbiolspec.FUNK-0001-2016
Luz, C., and Fargues, J. (1997). Temperature and moisture requirements for conidial germination of an isolate of Beauveria bassiana, pathogenic to Rhodnius prolixus. Mycopathologia 138, 117–125. doi: 10.1023/A:1006803812504
Maggi, O., Tosi, S., Angelova, M., Lagostina, E., Fabbri, A. A., Pecoraro, L., et al. (2013). Adaptation of fungi, including yeasts, to cold environments. Plant Biosyst. 147, 247–258. doi: 10.1080/11263504.2012.753135
Mahe, F., de Vargas, C., Bass, D., Czech, L., Stamatakis, A., Lara, E., et al. (2017). Parasites dominate hyperdiverse soil protist communities in Neotropical rainforests. Nat. Ecol. Evol. 1:0091. doi: 10.1038/s41559-017-0091
Mascarin, G. M., Lopes, R. B., Delalibera, I., Fernandes, E. K. K., Luz, C., and Faria, M. (2019). Current status and perspectives of fungal entomopathogens used for microbial control of arthropod pests in Brazil. J. Invertebr. Pathol. 165, 46–53. doi: 10.1016/j.jip.2018.01.001
Maurer, P., Couteaudier, Y., Girard, P. A., Bridge, P. D., and Riba, G. (1997). Genetic diversity of Beauveria bassiana and relatedness to host insect range. Mycol. Res. 101, 159–164. doi: 10.1017/S0953756296002213
Meyling, N. V., and Eilenberg, J. (2006a). Isolation and characterisation of Beauveria bassiana isolates from phylloplanes of hedgerow vegetation. Mycol. Res. 110, 188–195. doi: 10.1016/j.mycres.2005.09.008
Meyling, N. V., and Eilenberg, J. (2006b). Occurrence and distribution of soil borne entomopathogenic fungi within a single organic agroecosystem. Agric. Ecosyst. Environ. 113, 336–341. doi: 10.1016/j.agee.2005.10.011
Meyling, N. V., and Eilenberg, J. (2007). Ecology of the entomopathogenic fungi Beauveria bassiana and Metarhizium anisopliae in temperate agroecosystems: potential for conservation biological control. Biol. Control 43, 145–155. doi: 10.1016/j.biocontrol.2007.07.007
Meyling, N. V., Lubeck, M., Buckley, E. P., Eilenberg, J., and Rehner, S. A. (2009). Community composition, host range and genetic structure of the fungal entomopathogen Beauveria in adjoining agricultural and seminatural habitats. Mol. Ecol. 18, 1282–1293. doi: 10.1111/j.1365-294X.2009.04095.x
Migiro, L. N., Maniania, N. K., Chabi-Olaye, A., and Vandenberg, J. (2010). Pathogenicity of entomopathogenic fungi Metarhizium anisopliae and Beauveria bassiana (Hypocreales: Clavicipitaceae) isolates to the adult pea leafminer (Diptera: Agromyzidae) and prospects of an autoinoculation device for infection in the field. Environ. Entomol. 39, 468–475. doi: 10.1603/EN09359
Miranda-Hernández, F., Saucedo-Castañeda, G., Alatorre-Rosas, R., and Loera, O. (2014). Oxygen-rich culture conditions enhance the conidial infectivity and the quality of two strains of Isaria fumosorosea for potentially improved biocontrol processes. Pest Manage. Sci. 70, 661–666. doi: 10.1002/ps.3605
Moeskops, B., Sukristiyonubowo Buchan, D., Sleutel, S., Herawaty, L., Husen, E., et al. (2010). Soil microbial communities and activities under intensive organic and conventional vegetable farming in West Java, Indonesia. Appl. Soil Ecol. 45, 112–120. doi: 10.1016/j.apsoil.2010.03.005
Mukherjee, K., and Vilcinskas, A. (2018). The entomopathogenic fungus Metarhizium robertsii communicates with the insect host Galleria mellonella during infection. Virulence 9, 402–413. doi: 10.1080/21505594.2017.1405190
Oliveira, A. S., and Rangel, D. E. N. (2018). Transient anoxia during Metarhizium robertsii growth increases conidial virulence to Tenebrio molitor. J. Invertebr. Pathol. 153, 130–133. doi: 10.1016/j.jip.2018.03.007
Ormond, E. L., Thomas, A. P., Pugh, P. J., Pell, J. K., and Roy, H. E. (2010). A fungal pathogen in time and space: the population dynamics of Beauveria bassiana in a conifer forest. FEMS Microbiol. Ecol. 74, 146–154. doi: 10.1111/j.1574-6941.2010.00939.x
Ortiz-Urquiza, A., and Keyhani, N. O. (2015). Stress response signaling and virulence: insights from entomopathogenic fungi. Curr. Genet. 61, 239–249. doi: 10.1007/s00294-014-0439-9
Pal, K. K., and Gardener, M. B. (2006). Biological control of plant pathogens. Plant Health Instr. doi: 10.1094/PHI-A-2006-1117-02Available online at: http://www.apsnet.org/edcenter/advanced/topics/Pages/BiologicalControl.aspx
Perez-Gonzalez, V. H., Guzman-Franco, A. W., Alatorre-Rosas, R., Hernandez-Lopez, J., Hernandez-Lopez, A., Carrillo-Benitez, M. G., et al. (2014). Specific diversity of the entomopathogenic fungi Beauveria and Metarhizium in Mexican agricultural soils. J. Invertebr. Pathol. 119, 54–61. doi: 10.1016/j.jip.2014.04.004
Posada, F., and Vega, F. E. (2005). Establishment of the fungal entomopathogen Beauveria bassiana (Ascomycota: Hypocreales) as an endophyte in cocoa seedlings (Theobroma cacao). Mycologia 97, 1195–1200. doi: 10.1080/15572536.2006.11832729
Pulla, S., Riotte, J., Suresh, H. S., Dattaraja, H. S., and Sukumar, R. (2016). Controls of soil spatial variability in a dry tropical forest. PLoS ONE 11:e0153212. doi: 10.1371/journal.pone.0153212
Quesada-Moraga, E., Navas-Cortes, J. A., Maranhao, E. A., Ortiz-Urquiza, A., and Santiago-Alvarez, C. (2007). Factors affecting the occurrence and distribution of entomopathogenic fungi in natural and cultivated soils. Mycol. Res. 111(Pt 8), 947–966. doi: 10.1016/j.mycres.2007.06.006
Rangel, D. E. N., Braga, G. U. L., Anderson, A. J., and Roberts, D. W. (2005). Variability in conidial thermotolerance of Metarhizium anisopliae isolates from different geographic origins. J. Invertebr. Pathol. 88, 116–125. doi: 10.1016/j.jip.2004.11.007
Rangel, D. E. N., Braga, G. U. L., Fernandes, É. K. K., Keyser, C. A., Hallsworth, J. E., and Roberts, D. W. (2015). Stress tolerance and virulence of insect-pathogenic fungi are determined by environmental conditions during conidial formation. Curr. Genet. 61, 383–404. doi: 10.1007/s00294-015-0477-y
Rehner, S., and Buckley, E. (2005). A Beauveria phylogeny inferred from nuclear ITS and EF1-alpha sequences: evidence for cryptic diversification and links to Cordyceps teleomorphs. Mycologia 97, 84–98. doi: 10.3852/mycologia.97.1.84
Rehner, S. A. (2005). “Phylogenetics of the insect pathogenic genus Beauveria,” in Insect-Fungal Associations: Ecology and Evolution, eds F. E. Vega and M. Blackwell (New York, NY: Oxford University Press), 3–27.
Rombach, M. C., Humber, R. A., and Roberts, D. W. (1986). Metarhizium flavoviride var. minus, var. nov., a pathogen of plant- and leafhoppers on rice in the Philippines and Solomon Islands. Mycotaxon 27, 87–92.
Shin, T. Y., Bae, S. M., Kim, D. J., Yun, H. G., and Woo, S. D. (2017). Evaluation of virulence, tolerance to environmental factors and antimicrobial activities of entomopathogenic fungi against two-spotted spider mite, Tetranychus urticae. Mycoscience 58, 204–212. doi: 10.1016/j.myc.2017.02.002
Singha, D., Singha, B., and Dutta, B. K. (2010). Potential of Metarhizium anisopliae and Beauveria bassiana in the control of tea termite Microtermes obesi Holmgren in vitro and under field conditions. J. Pest. Sci. 84, 69–75. doi: 10.1007/s10340-010-0328-z
Skinner, M., Gouli, S., Frank, C. E., Parker, B. L., and Kim, J. S. (2012). Management of Frankliniella occidentalis (Thysanoptera: Thripidae) with granular formulations of entomopathogenic fungi. Biol. Control 63, 246–252. doi: 10.1016/j.biocontrol.2012.08.004
St. Leger, R. J., May, B., Allee, L. L., Frank, D. C., Staples, R. C., and Robers, D. W. (1992). Genetic differences in allozymes and in formation of infection structures among isolates of the entomopathogenic fungus Metarhizium anisopliae. J. Invertebr. 60, 89–101. doi: 10.1016/0022-2011(92)90159-2
Storey, G. K., and Gardner, W. A. (1988). Movement of an aqueous spray of Beauveria bassiana into the profile of four Georgia soils. Environ. Entomol. 17, 135–139. doi: 10.1093/ee/17.1.135
Studdert, J. P., and Kaya, H. K. (1990). Effect of water potential, temperature, and clay-coating on survival of Beauveria bassiana conidia in a loam and peat soil. J. Invertebr. Pathol. 55, 417–427. doi: 10.1016/0022-2011(90)90086-L
Sung, G.-H., Hywel-Jones, N. L., Sung, J.-M., Luangsa-ard, J. J., Shrestha, B., and Spatafora, J. W. (2007). Phylogenetic classification of Cordyceps and the clavicipitaceous fungi. Stud. Mycol. 57, 5–59. doi: 10.3114/sim.2007.57.01
Takatsuka, J. (2007). Characterization of Beauveria bassiana isolates from Japan using inter-simple-sequence-repeat-anchored polymerase chain reaction (ISSR-PCR) amplification. Appl. Entomol. Zool. 42, 563–571. doi: 10.1303/aez.2007.563
Thomas, M. B., and Jenkins, N. E. (1997). Effects of temperature on growth of Metarhizium flavoviride and virulence to the variegated grasshopper, Zonocerus variegatus. Mycol. Res 101, 1469–1474. doi: 10.1017/S0953756297004401
Thompson, L. R., Sanders, J. G., McDonald, D., Amir, A., Ladau, J., Locey, K. J., et al. (2017). A communal catalogue reveals Earth's multiscale microbial diversity. Nature 551, 457–463. doi: 10.1038/nature24621
Tigano-Milani, M. S., Gomes, A. C. M. M., and Sobral, B. W. S. (1995). Genetic variability amoung Brazilian isolates of the entomopathogenic fungus Metarhizium anisopliae. J. Invertebr. 65, 206–210. doi: 10.1006/jipa.1995.1031
Vänninen, I., Tyni-Juslin, J., and Hokkanen, H. (2000). Persistence of augmented Metarhizium anisopliae and Beauveria bassiana in Finnish agricultural soils. Biocontrol 45, 201–222. doi: 10.1023/A:1009998919531
Vega, F. E. (2008). Insect pathology and fungal endophytes. J. Invertebr. Pathol. 98, 277–279. doi: 10.1016/j.jip.2008.01.008
Vega, F. E., Goettel, M. S., Blackwell, M., Chandler, D., Jackson, M. A., Keller, S., et al. (2009). Fungal entomopathogens: new insights on their ecology. Fungal Ecol. 2, 149–159. doi: 10.1016/j.funeco.2009.05.001
Vega, F. E., Meyling, N. V., Luangsa-ard, J. J., and Blackwell, M. (2012). “Fungal entomopathogens,” in Insect Pathology, eds F. E. Vega and H. K. Kaya (Cambridge, MA: Academic Press), 171–220. doi: 10.1016/B978-0-12-384984-7.00006-3
Vidal, C., Fargues, J., and Lacey, L. A. (1997). Intraspecific variability of Paecilomyces fumosoroseus: effect of temperature on vegetative growth. J. Invertebr. 70, 18–26. doi: 10.1006/jipa.1997.4658
Wang, M., Tian, J., Xiang, M., and Liu, X. (2017). Living strategy of cold-adapted fungi with the reference to several representative species. Mycology 8, 178–188. doi: 10.1080/21501203.2017.1370429
Wielgolaski, F. E., and Inouye, D. W. (2003). “High latitude climates,” in Phenology: An Integrative Environmental Science, ed M. D. Schwartz (Dordrecht: Springer), 175–194. doi: 10.1007/978-94-007-0632-3_12
Wraight, S. P., Ugine, T. A., Ramos, M. E., and Sanderson, J. P. (2016). Efficacy of spray applications of entomopathogenic fungi against western flower thrips infesting greenhouse impatiens under variable moisture conditions. Biol. Control 97, 31–47. doi: 10.1016/j.biocontrol.2016.02.016
Xiao, G., Ying, S. H., Zheng, P., Wang, Z. L., Zhang, S., Xie, X. Q., et al. (2012). Genomic perspectives on the evolution of fungal entomopathogenicity in Beauveria bassiana. Sci. Rep. 2:483. doi: 10.1038/srep00483
Keywords: Beauveria bassiana, Metarhizium anisopliae, biological control, biopesticide, IPM, entomopathogenic fungi
Citation: McGuire AV and Northfield TD (2020) Tropical Occurrence and Agricultural Importance of Beauveria bassiana and Metarhizium anisopliae. Front. Sustain. Food Syst. 4:6. doi: 10.3389/fsufs.2020.00006
Received: 11 October 2019; Accepted: 09 January 2020;
Published: 29 January 2020.
Edited by:
Kris A. G. Wyckhuys, Chinese Academy of Agricultural Sciences, ChinaReviewed by:
Maria L. Pappas, Democritus University of Thrace, GreeceIbtissem Ben Fekih, Fujian Agriculture and Forestry University, China
Mark Goettel, Retired, Lethbridge, Canada
Copyright © 2020 McGuire and Northfield. This is an open-access article distributed under the terms of the Creative Commons Attribution License (CC BY). The use, distribution or reproduction in other forums is permitted, provided the original author(s) and the copyright owner(s) are credited and that the original publication in this journal is cited, in accordance with accepted academic practice. No use, distribution or reproduction is permitted which does not comply with these terms.
*Correspondence: Tobin D. Northfield, dG5vcnRoZmllbGRAd3N1LmVkdQ==