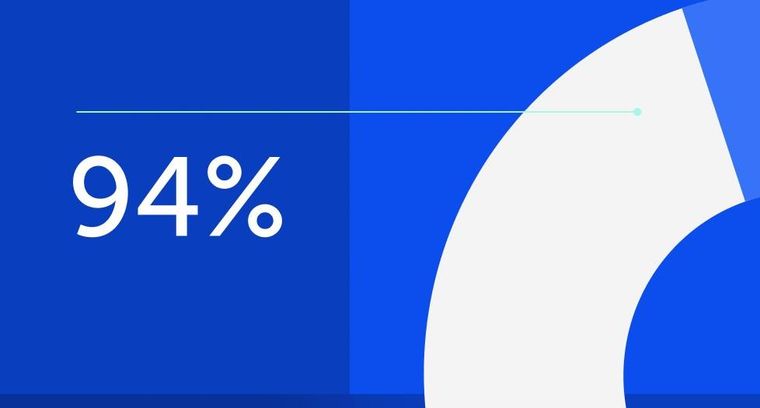
94% of researchers rate our articles as excellent or good
Learn more about the work of our research integrity team to safeguard the quality of each article we publish.
Find out more
ORIGINAL RESEARCH article
Front. Sustain. Food Syst., 25 October 2019
Sec. Nutrition and Sustainable Diets
Volume 3 - 2019 | https://doi.org/10.3389/fsufs.2019.00093
This article is part of the Research TopicImpacts of Tropical Landscape Change on Human Diet and Local Food SystemsView all 10 articles
Dams provide energy and irrigation water, but also alter natural water flows that support fisheries. This tradeoff presents a risk for human nutrition in regions dependent on aquatic foods, including the Lower Mekong Basin (LMB), where over 100 dams are planned or in construction. Previous models estimate significant reductions in fishery production resulting from these dams. This study estimates the number of new nutritionally insecure people (i.e., those at risk for nutritional deficiencies) associated with Mekong damming. We calculated population-level nutritional needs based on the Estimated Average Requirements (EARs) for Cambodia and the entire LMB. We then estimated fish-derived nutrient supplies by integrating data on annual fishery production and fish nutrient content for a wide range of species. Finally, we synthesized available literature and modeling results on the impacts of damming on fisheries production, and estimated the consequent impact on inadequate intakes of protein, zinc, niacin, thiamin, riboflavin, and calcium, as well as potential vulnerability to losses of dietary iron. Hydropower development could restrict access to subsistence fish from the Mekong River Basin and lead to increased risk of nutritional deficiencies in Cambodia and the LMB. Our median estimates suggest that by 2030, relative to 2010, inadequate intakes could lead to an increased population at risk of nutritional deficiencies in the LMB by 0.21 to 2.23 million people for protein, 0.12 to 1.17 million people for zinc, 0.41 to 1.58 million people for niacin, 0.47 to 0.87 million people for thiamin, 0.70 to 2.31 million people for riboflavin, and approximately 10,000 people for calcium. This increased population at risk is additional to those currently malnourished. We then calculated that the average iron intake of many age-sex groups (constituting 58% of the population) will be below 150% of their EAR in 2030, indicating a potential risk of increased inadequate iron intake. Fish is the main source of animal source foods and critical micronutrients in the LMB. In the absence of mitigation efforts, any reductions in fishery production could increase already high levels of nutrient deficiency, creating a widespread risk of nutrition insecurity.
Growing human populations and economic development drive increases in global demand for food and energy, interlinked sectors that heavily rely on limited water resources (World Economic Forum, 2011). Dams produce hydropower, provide water for food production, modify water flows, and restructure fisheries, placing them at the center of the challenge to sustainably manage the food-energy-water (FEW) nexus (D'Odorico et al., 2018). With 3,700 large hydropower dams planned worldwide (Zarfl et al., 2015), understanding FEW tradeoffs is increasingly important.
At the forefront of the FEW nexus and large-scale damming conversations is the Mekong River, which starts in the Tibetan Plateau and empties into the South China Sea. The Upper Basin is primarily in China and Myanmar, and the Lower Mekong Basin (LMB) spreads through Lao PDR, Thailand, Cambodia, and Vietnam (Mekong River Commission, 2010). The Mekong is a key trade route, provides irrigation water and electricity, and is one of the world's most productive inland fisheries (Baran and Myschowoda, 2009). With over 100 dams planned in the LMB, including eleven planned on the mainstream by 2020 (Dugan et al., 2010), electricity availability is projected to increase by 900% (Pittock et al., 2016).
However, these dams severely affect fisheries by disrupting flows, sediment load, migration routes, and downstream flood regimes. The quantity and timing of water flows shape Lower Mekong Basin (LMB) fisheries. Flood pulses are particularly integral for the ecology of Tonle Sap Lake (Kummu and Sarkkula, 2008), a major regional fishery, which receives 53.5% of its flow from the Mekong (Kummu et al., 2014) and supports the fisheries of one million people (Stone, 2011). By reducing downstream sediment loads, dams restrict nutrient inputs, limiting the primary production that supports fishery production (Baran et al., 2001). Dams also block spawning routes for migratory fish, which constitute at least 35% of fish caught in the Mekong (ICEM (International Centre for Environmental Management), 2010; Stone, 2011). As a result, the LMB provides an important case for studying the effects of damming on nutrition from lost fisheries, an often-overlooked cost to dam construction.
Reductions in fishery production will affect the nearly 50 million people dependent on LMB fisheries for food and livelihoods (Orr et al., 2012; Pittock et al., 2017). Migratory fish groups that are highly vulnerable to damming represent $1.4 billion of the $2.4–3 billion of first-sale price values in the LMB (Barlow et al., 2008). Throughout the LMB, fish are a critical part of the diet (Hortle, 2007; Buoy et al., 2009), and a major source of protein, iron, zinc, calcium, B vitamins, and other micronutrients (Roos et al., 2007a,b; Chamnan et al., 2009; Golden et al., 2016; Vilain and Baran, 2016; Vilain et al., 2016). However, the contribution of fish to nutrition security is often overlooked in decision-making.
Without targeted mitigation efforts, reductions in fishery production seriously impact nutrition and malnutrition-related diseases (ICEM (International Centre for Environmental Management), 2010; Pittock et al., 2016, 2017). While people will adapt to lower fish production in a variety of ways and to varying degrees, thus offsetting some nutritional losses, the capacity for adaptation is uncertain. The objective of this study is to quantify the number of nutritionally insecure people (i.e., those at risk of nutritional deficiencies)—that is, the population estimated to be below the Estimated Average Requirement (EAR) cut-point for adequate intake of nutrients—when fish consumption changes from Mekong damming.
Previous studies estimated that damming may decrease LMB fishery production by 4 to 42% (ICEM (International Centre for Environmental Management), 2010; Mekong River Commission, 2010; IFReDI (Inland Fisheries Research Development Institute), 2013). These studies range in methodology from process-based models of hydrology, fish populations, and ecology (Baran et al., 2007; ICEM (International Centre for Environmental Management), 2010; Ziv et al., 2012; IFReDI (Inland Fisheries Research Development Institute), 2013) to causal loop diagrams (Pittock et al., 2016) and time series modeling (Sabo et al., 2016). Studies have also estimated protein losses associated with fishery production reductions (ICEM (International Centre for Environmental Management), 2010; Pittock et al., 2016, 2017) and the water and land resources required to replace fish catch declines with terrestrial foods (Orr et al., 2012). However, without quantifying micronutrient losses or comparing nutrient losses to nutrient requirements, the literature may underestimate the human health risks of hydropower development.
In this work, we calculated population-level nutrient (protein, zinc, niacin, thiamin, riboflavin, and calcium) requirements for Cambodia and the entire LMB from existing demographic data. We used published fishery production projections to calculate nutrient supply from fishery production. By comparing nutrient requirements and fishery nutrient supply, we calculated the increase in prevalence of those at risk of nutrient deficiencies in 2030 relative to 2010. For iron, we quantified the population currently near the threshold of deficiency with the population near the same threshold as fish availability shrinks under damming scenarios. This analysis is the first study to connect projected fishery declines under damming to a potential increased risk of nutrient deficiencies, a critical step to fully accounting for the human health consequences of damming.
Our analysis of nutritional insecurity from damming in the LMB utilizes GENuS, a recently constructed database of fish nutrient content, to enrich previous modeling efforts on the impacts of damming on fish catch. To identify published estimates of fish catch declines under different damming scenarios, we first reviewed gray literature and government reports that we located during an in-country consultation in Cambodia. We then included journal and white paper projections of fisheries production declines attributed to damming. We searched Web of Science for publications in English with projections that met the following criteria: (1) included quantitative estimates of fish catch reductions for specific damming scenarios; (2) had a geographic scope of either Cambodia or the entire LMB, as defined by ICEM (International Centre for Environmental Management) (2010); and (3) was published in 2010 or after to ensure that the most recent data were being used. Of the eleven studies considered, only three met these criteria. Unused publications calculated reductions in fisheries production as a function of hydrological factors (Sabo et al., 2016, 2017) or in geographical areas that are not comparable to Cambodia or the LMB (Baran et al., 2007; Ziv et al., 2012). Changes within ecosystems or bodies of water that do not align geographically with the scope of this study cannot easily be scaled up or scaled down to produce comparable results.
The studies included in our analysis are: (ICEM (International Centre for Environmental Management), 2010; Mekong River Commission, 2010; IFReDI (Inland Fisheries Research Development Institute), 2013), the first for Cambodia and the latter two for the entire LMB (Table 1). Each model included multiple projections, representing various damming scenarios. We classified damming scenarios according to damming extent, where “none” represents construction of no mainstream dams, “max” represents construction of eleven mainstream dams, and “some” represents construction of an intermediate number of dams, which varies across the studies.
The IFReDI (Inland Fisheries Research Development Institute) (2013) estimated 2011 baseline fisheries production in Cambodia as 889,000 tons/year (damming extent: none). With only one (the Stung Treng dam) of the possible 11 dams constructed in the LMB, fisheries production is projected to decrease by 34,000 tons/year (4%) in Cambodia by 2030 (damming extent: some; Table 2). With all 11 mainstream dams, fisheries production is projected to decrease by 183,000 tons/year (21%) (damming extent: max; Table 2). ICEM (International Centre for Environmental Management) (2010) estimated 2,000 baseline fisheries production in LMB as 2,100,000 tons/year, similar to the Inland Fisheries Research and Development Institute baseline estimates for Cambodia on a tons/year/km2 basis. Without additional mainstream dams in 2030, this study estimated fisheries production to decline by 480,000 tons/year (23%) in the LMB (damming extent: none; Table 2). Fish catch was estimated to decline by 600,000 and 680,000 tons/year with the addition of six mainstream dams built upstream of Vientiane and nine mainstream dams operating upstream of Khone Falls, respectively (damming extent: some; Table 2). With 11 mainstream dams by 2030, fisheries production is estimated to decline by 880,000 tons/year (42%) (damming extent: max; Table 2). The Mekong River Commission (2010) estimated year 2000 baseline fisheries production in the LMB as 2,300,000 tons/year (damming extent: none). With construction of 11 mainstream dams by 2030, the study estimated fisheries production could decrease by 900,000 tons/year (41%) (damming extent: max; Table 2). Taken together, fishery catch is projected to decline by 4–33% with some damming and 21–42% with all 11 proposed dams.
The Estimated Average Requirement (EAR) is the amount of a given nutrient that is assumed to be sufficient to meet the needs of 50% of the population, and is used as an input in our deficiency risk calculations (section Prevalence of Inadequate Intake). EARs are generally calculated based on the reference nutrient intake (RNI)—the amount sufficient to meet the needs of ~97% of the population—and an RNI-to-EAR conversion factor. RNIs for calcium, thiamin, riboflavin, niacin, and iron are provided for each age and sex group by the World Health Organization (WHO) Food Agriculture Organization (FAO) of The United Nations (2004) and the conversion factors are provided separately by the World Health Organization (2006). For some age-sex groups, these conversion factors for iron did not exist, and we used the relationship between EAR and RNI in the Institute of Medicine's guide to nutritional requirements (Institute of Medicine of the National Academies, 2006) to derive them.
EARs for iron range from 2.2 to 42.8 mg/day (World Health Organization (WHO) Food Agriculture Organization (FAO) of The United Nations, 2004), depending on age sex, and iron availability in the diet. The capability of the body to absorb iron is controlled by a range of factors, including foods and nutrients eaten with the iron in the same meal (e.g., ascorbic acid, calcium, or polyphenols), the type of iron consumed (heme or non-heme iron), as well as each person's overall iron status or concurrent disease load (Lynch et al., 2018). However, we do not have sufficient data for several of these factors within GENuS (polyphenols, alcohol) to allow for explicitly calculating the absorbable iron supply from the diet. Therefore, we instead account for the differences in dietary bioavailability of iron by estimating the differences in each country's iron requirement based on the bioavailability of their diet. Each country was assigned to a bioavailability category based on criteria meant to serve as proxies of the relevant components of the diet which control iron absorption: overall meat, fruit, and vegetable intake. These criteria were derived based on guidance from Hurrell and Egli (2010) and Hallberg and Rossander-Hultén (1991), and previously applied to a similar analysis (Golden et al., 2016). The exact consumption thresholds and the corresponding bioavailability categories are described in detail in Supplementary Table 1.
Physiological zinc requirements were estimated by the International Zinc Nutrition Consultative Group (IZiNCG) et al. (2004). We estimated protein requirements following a methodology first used by Medek et al. (2017) and refined by Smith and Myers (2018). Because protein requirements are determined as grams per kilogram of body weight, we first estimated the baseline body weight for all age-sex groups in each country. For adults, we used mean height for each age, sex, and country (NCD Risk Factor Collaboration (NCD-RisC), 2016), paired with WHO's minimally acceptable BMI of 18.5 to estimate baseline weight. For adolescents and children over five, we used WHO height-for-age growth curves (World Health Organzation Multicentre Growth Reference Study Group, 2006), paired with the measured adult height in each country, to extrapolate the corresponding height of the younger age groups. For children under five where no height data was available, we used 50th percentile weight-for-age to determine inadequate protein intake (World Health Organzation Multicentre Growth Reference Study Group, 2006). The weight of each age-sex group was then multiplied by the corresponding WHO protein requirement to derive final requirements by demographic group (Joint FAO/WHO/UNU Expert Consultation, 2007).
For nearly all nutrients for which we estimate the risk of deficiency, the requirements for pregnant and lactating women are greater than those of non-pregnant or non-lactating women of the same age. The only exception is calcium for lactating women, for which the requirement is the same as for non-lactating women. For all other nutrients, we estimated the additional requirements of these women using the 2010 age-specific fertility rate from the United Nations World Population Prospects for each age category (United Nations, 2017). We multiplied the birth rate by the fraction of the year constituting the average gestational length (40 weeks) to estimate the population of pregnant women requiring additional nutrition. We estimated the population of lactating women by multiplying the median duration of breastfeeding in each country from the WHO Global Data Bank on Infant and Young Children Feeding (World Health Organization, 2018a); Laos and Thailand had missing data, and thus these countries were assigned the regional average.
To identify the nutritional adequacy in the diet after damming scenarios relative to the baseline, we needed to first estimate the total protein, zinc, niacin, thiamin, riboflavin, and calcium coming from both fish and from the total diet. Fish also provide many other important nutrients in the diet (Vilain and Baran, 2016; Vilain et al., 2016), but we were unable to include them in our analysis because we either lacked sufficient data on nutrient intake (vitamins B12, iodine, omega-3 fatty acids) or there were no established nutritional requirements (copper, manganese). Furthermore, fish can also significantly contribute to vitamin A intake (Roos et al., 2007a), but our dietary intake estimates were not sufficiently precise to be able to identify which parts of the fish were eaten in each country. This information exerts a large influence on any estimate of how much vitamin A is provided via fish, as eyes and liver contain nearly all the vitamin A in a given fish. Therefore, we did not feel justified in estimating vitamin A given the large uncertainties in our data.
We used the Global Expanded Nutrient Supply (GENuS) model to assign nutrient supplies for each age-sex group in 2010. The methodology of GENuS is described in detail in Smith et al. (2016) (Smith et al., 2016) and datasets are available at the Harvard Dataverse (Smith, 2016). To briefly summarize the construction of GENuS, the dataset uses FAO food balance sheet data, combined with additional production and trade data, to estimate the food supply for 225 foods in 151 countries since 1961. Of the 225 foods and food groups in GENuS, seven of these—freshwater fish, demersal fish, pelagic fish, crustaceans, cephalopods, molluscs, marine mammals, and other marine fish—capture the contributions of fish and seafood to the diet. Per capita food supplies are then paired with their corresponding nutrient densities from six regional food composition tables to estimate the nutrient supply in the diet across 23 different nutrients. Nutrients were only included in GENuS if they were found in more than one table, but most nutrients were found in all six. For this analysis of the four Mekong Basin countries, all nutrient density information was drawn from the Institute of Nutrition, Mahidol University (2014), which includes information on 71 different species of fish and seafood consumed regionally. Finally, the national nutrient supplies were then combined with data from the Global Dietary Database (GDD) (Global Nutrition Policy Consortium, 2018) on food consumption patterns by age and sex to generate detailed food and nutrient supply estimates across 34 demographic groups. GDD data were only used to identify different eating patterns of food groups between age and sex groups, but were not used to convert food supplies to food intakes due to the GDD data lacking full information on the entire diet. Because GENuS relies on FAO data to estimate the per capita supply of fish, and because it has been shown that freshwater fish catch data collected by FAO is particularly inaccurate (Bartley et al., 2015), we performed an additional correction factor on the supply of freshwater fish in the diet. A recent analysis by Fluet-Chouinard et al. (2018) re-derived the estimated catch of freshwater fish in many countries using a range of complementary data sources, particularly household expenditure surveys. We used the ratio between their corrected catch estimates and the primary FAO data and multiplied the supply of freshwater fish in each country by that value. Those factors were 1.48 (Cambodia), 3.02 (Laos), and 2.54 (Thailand). For Vietnam, Fluet-Chouinard et al. (2018) has insufficient data to derive corrected catch estimates, so we simply used the FAO values for freshwater fish supply. For comparison, we also ran a parallel set of analyses using the uncorrected GENuS estimates (Supplementary Table 2).
For zinc, we converted total zinc supplies to absorbable zinc to account for the absorption-inhibiting influence of phytate. We estimated paired phytate and zinc values for all foods in GENuS using a composite food composition table that was constructed using an array of data sources (Smith and Myers, 2018). For grains, we also accounted for the effect of processing and fermenting on each grain's zinc and phytate content. We used regional estimates of the percentage of grains processed and nutritional impact of processing from Wessells et al. (2012) and applied these to per capita food and nutrient supply estimates. We used the GENuS methodology to estimate dietary phytate and zinc from edible food supply, as well as associated uncertainties. We estimated absorbable zinc from dietary zinc and dietary phytate using the Miller equation (Miller et al., 2007; Hambidge et al., 2010). For protein, we followed established methodology (Medek et al., 2017) and assumed that plant-based protein was 80% digestible and animal-based protein was 95% digestible.
For all nutrients except iron, we estimated distributions of intra-individual intake to determine prevalence of inadequate intake. Distributions were assumed normal (in line with previous studies, e.g., Arsenault et al., 2015; Beal et al., 2017) and we estimated standard deviation using coefficients of variation (CV) based on nutrient: calcium (30%), zinc (25%), thiamin (30%), riboflavin (30%), and niacin (25%). For protein, we assumed that intake distributions were log-normal, with CV derived from a relationship between the Gini coefficient and the shape of the distribution. The methodology for CV derivation is further explained in Smith and Myers (2018) and Medek et al. (2017). The CV for each country is as follows: Cambodia (37%), Laos (47%), Thailand (50%), and Vietnam (46%).
Prevalence of inadequate intake was estimated using the EAR cut-point method (Institute of Medicine (US) Subcommittee on Interpretation Uses of Dietary Reference Intakes; Institute of Medicine (US) Standing Committee on the Scientific Evaluation of Dietary Reference Intakes, 2000). If certain key criteria are satisfied, the EAR cut-point method suggests that the number of people whose intake falls below the EAR is equivalent to the number of people who are inadequate in that nutrient. The necessary criteria are that each person's intake is independent of their requirement, the variability of intakes is greater than the variability of requirements, the distribution of requirements is approximately symmetrical, and the actual prevalence of inadequacy is neither very low nor high. For the nutrients studied here (except iron), each of these criteria hold, and we can apply this method. The cut-point method provides an estimate of the population that is consuming an inadequate amount of nutrients to meet their physiologic needs. Other intervening factors may affect whether a person with inadequate nutrient intake is physiologically deficient in that nutrient (e.g., consuming supplements, suffering additional illnesses), so we only characterize populations at risk of deficiency, not deficient, and this is the terminology we apply throughout the rest of our study. This method has been applied in numerous studies assessing the population-level status in the risk of dietary nutritional inadequacy (Wessells et al., 2012; Arsenault et al., 2015; Beal et al., 2017; Medek et al., 2017).
This calculation was performed using 2010 as a baseline. For each of the Mekong damming scenarios, we then reduced nutrient intake provided by fish by an amount proportional to the forecasted decline in per capita fish catch by 2030. The change in the prevalence of those at risk of deficiency for each scenario was measured relative to baseline, then multiplied by the population of each age-sex category in 2030 and by the proportion of each country that is located within the Mekong Basin.
We were unable to estimate the change in the risk of deficiency for iron, unlike with the other nutrients, because iron does not meet the criteria for the application of the cut-point method; namely, the distribution of requirements is not normal, particularly for menstruating women. Furthermore, we are unable to apply the probability method here because we do not have sufficient data to generate a distribution of requirements for the population, primarily because there are many confounding factors that influence the body's absorption of iron. However, we use GENuS data to provide estimates of iron contribution from seafood, as we know that iron is often sourced from Mekong fisheries (Roos et al., 2007b), calculating the sum of the populations of age-sex groups for whom the mean intake of the nutrient falls below 150% of their EAR, indicating a very rough measure of those that are close to inadequacy. This method was used similarly in a previous study as a proxy for risk of deficiency to lost fish in the diet (Golden et al., 2016).
Across the six reviewed damming scenarios (baseline plus five combinations of different damming futures), increasing human population and decreasing fishery production leads to hundreds of thousands to millions of newly nutritionally insecure people in Cambodia and the LMB by 2030, as compared to 2010 (Figure 1). For each nutrient, we present the medians of all projections simulating different damming scenarios and fish production (Figure 1). The scenarios range from a best case with only the Stung Treng dam as a mainstream dam to the worst case with 11 additional mainstream dams (Supplementary Table 2). The three main findings are: (1) all scenario projections are positive with error bars that do not include zero for all micronutrients and protein; (2) variance is higher in the worst-case scenarios; and (3) variance differs by nutrient (Figure 1). There is high uncertainty in the models, but all results indicate unequivocal and severe negative nutritional impacts. Results for the parallel model without the correction factor for freshwater fish are presented in Supplementary Table 3. All values reported below are from the model which includes the freshwater correction factor.
Figure 1. Estimated increases in 2030, relative to 2010, of the human population classified as nutrition insecure (i.e., below the EAR cut-point) for zinc, protein, niacin, thiamin, riboflavin, and calcium, according to each damming scenario projection. We classified each damming scenario according to the extent of additional mainstream damming (“none,” “some,” or “max”) which corresponds to 0, 1–9, and 11 additional dams. The white and gray panels break the bars into these categories. The error bars represent the 95% confidence interval for each scenario.
The median best- and worst-case scenarios indicated that, by 2030, there would be up to an additional 190,000–350,000 people newly at risk for deficiency for zinc in Cambodia and 90,000–910,000 in the LMB (Figure 1; Supplementary Table 3). For protein, as many as 340,000–660,000 more individuals would be at risk for deficiency in Cambodia and 160,000–1,690,000 more in the LMB (Figure 1; Supplementary Table 3). Projections for the maximum increase in the population at risk for niacin deficiency spanned 160,000–310,000 in Cambodia and 90,000–940,000 in the LMB (Figure 1; Supplementary Table 3). By 2030, we projected the population newly at risk for thiamin deficiency to increase by up to 70,000–130,000 people in Cambodia and 50,000–520,000 people in the LMB (Figure 1; Supplementary Table 3). Riboflavin projections indicated that there would be as many as 260,000–470,000 additional people at risk for deficiency in Cambodia and 140,000–1,380,000 in the LMB. For calcium, median projections indicated no additional risk of deficiency for Cambodia and an additional 10,000 people at risk in the LMB (Figure 1). This is largely because 99% of the Cambodian population is currently at risk for calcium deficiencies, not leaving much space to increase risk. Finally, we projected that up to 58% of the population (or 47 million people) in the LMB are in age-sex groups where their mean iron intake may be below 150% of their EARs by 2030, indicating a dire need to increase consumption of iron-rich foods such as fish. These populations include all age-sex groups in Cambodia and Laos, women aged 10–49 in Thailand and Vietnam, and men aged 10–24 and 50+ in Thailand.
In this study, we calculated the increased risk of potential nutritional deficiencies that could arise from hydropower damming across a broad range of micronutrients and protein for Cambodia and the entire LMB. Our results extend prior studies which have primarily considered reductions in fishery production alone or have focused only on the protein contribution of fish. By comparing human nutrient requirements and shifts in fishery nutrient supplies resulting from future damming scenarios, we calculated the increased risk of inadequate intakes in 2030 relative to 2010. This analysis demonstrates that, in the absence of mitigation efforts, all forms of damming will increase nutritional insecurity in Cambodia and the LMB. Our calculations also add nuance to the nutritional needs of people in this region, by accounting for varying nutritional demands by individuals of different ages and sexes, as well as increased nutritional demands during pregnancy and lactation.
By comprehensively estimating a broad suite of potential nutritional outcomes that could arise from damming, we provide a foundation for more precisely quantifying the health costs of hydropower development. Each of these risks of nutritional deficiency has consequent health impacts that lead to profound risks of disability and disease. For instance, protein deficiencies can influence almost all aspects of physiological functioning, and we estimated a median increase of up to 2.2 million people at risk of protein deficiency by 2030 in the LMB. Our estimates of protein deficits improve on previous calculations of protein reductions in the Mekong river basin (ICEM (International Centre for Environmental Management), 2010; Orr et al., 2012; Pittock et al., 2016, 2017) by incorporating multiple fishery projection estimates and relating the protein losses to actual population requirements (Pittock et al., 2016). Our results reinforce the previous findings that protein availability from fish catch will decrease and will broadly impact regional food security.
Beyond protein benefits, fish from the Mekong river basin provide significant micronutrient supplies to the region, especially iron and zinc. Iron-deficiency anemia is responsible for high maternal mortality rates, reduced school performance in children, and lost economic productivity (World Health Organization, 2018b). In fact, iron deficiency is estimated to account for 2.29% of all disability-adjusted life years (DALYs) lost in Cambodia and for 3.21% of all DALYs lost among Cambodian women (Hay et al., 2017). In 2030, we project that 58% of the population will have an average iron intake that is already lower than 150% of their estimated requirement, which will only worsen under projected damming scenarios.
Zinc is critical for immune function and preventing serious childhood illnesses such as diarrhea (National Institute of Health, 2018c). We predicted a median increase in those at risk for zinc deficiency for more than 1 million people in the LMB by 2030 relative to baseline. Niacin deficiency can lead to diarrhea, wasting, and dementia (World Health Organization (WHO) Food Agriculture Organization (FAO) of The United Nations, 2004). In the best-case scenario, by 2030, we estimate a potential increase of up to 1.5 million people at risk of niacin deficiency in the LMB. Under the worst-case damming scenarios, niacin deficiencies could reach an additional 4.4 million people (5.5% of the population within the LMB). Thiamin is essential for cell growth and development, and thiamin deficiency can cause weight loss, cognitive deficits, muscle weakness, and cardiovascular symptoms (National Institute of Health, 2018a). We estimate a median increase of more than 800,000 people at risk for thiamin deficiency in the LMB by 2030, which could lead to an array of health consequences. Maximal worst-case scenarios could lead to an additional 4.0 million people at risk of riboflavin deficiencies, known to contribute to an increased risk of anemia and cardiovascular diseases (Powers, 2003). Calcium deficiencies, leading to increased risk of reduced bone mass and osteoporosis (Nordin, 1997), are expected to increase the least of all modeled nutritional deficiency risks, with 370,000 people projected to be at risk of deficiency in maximal worst-case scenarios in the LMB.
Although we were unable to quantify vitamin A and B12 deficiency, we expect reduced fishery production to greatly impact vitamin intake in LMB. Vitamin B12 is only found in animal products, and recent estimates indicate that about 19% of the global population is at risk for deficiencies of nutrients that derive mostly from animal consumption (which includes vitamin B12) (Golden et al., 2016). Given that Cambodians obtain 80% of animal source foods intake from fish (Royal Government of Cambodia, 2010), we expect the population to have few other sources of vitamin B12, which is essential for blood cell formation and neurological functioning (National Institute of Health, 2018b). Although found in colorful fruits and vegetables, freshwater fish are very rich sources of vitamin A (Roos et al., 2007a). Without adequate consumption of vitamin A, immunity is compromised leading to increased risk of infectious disease and night blindness (Lim et al., 2012).
Increases in the risk of protein and micronutrient deficiencies are among a wide array of social costs related to hydropower development. There are also clear benefits. Hydropower can help satisfy the Mekong region's electricity demand, which is increasing at a rate greater than the rest of the world [ICEM (International Centre for Environmental Management), 2010]. Increased hydropower can enhance the region's trade, reduce its reliance on fossil fuels, and reduce greenhouse gas emissions for the power sector [ICEM (International Centre for Environmental Management), 2010]. Cambodia and Lao PDR also plan to export power, the revenue from which can develop education and health care [ICEM (International Centre for Environmental Management), 2010].
Our analysis is limited by risks of both over- and under-estimation. We may be underestimating the impact of fish catch declines on nutrition security because we are not accounting for indirect pathways to nutrition, such as through income generation. We may also be biasing our results by using nutrient supply data as a proxy for nutrient intake data, though the GENuS data apportions the FAO food supply data to age-sex groups using nationally representative food consumption survey recalls conducted in 187 countries (Smith et al., 2016). Although 24 h recalls are often seen as the gold standard for food consumption statistics, GENuS provides us with systematic data across the LMB that proxies consumption. Furthermore, we may also be underestimating the amount of fish in the diets based on comparison between FAO per capita fish availability and more detailed information on fish consumption (Hortle, 2007). There is also a possibility that we are overestimating the nutritional impacts from hydropower development because we do not consider replacement diets when fish is lost (e.g., through livestock, agriculture, or aquaculture development).
It is clear that dams positively contribute to other food production systems by storing irrigation water and creating habitat for aquaculture. Unfortunately, there are numerous barriers to adopting small-scale aquaculture practices (Beveridge et al., 2013; Béné et al., 2016; Richardson and Suvedi, 2018), making it unlikely that aquaculture could compensate for losses in fish catch in providing food for those vulnerable to malnutrition [ICEM (International Centre for Environmental Management), 2010]. Replacement of lost fish with other food sources is beyond the scope of this analysis, but is an important future direction for research. Some groups have even demonstrated, through randomized control trials, the importance of local fish food products in improving Cambodian nutrition (Sigh et al., 2018).
New hydropower management approaches may be able to reduce food-energy tradeoffs, particularly if efforts are made to retrofit hydropower development to mitigate environmental impacts (Arias et al., 2014). One recent study argues that operating dams to better match natural hydrologic regimes could increase fish catch by ~50% within 8 years, while producing the same amount of power (Sabo et al., 2017). Many experts doubt the analysis and interpretations of this study (Halls and Moyle, 2018; Williams, 2018), however, and other experts doubt that necessary investments will be made to retrofit the dams if they are constructed (Dugan et al., 2010). Regardless of the exact predictions of fish futures in the Lower Mekong, accounting for the full nutritional impacts of dams may incentivize prevention or mitigation actions. This type of evidence could also enable collective action from affected citizens to integrate fisheries management into decentralized development planning (Ratner et al., 2017).
Our study estimates the nutritional risk associated with declines in fisheries production driven by hydropower development. Millions of people are at risk for nutritional deficiencies if proper attention is not paid to the health consequences of damming. It is clear that fisheries are important for Cambodia's path to meeting the Sustainable Development Goals to eliminate poverty and ensure food security and nutrition (Ratner et al., 2017), as the region's fish provide employment and food for millions of people (Hortle, 2007; Orr et al., 2012). But in a region that is already stretched for nutritional resources, further damming has the potential to place millions of people at risk of new nutritional deficiencies. This is not a challenge unique to the Lower Mekong Basin but affects all major riverine systems in nutritionally vulnerable countries around the world.
CG, BV, and JG designed the study. AS, ES, and JG conducted the literature review, data extraction, and analysis and production of visualizations. MS and SM provided analytical support in estimating dietary intake and the contribution of fish. CG and BV visited the Lower Mekong to discuss these issues with local stakeholders, NGOs, and government officials who provided input on our data analysis and interpretation. CG and AS drafted the article. All authors contributed to revising and approving the manuscript.
This work was supported by the Wellcome Trust Our Planet, Our Health [Grant number: 106864MA] and the National Socio-Environmental Synthesis Center (SESYNC) under funding from National Science Foundation DBI-1052875.
The authors declare that the research was conducted in the absence of any commercial or financial relationships that could be construed as a potential conflict of interest.
We would like to thank Kathryn Fiorella for editing a draft of this manuscript, and Olivier Joffre, Eric Baran, Shakuntala Thilsted, and WorldFish Cambodia for their intellectual insights and foundational work in this subject area and for allowing us to spend time with their teams in the field in Cambodia.
The Supplementary Material for this article can be found online at: https://www.frontiersin.org/articles/10.3389/fsufs.2019.00093/full#supplementary-material
Arias, M. E., Piman, T., Lauri, H., Cochrane, T. A., and Kummu, M. (2014). Dams on mekong tributaries as significant contributors of hydrological alterations to the tonle sap floodplain in Cambodia. Hydrol. Earth Syst. Sci. 18, 5305–5315. doi: 10.5194/hess-18-5303-2014
Arsenault, J. E., Hijmans, R. J., and Brown, K. H. (2015). Improving nutrition security through agriculture : an analytical framework based on national food balance sheets to estimate nutritional adequacy of food supplies. Food Secur. 7, 693–707. doi: 10.1007/s12571-015-0452-y
Baran, E., and Myschowoda, C. (2009). Dams and fisheries in the Mekong basin. Aquat. Ecosyst. Health Manage. 12, 227–234. doi: 10.1080/14634980903149902
Baran, E., Starr, P., and Kura, Y. (2007). Influence of Built Structures on Tonle Sap Fisheries. Cambodia National Mekong Committee and the WorldFish Center, oPhnom Penh, 44.
Baran, E., Van Zalinge, N., Ngor, P. B., Baird, I., and Coates, D. (2001). Fish Resource and Hydrobiological Modelling Approaches in the Mekong Basin. ICLARM, Penang, Malaysia and the Mekong River Commission Secretariat, Phnom Penh, 60.
Barlow, C., Baran, E., Halls, A. S., and Kshatriya, M. (2008). How much of the Mekong fish catch is at risk from mainstream dam. Catch Cult. 14, 16–21.
Bartley, D. M., De Graaf, G. J., Valbo-Jørgensen, J., and Marmulla, G. (2015). Inland capture fisheries: status and data issues. Fish. Manage. Ecol. 22, 71–77. doi: 10.1111/fme.12104
Beal, T., Massiot, E., Arsenault, J. E., Smith, M. R., and Hijmans, R. J. (2017). Global trends in dietary micronutrient supplies and estimated prevalence of inadequate intakes. Food Secur. 12:4. doi: 10.1371/journal.pone.0175554
Béné, A., Arthur, R., Norbury, H., Allison, E. H., Beveridge, M., Bush, S., et al. (2016). Contribution of fisheries and aquaculture to food security and poverty reduction: assessing the current evidence. World Dev. 79, 177–196. doi: 10.1016/j.worlddev.2015.11.007
Beveridge, M. C. M., Thilsted, S. H., Phillips, M. J., Metian, M., Troell, M., and Hall, S. J. (2013). Meeting the food and nutrition needs of the poor: the role of fish and the opportunities and challenges emerging from the rise of aquaculture. J. Fish Biol. 83, 1067–1084. doi: 10.1111/jfb.12187
Buoy, R., Chamnan, C., and Thilsted, S. H. (2009). Fish is an integral part of the diet of the rural poor in Cambodia: results from fish consumption surveys. Asian Fish. Sci. 22, 445–452.
Chamnan, C., Shakuntala, H. T., Bouy, R., Lieng, S., Gerpacio, R. V., and Roos, N. (2009). The Role of Fisheries Resources in Rural Cambodia: Combating Micronutrient Deficiencies in Women and Children. Department of Fisheries Post-Harvest Technologies and Quality Control, Fisheries Administration, Ministry of Agriculture Forestry and Fisheries. Phnom Penh, 106.
D'Odorico, P., Frankel, D. K., Rosa, L., Carr, J., Chiarelli, D., Dell'Angelo, J., et al. (2018). The global food-energy-water Nexus. Rev. Geophys. 56:3. doi: 10.1029/2017RG000591
Dugan, P. J., Barlow, C., Agonstinho, A. A., Baran, E., Cada, G. F., Chen, D., et al. (2010). Fish migration, dams, and loss of ecosystem services in the Mekong basin. AMBIO 39, 344–348. doi: 10.1007/s13280-010-0036-1
Fluet-Chouinard, E., Funge-Smith, S., and McIntyre, P. B. (2018). Global hidden harvest of freshwater fish revealed by household surveys. Proc. Natl. Acad. Sci. U.S.A. 115, 7623–7628. doi: 10.1073/pnas.1721097115
Global Nutrition Policy Consortium (2018). The Global Dietary Database. Boston, MA: Tufts Friedman School of Nutrition Science and Policy. Available online at: http://www.globaldietarydatabase.org/ (accessed November 8, 2018).
Golden, C., Allison, E., Cheung, W., Dey, M., Halpern, B., Mccauley, D., et al. (2016). Nutrition: fall in fish catch threatens human health. Nature 534, 317–320. doi: 10.1038/534317a
Hallberg, L., and Rossander-Hultén, L. (1991). Iron requirements in menstruating women. Am. J. Clin. Nutr. 54, 1047–1058. doi: 10.1093/ajcn/54.6.1047
Halls, A. S., and Moyle, P. B. (2018). Comment on “designing river flows to improve food security futures in the lower mekong basin.” Science 361:6398. doi: 10.1126/science.aat1989
Hambidge, K. M., Miller, L. V., Westcott, J. E., Sheng, X., and Krebs, N. F. (2010). Zinc bioavailability and homeostasis. Am. J. Clin. Nutr. 91, 1478S−1483S. doi: 10.3945/ajcn.2010.28674I
Hay, S., Abajobir, A., Abate, K., Abbafati, C., Abbas, K., Abd-Allah, F., et al. (2017). Global, regional, and national Disability-Adjusted Life-Years (DALYs) for 333 diseases and injuries and Healthy Life Expectancy (HALE) For 195 countries and territories, 1990 – 2016: a systematic analysis for the global burden of disease study 2016. Global Health Metrics 290, 1260–1344. doi: 10.1016/S0140-6736(17)32130-X
Hortle, K. G. (2007). “Consumption and the yield of fish and other aquatic animals from the lower Mekong basin,” in MRC Technical Paper No. 16 (Ventiane: Mekong River Commission), 113.
Hurrell, R., and Egli, I. (2010). Iron bioavailability and dietary reference values. Am. J. Clin. Nutr. 91, 1461–1467. doi: 10.3945/ajcn.2010.28674F
ICEM (International Centre for Environmental Management) (2010). Strategic Environmental Assessment of Hydropower on the Mekong Mainstream. Summary of the Final Report. ICEM. Hanoi, 197.
IFReDI (Inland Fisheries Research and Development Institute) (2013). Food and Nutrition Security Vulnerability to Mainstream Hydropower Dam Development in Cambodia. Ministry of Agriculture, Forestry, and Fisheries. Phnom Penh, 47.
Institute of Medicine (US) Subcommittee on Interpretation and Uses of Dietary Reference Intakes; Institute of Medicine (US) Standing Committee on the Scientific Evaluation of Dietary Reference Intakes (2000). DRI Dietary Reference Intakes: Applications in Dietary Assessment. Washington, DC.
Institute of Medicine of the National Academies (2006). Dietary Reference Intakes: The Essential Guide to Nutrient Requirements. The National Academies Press. Washington, DC.
Institute of Nutrition Mahidol University. (2014). ASEAN Food Composition Database, Electronic Version 1. Thailand. Available online at: http://www.inmu.mahidol.ac.th/aseanfoods/composition_data.html
International Zinc Nutrition Consultative Group (IZiNCG), Brown, K. H., Rivera, J. A., Bhutta, Z., Gibson, R. S., King, J. C., et al. (2004). Assessment of the risk of zinc deficiency in populations and options for its control. Food Nutr. Bull. 25, S91–S204.
Joint FAO/WHO/UNU Expert Consultation (2007). Protein and Amino Acid Requirements in Human Nutrition. World Health Organization Technical Report Series. World Health Organization.
Kummu, M., and Sarkkula, J. (2008). Impact of the Mekong river flow alteration on the Tonle Sap flood pulse. AMBIO 37, 185–192. doi: 10.1579/0044-7447(2008)37[185:IOTMRF]2.0.CO;2
Kummu, M., Tes, S., Yin, S., Adamson, P., Józsa, J., Richey, J., et al. (2014). Water balance analysis for the tonle Sap Lake – floodplain system. Hydrol. Proc. 28, 1722–1733. doi: 10.1002/hyp.9718
Lim, S., Vos, T., Flaxman, A. D., Danaei, G., Shibuya, K., Adair-Rohani, H., et al. (2012). A comparative risk assessment of burden of disease and injury atributable to 67 risk factors and risk factor clusters in 21, 1990–2010: a systematic analysis for the global burden of disease study 2010. Lancet 380, 2224–2260. doi: 10.1016/S0140-6736(12)61766-8
Lynch, S., Pfeiffer, C. M., Georgieff, M. K., Brittenham, G., Fairweather-Tait, S., Hurrell, R. F., et al. (2018). Biomarkers of nutrition for development (BOND)—iron review. J. Nutr. 148, 1001S−1067S. doi: 10.1093/jn/nxx036
Medek, D. E., Schwarts, J., and Meyers, S. (2017). Estimated effects of future atmospheric CO2 concentrations on protein intake and the risk of protein deficiency by country and region. Environ. Health Perspect. 125:8. doi: 10.1289/EHP41
Mekong River Commission (2010). Assessment of Basin-Wide Development Scenarios Main Report. MRC Technical Note 11. Vientiane, 45.
Miller, L. V., Krebs, N. F., and Hambidge, K. M. (2007). A mathematical model of zinc absorption in humans as a function of dietary zinc. J. Nutr. 137, 135–141. doi: 10.1093/jn/137.1.135
National Institute of Health (2018a). Thiamin: Fact Sheet for Health Professionals. National Institute of Health. Available online at: https://ods.od.nih.gov/factsheets/Thiamin-HealthProfessional/ (accessed May 22, 2018).
National Institute of Health (2018b). Vitamin B12: Fact Sheet for Health Professionals. National Institute of Health. Available online at: https://ods.od.nih.gov/factsheets/VitaminB12-HealthProfessional/ (accessed April 7, 2018).
National Institute of Health (2018c). Zinc: Fact Sheet for Health Professionals. National Institute of Health. Available online at: https://ods.od.nih.gov/factsheets/Zinc-HealthProfessional/ (accessed May 22, 2018).
NCD Risk Factor Collaboration (NCD-RisC) (2016). A century of trends in adult human height. eLife 5:e13410. doi: 10.7554/eLife.13410
Nordin, B. E. (1997). Calcium and osteoporosis. Nutrition 13, 664–686. doi: 10.1016/S0899-9007(97)83011-0
Orr, S., Pittock, J., Chapagain, A., and Dumaresq, D. (2012). Dams on the Mekong river: lost fish protein and the implications for land and water resources. Glob. Environ. Change 22, 925–932. doi: 10.1016/j.gloenvcha.2012.06.002
Pittock, J., Dumaresq, D., and Bassi, A. (2016). Modeling the hydropower–food nexus in large river basins: a Mekong case study. Water 8, 1–18. doi: 10.3390/w8100425
Pittock, J., Dumaresq, D., and Orr, S. (2017). The Mekong river: trading off hydropower, fish, and food. Reg. Environ. Change 17, 2443–2453. doi: 10.1007/s10113-017-1175-8
Powers, H. J. (2003). Riboflavin (Vitamin B-2) and health. Am. J. Clin. Nutr. 77, 1352–1360. doi: 10.1093/ajcn/77.6.1352
Ratner, B. D., So, S., Mam, K., Oeur, I., and Kim, S. (2017). Conflict and collective action in tonle sap fisheries: adapting governance to support community livelihoods. Nat. Resour. Forum 41:2. doi: 10.1111/1477-8947.12120
Richardson, R. B., and Suvedi, M. (2018). Assessing the potential for small-scale aquaculture in Cambodia. Environments 5:76. doi: 10.3390/environments5070076
Roos, N., Chamnan, C., Deap, L., Jakobsen, J., and Thilsted, S. H. (2007a). Freshwater fish as a dietary source of vitamin A in Cambodia. Food Chem. 103, 1104–1111. doi: 10.1016/j.foodchem.2006.10.007
Roos, N., Thorseng, H., Chamnan, C, Larsen, T., Gondolf, U. H., Bukhave, K., et al. (2007b). Iron content in common cambodian fish species: perspectives for dietary iron intake in poor, rural households. Food Chem. 104, 1226–1123. doi: 10.1016/j.foodchem.2007.01.038
Royal Government of Cambodia (2010). The Strategic Planning Framework For Fisheries: 2010 – 2019. Phnom Penh, 59.
Sabo, J. L., Ruhi, A., Holtgrieve, G. W., Elliot, V., Arias, M. E., Bun Ngor, P., et al. (2017). Designing river flows to improve food security futures in the lower Mekong basin. Science 358:eaao1053. doi: 10.1126/science.aao1053
Sabo, J. L., Ruhi, A., Holtgrieve, G. W., Elliott, V., and Arias, M. (2016). Assessing hydrologic drivers of fisheries and forecasting future catches. Catch Cult. 22, 24–27.
Sigh, S., Roos, N., Chamnan, C., Laillou, A., Prak, S., and Wieringa, F. T. (2018). Effectiveness of a locally produced, fish-based food product on weight gain among cambodian children in the treatment of acute malnutrition: a randomized controlled trial. Nutrients 10:7. doi: 10.3390/nu10070909
Smith, M. (2016). Nutrient Totals by Age and Sex (2011). Harvard Dataverse, V4. doi: 10.7910/DVN/XIKNDC
Smith, M., and Myers, S. (2018). The risk of anthropogenic CO2 emissions on global human nutrition. Nat. Clim. Chang. 8, 834–839. doi: 10.1038/s41558-018-0253-3
Smith, M. R., Micha, R., Golden, C. D., Mozaffarian, D., and Myers, S. (2016). Global Expanded Nutrient Supply (GENuS) model: a new method for estimating the global dietary supply of nutrients. PLoS ONE. 11:e014697. doi: 10.1371/journal.pone.0146976
United Nations, D. P. D. (2017). World Population Prospects: 2017 Revision. Available online at: https://esa.un.org/unpd/wpp/
Vilain, C., and Baran, E. (2016). Nutritional and Health Value of Fish: The Case Of Cambodia. Inland Fisheries Research and Development Institute (Fisheries Administration) and WorldFish. Phnom Penh, 45.
Vilain, C., Baran, E., Gallego, G., and Saray, S. (2016). Fish and the nutrition of rural Cambodians. Asian J. Agric. Food Sci. 4, 26–34.
Wessells, K. R., Singh, G. M., and Brown, K. H. (2012). Estimating the global prevalence of inadequate zinc intake from national food balance sheets: effects of methodological assumptions. PLoS ONE. 7:e50565. doi: 10.1371/journal.pone.0050565
Williams, J. G. (2018). Comment on “Designing river flows to improve food security futures in the lower mekong basin.” Science. 361:eaat1225. doi: 10.1126/science.aat1225
World Health Organization (2006). Guidelines on Food Fortification With Micronutrients. WHO, Geneva.
World Health Organization (2018a). Infant and Young Child Feeding Data By Country. World Health Organization. Available online at: http://www.who.int/nutrition/databases/infantfeeding/countries/en/ (accessed May 13, 2018).
World Health Organization (2018b). Micronutrient Deficiencies. World Health Organization. Available online at: http://www.who.int/nutrition/topics/vad/en/ (accessed February 22, 2018).
World Health Organization (WHO) and Food and Agriculture Organization (FAO) of The United Nations (2004). Vitamin and Mineral Requirements in Human Nutrition: 2nd Edn. WHO/FAO. Bangkok, 362.
World Health Organzation Multicentre Growth Reference Study Group (2006). WHO Child Growth Standards: Length/Height-for-Age, Weight-for-Age, Weight-for-Length, Weight-for-Height and Body Mass Index-for-Age: Methods And Development. WHO. Geneva, 312.
Zarfl, C., Lumsdon, A. E., Berlekamp, J., Tydecks, L., and Tockner, K. (2015). A global boom in hydropower dam construction. Aquat. Sci. 77, 161–170. doi: 10.1007/s00027-014-0377-0
Keywords: food security, micronutrient deficiencies, fisheries, malnutrition, dams, health impact assessment
Citation: Golden CD, Shapero A, Vaitla B, Smith MR, Myers SS, Stebbins E and Gephart JA (2019) Impacts of Mainstream Hydropower Development on Fisheries and Human Nutrition in the Lower Mekong. Front. Sustain. Food Syst. 3:93. doi: 10.3389/fsufs.2019.00093
Received: 10 February 2019; Accepted: 03 October 2019;
Published: 25 October 2019.
Edited by:
Amy Ickowitz, Center for International Forestry Research, IndonesiaReviewed by:
Jessica Evelyn Raneri, Bioversity International, ItalyCopyright © 2019 Golden, Shapero, Vaitla, Smith, Myers, Stebbins and Gephart. This is an open-access article distributed under the terms of the Creative Commons Attribution License (CC BY). The use, distribution or reproduction in other forums is permitted, provided the original author(s) and the copyright owner(s) are credited and that the original publication in this journal is cited, in accordance with accepted academic practice. No use, distribution or reproduction is permitted which does not comply with these terms.
*Correspondence: Christopher D. Golden, Z29sZGVuQGhzcGguaGFydmFyZC5lZHU=
Disclaimer: All claims expressed in this article are solely those of the authors and do not necessarily represent those of their affiliated organizations, or those of the publisher, the editors and the reviewers. Any product that may be evaluated in this article or claim that may be made by its manufacturer is not guaranteed or endorsed by the publisher.
Research integrity at Frontiers
Learn more about the work of our research integrity team to safeguard the quality of each article we publish.