- 1Sustainable Seed Systems Lab, Department of Crop and Soil Sciences, Washington State University, Pullman, WA, United States
- 2Bread Lab, Department of Crop and Soil Sciences, Washington State University, Burlington, WA, United States
- 3Agroecology Lab, Department of Crop and Soil Sciences, Washington State University, Pullman, WA, United States
- 4Department of Crop and Soil Sciences, Washington State University, Pullman, WA, United States
Annual plants are currently the dominant growth habit for grain production systems but often create agroecosystems with negative environmental consequences. Developing grains with a perennial growth habit provides an opportunity to produce staple crops in a more environmentally beneficial manner. Amphiploids of perennial tall wheatgrass (Thinopyrum elongatum) and common annual wheat (Triticum aestivum) have been produced for wheat-like traits while exhibiting post-sexual cycle regrowth (PSCR). Here we report results from two experiments at two locations in the Palouse region of the U.S. Pacific Northwest (PNW) designed to (1) evaluate a subset of the current perennial grain germplasm base and (2) test post-harvest management strategies on key perennial growth habit traits. The first experiment evaluated 18 putative perennial amphiploid lines, two annual wheat varieties, and one intermediate wheatgrass variety (Thinopyrum intermedium) for yield, PSCR, key agronomic traits, and grain quality characteristics. Results from this trial showed mean yields ranged from 713 to 2874 kg/ha among the amphiploids and 1,627 to 6,867 kg/ha with the annual wheat varieties. Variation across the amphiploids was found for key agronomic and quality traits, indicating potential for continued selection and improvement. When compared to the two annuals, the four highest-yielding amphiploids produced the same yield at one location but 50–60% less at the other. Amphiploids were generally taller with increased tiller number, flowered later, had lower leaf chlorophyll content, and decreased threshability, compared to annual varieties. Regarding grain qualities, amphiploids had lower test weights and thousand kernel weights, smaller kernel diameters, lower starch content, and higher protein, ash, and fiber. The second experiment investigated the effect of post-harvest residue management on PSCR and winter survival of two amphiploids using mowing, burning, and a control. Mowing the residue and PSCR significantly increased winter survival across both amphiploids at one location from 3% in the control to 63% in the mowed treatment. Burning the residue did not improve survival. Our results address three important challenges in perennial grain development in the Palouse region: selecting a stable perennial habit, increasing grain yield, and improving marketable grain quality traits. Each of these challenges will require significant research efforts by plant breeders and agronomists prior to widespread adoption of perennial grains in the Palouse region of the PNW.
Introduction
The Palouse region of the Pacific Northwest is sui generis; a high-yielding, rainfed grain-producing area with a history of some of the highest dryland grain yields and most severe soil erosion rates in the United States (Steiner, 1987; Duffin, 2007). The Palouse region is temperate with warm, dry summers (Köppen Classification Csb), and an average annual precipitation ranging from 280 to 660 mm (Hall et al., 1999). Soils are loessial in origin and generally rich and deep. Considerable evidence suggests conventional cereal-legume systems in the Palouse have degraded soil health and increased soil pollution (Saxton et al., 2000).
The earliest grasses in the Poaceae family were perennial and the perennial growth habit remains the most prevalent, with just 348 of the 870 genera in the Poaceae family containing annual species (Watson et al., 2017). In contrast, staple cereal grains, such as wheat, rice, and maize, are almost entirely annuals, and therefore must be replanted each year from seed. Compared to perennial plants, annuals typically have shallower rooting depths and lower root densities (Glover et al., 2010b). Even with crop management advances, such as no-tillage practices, these traits limit their access to nutrients and water, leave croplands more vulnerable to soil degradation and erosion, and make annual plants less resilient to the increased environmental stress expected from climate change (Glover and Reganold, 2010).
Converting our grain production systems from annual to perennial has been proposed to mitigate erosion and improve long-term food security and soil health (Glover et al., 2010b). Perennial plants tend to allocate a higher proportion of photosynthates into the roots and associated rhizosphere than annuals (DuPont et al., 2014; Marshall et al., 2016). The continuous presence of proliferative live roots reduces the risk and rate of erosion, increases soil organic matter, enhances invertebrate community structure, and improves overall soil ecosystem services (Glover et al., 2010a; DuPont et al., 2014; Marshall et al., 2016).
Two predominant and contrasting approaches are currently used to produce wheat-related perennial grains. One method is to domesticate an existing perennial grass for wheat-like traits, such as free threshing, non-shattering, and higher yielding with end-use qualities useful for the production of foods, such as bread and beer (Cox et al., 2010). Although by nature a gradual process, breeders are making excellent progress utilizing this domestication-based method. For example, “Kernza™” is an intermediate wheatgrass variety (Thinopyrum intermedium L., 2n = 6x = 42) that has been recently released as an agronomically improved grain by breeders at The Land Institute (Salina, KS). Current challenges with Kernza™ and other perennial grain germplasm include increasing seed size and yield, which are often significantly lower than annual wheat (Hayes et al., 2012), and maintaining yield stability in subsequent years after establishment (Jakumar et al., 2012; Vico et al., 2016; Jungers et al., 2017).
The second method is to hybridize annual wheat with a perennial relative, most often a species in the Thinopyrum genus, though other potential donors of the perennial growth habit trait exist and could be more fully exploited (Lammer et al., 2004; Murphy et al., 2007; Curwen-McAdams et al., 2017; Piaskowski et al., 2017). This forms a Triticum aestivum × Thinopyrum spp. amphiploid (either complete or partial amphiploid). The hybridized annual grain × perennial relative method was used at Washington State University to produce the lines in this study (Murphy et al., 2009b). The initial transgeneric crosses were made with the spring wheat “Chinese Spring” (2n = 6x = 42) × Thinopyrum elongatum L. (2n = 2x = 14). These amphiploid hybrids were then backcrossed to regionally adapted annual winter wheat varieties, including “Madsen” (2n = 6x = 42) (Allan et al., 1989). The amphiploid plants produced were selected for annual wheat-like traits, such as large seeds, high yields, and improved threshability, and perennial traits, most notably post-sexual cycle regrowth (PSCR). PSCR is a necessary first step in the development of novel germplasm with the perennial habit (Lammer et al., 2004; Murphy et al., 2009b).
In addition to PSCR, another critical trait in the development of perennial grain amphiploids is post-harvest winter survival. Many of the PSCR tillers in the amphiploid lines are reproductive rather than the vegetative tillers common in perennial grasses (including Th. elongatum). Autumn growth in perennial grasses focuses on the accumulation of essential cold acclimation components, such as non-structural carbohydrates and specialized proteins and enzymes at the crown, required to survive the winter and regrow the next spring (Sandve et al., 2011; Ergon, 2017). Many of the WSU T. aestivum × Th. elongatum lines do not accumulate enough non-structural carbohydrates to survive the second and subsequent winters after planting (Greco, 2004). These amphiploid lines accumulate some non-structural carbohydrates in the crown; however, at lower levels than in a true perennial like Thinopyrum. Greco (2004) showed that 20.7% of the total non-structural carbohydrates in the plant had accumulated in the crowns of Th. elongatum at senescence, compared to 7.7% found in amphiploid lines with the same pedigree used in this study, and the 7.1% found in Chinese Spring. In addition, the concentration of fructans in the non-structural carbohydrates was shown to be considerably higher in Th. elongatum than either Chinese Spring or the amphiploid lines (Greco, 2004).
When growing perennial grasses for forage, best management practices revolve around properly removing or grazing plant material at the ideal growth stage and time for the region and crop phenotype (Gautier et al., 1999; Dickeduisberg et al., 2017). If plants are left without being grazed or hayed, the crown becomes crowded, thereby limiting light penetration into the crown, and young tillers are held back from growth (Wan and Sosebee, 1998). Alternatively, if the grass is cut too short or too frequently, it can negatively impact the quality of the sward (Dickeduisberg et al., 2017). Prescribed grazing could be an effective tool for removing unwanted or dead plant material, which would enable the perennial grain to be used as a dual-purpose crop. Swathing and removing the material as a hay crop would be another viable dual-purpose use. If livestock were not present in the region, mowing, flailing, or burning the residue are additional options for the removal of dead plant material.
The hypothesis of this study was that some perennial amphiploid lines have agronomic and quality traits similar to common annual wheat varieties and that various post-harvest residue management strategies of amphiploids have significantly different effects on their winter survival. Specific objectives of this study were two-fold: (i) evaluate agronomic and quality traits of 18 putatively perennial amphiploid lines, two commonly grown annual winter wheat varieties, and one perennial intermediate wheatgrass variety; and (ii) examine the effect of post-harvest residue management strategies, in particular mowing and burning of grain stubble, on PSCR and subsequent, second-year winter survival. Irrespective of their perennation, this germplasm is uniquely recherché and may possess attributes that contribute to more robust agroecological food production systems.
Materials and Methods
Study Areas
The trials took place in two locations, Tukey and Higbee, in the higher rainfall area (~530 mm) of the Palouse region in Washington State. The experimental field at Tukey Organic Farm at Washington State University in Pullman (46° 44′ N, 117° 07′40″ W) had a well-drained Palouse silt loam soil (fine-silty, mixed, superactive, mesic Pachic Ultic Haploxerolls) and was previously cropped with spring peas in 2015 that were incorporated as a green manure. At Higbee, a conventional, minimum tillage on-farm site located three miles north of Albion (46° 49′19″ N, 117° 12′1″ W), the experimental field had a Latah silt loam soil (fine, mixed, superactive, mesic Xeric Argialbolls), which was previously cropped with spring canola. Pre-trial examination of the two experimental fields showed that all soil-forming factors, such as microclimate, soil profile development, and organic matter content, were the same across each of the two fields (Reganold, 2013).
Weather data for Tukey was taken at Pullman Central, located 1 km from the experimental location. The Pullman NE station was the closest weather station to the Higbee location (10 km away). A base temperature of 0°C was used to calculate growing degree days (GDD), with the day of planting as the starting point.
Experimental Design and Data Collection
Variety Trial
The pedigree of the ~F10 amphiploid lines developed at WSU and used in this study is Th. elongatum/Chinese Spring//Madsen (Murphy et al., 2009a). The variety trial was planted in a randomized complete block design (RCBD) with four replicates at each location. The 21 genotypes included 18 amphiploid lines; two annual wheat varieties, the soft white winter wheat “Jasper” released by Washington State University (Carter et al., 2017) and the hard red winter wheat “Norwest 553” (PI655030) released in 2007 by Oregon State University; and “Kernza” (Th. intermedium L.) (Van Tassel and DeHaan, 2013). Plots were 1.2-m wide by 4.9-m long and planted with a Wintersteiger TRM plot planter (Wintersteiger Inc., Ried im Inndreis, Austria) on 15 October 2015. The variety trial was seeded with three rows per plot with a 0.46-m spacing between rows. Because of the difference in seed size between the wheat and amphiploid lines compared to Kernza™, the Kernza™ was planted by hand just below the surface. Plots were fertilized at a rate of 1,120 kg/ha of Nutri-rich 4-3-2 with 7% calcium dry pellet organic fertilizer (Stutzman Environmental Products, Inc., Canby OR) at both locations on 6 March 2016 with a hand spreader.
Emergence notes were taken at both locations on 18 December 2015 (308 GGD Higbee, 338 GGD Tukey). Winter survival was evaluated on 14 May 2016 at Tukey (1330 GDD) and 13 May 2016 at Higbee (1218 GDD). At Tukey, plant height was measured on 14 May (1330 GDD), 31 May (1536 GDD), and 26 July 2016 (2499 GDD). At Higbee, plant height was measured on 13 May (1218 GDD), 1 June (1442 GDD), and 26 July 2016 (2310 GDD). Vegetative growth habit was evaluated at the Higbee on 13 May 2016 (1218 GDD) and rated as a 1 (planophile growth habit), 2 (intermediate growth habit), or 3 (erectophile growth habit). Days to flowering was evaluated based on when 50% of the plants in the plot started flowering.
Stripe rust caused by the fungus Puccinia striiformis Westend. f. sp. tritici is the primary polycyclic disease that affects grains in the region (Chen, 2007). Stripe rust incidence was scored according to the CIMMYT Rust Scoring Guide (http://hdl.handle.net/10883/1109) at Higbee on 13 May (1218 GDD) and 31 May 2016 (1536 GDD) and at Tukey on 14 May 2016 (1330 GDD) and 1 June 2016 (1536 GDD). On the second incidence measurement, the presence of a hypersensitive response was also evaluated at both locations. Percent stripe rust severity and field response (susceptible, moderately susceptible, moderately resistant, or resistant) were evaluated on 26 July 2016 at both locations (2310 GDD at Higbee, 2499 GDD at Tukey).
Tiller number counts were taken at Tukey on 21 July (2398 GDD) and at Higbee on 15 September 2016 (3210 GDD). Lodging, spike length, distance between spikelets and qualitative awn characteristics were measured at Tukey on 14 September (3415 GDD) and at Higbee on 15 September 2016 (3210 GDD). Lodging was evaluated on a scale of 1–9, with 1 being no lodging and 9 being fully horizontal.
On 21 June 2016 (1865 GDD), leaf area index (LAI) and leaf greenness index (LGI) were measured at Tukey. To estimate leaf area index, an Lp-80 PAR/LAI ceptometer (Decagon Devices, Pullman, WA) was used in conjunction with an Apogee PAR sensor (Apogee Instruments, Logan, UT) to get simultaneous PAR measurements from above and below the canopy. Three readings of each upper and lower PAR readings were then collected and the mean value recorded. LAI was then calculated using the LP-80 formula. Leaf greenness was evaluated using the SPAD-502 Plus (Konica Minolta, Ramsey, NJ). These readings are a unitless index ranging from −9.9 to 199.9. To represent leaf greenness index across the flag leaf, readings from the base, middle and upper portions of the leaf were taken. Five flag leaves were sampled from each plot.
PSCR was estimated post-harvest as percent of plants per plot initiating tillering on 29 November 2016 (3951 GDD at Higbee and 4191 GDD at Tukey). Regrowth height was measured at Higbee on 4 November (3776 GDD) and at Tukey on 7 November 2016 (4044 GDD). Reproductive tillering (1–9) was scored on 29 November 2016, with a score of 1 equivalent to an entirely vegetative sward of grass and a score of 9 equivalent to a stand of wheat after jointing, as would be commonly seen during the traditional growing season.
The grain was harvested on 16 September 2016 at both locations using a 1999 Wintersteiger Nursery Master Elite plot combine (Wintersteiger Inc., Ried im Inndreis, Austria). The threshed grain was bagged and weighed. The grain was then cleaned using a single screen and fan, then weighed again. Dividing the cleaned grain weight by the threshed grain weight led to the threshability index (TI). Grain quality and characteristics were tested on the lines from the variety trials. Thousand kernel weight (TKW) was measured using the Seedburo 801 Count-A-Pack (Seedburo Equipment Co., Des Plaines, IL). Protein, starch, neutral detergent fiber (NDF), fiber, and ash were measured using a Perten DA7250 NIR analyzer (Perten Instruments, Hägersten, Sweden). Protein was measured with a fixed moisture content of 12%. Ash was evaluated on a dry basis, while NDF, fiber, and starch were measured as is. Kernel hardness and kernel diameter were tested using the Perten SKCS 4100 (Perten Instruments, Hägersten, Sweden). Two hundred seeds per plot were used to determine the kernel hardness and diameter.
Residue Management Trial
A residue management trial was established adjacent to the variety trials at both locations. The experimental design was a split-block RCBD, with three whole plot post-harvest residue management treatments, two genotype sub-plot treatments, and four replicates per experimental unit. Whole plot treatments included (1) mowing of stubble, (2) burning of stubble, and (3) stubble left standing (control) and were carried out in the fall after the first-year harvest and before the second winter. Sub-plots were two different hybrids, also included in the variety trial (SPS9 and SPS22). The two lines were chosen based on previous observations suggesting high rates of PSCR, acceptable grain yields, and seed availability.
Plot size was the same as the variety trial; however, six rows were planted with 0.23-m row spacing. All plots in both trials were seeded at a density of 1,920 seeds per plot. The residue management treatments were conducted on 9 November 2016 at both locations (3834 GDD at Higbee and 4071 GDD at Tukey). For the stubble mowing treatment, the plots were cut with a Husqvarna 323C weed trimmer (Husqvarna Group, Stockholm, Sweden) to a height of 10 cm above the crown. The stubble burning treatment was carried out with a propane-powered flame weeder.
PSCR was evaluated 20 days after the residue management treatments occurred. Methodology for PSCR and winter survival evaluations were the same as described above for the variety trials.
Statistical Analyses
Data collected were analyzed as a RCBD using PROC MIXED (SAS Institute, 2014) and were assessed for normal errors using PROC UNIVARIATE (SAS Institute, 2014). Equal variance was evaluated using Brown and Forsythe's test. If variances were unequal, variance was modeled as unstructured using PROC MIXED (SAS Institute, 2014). Unstructured variance was used to model distance between spikelet rows, neutral detergent fiber, and TKW. For comparisons, least squares means (LSMeans) were used and reported with an α < 0.05 being considered significantly different. Tillering counts were modeled using a negative binomial distribution. For comparing plant height and rust incidence over time, a heterogeneous compound symmetry model was chosen. Kernza™ was removed from the plant-height, tillering, and all grain quality analyses because of missing data and a lack of grain yield. Emergence, winter survival, vegetative habit, days to flowering, rust severity, protein, and percent regrowth (in both the residue management and variety trials in both spring and autumn) were non-parametric and so were ranked using PROC RANK (SAS Institute) and run through PROC MIXED (SAS Institute, 2014) using Friedman's non-parametric rank test. The ratings of reproductive tillers were transformed with a natural logarithm to meet the assumption of normal errors. TI was transformed with the arcsine of the square root to meet the assumption of normal errors and then variance was modeled as unstructured. For post-harvest field evaluations, Jasper, Norwest 553, and SPS 10 were excluded from the analyses because of their annual growth habit. For comparing PSCR height of the amphiploid lines to Kernza™, the Tukey location was analyzed alone as well as with both locations.
Results
Variety Trial
Emergence, Winter Survival (Year 1), and Vegetative Habit
The effect of genotype and location, but not the interaction, was significant for emergence and winter survival (Table 1). Higher emergence and winter survival was observed at the Tukey location as compared to Higbee, though most genotypic means were not different from each other with the annual and amphiploid lines ranging from 84 to 95% emergence. Kernza™ had poor emergence in comparison, averaging 39%. The annual wheat varieties Jasper and Norwest 553 had higher winter survival rates in the first year than the other lines. The mean vegetative habit was significant for genotype. The annual wheat varieties, along with over half of the amphiploid lines (SPS24, SPS10, SPS19, SPS20, SPS26, SPS29, SPS22, SPS25, and SPS37), had an erectophile vegetative habit, ranging from 2.5 to 3. Other amphiploid lines were grouped as intermediate in growth habit, ranging from 2 to 2.5. None of the lines showed strong planophile growth habits.
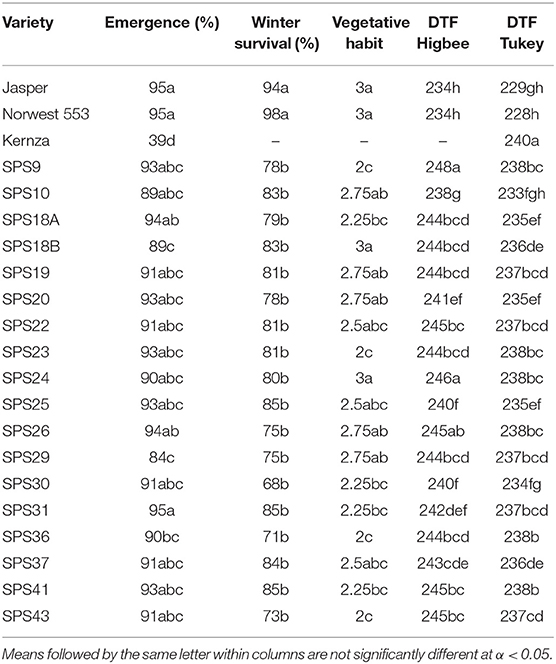
Table 1. Growth characteristics of annual wheat (Jasper and Norwest 553), amphiploid lines and wheatgrass (Kernza) including emergence, first-year winter survival during establishment, vegetative growth habit (1 = planophile, 2 = intermediate, 3 = erectophile) at Higbee and days to flowering (DTF) at both locations.
Plant Height
Plant height was significant for location, genotype, and their interaction (Table 2). In general, plants were taller at Tukey. The annual wheat varieties were taller in early May 2016 than most other lines. The exception to this is Norwest 553 at Tukey, which was not different from most amphiploid lines. As the season progressed, the annual lines grew less than the others. By 26 July 2016, Jasper and Norwest 553 were significantly shorter than all other genotypes. SPS18A and SPS18B grew taller than all of the genotypes, except SPS31 at Higbee, by the final measuring date. The majority of lines followed a similar growth trend to each other, showing extensive late season growth compared to the annuals; the exception was SPS10, which grew earlier, particularly at the Tukey location.
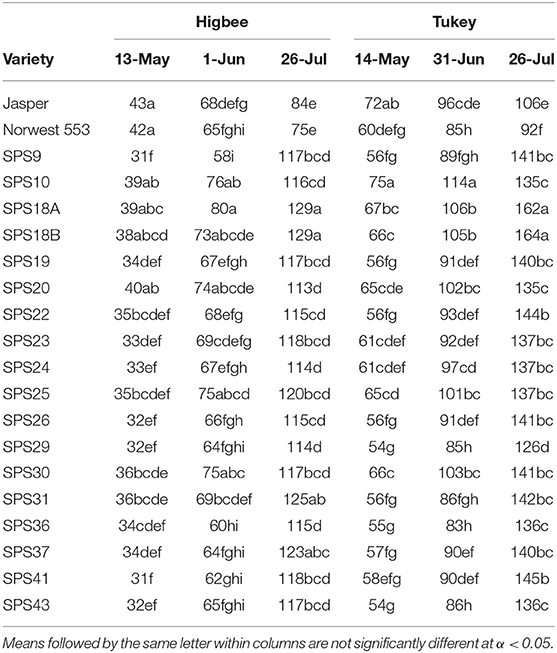
Table 2. Plant height (cm) for annual wheat (Jasper and Norwest 553) and amphiploid lines on different dates at the Higbee and Tukey locations.
Days to Flowering
Days to flowering was significant for location, genotype, and their interaction (Table 1). Varieties began flowering at Higbee 234–248 days after planting (DAP). At Tukey, they began flowering 228–240 DAP. At both locations, Jasper and Norwest 553 were in the earliest flowering groups. At Tukey, SPS10 was also in this early flowering group. Days to flowering for SPS10 was earlier than any other amphiploid lines at Higbee. SPS 20, SPS 25, SPS30, and SPS31 were the earliest flowering amphiploids after SPS10 at Higbee. At Tukey, SPS30 was similar in flowering date to SPS10 and Jasper. The next grouping to flower was SPS10, SPS 18A, SPS20, SPS25, and SPS30. The latest flowering at Tukey was Kernza™ at 240 DAP. All of the amphiploids all began flowering from 233 to 238 DAP at Tukey and 238 to 248 DAP at Higbee.
Leaf Area and Leaf Greenness Indices
Leaf area index varied significantly among genotypes (Table 3). SPS18A and SPS18B were grouped with the largest LAI, with SPS18B averaging 3.1. The two annual varieties were not significantly different than the majority of amphiploid lines. Other than SPS18A and SPS18B, all amphiploids had LAI values ranging from 1.4 to 2.4. Kernza™ and SPS29 had the lowest LAI, with Kernza™ averaging 0.89.
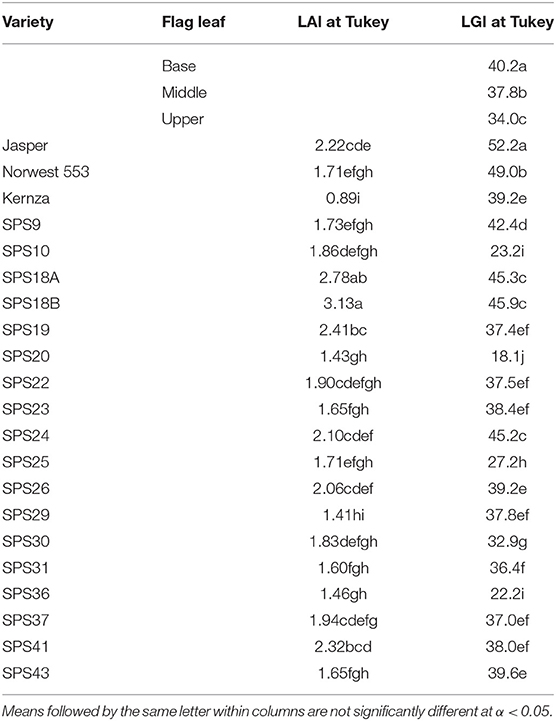
Table 3. Mean leaf area index (LAI) and mean leaf greenness index (LGI) at the Tukey location for all varieties. Mean LGI based on portion of the flag leaf (base, middle or upper portion) was measured on 21 June 2016.
LGI measurements were significant for both the genotype and the portion of the leaf sampled (Table 3). The genotype × leaf portion interaction was not significant. Jasper had the highest LGI reading of 52 followed by Norwest 553. SPS18B, SPS18A, and SPS24 were then grouped with the highest LGI readings of the amphiploid lines ranging from 45.2 to 45.9. Kernza™ was grouped with eight other amphiploid lines, ranging from 37.0 to 39.6. The lowest LGI readings were from SPS20 at 18.1. SPS10, SPS36, SPS25, and SPS30 had considerably lower LGI readings, ranging from 22.2 to 32.9. The base of the flag leaf had the highest LGI readings at 40.2 compared to the middle or upper leaf portions. The upper leaf portion had the lowest LGI readings averaging 34.0.
Tiller Number and Spikelet Phenology
Both location and genotype were significant for tiller number, but not the interaction (Table 4). Higbee had an average of four tillers more per plant than the Tukey location (12.9 compared to 8.7). SPS10, Kernza™, and Norwest 553 had the lowest tiller counts.
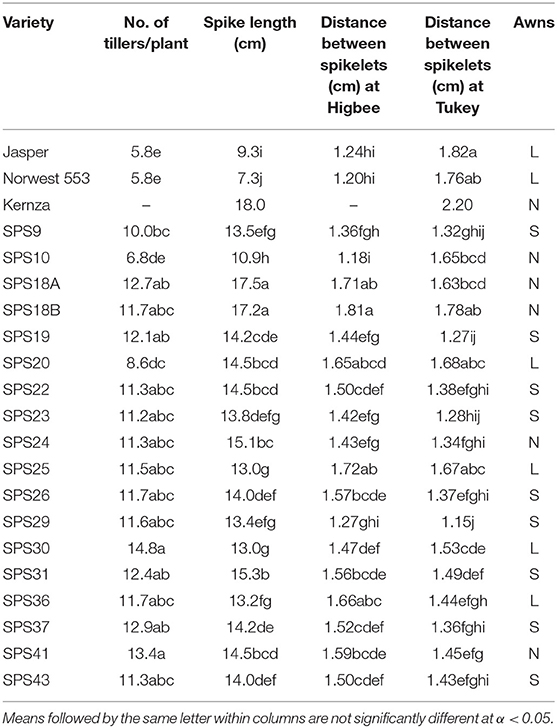
Table 4. Tiller and spike characteristics for all varieties, including mean number of tillers per plant, mean spike length, mean distance between spikelets at both locations (Higbee and Tukey), and awn characteristics (S = short, <2 cm, L = long, >2 cm, N = awnless).
Spike length had only genotype as a significant factor (Table 4). SPS18A and SPS18B had the longest spikes, averaging over 17 cm per spike. Most other amphiploid line means were 13–15 cm long. SPS 10 was the primary exception with shorter spike length than all other amphiploids at 10.9 cm. Jasper and Norwest 553 both had shorter spike lengths of 9.3 and 7.3 cm, respectively, than all other lines.
All lines evaluated had two rows per spikelet. Spikelet distance was significant for genotype and genotype × location (Table 4). At Higbee, SPS 18B, SPS25, SPS18A, SPS36, and SPS20 had the longest distances between spikelets. The shortest spikelet distances were SPS 10, Norwest 553, Jasper, and SPS 29. In contrast to this, the longest spikelet distances at Tukey were Jasper, SPS 18B, Norwest 553, SPS20, and SPS 25. Lodging did not occur at either location.
Stripe Rust Incidence and Severity
Stripe rust incidence was significant for genotype, location, and their interaction (Table 5). Higbee had less rust incidence in the early May measurements than Tukey. On 13 May 2016 (1218 GDD), no lines significantly differed in mean rust incidence, ranging from 0 to 15% incidence at the Higbee location. In contrast at Tukey, SPS36, SPS 20, SPS22, SPS9, and SPS37 showed the highest incidence of stripe rust, ranging from 53 to 68%. Norwest 553, Kernza™, SPS18A, SPS18B, and SPS24 had the lowest incidence, ranging from 0 to 11%. Kernza™ consistently had the lowest incidence of all varieties evaluated. The majority of amphiploid lines reached 100% incidence by the 31 May and 1 June sampling dates. SPS 18A, SPS18B, SPS24, and Kernza™ had the lowest incidence at both locations, ranging from 0 to 43% incidence. All other amphiploid lines had similarly a high incidence of stripe rust, ranging from 85 to 100%. All lines, except SPS20 and SPS36, exhibited a hypersensitive response to stripe rust at this second incidence evaluation. SPS25 showed a hypersensitive response in half of the plots at each location.
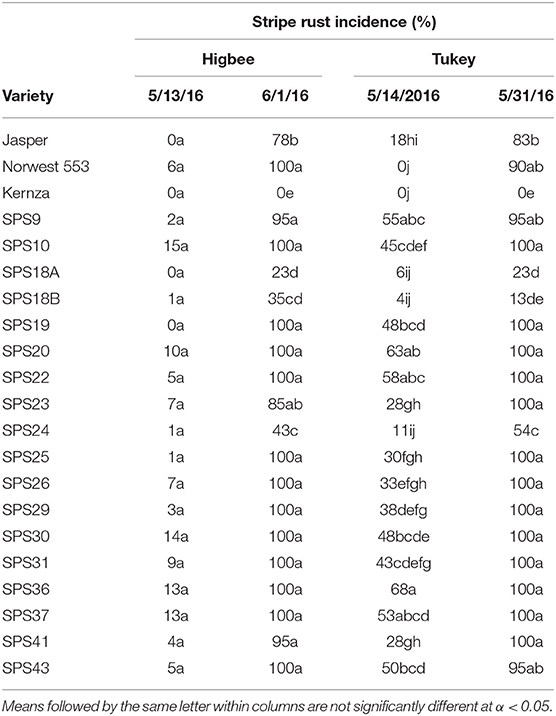
Table 5. Mean stripe rust incidence (%) across both locations (Higbee and Tukey) over two sampling periods for all varieties tested.
Stripe rust severity were significant for genotype, location, and the interaction between the two (Table 6). SPS10, SPS20, and SPS 36 had the highest severity at both locations. Other than SPS10, SPS20, and SPS36, Higbee had relatively low severity. The lines with the lowest severity at Higbee were SPS19, SPS 43, Jasper, SPS18A, SPS18B, SPS31, and SPS23. At Tukey, SPS18B and Kernza™ had the lowest severity with just trace amounts of rust visible. SPS18A, Norwest 553, SPS24, and Jasper also showed low levels of severity. SPS10, SPS20, and SPS36 were subsequently labeled as having a field response of susceptible (Table 6). SPS25 was moderately susceptible, while SPS22, SPS29, SPS30, and SPS37 were moderately resistant. The rest of the lines in the trial were scored as resistant.
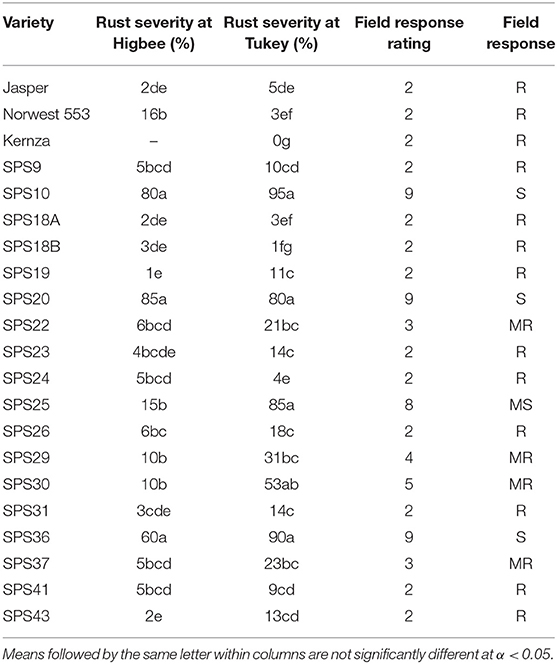
Table 6. Mean stripe rust severity (%), stripe rust field response rating (1 = no infection, 9 = susceptible), and stripe rust field response assessment (R = resistant, MR = moderately resistant, MS = moderately susceptible, S = susceptible) scored on 26 July 2016 for all varieties.
Grain Yield and Threshability
Grain yield was significant for genotype, location, and their interaction (Table 7). At Tukey, grain yield (excluding Kernza™) ranged from 893 to 6,867 kg/ha. Grain yield for Norwest 553 was not statistically different than Jasper. Kernza™ was the lowest yielding of all the varieties with at 108 kg/ha.
At Higbee, SPS10, SPS18B, SPS31, and SPS18A yielded the same as the annual varieties. Higbee had fewer statistical differences in yield, with mean plot yields ranging from 522 to 1,986 grams per plot. Between locations, Tukey yielded higher than Higbee for most lines. Lines that were not significantly different between locations included SPS10, SPS20, SPS25, SPS31, SPS36, SPS37, SPS43, and SPS9. The highest yielding amphiploid lines at both Higbee and Tukey were SPS18A and B, which compared to the two annual wheats, yielded the same at Higbee but roughly 50–60% less at Tukey.
Threshability was significant for genotype, location, and their interaction (Table 8). At both locations, the annual wheat varieties had the highest TI at 99%. SPS10 was lower but only slightly, at 98% at both locations. A wide variation was seen in the rest of the amphiploids at both locations with TI scores ranging from 43 to 88%. SPS29 and SPS31 had relatively high TI at both locations while SPS30, SPS36, SPS25, and SPS20 were consistently poor threshing at both locations ranging from 43 to 69%.
Post Sexual Cycle Regrowth (PSCR)
PSCR was significant for location, with Higbee having 89% compared to 48% regrowth seen at Tukey. Reproductive PSCR was significant for genotype and the location × genotype interaction (Table 9). Only Kernza™ at Tukey achieved a mean score of 1. Mean scores of the amphiploid lines exhibiting PSCR ranged from 2.25 to 5.75. The lines that consistently showed the highest reproductive PSCR at both sites were SPS18B, SPS24, and SPS37. These lines ranged from mean reproductive PSCR scores of 4–5.75. The amphiploid lines consistently low in mean reproductive PSCR scores were SPS9, SPS19, SPS20, SPS26, SPS29, SPS31, SPS36, and SPS43, ranging from 2.25 to 3.25.
Regrowth height was significant for location and genotype, but not the interaction (Table 9). Tukey had taller regrowth (62.3 cm) than Higbee (46.9 cm). The height of the regrowth between amphiploid lines varied from 48.5 to 60.8 cm. Although groupings were significant, four amphiploid lines were grouped in both the tallest and shortest groupings. Kernza™ was significantly shorter than all of the amphiploid lines at Tukey. SPS10 did not exhibit any PSCR.
Winter survival and subsequent spring regrowth were significant for genotype, location, and the interaction between the two (Table 10). Kernza™ was in the group with highest mean regrowth at both locations. The amphiploid lines were highly variable with no discernable trend between the two locations. In general, Higbee had better spring regrowth than Tukey, but there was no consistency.
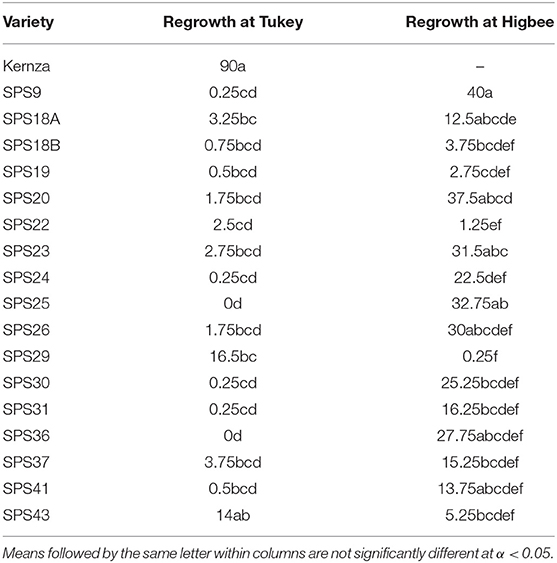
Table 10. Mean winter survival/spring regrowth (% of plants per plot) in the second year for different locations (Higbee and Tukey).
Grain Quality Evaluations
Test weight was significant for genotype and location (Table 8). Entries grown at Higbee had a mean test weight of 62.3 kg/hL compared to Tukey at 65.7 kg/hL. Jasper, Norwest 553, SPS10, and SPS18B had the highest test weights, ranging from 70.0 to 78.4 kg/hL. The rest of the test weights varied from 58.1 to 67.9 kg/hL.
Thousand kernel weight (TKW) was significant for genotype, location, and their interaction (Table 8). At both locations, Jasper had the highest mean TKW followed by Norwest 553 and then SPS10. SPS18A and SPS18B were then grouped below SPS10 with SPS30 being statistically similar to SPS18A and SPS18B at Tukey. At both locations SPS29 had the lowest mean TKW.
Kernel hardness was significant for genotype and genotype × location interaction (Table 11). For both locations, Norwest 553 had the hardest kernels (55.8 at Higbee and 62.2 at Tukey), whereas Jasper had the softest kernels (10.2 at Higbee and 15.2 at Tukey). All of the amphiploid lines were intermediate between the two extremes represented by the soft white Jasper and hard red Norwest 553 winter wheat varieties. The hardest values within the amphiploid lines at both locations were SPS20, SPS24, and SPS9, with means ranging from 31.4 to 34.9. The softest amphiploid lines were SPS25 and SPS31, with means ranging from 22.6 to 24.3.
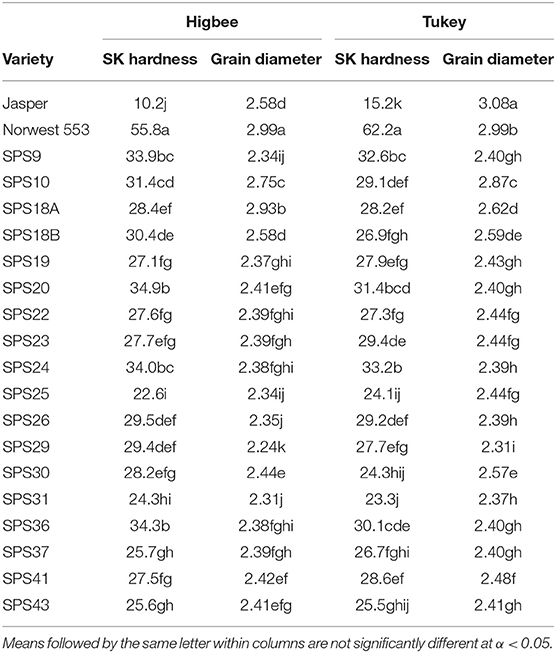
Table 11. Grain hardness (SK hardness index) and grain diameter (mm) for varieties at Higbee and Tukey locations.
Kernel diameter was significant for genotype, location and their interaction (Table 11). The annual varieties had wider diameters than any of the amphiploid lines ranging from 2.93 to 3.08 mm. SPS10 was narrower than the annuals but wider than any of the other amphiploid lines at both locations. SPS18A and SPS18B were narrower yet with kernel diameters ranging from 2.59 to 2.62 mm. The narrowest kernel diameter was found in SPS29, ranging from 2.24 to 2.31 mm.
Grain protein content was significant for genotype and genotype × location (Tables 12, 13). Protein content varied from 9.9 to 15.6%. Jasper, Norwest 553, and SPS18B were in the group with the lowest protein at both locations. SPS22, SPS43, SPS23, SPS19, SPS37, and SPS36 were all grouped with the highest protein at both locations, with protein content ranging from 15.0 to 15.6% protein.
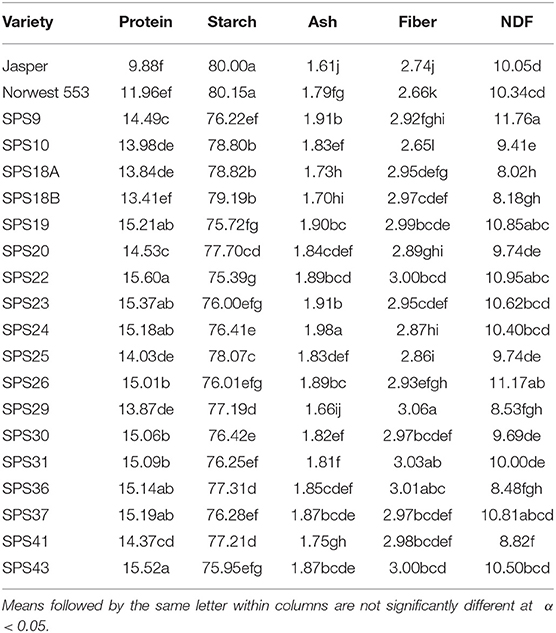
Table 12. Protein (%), starch (%), ash (%), crude fiber (%), and neutral detergent fiber (NDF, %) at the Higbee location.
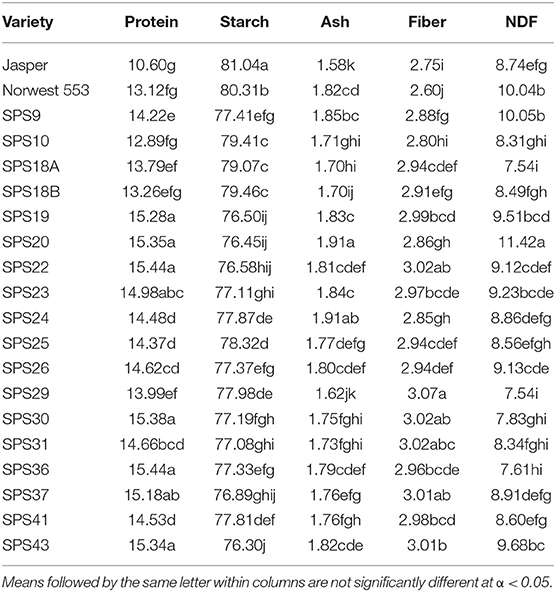
Table 13. Protein (%), starch (%), ash (%), crude fiber (%), and neutral detergent fiber (NDF, %) at the Tukey location.
Ash content was significant for genotype, location, and their interaction (Tables 12, 13). Ash content varied from 1.6 to 2.0% among genotypes. SPS24 was in the highest grouping at both locations while Jasper and SPS29 had the lowest ash content at both locations. Starch content was significant for genotype, location, and the interaction between the two (Tables 12, 13). Jasper and Norwest 553 had higher starch content (80–81%, respectively) than any of the amphiploid hybrids. SPS18A, SPS18B, and SPS10 had the highest starch content of any of the amphiploid lines (78.8–79.5%). The lines consistently grouped with the lowest starch content were SPS22, SPS19, and SPS43 with means ranging from 75.4 to 76.6%.
Fiber content was significant for genotype and the location × genotype interaction (Tables 12, 13). Fiber content varied from 2.6 to 3.1% among the treatments. SPS29 and SPS31 were both in the grouping with the highest fiber content while Jasper, Norwest 553, and SPS10 were grouped with lower fiber content. NDF was significant for genotype, location, and their interaction (Tables 12, 13). NDF varied from 7.5 to 11.8% among genotypes. SPS18A, SPS29, and SPS31 were in the group with the lowest NDF content at both locations.
Residue Management Trial
Post Sexual Cycle Regrowth
The residue management trial had significant differences in mean autumn regrowth following the first harvest based on residue management, location, and their interaction (Figure 1). At Higbee, no residue management treatments differed with percent regrowth ranging from 98.75 to 100%. At Tukey, mowing had the highest response to treatment with a mean of 89% regrowth across the amphiploids. Burning showed the second highest regrowth with a mean 59% regrowth. The control was the lowest regrowth with a mean 38% regrowth. The two genotypes, SPS 9 and SPS 22, did not differ from each other.
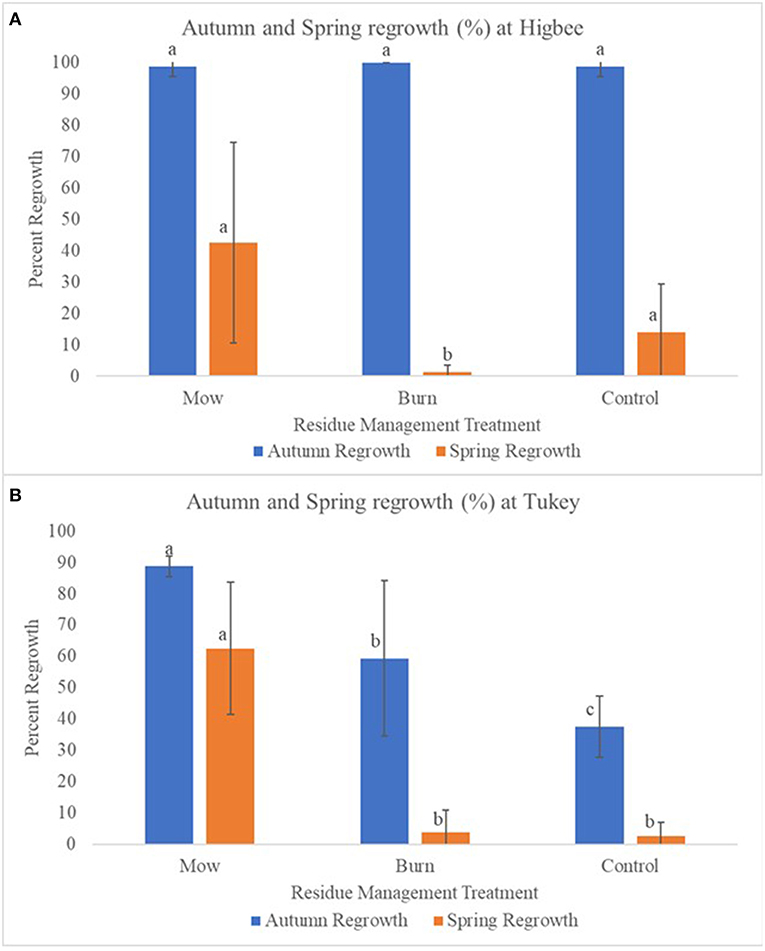
Figure 1. Mean autumn 2016 and spring 2017 post-sexual cycle regrowth (%) across both SPS 9 and SPS 22 genotypes, following the first harvest comparing different residue management treatments (A) at Higbee and (B) at Tukey. Error bars represent standard deviation of the treatments. Means (bars) below the same letters “a,” “b,” or “c” across treatments and within season each season (autumn regrowth = blue bars; spring regrowth = orange bars) are not significantly different at α < 0.05.
Autumn reproductive tillering was significant for residue management, location, and the interaction between the two. Tukey did not have a significant difference between any treatments. The control treatment at Higbee had significantly more reproductive tillers than either the burn or the mow treatment.
Winter Survival and Spring Regrowth
Winter survival and subsequent spring regrowth in the average of both amphiploids had significant differences between residue management treatment and the location × residue management interaction (Figure 1). Genotype was not significant. Tukey had a mean of 63% winter survival and spring regrowth from the mowing treatment, which was significantly higher than the other two residue management treatment means. The burned and control treatments did not significantly differ with 3–4% winter survival and spring regrowth. At Higbee, the mowed and control treatments did not significantly differ, despite mowing having a spring regrowth of 43% and a regrowth of 14% in the control. Burning had significantly less spring regrowth than both other treatments with 1% regrowth.
Discussion
Significant efforts have been made to develop perennial grain crops including wheat, rye, rice, sorghum, and maize (Cox et al., 2002; Sacks et al., 2006a,b), in large part to manage concerns over soil erosion (Pimentel et al., 1987). Rhizomes, or horizontal underground stems, are key for dispersal and propagation of perennial grasses, but can also have a negative effect on weediness (Paterson et al., 1995; Jang et al., 2006). One strategy used for the generation of perennial rice cultivars has been to generate interspecific crosses between annual O. sativa L. and O. longistaminata, an African perennial rice with extensive rhizome formation (Sacks et al., 2006a,b). Rhizome presence was positively associated with survival and vigor of the interspecific lines (Sacks et al., 2006a). Moreover, they found selecting female parents for long rhizomes and male parents for fertility provided considerable gains in rhizome expression, survival and yield (Sacks et al., 2006a). In another study, it was found that rhizome expression in O. longistaminata is controlled by two dominant genes, Rhz2 and Rhz3 (Hu et al., 2003). In addition, the rhizomatous trait in O. longistaminata has been shown to be controlled by a complex gene network, which suggests that the phytohormone auxin acts as a negative regulator of rhizome development whereas gibberellin acts as an activator (Hu et al., 2011). Taken together, these studies suggest selection of rhizome traits is a potential for improving perenniality of grasses.
One of the key challenges in establishing a viable perennial grain in the Palouse dryland agroecosystem is second-year winter survival and stand establishment the following season. In our study, much of the poor second winter survival during stand establishment could be attributed to the excess moisture during the spring at the low-lying Higbee location. Emergence and winter survival were also possibly affected in this study by seed size, as previously shown in Farahani et al. (2011), where larger seeds resulted in better stand establishment and early seedling vigor in wheat. By harvest, differences in stand quality were not noticeable at the Tukey location, suggesting that seed size is not a major issue in stand establishment for these amphiploid lines. At the Higbee location, many of the plants recovered from the extremely wet spring soil conditions but this excess moisture negatively affected yield results. For this reason, Tukey should be viewed as a better representation of conditions in the high-rainfall zones of the Palouse region.
Relatively low yield of perennial grains is a deterrent for adoption by growers. Even the highest yielding lines, such as SPS18A and SPS18B, yielded far less than the annuals at Tukey (Table 7). Interestingly, yield loss at Higbee compared to Tukey was less in the amphiploid lines as compared to the annual varieties. Four of the perennial lines had yields equal to both annual cultivars (Table 7). A robust G × E trial would be informative regarding the stability of the amphiploid lines across diverse conditions. SPS31 and other amphiploid lines did not differ between locations despite the poor stand. This lack of variation suggests strong sink limitations in the amphiploid lines, particularly when grown under optimal conditions.
To test the performance of our amphiploid lines compared to an established perennial grain variety, the wheatgrass Kernza™ was included as a perennial control. Unfortunately, the Kernza™ plants remained small and uncompetitive throughout the growing season. This is best exemplified with the low LAI exhibited by Kernza™. If the study were carried out for multiple seasons, the LAI of Kernza™ would be expected to increase, based on biomass exhibited by Kernza™ in other growing regions (Jungers et al., 2017). The timing of planting and late germination may have also played a role in the reduced vigor compared to the environment it originates from in Kansas. Perhaps with the short, dry growing season of the Palouse region, Kernza™ requires an establishment year to become a strong, vigorous stand.
Stripe rust is one of the most devastating pathogens to PNW wheat, particularly in years with extended cool and wet periods during the growing season (Sharma-Poudyal and Chen, 2011). Fortuitously, the ideal conditions for stripe rust development in 2016 allowed for screening for stripe rust resistance (Chen, 2016). Susceptible lines had nearly no green tissue left on the flag leaves during flowering. SPS18A, SPS18B, and SPS24 had the lowest rust incidence and severity of all the amphiploid lines, and so are presumed to be the most resistant to the pathogen (Table 3). They also were the highest yielding genotypes, which is likely related to the rust resistance. SPS20 and SPS36 were rated as being susceptible to rust and were in the lowest yielding group of amphiploids at both locations.
SPS10, SPS18A, and SPS18B represented an intermediate phenotype between the annual lines and the amphiploid lines when evaluating characteristics, such as test weight, protein, starch, and yield. Any connections to the yield, however, break down when looking at SPS24. SPS24 is also high yielding but has many quality characteristics similar to the amphiploid lines (Tables 7, 8, 11–13). SPS18A and SPS18B represent particularly unique lines compared to the other amphiploid lines for both agronomic and grain qualities. Many of the noteworthy characteristics are improved agronomic performance, such as yield, rust resistance, and TKW. As the tallest lines in this study, these two lines could integrate well into certain production scenarios. For example, the increased height advantage coupled with the highest LAI may fit well into an organic agroecosystem or a production system that values production of biomass and enhancement of soil organic matter.
Another potential use for SPS18A and SPS18B would be as a dual-purpose crop suitable for humans and livestock. The LAI suggests that they produced the most biomass as well as grain yield. In production scenarios where growers were looking primarily for animal feed, the vegetation:grain ratio may be less important. Additionally, the grain NDF was the lowest of all samples tested in this study, indicating that the grains could be of improved quality for animal consumption. Further studies testing how these grains would affect animal health are needed before the feed quality of the grain is known. Although using these lines for animal feed may not represent the original goal of the project, it might be a valuable intermediate for growers and breeders to become more acquainted with how perennial grains would fit into a production scenario. It would also allow more flexibility for breeders to focus on establishing reliable perennial habits, without having such strict end-use qualities required immediately.
Another consideration for a perennial grain is end use quality traits. In comparing the end-use qualities of the amphiploid lines to the released annuals, we found a wide diversity in the amphiploid germplasm. There are no lines that are equivalent to the annual wheat varieties in all of the characteristics we tested. This reinforces the findings of Murphy et al. (2009a) that amphiploid lines do not adequately fit into existing market classes of wheat. Based on the previous findings of inferior baking qualities (Murphy et al., 2009a) and the results from the SK hardness test, it is assumed that a focus on meeting the requirements of a soft white wheat class is more attainable than meeting the complex requirements for a hard wheat class.
Taken together, these data suggest that for success in establishing a new class of perennial grain, the market potential has to be evaluated more carefully. Indeed, the media attention already payed to Kernza™, coupled with the development of new markets for the novel grain, represents an opportunity for marketing perennial grains (Black, 2016; Bland, 2016). Grain protein and mineral concentration are higher in amphiploid lines, though loaf volume is decreased and kernels are softer compared to annual bread wheat cultivars (Murphy et al., 2009a). The environmental benefits of perennial agroecosystems can be marketed along with the unique nutritional aspects to increase the value of the grain. These value-added marketing chains could help offset the economics of reductions in yield. Moreover, they have the potential to derive enough income and awareness of the issue to justify more breeding efforts to advance perennial grains into mainstream markets.
Another bottleneck for adoption of perennial grains is establishing best management practices to optimize growth and yield. From our work, it appears that managing PSCR may be an important factor for subsequent winter survival. This preliminary study on post-harvest management suggests that the plants are responsive to the mowing treatment in the autumn after harvest (Figure 1). The Tukey location showed strong effects from mowing the residue and regrowth that could be seen into the following spring. The mechanism behind this is unknown. Young tillers of true perennial grasses are known to be sensitive to light levels and balance after flowering (Wan and Sosebee, 1998; Gautier et al., 1999; Bahmani et al., 2000). A high ratio of red:far-red light tends to induce increased tillering in perennial grasses (Wan and Sosebee, 1998). The actual physiological response to red:far-red balance in the amphiploids is unknown, but should be a focus of future studies.
It is important to take into account that the growth habit of these plants is not an evolved perennial habit refined and strengthened by millennia of natural selection. Therefore, comparisons to perennials previously studied cannot be directly translated to the amphiploid lines used in this study. One possibility is that when the residue and new growth were removed, the red:far-red ratio reaching the crown increased, leading to physiological changes that improved survival. The burn treatment could also affect the red:far-red balance, but burning in this trial appeared to hinder survival. This could be explained by poor burning conditions when the trial was being carried out. Because the residue was so wet, the flame had to be kept on the plants for extended periods and damage may have been caused to the crown and auxiliary buds at that time.
Another possible explanation for the increased survival from mowing is that the defoliation physically forced the plant to accumulate more photosynthates near the crown by removing all growth away from the crown. The management results here are relatively in agreement with previous results that one cutting is not adequate for the best vigor of Thinopyrum spp. Deciphering the mechanism behind how defoliation affected the physiology of the plant was beyond the scope of this study.
The regrowth scores from the variety trial, where no post-harvest management was conducted, show clearly that without careful management, the current germplasm will unlikely function as reliably perennial plants and further breeding is necessary to improve the perennation of these plants. Based on this study, however, it is likely that multi-year survival rates among the amphiploid lines would likely increase with improved management practices. Literature suggests that grazing/cutting height and frequency, sward density, and soil fertility are some of the most impactful factors for both forage and seed production in a perennial grass (Buglass, 1964; Lafarge et al., 2005; Dickeduisberg et al., 2017). Future research investigating those factors could be crucial in developing best management practices.
Conclusion
In our variety trials, we found significant variation for agronomic traits of importance, including emergence, days to flowering, plant height, leaf area index, tiller number, spike length, and grain yield. Traits related to the perennial growth habit also varied significantly across amphiploids, and we identified germplasm with improved post-sexual cycle regrowth and second-year winter survival and subsequent regrowth. This variation across the amphiploids suggests the potential for continued selection and improvement among these breeding lines for agronomic traits of interest.
Additional results from the variety trials showed that the four highest-yielding amphiploids produced the same yield at one location but 50–60% less at the other. Given these promising grain yields, further studies into strengthening perennial growth habit is critical. We also found significant differences among amphiploids in grain quality traits, including grain hardness, protein content, neutral detergent fiber, crude fiber, starch, and ash content. The amphiploids had generally lower test weight and thousand kernel weight, smaller kernel diameters, and lower starch content, while exhibiting higher protein, ash, and fiber contents. These positive quality traits can be exploited in terms of unique functionality and potentially higher nutritional value for use in novel food and beverage products.
Little is known about how to appropriately manage perennial grains in the dryland agroecosystem of the Palouse region of the PNW. Therefore, we tested the effect of post-harvest residue management on PSCR and winter survival of two amphiploids using mowing and burning treatments compared to a control. Burning the residue did not improve survival; however, mowing the residue and PSCR significantly increased winter survival across both amphiploids at one location from 3% in the control to 63% in the mowed treatment. This could have significant positive impacts on future perennial grain breeding and production in the Palouse. These promising results suggest the need to investigate diverse and innovative agronomic strategies to optimize selection of amphiploids with a stable perennial habit.
Author Contributions
IC and KM designed the experiment, with input from SJ, JR, and KS. KM and SJ provided the germplasm. IC carried out the experiment and wrote the manuscript. JR, KS, SJ, and KM edited the manuscript.
Funding
Funding for this research was provided by Seed Matters, through the Clif Bar Family Foundation.
Conflict of Interest Statement
The authors declare that the research was conducted in the absence of any commercial or financial relationships that could be construed as a potential conflict of interest.
References
Allan, R. E., Peterson, C. J., Rubenthaler, G. L., Line, R. F., and Roberts, D. E. (1989). Registration of ‘Madsen’ wheat. Crop Sci. 29, 1575–1576. doi: 10.2135/cropsci1989.0011183X002900060068x
Bahmani, L., Varlet-Grancher, C., Hazard, L., Matthew, C., Betin, M., Langlais, A., et al. (2000). Post-flowering tillering in contrasting light environments of two New Zealand perennial ryegrass cultivars with different perennation strategies. Grass Forage Sci. 55, 367–371. doi: 10.1046/j.1365-2494.2000.00228.x
Black, J. (2016, October 2). Perennial wheat is an ecologist's dream. Soon it may be what's for dinner. Washington Post.
Bland, A. (2016, October 26). After a long day of fighting climate change, this grain is ready for a beer. National Public Radio.
Buglass, E. (1964). Seed production of crested wheatgrass as influenced by various management practices. Can. J. Plant Sci. 44, 66–74. doi: 10.4141/cjps64-012
Carter, A. H., Jones, S. S., Balow, K. A., Shelton, G. B., Burke, A. B., Lyon, S., et al. (2017). Registration of ‘Jasper’ soft white winter wheat. J. Plant Reg. 11, 263–268. doi: 10.3198/jpr2016.09.0051crc
Chen, X. M. (2007). Challenges and solutions for stripe rust control in the United States. Aust. J. Agr. Res. 58, 648–655. doi: 10.1071/AR07045
Chen, X. M. (2016). Stripe Rust Update, April 13, 2016. Washington State University Extension. Available online at: http://striperust.wsu.edu/2016/04/13/stripe-rust-update-april-13-2916/ (accessed June 21, 2017).
Cox, T. S., Bender, M., Picone, C., Van Tassel, D. L., Holland, J. B., Brummer, E. C., et al. (2002). Breeding perennial grain crops. Crit. Rev. Plant Sci. 21, 59–91. doi: 10.1080/0735-260291044188
Cox, T. S., Van Tassel, D. L., Cox, C. M., and DeHaan, L. R. (2010). Progress in breeding perennial grains. Crop Pasture Sci. 61, 513–521. doi: 10.1071/CP09201
Curwen-McAdams, C., Arterburn, M., Murphy, K., Cai, X., and Jones, S. S. (2017). Toward a taxonomic definition of perennial wheat: a new species × Tritipyrum aaseae described. Gen. Resour. Crop Evol. 64, 1651–1659. doi: 10.1007/s10722-016-0463-3
Dickeduisberg, M., Laser, H., Tonn, B., and Isselstein, J. (2017). Tall wheatgrass (Agropyron elongatum) for biogas production: crop management more important for biomass and methane yield than grass provenance. Indust. Crops Prod. 97, 653–663. doi: 10.1016/j.indcrop.2016.12.055
Duffin, A. P. (2007). Plowed Under: Agriculture and Environment in the Palouse. Seattle, WA: University of Washington Press.
DuPont, S. T., Beniston, J., Glover, J. D., Hodson, A., Culman, S. W., Lal, R., et al. (2014). Root traits and soil properties in harvested perennial grassland, annual wheat, and never-tilled annual wheat. Plant Soil 381, 405–420. doi: 10.1007/s11104-014-2145-2
Ergon, Å. (2017). Optimal regulation of the balance between productivity and overwintering of perennial grasses in a warmer climate. Agronomy 7:19. doi: 10.3390/agronomy7010019
Farahani, H. A., Moaveni, P., and Maroufi, K. (2011). Effect of seed size of seedling production in wheat (Triticum aestivum L.). Adv. Environ. Biol. 5, 1711–1715.
Gautier, H., Varlet-Grancher, C., and Hazard, L. (1999). Tillering responses to the light environment and to defoliation in populations of perennial ryegrass (Lolium perenne L.) selected for contrasting leaf length. Ann. Bot. 83, 423–429. doi: 10.1006/anbo.1998.0840
Glover, J. D., Culman, S. W., DuPont, S. T., Broussard, W., Young, L., Mangan, M. E., et al. (2010a). Harvested perennial grasslands provide ecological benchmarks for agricultural sustainability. Agr. Ecosyst. Environ. 137, 3–12. doi: 10.1016/j.agee.2009.11.001
Glover, J. D., and Reganold, J. P. (2010). Perennial grains: food security for the future. Issues Sci. Tech. 26, 41–47.
Glover, J. D., Reganold, J. P., Bell, L. W., Borevitz, J., Brummer, E. C., Buckler, E. S., et al. (2010b). Increased food and ecosystem security via perennial grains. Science 328, 1638–1639. doi: 10.1126/science.1188761
Greco, A. C. (2004). Botanical characterization of hybrid perennial wheat and parent lines (M.S. thesis). Washington State University, Pullman, WA, United States.
Hall, M., Young, D. L., and Walker, D. J. (1999). Agriculture in The Palouse: A Portrait of Diversity. Extension Bulletin. Moscow: University of Idaho.
Hayes, R. C., Newell, M. T., DeHaan, L. R., Murphy, K. M., Crane, S., Norton, M. R., et al. (2012). Perennial cereal crops: an initial evaluation of wheat derivatives. Field Crops Res. 133, 68–89. doi: 10.1016/j.fcr.2012.03.014
Hu, F., Wang, D., Zhao, X., Zhang, T., Sun, H., Zhu, L., et al. (2011). Identification of rhizome-specific genes by genome-wide differential expression analysis in Oryza longistaminata. BMC Plant Biol. 11, 1471–2229. doi: 10.1186/1471-2229-11-18
Hu, F. Y., Tao, D. Y., Sacks, E., Fu, B. Y., Xu, P., Li, J., et al. (2003). Convergent evolution of perenniality in rice and sorghum. Proc. Natl. Acad. Sci. U.S.A. 100, 4050–4054. doi: 10.1073/pnas.0630531100
Jakumar, N., Snapp, S., Murphy, K. M., and Jones, S. S. (2012). Agronomic assessment of perennial wheat and perennial rye as cereal crops. Agron. J. 104, 1716–1726. doi: 10.2134/agronj2012.0291
Jang, C. S., Kamps, T. L., Skinner, D. N., Schulze, S. R., Vencill, W. K., and Paterson, A. H. (2006): Functional classification, genomic organization, putatively cis-acting regulatory elements, relationship to quantitative trait loci, of sorghum genes with rhizome-enriched expression. Plant Phys. 142,1148–1159. doi: 10.1104/pp.106.082891
Jungers, J. M., DeHaan, L. R., Betts, K. J., Sheaffer, C. C., and Wyse, D. L. (2017). Intermediate wheatgrass grain and forage yield responses to nitrogen fertilization. Agron. J. 109, 462–472. doi: 10.2134/agronj2016.07.0438
Lafarge, M., Mazel, C., and Hill, D. R. C. (2005). A modelling of the tillering capable of reproducing the fine-scale horizontal heterogeneity of a pure grass sward and its dynamics. Ecol. Model. 183, 125–141. doi: 10.1016/j.ecolmodel.2004.08.003
Lammer, D., Cai, X., Arterburn, M., Chatelain, J., Murray, T. D., and Jones, S. S. (2004). A single chromosome addition from Thinopyrum elongatum confers a polycarpic, perennial habit to annual wheat. J. Exp. Bot. 55, 1715–1720. doi: 10.1093/jxb/erh209
Marshall, A. H., Collins, R. P., Humphreys, M. W., and Scullion, J. (2016). A new emphasis on root traits for perennial grass and legume varieties with environmental and ecological benefits. Food Energy Sec. 5, 26–39. doi: 10.1002/fes3.78
Murphy, K., Carter, A., Zemetra, R. S., and Jones, S. S. (2007). Karyotype and ideogram analyses of four wheatgrass cultivars for use in perennial wheat breeding. J. Sust. Agr. 31, 137–149. doi: 10.1300/J064v31n01_11
Murphy, K. M., Hoagland, L. A., Reeves, P. G., Baik, B., and Jones, S. S. (2009a). Nutritional and quality characteristics expressed in 31 perennial wheat breeding lines. Renew. Agr. Food Sys. 24, 285–292. doi: 10.1017/S1742170509990159
Murphy, K. M., Lyon, S. R., Balow, K. A., and Jones, S. S. (2009b). Post-sexual cycle regrowth and grain yield in Thinopyrum elongatum × Triticum aestivum amphiploids. Plant Breed. 129, 480–483. doi: 10.1111/j.1439-0523.2009.01712.x
Paterson, A. H., Schertz, K. F., Lin, Y. R., Liu, S. C., and Chang, Y. L. (1995). The weediness of wild plants: molecular analysis of genes influencing dispersal and persistence of johnsongrass, Sorghum halepense (L.) Proc. Natl. Acad. Sci. U.S.A 92, 6127–6131. doi: 10.1073/pnas.92.13.6127
Piaskowski, J., Murphy, K., Kisha, T., and Jones, S. S. (2017). Perennial wheat lines have highly admixed population structure and elevated rates of outcrossing. Euphytica 213:171. doi.org/10.1007/s10681-017-1961-x doi: 10.1007/s10681-017-1961-x
Pimentel, D., Allen, J., Beers, A., Guinand, L., Linder, R., McLaughlin, P., et al. (1987). World agriculture and soil erosion. BioScience 37, 277–283. doi: 10.2307/1310591
Reganold, J. P. (2013). Comparing organic and conventional farming systems: metrics and research approaches. Crop Manag. 12, 1–6. doi: 10.1094/CM-2013-0429-01-RS
Sacks, E. J., Dhanapala, M. P., Sta. Cruz, M. T., and Sallan, R. (2006a). Breeding for perennial growth and fertility in an Oryza sativa/O. longistaminata population. Field Crops Res. 95, 39–48. doi: 10.1016/j.fcr.2005.01.021
Sacks, E. J., Schmit, V., McNally, K. L., and Sta Cruz, M. T. (2006b). Fertility in an interspecific rice population and its effect on selection for rhizome length. Field Crops Res. 95, 30–38. doi: 10.1016/j.fcr.2005.01.026
Sandve, S. R., Kosmala, A., Rudi, H., Fjellheim, S., Rapacz, M., Yamada, T., et al. (2011). Molecular mechanisms underlying frost tolerance in perennial grasses adapted to cold climates. Plant Sci. 180, 69–77. doi: 10.1016/j.plantsci.2010.07.011
Saxton, K., Chandler, D., Stetler, L., Lamb, B., Claiborn, C., and Lee, B.-H. (2000). Wind erosion and fugitive dust fluxes on agricultural lands in the Pacific Northwest. Trans. ASAE 43, 623–630. doi: 10.13031/2013.2743
Sharma-Poudyal, D., and Chen, X. M. (2011). Models for predicting potential yield loss of wheat caused by stripe rust in the U.S. Pacific Northwest. Phytopathology 101, 544–554. doi: 10.1094/PHYTO-08-10-0215
Steiner, F. R. (1987). The Productive and Erosive Palouse Environment. Pullman, WA: Washington State University Cooperative Extension.
Van Tassel, D., and DeHaan, L. (2013). Wild plants to the rescue. Am. Sci. 101, 218–225. doi: 10.1511/2013.102.218
Vico, G., Manzoni, S., Nkurunziza, L., Murphy, K. M., and Weih, M. (2016). Trade-offs between seed output and life span – a quantitative comparison of traits between annual and perennial congeneric species. New Phytol. 209, 104–114. doi: 10.1111/nph.13574
Wan, C., and Sosebee, R. E. (1998). Tillering responses to red:far-red light ratio during different phenological stages in Eragrostis curvula. Environ. Exp. Bot. 40, 247–254. doi: 10.1016/S0098-8472(98)00044-6
Watson, L., Macfarlane, T. D., and Dallwitz, M. J (2017). The Grass Genera of the World: Descriptions, Illustrations, Identification, and Information Retrieval; Including Synonyms, Morphology, Anatomy, Physiology, Phytochemistry, Cytology, Classification, Pathogens, World and Local Distribution, and References. 12th Version. Available online at: delta-intkey.com/grass
Keywords: perennial grains, post-sexual cycle regrowth, winter survival, Thinopyrum, residue management
Citation: Clark I, Jones SS, Reganold JP, Sanguinet KA and Murphy KM (2019) Agronomic Performance of Perennial Grain Genotypes in the Palouse Region of the Pacific Northwest, USA. Front. Sustain. Food Syst. 3:39. doi: 10.3389/fsufs.2019.00039
Received: 11 December 2018; Accepted: 01 May 2019;
Published: 22 May 2019.
Edited by:
Rakesh Bhardwaj, National Bureau of Plant Genetic Resources (ICAR), IndiaReviewed by:
Sapna Langyan, National Bureau of Plant Genetic Resources (ICAR), IndiaAvinash Chandra Pandey, Metahelix Life Sciences Limited, India
Jyoti Kumari, National Bureau of Plant Genetic Resources (ICAR), India
Copyright © 2019 Clark, Jones, Reganold, Sanguinet and Murphy. This is an open-access article distributed under the terms of the Creative Commons Attribution License (CC BY). The use, distribution or reproduction in other forums is permitted, provided the original author(s) and the copyright owner(s) are credited and that the original publication in this journal is cited, in accordance with accepted academic practice. No use, distribution or reproduction is permitted which does not comply with these terms.
*Correspondence: Kevin M. Murphy, kmurphy2@wsu.edu