- Department of Plant and Environmental Sciences, Faculty of Science, University of Copenhagen, Frederiksberg, Denmark
Biorefining of green biomass to produce proteins for feed and food provides an important challenge in relation to development of a future sustainable and climate-neutral agriculture. In order to make it a viable value chain, all parts of the process need to be optimized. This includes careful selection of the source of biomass, the procedure used for protein extraction as well as the separation and recovery of proteins from the press juice. We here focus on recovery of proteins from juice made from screw press fractionation of four green biomasses, viz. ryegrass, red clover, ryegrass-white clover mixture and spinach. Separating out protein from the juice is a key step and several different methods have routinely been applied. We demonstrate that lignosulfonates aid in the precipitation of proteins in the juice. The optimal loading of lignosulfonate was 0.6–0.7 g per g crude protein in the juice. For ryegrass and red clover juice, this loading resulted in 10–25% increase in precipitated protein compared to the classical precipitation by heat treatment or acidification. For spinach, up to 60% increase in protein recovery was obtained by lignosulfonate addition. We conclude that lignosulfonate-assisted precipitation holds potential for improving the protein yield in biorefining of green biomass.
Introduction
Every year, large amounts of soybean are transported over long distances from e.g., South America to support production of monogastric animals such as pigs and chickens in Europe, while local sources of plant proteins are underutilized (Parajuli et al., 2015). One potential alternative protein source is green protein extracted from green biomasses such as grass, clover and alfalfa through a biorefinery process. The carbon footprint of this local protein feed production is lower than the carbon footprint soybean meal from South America (Kamp et al., 2019). Studies have also documented that under North European conditions, green perennial crops could provide larger biomass and protein output along with low environmental footprint compared to traditional crops such as wheat, beet or maize (Manevski et al., 2018). Therefore, biorefining of green biomasses has attracted a lot of attention in recent years, especially in Denmark (Corona et al., 2018a).
The green biomasses cannot be used directly as feed for monogastric animals because of the high fiber content. A key step in the green biorefinery is the protein separation. Typically, the green biomass is fractionated into two different fractions by pressing in e.g., a screw press (Zhang et al., 2017; Corona et al., 2018a). One fraction is the pulp, which contains fibers along with insoluble and fiber bound proteins. This fraction is generally used for ruminant animals, which are capable of digesting the material and utilize the fiber bound protein (Chowdhury et al., 2018). The other fraction is a juice, where the soluble proteins are found. Most of these proteins have a much higher bioavailability and are therefore suitable for monogastric animals and even human nutrition. The soluble protein is separated from the juice and turned into a protein concentrate. Feeding trials with protein concentrate obtained from white clover, red clover, perennial grass and alfalfa have documented a high digestibility and a well-balanced amino acid composition, which makes it a promising protein source for monogastrics (Stødkilde et al., 2018, 2019).
The separation of protein from the juice can be done in several different ways (Zhang et al., 2017). The classical methods include acidification or heat treatment. In the first mentioned approach, isoelectric point aggregation and precipitation is obtained by acidification of the juice to a pH between 4.0 and 4.5 by addition of e.g., hydrochloric acid. Heat precipitation occurs by denaturation and aggregation of proteins at 60–80°C (Bray and Humphries, 1979). A variant of the isoelectric precipitation relies on fermentation with lactic acid bacteria, converting the free sugars present in the juice to organic acids thereby lowering the pH (Santamaría-Fernández et al., 2017). Using lactic acid bacteria is a more difficult process that requires some more expertise and takes more time, but the conditions are gentler and may therefore offer an advantage. An additional advantage could be the possible probiotic effect of lactic acid bacteria in the gastrointestinal system of animals (Khan and Iqbal, 2016). To facilitate precipitation of proteins, addition of a flocculant to the juice might provide an alternative the two classical methods based on acidification or heat treatment (Felicetta and Peacock, 1971; Bray and Humphries, 1979; Knuckles et al., 1980; Chen et al., 1992; Sediq et al., 2015). Flocculents are compounds that facilitate aggregation of smaller particles making them easier to separate from the liquid phase. Some very different types of flocculants have been used for protein precipitation, e.g., Knuckles et al. used both anionic, cationic and non-ionic commercially available flocculants (Knuckles et al., 1980).
Lignosulfonate, a by-product of the sulfite pulping process, is a hydrophobic, water soluble, and negative charged biobased polymer, which has been used as a flocculent to facilitate removal of organic substances including proteins from wastewater (Chen et al., 1992). The objective of the present work was to investigate the potential of using lignosulfonates as a flocculant for aggregation and precipitation of proteins in juice obtained from green biomass. The hypothesis of the work was that lignosulfonate-assisted protein precipitation was more effective compared to classical precipitation by heat treatment or isoelectric precipitation. The study involved testing three different commercially available lignosulfonates on juice obtained from four different relevant biomasses, spinach, ryegrass-white clover mixture, ryegrass, and red clover.
Materials and Methods
Sample Material
Plant material was sampled at an experimental field near Store Heddinge, Zealand, Denmark. The material collected consisted of ryegrass (Lolium perenne cv. Mahogany), red clover (Trifolium pratense cv. Callisto) and a mixture of ryegrass and white clover (Trifolium repens). After harvest, the samples were frozen within 3 h and stored at −20°C until the experiments were performed. In the initial experiment, fresh spinach stored at 5°C from a local supermarket was used. The lignosulfonates were provided by the company Borregaard Lignotech, Sarpsborg, Norway. Three different types of lignosulfonates were used (Table 1).
Sample Preparation and Precipitation
Spinach samples were used directly from the fridge, whereas frozen samples of clover and grass were thawed at 4°C overnight. Once thawed, 2,000 grams of the material was run through a twin-screw press (Angelia 8500S Angel juicer, Angel Co. Ltd., Busan, South Korea), equipped with a coarse screen with hole sizes of 1 mm. The resulting pulp fraction was mixed with demineralized water 1:1 (w/w) and run through the juicer once more. The two resulting juices were mixed and used for the precipitation experiment.
Precipitation was performed either on the juice directly (only initial experiment) or on the supernatant after a clearing to remove larger particles and fibers. The clearing was done either directly on the juice or after adjusting the pH to 2.5 by addition of 6 M HCl. The clearing involved gentle centrifugation at 500 g for 1 min. The supernatant was used for precipitation.
Juice or supernatant was split into 30 mL aliquots in 50 mL Falcon tubes. The precipitation was performed directly in the tubes and in duplicate. Traditional precipitation methods involved heating to 80°C and keeping it there for 30 s or addition of hydrochloric acid (6 M) to pH 4–4.5—termed isoelectric precipitation. Lignosulfonate precipitation was done by adding solid lignosulfonate directly to the tubes in the required amount. After lignosulfonates were dissolved, the pH was lowered to 2–3 by addition of 6 M HCl. The lignosulfonate loading was based on content of crude protein in the sample. The loading for the initial experiments was 1:1 (w/w). Based on a conversion factor of 6.25 between total N measured and crude protein, a 1:1 loading corresponded to roughly 6 g lignosulfonate per g N, hereafter defined as 6 N eq. For subsequent experiments, the loading was between 0 and 6 N eq. Lignosulfonate treatment also including a control without lignosulfonate addition (i.e., loading 0 N eq.). After precipitation, all samples were centrifuged for 20 min at 4000 g. The supernatant (brown juice) was decanted off and the resulting pellet was dried by freeze drying for 72 h.
Analysis
Nitrate was measured on the supernatant resulting from the precipitation. The supernatant was diluted and analyzed on a Dionex ICS-2100, with an anion exchange column (AS11-HC, 2 × 250 mm). Isocratic elution (30 mM KOH) was performed using an eluent generator. Nitrate was detected by UV at 214 nm.
Free amino acids were determined on the supernatant. The supernatant was diluted and coupled with aminoquinoline reagent (AccQ-Tag, Waters, Millford, MA, USA) according to Dahl-Lassen et al. (2018). The analysis was performed with a Water's UPLC and for detection and quantification a Water's QDa single quadrupole mass spectrometer was used.
Amino acid composition of the protein concentrate was determined according to Dahl-Lassen et al. (2018). In brief, after acid hydrolysis (6 M HCl with 0.1% w/v phenol, 110°C for 24 h) samples were neutralized and coupled with aminoquinoline reagent (AccQ-Tag, Waters, Millford, MA, USA). Separation was performed on a Water's UPLC with detection and quantification on a Water's QDa single quadrupole mass spectrometer.
DNA measurements were performed by spectrophotometry at 260 nm, correcting for protein content by a measurement on 280 nm, using a NanoDrop instrument (Thermo Fischer Scientific, Waltham, MA, USA). The samples were measured directly without dilutions.
Dry matter in all samples was determined by freeze drying for 72 h.
N concentrations of the dried and finely ground materials were analyzed by Dumas combustion using a Vario Macro and Vario Pyro Cube elemental analyzer (Elementar Analysensysteme GmbH, Hanau, Germany). Data quality was evaluated by analysis of standard reference materials [RM8494 and 141d acetanilide, National Institute of Standards and Technology (NIST), Gaithersburg, MD, USA].
The SDS-PAGE analysis was performed on a 16% polyacrylamide tris/tricine-gel from Anamed Elektrophorese, Rodau, Germany. The molecular weight marker used was ProteMix Proteinstandard 2.5–220 kDa (Anamed Elektrophorese, Rodau, Germany). The commercially available tris/tricine sample buffer was used. The running buffer was made by adding 121.1 g of tris base and 179.2 g tricine to 800 mL milliQ water and adding 50 mL SDS solution (20% w/w). Then fill up to a total volume of 1 L. The gel was run according to the recommendation from the manufacturer (125 V for 100 min). The gel was stained using InstaBlue coomassie stain (Expedeon, Cambridgeshire, UK).
Results and Discussion
Initial Experiment
An initial experiment was performed using spinach juice to test three different types of lignosulfonates (Table 1). The lignosulfonates were added as a solid powder to a final loading of 1:1 (w/w) with crude protein. It was encountered that the pellet formed after precipitation and centrifugation of the samples treated with lignosulfonates was very unstable, making the decanting of the supernatant very difficult. This effect could possibly be caused by lignosulfonates acting as dispersant of the plant fibers and debris present in the crude juice. Lignosulfonates are in some applications used as dispersant of particles (Chen et al., 2018). The problem was solved by a mild centrifugation (called a clearing) of the juice prior to the precipitation.
The experiment with cleared spinach juice showed rather similar performance among the three types of lignosulfonates tested (Figure 1). The use of the lignosulfonate designated 2960 precipitated 47.8% of N whereas the use of 2961 resulted in the lowest precipitation of 45.8%. It appeared that for this type of application, the type of counter-ion (calcium or potassium, Table 1) had no effect on the interaction with the protein and the precipitation efficiency. The lignosulfonate 2961 was indicated as a high molecular weight fraction and it can be speculated that slightly lower efficiency on a weight basis was a result of less lignosulfonate molecules to interact with the proteins.
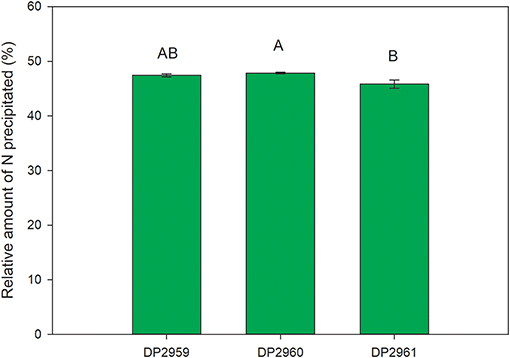
Figure 1. Relative amount of total N precipitated from spinach juice for the three different types of lignosulfonates. Values are means and standard deviation of two replicates. Data were analyzed with ANOVA followed by a Tukey HSD test. Different letters denote significant difference at P = 0.05.
As lignosulfonate 2960 had the best precipitation among the three tested materials it was selected for further experiments. The precipitation of protein from clear spinach with lignosulfonate 2960 was tested against the traditional methods of heat and isoelectric point precipitation (Figure 2). Both heat and isoelectric point precipitation resulted in ~35% of the total N in the cleared juice to be precipitated. By addition of lignosulfonates, the recovery of total N was increased to ~55%. The results thus confirmed that addition of lignosulfonates improved the precipitation efficiency. However, a large fraction of the total N still remained in the supernatant.
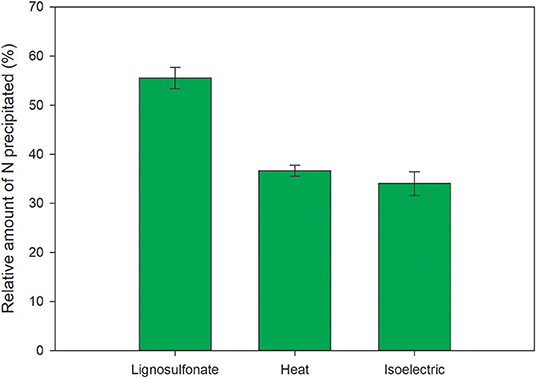
Figure 2. Relative amount of total N precipitated from spinach juice for the three different treatments. Values are means and standard deviation of three replicates.
An SDS-PAGE analysis of the supernatants revealed that the heat and isoelectric precipitations efficiently removed larger proteins, but a large number of smaller proteins were left behind in the supernatant (Figure 3). The lignosulfonate treatment showed very little evidence of any proteins remaining in the supernatant, although some weak staining at the bottom of the lane indicated that some smaller peptides (up to ~30 AAs) did not precipitate. However, almost all larger peptides and proteins precipitated out of the sample. Based on this result, and taking into account the possible mode by which lignosulfonates interact with proteins, it is likely that lignosulfonates can assist in precipitating smaller and highly soluble proteins. At pH 2–3, the lignosulfonates are negatively charged and most proteins and peptides are positively charged creating an electrostatic interaction. The neutralization of the surface charges will give the formed complex a net charge of zero and thereby promote aggregation via van der Waals interaction. The neutralization along formation of large complexes or aggregates accelerates the precipitation.
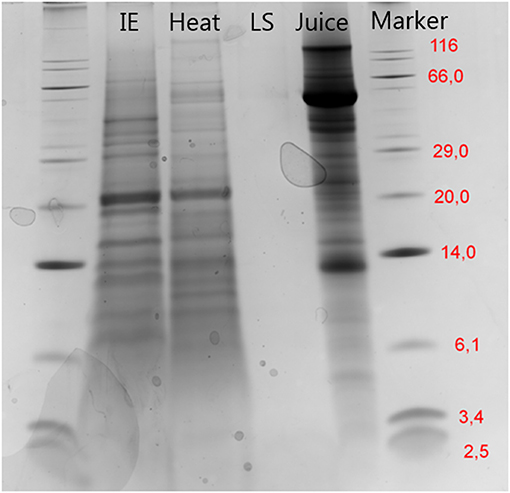
Figure 3. SDS-PAGE analysis of the brown juices after precipitation of protein from spinach juice. LS is lignosulfonates. Thirty microliter sample was loaded for lignosulfonates, heat and acid. In the case of juice, 4 μL sample was loaded. The numbers in red indicate the size of proteins in kDa.
As SDS-PAGE analysis showed only minor amounts of protein N left in the supernatant, further analysis of N speciation was conducted to account for the remaining 45% N. Anion analysis of the supernatant revealed that nitrate accounted for ~11% of N in the supernatant. N in free amino acids accounted for another 17%. Measurements of DNA was performed, but lignosulfonates remaining in the supernatant interfered with the measurements. The DNA measurement was consequently performed on the juice before treatment. This added some uncertainty to the measurements, but assuming that no DNA was precipitated with the lignosulfonates, DNA accounted for ~14% of the N in the cleared juice. In total, 97% of N was thereby accounted for. This supports the conclusion drawn from the SDS-PAGE analysis that lignosulfonate treatment resulted in almost complete precipitation of protein from the juice whereas the heat treatment and isoelectric precipitation left a significant proportion, ~20%, of the protein in the supernatant.
Loading Experiment From Ryegrass-Clover
Crops that have frequently been tested for extraction of green protein are various grasses (e.g., ryegrass), clover (white or red) and alfalfa (Corona et al., 2018b; Stødkilde et al., 2019). A common forage crop is a mixture of ryegrass and clover, which was in this study used for establishing the effect of lignosulfonate loading on the protein precipitation.
The initial experiment was performed with a lignosulfonate loading of ~1:1 (w/w) relative to crude protein in the juice. This represents a large amount of lignosulfonate added, both from an economical perspective but also from the point of purity of the final product. A lignosulfonate loading experiment was performed in order to minimize the amount of lignosulfonate used. Since crude protein is a calculated value of 6.25 times measured total N, the lignosulfonate loading was determined relative to total N measured. For the experiments with optimal lignosulfonate loading, the unit N equivalents (N eq) was introduced to describe the mass of lignosulfonate relative to the amount of N in the sample. So 6 N eq of lignosulfonate means a mass of lignosulfonates equal to 6 times the mass of total N, which also was equal to approximately a 1:1 loading relative to crude protein.
Using juice form a grass-clover mixture, the loading experiment showed that the amount of precipitated protein increased up to a loading of ~4 N eq, precipitating 41.0% (Figure 4). This was a 16% improvement compared to the control without adding lignosulfonate. In a previous study describing the use of lignosulfonates for removal of proteins from wastewater, a loading of ~1.2 N eq was applied, which was a much lower dose than shown in this work (Felicetta and Peacock, 1971). It is however likely that other compounds in the wastewater from a fish processing plant could assist in the flocculation or aggregation of proteins thereby improving the effect of lignosulfonates in that case. The type and composition of proteins in this type of wastewater will also differ from plant proteins used here, which could contribute to the different optimum in lignosulfonate loading.
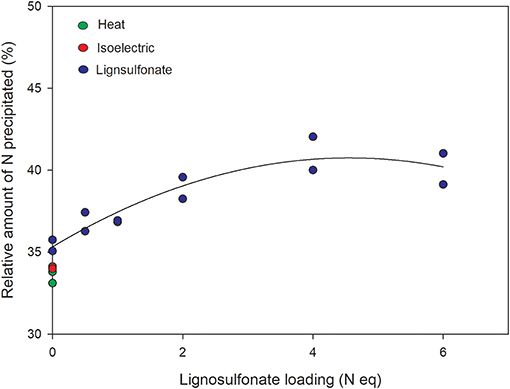
Figure 4. Relative amount of N precipitated from grass-clover juice at different lignosulfonate loadings expressed as nitrogen equivalents (N eq), i.e., the mass of lignosulfonate relative to the amount of nitrogen. A loading of 6 N eq corresponds to ~1:1 ratio of lignosulfonate to crude protein. A quadratic regression line is included. Heat and isoelectric precipitation is included as reference method.
For reference, heat and isoelectric precipitation was included (Figure 4). Heat precipitation yielded the lowest precipitation yield, on average 33.5%, whereas isoelectric precipitation at pH 4–4.5 precipitated 34.0%. Based on the average isolectric point of all proteins in green juice, the optimum for isolectric precipitation is typically pH 4–4.5 (Santamaría-Fernández et al., 2017; Zhang et al., 2017). The results obtained here revealed that precipitation at a lower pH of 2.5, i.e., the pH used for lignosulfonate precipitation, gave a small improvement of the precipitation by 4%. Although lowering pH below the isoelectric point improved the precipitation, the beneficial effect of adding lignosulfonates was clear. The improvement in protein precipitation from grass-clover juice with the use of lignosulfonates compared to the traditional methods was around 20%. Interestingly, this was clearly much lower than what was observed for spinach juice, where lignosulfonate precipitation improved the precipitation by almost 60% at the same lignosulfonate loading of 6 N eq. Possible differences in the juice sample matrix from various crops could likely influence the function of lignosulfonates.
Precipitation of Protein From Ryegrass and Red Clover Juice
A more though experiment studying the effect of crop type on protein precipitation was done by performing precipitation of protein from ryegrass (Lolium perenne) and red clover (Trifolium pratense). Based on the loading study, the experiments were performed in the range up to 4 N eq.
For both ryegrass and red clover, the lignosulfonate treatment was significantly better than the classical treatments (Figure 5). For ryegrass juice, the relative amount of N precipitated was increased from 35 to 44% by addition of 4 N eq. lignosulfonates compared to the heat treatment. For red clover, the corresponding number was from 45 to 51%. Acid isoelectric precipitation was better than heat treatment for both ryegrass and red clover, but not significant (Figure 5). Comparing lignosulfonate treatment (4 N eq) with isoelectric precipitation revealed an increase in precipitation of ~18% for ryegrass and 11% for red clover, which was a significant increase. For both ryegrass and red clover juice, the effect of lignosulfonate increased with loading up to 2–4 N eq in accordance with the observations done on grass-clover juice in the loading study (Figure 4).
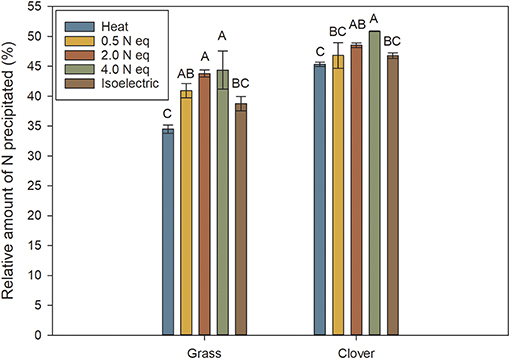
Figure 5. Relative amount of N precipitated by heat, isoelectric precipitation, or lignosulfonate treatment of cleared juice from different biomasses. Values are means ± S.D. (n = 3). Data were analyzed with ANOVA followed by a Tukey HSD test. Different letters denote significant difference at P = 0.05.
The improvement in precipitation by addition of optimum lignosulfonate loading of 4 N eq. was for both ryegrass and red clover less than observed for spinach in the initial study. For ryegrass, the improvement was 29% when compared to heat precipitation and 13% in the case of red clover. The improvement previously observed with the grass-clover mixture was roughly 20%. Whereas, the results proved that lignosulfonate addition in general improved protein precipitation they also revealed differences in the observed effect among the tested biomasses. Previous studies using isoelectric precipitation have found significant differences in the degree of protein precipitation from juice derived from various crops and from different development stages (Santamaría-Fernández et al., 2017, 2019). In this given case, the effect of lignosulfonates was more comparable between grass-clover, ryegrass and red cover than compared to spinach. The reason for this is currently unknown, but the time from harvest to processing could have influenced the results. The grass-clover, ryegrass, and red clover samples were all frozen shortly after harvest to minimize post-harvest degradation of proteins by endogenous proteases. The spinach sample was on the other hand obtained from the supermarket, and time from harvest was unknown, but obviously much longer. For spinach, post-harvest protein degradation and/or modifications therefore likely occurred. The proteolytic degradation of proteins could increase the solubility of some proteins (Sari et al., 2013), thereby making them more difficult to precipitate by heat or isoelectric precipitation. In the case of lignosulfonates addition, the hypothesis is that the complexation and aggregation of lignosulfonates, proteins and peptides due to electrostatic interaction favored precipitation of even the degraded protein. For the other samples, the freezing-thawing could have denatured proteins making them easier to precipitate with all tested methods thereby minimizing the effect of the lignosulfonates.
As precipitation was calculated on the basis of total N, the presence of other N containing compounds could influence the apparent precipitation efficiency. For ryegrass and red clover, 50–55% of N was in the best case not precipitated. Analysis of the brown juice after the precipitation revealed that nitrate was only present in insignificant amounts (~0.5% of the total N in the cleared juice). Unlike nitrate, free amino acids contributed significantly to the total N in the juice. Of total N, 31 and 30% was determined to come from free amino acids in ryegrass and red clover juice, respectively. Since free amino acids are highly soluble in water, it was unlikely that they would precipitate under any condition tested in this experiment. Free amino acids therefore accounted for the majority of the N not precipitated. DNA was not measured, but constituted 14% of N in spinach juice, and could contribute the remaining N not recovered in the precipitate.
A modified version of the clearing of the juice where the pH was already lowered to 2–3 before centrifugation was also tested. From the acid cleared samples, in general a smaller fraction of the N was precipitated (Figure 6). This was a consequence of a large part of the protein already being precipitated in the clearing process and thereby the other sources of N (nitrate, free amino acids and DNA) got more predominant in the acid cleared juice. With regards to the lignosulfonates, the results supported the findings from the previous experiment, demonstrating that a loading of 4.0 N eq was the most efficient and that even addition of small amounts of lignosulfonates increased the precipitation efficiency (Figure 6). The effect was strongest on grass juice compared to clover. An SDS-PAGE analysis confirmed that acid clearing removed significant parts of particularly the larger proteins in the juice compared to the clearing by just centrifugation (Figure 7). In all supernatants after precipitation with heat and lignosulfonates bands were visible, but the higher the lignosulfonate loading, the weaker the bands (Figure 7). Compared to heat precipitation, bands with size <14 kDa appeared less predominant in case of lignosulfonate treatment. This suggests that the lignosulfonates interacted equally well with most proteins.
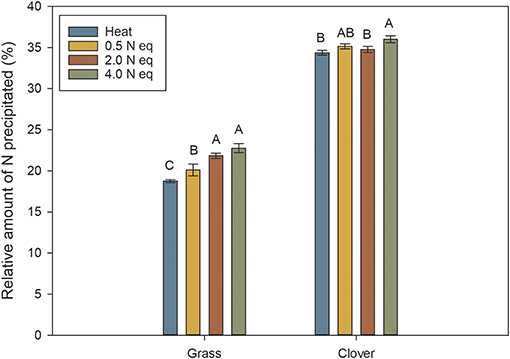
Figure 6. Relative amount of N precipitated with different treatments from acid-cleared juice of different biomasses. Values are means ± S.D. (n = 3). Data were analyzed with ANOVA followed by a Tukey HSD test. Different letters denote significant difference at P = 0.05.
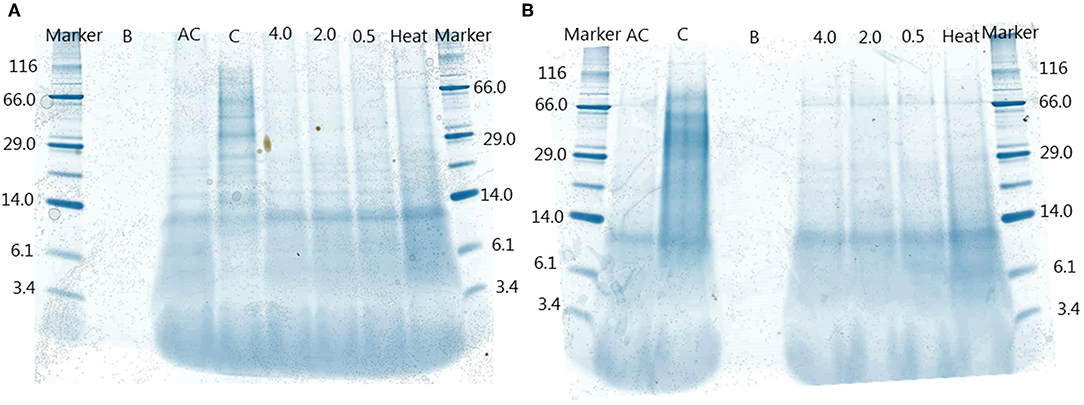
Figure 7. SDS-PAGE analysis of supernatant after precipitation from (A) red clover (B) ryegrass. C indicates the cleared juice, AC indicates acid cleared juice, B indicates blank lanes, and the numbers are N eq of lignosulfonates.
Juice Clearing and Purity of Protein Concentrate
The initial clearing of the juice to remove particular matter and fibers prior to the precipitation also removed proteins still associated with fibers. The juice N ending up in the fiber fraction was around 51% for ryegrass and 56% for red clover when performing the clearing by mild centrifugation. This means that for the best case (4 N eq. lignosulfonate), 73% of total N in the ryegrass juice was recovered in the fibers and protein concentrate. For red clover 78% was recovered in the best case (Table 2). For the acid-clearing, around 62% of the N in ryegrass was removed with the fiber fraction and 68% in the case of red clover. The total recovery was with this setup in the best case, again 4 N eq. lignosulfonate, 71% of total N recovered in the fiber and protein concentrate for ryegrass and 80% for red clover. For comparison direct heat precipitation of the juice resulted in N recoveries of 82 and 84% from ryegrass and red clover, respectively. The fiber fraction can still be used in animal feed, but the bioavailability of the protein is lower as these proteins will be bound or associated with the lignocellulosic fibers. By removing this fibrous fraction, a more pure protein fraction with higher bioavailability can be obtained from the juice. This will result in three protein containing fractions being produced. The press pulp, where the protein is mostly unavailable for monogastric animals, the fiber fraction where the bioavailability is higher but still limited, and a protein concentrate of high purity and bioavailability.
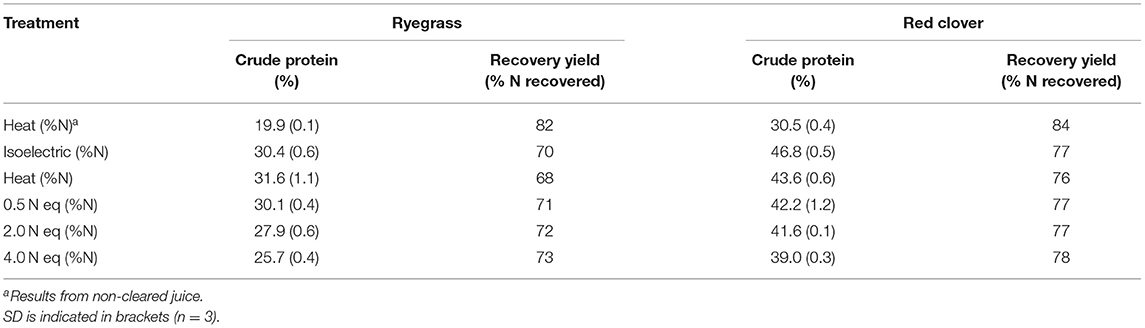
Table 2. Relative amount of crude protein in protein concentrate on a DM basis from cleared juice and total recovery yield of N from the juice including the clearing.
The purity of the protein concentrate was significantly better for the cleared juice compared to a heat precipitation on the uncleared juice (Table 2). The protein concentrates with the highest purity were obtained from the classical precipitation methods, acid or heat. For red clover a protein concentrate with up to 47% crude protein was obtained, which is comparable to crude protein content of soybean meal (Lagos and Stein, 2017). The lignosulfonates used in the treatment precipitated with the protein from the juice, thereby significantly lowering the purity with addition of lignosulfonates. The increasing total protein yield (Figure 5 and Table 2) was therefore off-set by a gradually decrease in purity. However, the purity of the protein precipitate even at 4 N eq. lignosulfonate was much better than the heat precipitate from uncleared juice. The purities obtained in the protein concentrates were comparable to what have been produced in other studies from similar biomasses (Stødkilde et al., 2019).
Based on the red clover data, it was estimated that at the low lignosulfonate loading of 0.5 N eq. the lignosulfonates accounted for around 10% of DM in the protein concentrate, whereas at 4 N eq. it was around 25%. Lignosulfonates are already used in formulation of feed tablets today as a binder and therefore approved to be used in feed in concentrations up to 10 g/kg (EFSA, 2016). The high amounts of lignosulfonates in the protein concentrates therefore limits the inclusion rates in the final feed. It would be relevant to optimize the process to lower the lignosulfonate addition or develop methods to remove some of the lignosulfonates again.
Testing the bioavailability of the protein in the protein concentrates, e.g., by feeding trials, was beyond the scope of this paper. However, amino acid analysis revealed that the amino acid profile of the protein concentrates produced by heat or isoelectric precipitation did not differ from the one produced by lignosulfonate precipitation (Figure 8). On the basis of amino acid composition, the precipitation method did not influence the feed quality of the product. Compared to soybean meal commonly used in feed (Lagos and Stein, 2017), both ryegrass and red clover protein had well-balanced amino acid composition with favorable content of essential amino acids (Figure 8). Previous studies with proteins extracted from white clover, red clover and grass and precipitated by traditional methods obtained similar results and also documented good digestibility of the produced protein concentrates (Stødkilde et al., 2018, 2019). The effect of lignosulfonate on digestibility therefore remains to be investigated.
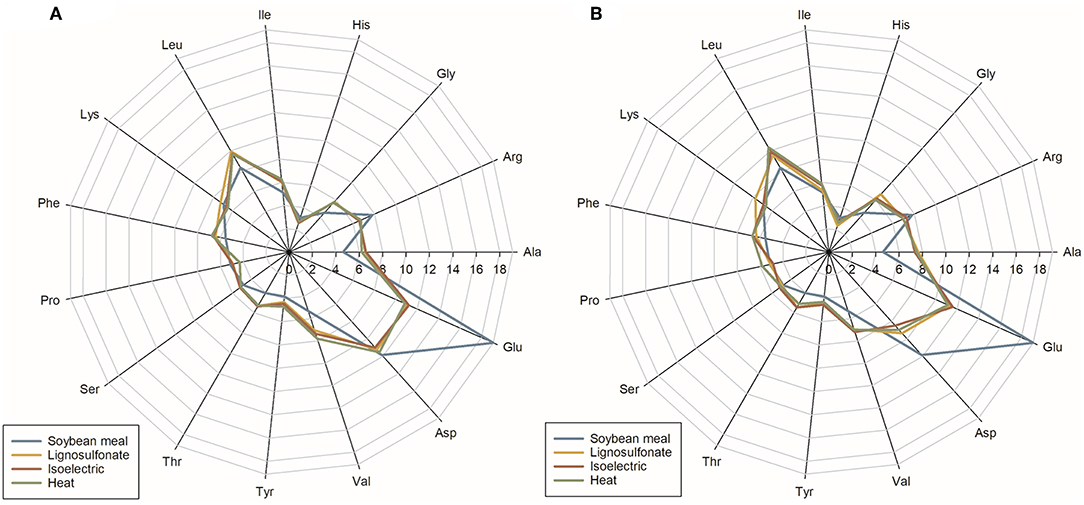
Figure 8. Amino acid profile of the protein concentrates produced after precipitation of cleared juice from (A) red clover and (B) ryegrass. Values (%) are relative to total amino acid content. The protein was precipitated either by heat or isoelectric precipitation or addition of 4 N eq. lignosulfonates. For comparison the amino acid profile of soy bean meal is included (Lagos and Stein, 2017).
Conclusion
The efficiency of lignosulfonates as a co-precipitant for proteins in juice extracted from four different green biomasses was demonstrated. Compared to classical precipitation by heat treatment or isoelectric precipitation, the addition of lignosulfonates increased the relative amount of protein precipitated from the juice of ryegrass from 38 to 44%. For red clover, the corresponding increase was from 46 to 51%, for a grass-clover mixture from 34 to 41% and for spinach from 35 to 55%. The optimal lignosulfonate loading for ryegrass and red clover was determined to be ~4 N eq, corresponding to 0.6–0.7 g lignosulfonate per g protein. Although the effect of lignosulfonate addition was not equally high for all biomasses types when compared to heat treatment or isoelectric precipitation, we conclude that lignosulfonate addition may improve the protein yield in biorefining of green biomass.
Data Availability Statement
The datasets generated for this study are available on request to the corresponding author.
Author Contributions
RC developed and performed the experimental work. All authors contributed to the design of the work, the analysis of the data, the writing of the manuscript, read, and approved the final manuscript.
Funding
This work was supported by funding from Stiftelsen Hofmansgave to HJ and the Bio-Value Strategic Platform for Innovation and Research, funded by Innovation Fund Denmark (Grant No. 0603-00522B to JS).
Conflict of Interest
The authors declare that the research was conducted in the absence of any commercial or financial relationships that could be construed as a potential conflict of interest.
Acknowledgments
The authors would like to acknowledge the company DLF, Store Heddinge, Denmark for providing the grass and clover plant materials and Borregaard Lignotech, Sarpsborg, Norway for providing the lignosulfonates used in the experiments.
References
Bray, W. J., and Humphries, C. (1979). Preparation of white leaf protein concentrate using a polyanionic flocculant. J. Sci. Food Agric. 30, 171–176. doi: 10.1002/jsfa.2740300212
Chen, J., Kazzaz, A. E., AlipoorMazandarani, N., Feizi, Z. H., and Fatehi, P. (2018). Production of flocculants, adsorbents, and dispersants from lignin. Molecules 23, 1–25. doi: 10.3390/molecules23040868
Chen, W., Walker, S., and John, C. B. (1992). The mechanism of floc formation in protein precipitation by polyelectrolytes. Chem. Eng. Sci. 47, 1039–1045. doi: 10.1016/0009-2509(92)80230-A
Chowdhury, M. R., Lashkari, S., Jensen, S. K., Ambye-Jensen, M., and Weisbjerg, M. R. (2018). Effects of heat treatment of green protein on in situ protein disappearance and in vitro fatty acid biohydrogenation. J. Agric. Food Chem. 66, 8169–8178. doi: 10.1021/acs.jafc.8b02176
Corona, A., Ambye-Jensen, M., Vega, G. C., Hauschild, M. Z., and Birkved, M. (2018a). Techno-environmental assessment of the green biorefinery concept: combining process simulation and life cycle assessment at an early design stage. Sci. Total Environ. 635, 100–111. doi: 10.1016/j.scitotenv.2018.03.357
Corona, A., Parajuli, R., Ambye-Jensen, M., Hauschild, M. Z., and Birkved, M. (2018b). Environmental screening of potential biomass for green biorefinery conversion. J. Clean. Prod. 189, 344–357. doi: 10.1016/j.jclepro.2018.03.316
Dahl-Lassen, R., Van Hecke, J., Jørgensen, H., Bukh, C., Andersen, B., and Schjoerring, J. K. (2018). High-throughput analysis of amino acids in plant materials by single quadrupole mass spectrometry. Plant Methods 14:8. doi: 10.1186/s13007-018-0277-8
EFSA (2016). Scientific Opinion on the safety and efficacy of lignosulphonate as a feed additive for all animal species. EFSA J. 13, 1–17. doi: 10.2903/j.efsa.2015.4160
Felicetta, V. F., and Peacock, R. (1971). Recovery of Proteinaceous Material from Waste Effluents. U.S. Patent No. 3,662,510.
Kamp, A., Ambye-Jensen, M., and Østergård, H. (2019). Modelling matter and energy flows of local, refined grass-clover protein feed as alternative to imported soy meal. Ecol. Modell. 410:108738. doi: 10.1016/j.ecolmodel.2019.108738
Khan, S. H., and Iqbal, J. (2016). Recent advances in the role of organic acids in poultry nutrition. J. Appl. Anim. Res. 44, 359–369. doi: 10.1080/09712119.2015.1079527
Knuckles, B. E., Edwards, R. H., Kohler, G. O., and Whitney, L. F. (1980). Flocculants in the separation of green and soluble white protein fractions from Alfalfa. J. Agric. Food Chem. 28, 32–36. doi: 10.1021/jf60227a032
Lagos, L. V., and Stein, H. H. (2017). Chemical composition and amino acid digestibility of soybean meal produced in the United States, China, Argentina, Brazil, or India. J. Anim. Sci. 95:1626. doi: 10.2527/jas.2017.1440
Manevski, K., Lærke, P. E., Olesen, J. E., and Jørgensen, U. (2018). Nitrogen balances of innovative cropping systems for feedstock production to future biorefineries. Sci. Total Environ. 633, 372–390. doi: 10.1016/j.scitotenv.2018.03.155
Parajuli, R., Dalgaard, T., Jørgensen, U., Adamsen, A. P. S., Knudsen, M. T., Birkved, M., et al. (2015). Biorefining in the prevailing energy and materials crisis: a review of sustainable pathways for biorefinery value chains and sustainability assessment methodologies. Renew. Sustain. Energy Rev. 43, 244–263. doi: 10.1016/j.rser.2014.11.041
Santamaría-Fernández, M., Karkov Ytting, N., and Lübeck, M. (2019). Influence of the development stage of perennial forage crops for the recovery yields of extractable proteins using lactic acid fermentation. J. Clean. Prod. 218, 1055–1064. doi: 10.1016/j.jclepro.2019.01.292
Santamaría-Fernández, M., Molinuevo-Salces, B., Kiel, P., Steenfeldt, S., Uellendahl, H., and Lübeck, M. (2017). Lactic acid fermentation for refining proteins from green crops and obtaining a high quality feed product for monogastric animals. J. Clean. Prod. 162, 875–881. doi: 10.1016/j.jclepro.2017.06.115
Sari, Y. W., Bruins, M. E., and Sanders, J. P. M. (2013). Enzyme assisted protein extraction from rapeseed, soybean, and microalgae meals. Ind. Crops Prod. 43, 78–83. doi: 10.1016/j.indcrop.2012.07.014
Sediq, A. S., Nejadnik, M. R., El Bialy, I., Witkamp, G. J., and Jiskoot, W. (2015). Protein-polyelectrolyte interactions: monitoring particle formation and growth by nanoparticle tracking analysis and flow imaging microscopy. Eur. J. Pharm. Biopharm. 93, 339–345. doi: 10.1016/j.ejpb.2015.04.021
Stødkilde, L., Damborg, V. K., Jørgensen, H., Lærke, H. N., and Jensen, S. K. (2018). White clover fractions as protein source for monogastrics: dry matter digestibility and protein digestibility-corrected amino acid scores. J. Sci. Food Agric. 98, 2557–2563. doi: 10.1002/jsfa.8744
Stødkilde, L., Damborg, V. K., Jørgensen, H., Lærke, H. N., and Jensen, S. K. (2019). Digestibility of fractionated green biomass as protein source for monogastric animals. Animal. 13, 1817–25. doi: 10.1017/S1751731119000156
Keywords: green juice, protein feed, flocculent, green biomass, grass, clover, plant protein
Citation: la Cour R, Schjoerring JK and Jørgensen H (2019) Enhancing Protein Recovery in Green Biorefineries by Lignosulfonate-Assisted Precipitation. Front. Sustain. Food Syst. 3:112. doi: 10.3389/fsufs.2019.00112
Received: 07 October 2019; Accepted: 27 November 2019;
Published: 12 December 2019.
Edited by:
José Martinez, National Research Institute of Science and Technology for Environment and Agriculture (IRSTEA), FranceReviewed by:
Beatriz Molinuevo-Salces, Instituto Tecnológico Agrario de Castilla y León, SpainMarieke E. Bruins, Wageningen University & Research, Netherlands
Copyright © 2019 la Cour, Schjoerring and Jørgensen. This is an open-access article distributed under the terms of the Creative Commons Attribution License (CC BY). The use, distribution or reproduction in other forums is permitted, provided the original author(s) and the copyright owner(s) are credited and that the original publication in this journal is cited, in accordance with accepted academic practice. No use, distribution or reproduction is permitted which does not comply with these terms.
*Correspondence: Henning Jørgensen, hej@plen.ku.dk