- 1Department of Food Science and Center for Food Safety, University of Arkansas, Fayetteville, AR, United States
- 2Jones-Hamilton Co., Walbridge, OH, United States
The prevalence of Listeria monocytogenes under refrigerated conditions is a concern for ready to eat meats like Frankfurters. The purpose of this study was to evaluate the bactericidal effect of potential antimicrobials, Bisulfate of Soda (SBS), nisin, and their combination on L. monocytogenes in frankfurters. Antimicrobial treatment concentrations of SBS and nisin were indicated by performing minimum inhibitory concentration (MIC) and minimum bactericidal concentration (MBC) test. Results of the MIC indicated the concentrations 0.75 and 1.5% SBS and 0.5, 1, and 2% nisin. Frankfurters were inoculated with L. monocytogenes (initial population: 6.65 log CFU/mL and treated with water, SBS (0.75 and 1.5%) nisin (0.5, 1, and 2%) and combinations (0.75% SBS+0.5% nisin, 0.75% SBS+1% nisin, 1.5% SBS+1% nisin, and 1.5% SBS+2% nisin). After treatment, frankfurters were removed, rinsed in neutralizing buffer, and plated on Oxford agar. Data were analyzed using One-Way ANOVA, linear contrasts and pairwise comparisons in JMP 14.0. Differences were determined using Tukey's protected HSD and considered significant when P ≤ 0.05. The treatment 1.5% SBS + 2% nisin exhibited the greatest reduction of L. monocytogenes (2.66 log CFU/mL; P < 0.001). Although the effectiveness of both SBS and nisin concentrations were similar when compared to (0.75% SBS-2.19 log CFU/mL; 1.5% SBS-2.29 log CFU/mL; 5% nisin-1.99 log CFU/mL; 1% nisin-2.47 log CFU/mL; 2% nisin-2.42 log CFU/mL), there was a linear effect shown by the SBS and nisin treatments compared to the tap water treatment (P < 0.0001; P < 0.0001). The effectiveness of 0.75% SBS compared to other treatments using Pairwise comparisons determined that frankfurters treated with 0.75% SBS and 0.75% SBS+1% nisin resulted in lower L. monocytogenes populations than tap water (TW), whereas 0.75% SBS+0.5% nisin was not different (P < 0.0001). Furthermore, when evaluating the efficacy of 1.5% SBS using Pairwise comparisons, 1.5% SBS, and 1.5% SBS combinations were all significantly different than TW (P < 0.0001). Together, both antimicrobials can reduce Listeria without diminishing the appearance of the frankfurter. The results suggest incorporating SBS and nisin as an alternative antimicrobials for frankfurters can be effective for decreasing L. monocytogenes.
Introduction
Ready-to-Eat (RTE) products are those that are generally safe to consume without additional preparation or cooking. These products often include beef and poultry products such as deli meats, frankfurters, canned meats (tuna, chicken, and spam), as well as beef and another meat jerky (Seman et al., 2018). Due to the nature of RTE foods, there is an outstanding concern for the consumption of contaminated food (Yehia et al., 2016). One of the leading foodborne pathogens associated with RTE foods is Listeria monocytogenes (McLauchlin et al., 2004; Zhu et al., 2005). L. monocytogenes is ubiquitous in nature and able to grow in both aerobic and anaerobic environments, enabling it to persevere in several different conditions (Saha et al., 2015; Yehia et al., 2016). In addition, L. monocytogenes acts as a saprophytic organism with the capability to adapt to an ever-changing environment because it possess multiple stress response mechanisms such as overcoming varying temperatures (0–45°C), salt concentrations (up to 10% NaCl) and is non-extremophilic (pH 4.5–9.2) (Ryser et al., 1997; Ryser and Marth, 2007; Wang et al., 2017). Therefore, L. monocytogenes is a significant threat to consumer welfare and the RTE food industry.
As L. monocytogenes is distributed ubiquitously throughout the environment, it has emerged as a major foodborne pathogen. The ingestion of L. monocytogenes causes listeriosis, a foodborne disease, which is recognized as an important worldwide public health problem (Mateus et al., 2013). Listeriosis accounts for 1,600 cases of illness and Listeria is associated with ~$2.7 billion in monetary loss in the United States annually [Economic Research Service (ERS), 2014]. The major concern surrounding listeriosis is the severity of the disease. Listeriosis has been reported to have a mortality rate of 19–30% in immunocompromised patients in the United States (Swaminathan and Gerner-Smidt, 2007; Goulet et al., 2012; Center for Disease Control Prevention (CDC), 2019). Most listeriosis cases are caused by transmission through fresh produce and RTE food (Yehia et al., 2016; Zhu et al., 2017).
RTE meat is one of the most common food products associated with L. monocytogenes in the USA (United States Department of Agriculture, Food Safety and Inspection Service, 2017). Listeria transmission in RTE meat occurs through cross-contamination during post processing steps, and unlike most food products that require an initial cook process, RTE food products do not require thermal cooking in order to be consumed (Swaminathan and Gerner-Smidt, 2007; Martin et al., 2010; Goulet et al., 2012). Furthermore, Listeria have the ability to grow at 40–0°C, which increases the chance consumers will ingest L. monocytogenes (Ramaswamy et al., 2007). According to the FSIS Risk assessment in 2010, RTE meat products have the highest risk of deaths per serving and deaths per annum among all other food products (United States Department of Agriculture, Food Safety and Inspection Service, 2017). In 2018, there were two outbreaks associated with RTE meat products [Center for Disease Control Prevention (CDC), 2019]. The total cases of both outbreaks were 8 persons. Though a small number, one death was recorded bringing the mortality rate of 12.5% in 2018 for RTE meats. With L. monocytogenes being present in RTE foods and possessing the capability to survive in normal refrigeration temperatures, it is evident that additional action must be taken to ensure the safety of RTE meats.
A potential method to improve the shelf life of RTE meats and reduce the presence of foodborne pathogens such as L. monocytogenes is the introduction and use of novel antimicrobials (Geornaras et al., 2006). The application of antimicrobial compounds can be utilized after lethality steps, such as pasteurization and steaming, with the purpose of controlling and diminishing post-processing contamination (Koseki et al., 2007). Other lethality steps have demonstrated the potential to initially reduce L. monocytogenes population; however, as Listeria can grow at refrigerator temperatures, there is a concern for Listeria regrowth during cold storage (Chan and Wiedmann, 2008; Orsi and Wiedmann, 2016). In contrast, the use of antimicrobials has demonstrated the potential to control the growth of L. monocytogenes alone or when used in combination with other lethal and post-lethal steps (Thompson et al., 2008; Lourenco et al., 2016). Previously, Martin et al. (2009) studied combinations of lauric arginate and sodium lactate/potassium diacetate, which would produce an immediate lethality against L. monocytogenes on frankfurters (Martin et al., 2009). The combination of either potassium lactate/sodium diacetate with 22 ppm lauric arginate caused more than a 2-log reduction throughout its shelf life. Therefore, there is a legitimate need to investigate the application of alternative antimicrobials on RTE foods. Thus, the purpose of the current study was to evaluate the antimicrobial effect of an alternative acidifier bisulfate of soda (SBS) and the bacteriocin nisin used alone and in combination on the reduction of Listeria monocytogenes using commercial frankfurters as the model food matrix.
Materials and Methods
Frankfurter Procurement and Listeria Screening
Commercially available frankfurters (eight, uncured, no-nitrate or nitrite-added, no preservatives, no by-products, and two nitrite added controls) were obtained from commercial retail outlets and transported under refrigerated conditions to the University of Arkansas Center for Food Safety (Fayetteville, AR, USA) on four separate occasions (replications). Selection of frankfurters was based on sell by dates indicated on the package. Prior to the experiment, frankfurter from random packets were chosen and screened for the unintended presence of Listeria by rinsing in neutralizing buffered peptone water (nBPW; United States Food and Drug Administration, Food Safety Inspection Service, 2016) for 1 min, plating on Oxford agar, and aerobically incubating for 24 h at 37°C.
Inocula Preparation
Listeria monocytogenes strain EDG-e was used for this study because it is one of the more studied strains with its ecology, functionality, genetic, and biochemical data available and its genome is fully sequenced. L. moncytogenes EDG-e was grown on Oxford agar (Himedia Company, West Chester, Pennsylvania, USA) aerobically at 37°C for 24 h. After incubation, one colony was inoculated in 20 ml of Tryptic soy broth (TSB; Becton, Dickinson, and Company, Sparks, Maryland, USA), followed by incubation at 35°C with constant shaking at 100 RPM for 24 h. The pure L. monocytogenes culture was then washed twice with 20 mL of sterile 1 × Phosphate Buffered Saline (PBS; 8 g of NaCl, 0.2 g of KCl, 1.44 g of Na2HPO4, and 0.24 g of KH2PO4 per 1 L, with the pH adjusted to 7.4 with HCl) using centrifugation at 25,000 g's for 5 min. The final pellet was re-suspended in 20 mL of PBS and was utilized as the inoculum.
Minimum Inhibitory Concentration (MIC)
Listeria monocytogenes strain EDG-e was grown on Oxford agar at 37°C for 24 h. A 96 well microplate was subsequently filled with 100 μL of TSB. A single colony was selected and aseptically transferred into each well of 100 μL of TSB followed by growth overnight aerobically at 37°C for 24 h.
Antimicrobial treatments, SBS and nisin, were used as an indicator for the MIC in the current study. The antimicrobials were prepared in TSB, 20 mL of SBS at 25% and nisin at 25% weight to volume. Following, dilutions (1:2) were achieved by adding 100 μL of TSB to subsequent wells in a 96 well microplate. Listeria was pin replicated into treatment plates using a 96 pin replicator. Immediately following pin replication, the microplates were incubated for 18 h at 37°C in aerobic conditions. After incubation, the microplates were observed for bacterial growth. There were six plates in total utilized in the study (3 total replication of both SBS and nisin).
Minimum Bactericidal Concentration (MBC)
The observed MIC was subsequently plated on Oxford agar and incubated aerobically for 24 h at 37°C. Following, 10 μL of the MIC solution and two wells above and below the MIC concentration were plated in triplicate and incubated under previously mentioned conditions. MBC was defined as the concentration plated that demonstrated no growth after 24 h of incubation.
Inoculation and Treatment Application
Antimicrobial solutions were prepared with SBS [0.75 and 1.5% (w/v)] (Jones Hamilton Company, USA), nisin [0.50, 1.0, and 2% (w/v)] (Sigma-Aldrich), and the combination [0.75% SBS + 0.5% nisin, 0.75% SBS + 1.0% nisin, 1.5% SBS + 1% nisin, and 1.5% SBS + 2% nisin (w/v)] in 20 mL of tap water at room temperature. In the current study, only confirmed Listeria-free frankfurters were used. Commercial beef frankfurters were purchased and used throughout the study. There was one frankfurter per treatment, with four replications. Frankfurters were placed into sterile sample bags (VWR, Radnor, Pennsylvania, USA), where the inoculum was then administered. The frankfurters were spot inoculated by dispersing 1 mL of the inoculation solution (5.86 × 106 CFU/mL) per frankfurter. After frankfurters were inoculated, each group was stored at 4°C for an hour to allow for attachment.
Following inoculation, frankfurters were submerged in their respective antimicrobial treatments. Frankfurters were placed in sterile containers with 20 mL of antimicrobial solution. There was a sample size of 44 frankfurters, with 4 replications. Each frankfurter was manually agitated for 1 min and allowed to rest for 2 min. After frankfurters were aseptically removed from the container containing the respective antimicrobial treatments, frankfurters were placed into sterile sample bags. Following removal, frankfurters were stomached for 1 min in 100 mL of nBPW so that injured, viable bacteria and biofilm forming bacteria could be recovered, without the continued action of the antimicrobials (Bourassa et al., 2019). The resulting rinsates were diluted 10−1-10−6 and spread plated on Oxford agar in duplicates. Plates were inverted and aerobically incubated for 24 h in 37°C. A detection limit of 30–300 colonies per plate was used.
Statistical Analysis
From the frankfurter study, only Listeria monocytogenes colony counts between 30 and 300 were recorded and log transformed. Log transformed colonies were utilized to determine total log reduction of TW and treatments from the inoculated control. Therefore, only log reductions were analyzed using One-Way ANOVA, Linear contrasts and Pairwise comparison in JMP 14.0 (SAS Institute, City, State, US). Linear contrasts were performed to determine if there was an effect of increasing the dosage of SBS or nisin on mean log reduction of L. monocytogenes. Means were separated using Tukey's protected HSD with a significance level of P ≤ 0.05.
Results
The goal of performing the MIC in the current study was to determine the range of antimicrobial concentration that would inhibit visible growth of Listeria monocytogenes. Therefore, the concentration of antimicrobials used in the current experiment with frankfurters was based on the clarity of the observed microtiterplate wells. The wells that were ruled clear were plated on Oxford agar to obtain the MBC. The MIC and MBC results indicated the use of concentrations 0.75 and 1.5% SBS and 0.5, 1, and 2%.
The results of the One-Way ANOVA suggest that there was a treatment effect (P < 0.0001, Table 1). All experimental treatments reduced the population of L. monocytogenes on frankfurters compared to the no treatment control (6.65 log CFU/mL). Reduction was calculated by subtracting the Log CFU/mL of treatment from the average Log CFU/mL of the No treatment (NT) frankfurters. The mean reduction of the individual treatments 0.5, 1, and 2% nisin (1.99, 2.47 and 2.42 log CFU/mL) and 0.75 and 1.5% SBS (2.19 and 2.29 CFU/mL) were not different from one another. All treatments except 0.5% nisin and TW (1.99 and 1.23 log CFU/mL) had a significant reduction of L. monocytogenes on frankfurters compared to the NT control (6.65 log CFU/mL, respectively). Overall, the greatest numerical reduction of L. monocytogenes compared to the NT control was demonstrated in the use of 1.5% SBS plus 1% nisin and 1.5% SBS plus 2% nisin as the use of both combinations resulted in 2.5 log CFU/mL reduction of L. monocytogenes (2.66 and 2.61 log CFU/mL). Although there was a numerical difference exhibited by treatments, there was no indicated statistical separation between experimental treatments except tap water. Therefore, further statistical analyses were utilized to determine differences between experimental treatments.
The linear trends of two SBS concentrations, 0.75 and 1.5%, on reducing Listeria monocytogenes on frankfurters were investigated for the increasing concentrations of SBS within treatments (P < 0.0001, R2 = 0.84, Table 2). There was a linear difference between the TW and treatments. Both SBS treatments (0.75 and 1.5%) demonstrated a linear trend. However, there was no difference between the two treatments when compared to each other. The linear effects of the three nisin concentrations, 0.5, 1.0, and 2%, on reducing Listeria monocytogenes on frankfurters was also investigated (P < 0.0001, R2 = 0.70, Table 3). It was demonstrated that TW and nisin at 0.5% are similar, whereas nisin treatments 1 and 2% are similar to each other but statistically different from the other treatments.
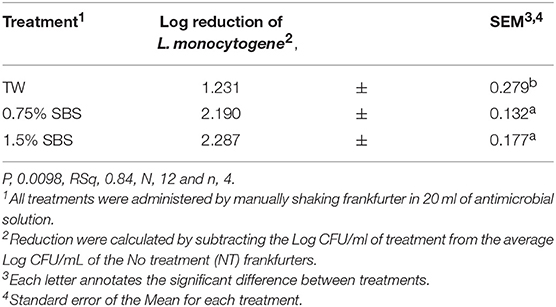
Table 2. Linear Effect two SBS concentrations, 0.75 and 1.5%, on reducing Listeria monocytogenes on frankfurters.
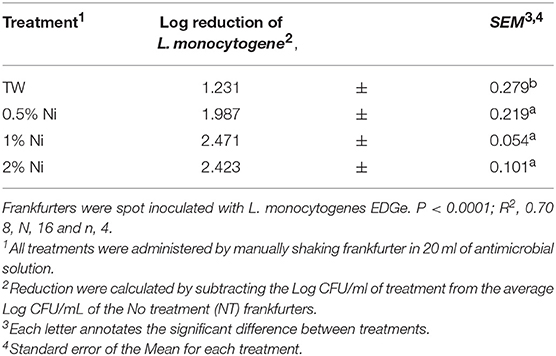
Table 3. The linear effects of three nisin concentrations, 0.5, 1, and 2%, on reducing Listeria monocytogenes on frankfurters.
The effectiveness of the SBS treatments was further analyzed by conducting a Pairwise comparison. The effect of 0.75% of SBS used alone or in combination with varying concentrations of nisin was investigated (P < 0.0001, Table 4). Compared to TW, 0.75% SBS was statistically different. However, all other treatments were not different as 0.75% SBS demonstrated similar effects as 0.5, 1, 2% nisin and the combinations of 0.75% SBS with 0.5 and 1% nisin. The effects of 1.5% of SBS used alone or in combination with varying concentrations of nisin were also compared (P < 0.0001, Table 5). Although not statistically different, the results showed that 1.5% SBS and 1.5% SBS in combination with 2% nisin yielded the lowest population of L. monocytogenes per mL. All 1.5% SBS treatments were statistically different from the TW but, all 1.5% SBS treatments (1% nisin, 2% nisin, Combination with nisin 1 and 2%) were statistically the same. There were no visual changes observed among treated frankfurters.
Discussion
In the current study, frankfurters were submerged into antimicrobial solutions and evaluated for the ability to diminish post-processing L. monocytogenes contamination. Therefore, only frankfurters made with no additives were obtained from a local supermarket and artificially inoculated with L. monocytogenes. Though there was potential for residual antimicrobials, the inoculation and use of controls minimized the influence these factors had on the results of the current study to be nominal.
The purpose of this study was to evaluate the effect of potential alternative antimicrobials of SBS and nisin on frankfurters. There were 11 treatments comprised of SBS and nisin utilized alone or in various combinations. The treatment of frankfurters with 1.5% SBS + 2% nisin exhibited the lowest population of L. monocytogenes. There was a 2–2.5 log reduction in all treatments compared to the NT. These results demonstrate that a bactericidal treatment such as SBS and nisin alone or in combination, at all concentrations, were able to reduce the growth and, thus, reduce the risk of L. monocytogenes on RTE meats such as frankfurters.
SBS was used to examine its potential antimicrobial effect against controlling Listeria in frankfurters. L. monocytogenes has been reported to be inhibited by lowering the pH, creating an adverse environment (Tienungoon et al., 2000). Similar to organic acids, SBS has the ability to reduce the pH level of surrounding materials once it is applied (Micciche et al., 2019). With a pKa of 1.96, SBS can be effective in reducing L. monocytogenes on frankfurters. Throughout this study, SBS treatments reduced the concentration of L. monocytogenes. Similar results have been demonstrated using organic acids to control Listeria. According to Martin et al. (2010), Lactic acid bacteria (LactiGuardTM), were able to reduce L. monocytogenes population by 2 log CFU/g2 on frankfurters formulated with lactate/diacetate. It has been reported that organic acids alleviate intracellular pH more effectively than inorganic acids, which can cause differences in reduction (Papatsiros and Billinis, 2012). However, L. monocytogenes responses vary between organic and inorganic acids to maintain pH homeostasis, which in turn causes some pathogenic strains of Listeria monocytogenes to be resistant (Cheng et al., 2015). Therefore, SBS could be considered a desired alternative because of antimicrobial resistance to organic acids.
Due to the growing concern surrounding the use of synthetic food additives in the US (Devcich et al., 2007), the addition of SBS could be a novel antimicrobial. It is Generally Recognized as Safe (GRAS) (United Stated Department of Agriculture, 2015), and gives off a slightly less salty taste than organic acids such as lactic and acetic acid (Kim et al., 2018). Furthermore, SBS has been declared as a safer alternative choice as an antimicrobial by the [United States Environmental Protection Agency (EPA), 2018; United States Food and Drug Administration, 2018]. Currently, there are no regulations for SBS used in RTE meat products. There is limited literature on the application of SBS on RTE meat but, SBS has been reported to be able to reduce microorganisms in multiple environments. According to Kim et al. (2018), SBS exhibits an antimicrobial effect on whole apples inoculated with Listeria innocua. Kim et al. (2018) observed a 3.66 log reduction of Listeria innocua to 30 min exposure with 3% SBS in combination with 60 ppm peracetic acid. Although reduction differences exist, the current study used lower concentration of SBS (1.5 and 0.75%). Different strains of Listeria could also potentially affect the antimicrobial activity of SBS. Therefore, more mechanistic studies must be conducted using SBS to understand its antimicrobial effect on Listeria fully.
Research has also demonstrated that the efficacy of nisin at reducing populations of Listeria in RTE meat (Leverentz et al., 2003). Results from Uesugi and Moraru (2009) support the findings in the current study that the treatment of frankfurters with nisin results in an initial log reduction in L. monocytogenes levels, as they reported a 2.35 log reduction in RTE sausage. According to Naas et al. (2013), nisin along with modified packaging possessed synergistic qualities for controlling the growth of Listeria on RTE turkey bologna. Ruiz et al. (2010) also observed a 4 log reduction in Listeria growth when nisin was applied on RTE turkey slices. Therefore, the authors of the current study investigated the potential synergistic effect between SBS and nisin for controlling populations of Listeria on artificially inoculated frankfurters.
Synergistic interaction has been defined as a 2 log CFU/mL decrease between the combinations of both antimicrobials compared to their individual antimicrobial effect (Dong et al., 2016; Wei et al., 2016). The combination treatments showed a greater decrease of Listeria population than all other treatments. Though both solutions demonstrated antimicrobial activity, there was no detected synergism between the combination of SBS and nisin. Furthermore, the quantities of both antimicrobials when combined appeared to be capable of reducing Listeria without any change in the visual appearance of the frankfurter.
Conclusion
The results suggest that incorporating SBS and nisin as an antimicrobial dip on frankfurters during post production has the capability to reduce L. monocytogenes populations on artificially inoculated frankfurters. In the current study, there was a significant difference between all treatments as Listeria population reduced 2.0–2.5 log CFU/mL, with the combination of 1% SBS and 2% nisin demonstrated the greatest log CFU/ml reduction compared to the NT control. Additionally, there were no synergistic effects demonstrated by combining SBS and nisin. These findings from the study suggest SBS or nisin alone, or in combination are effective antimicrobial agents for controlling L. monocytogenes on frankfurters and could be considered for alternative use to current antimicrobials used in the food industry today. Further research is necessary to determine the full efficacy of various concentrations of SBS on frankfurters on diminishing the populations of other Listeria strains. Furthermore, to elucidate the long-term effects of SBS, a shelf life study should be conducted to determine if SBS has the capability to not only reduce pathogens associated with RTE meats but to extend the shelf life of frankfurters. As a result, these findings will help further understand the antimicrobial efficiency of SBS for controlling Listeria in RTE food products.
Author Contributions
All authors discussed results and contributed to the final manuscript. AB, SR, KF, and CK created the protocol for the current study. AB performed the study. AB and DD analyzed the data. AB wrote the current manuscript with the assistance from DD, KF, and SR.
Conflict of Interest Statement
CK is employed by the company Jones-Hamilton Co., Tracy Road, Walbridge, OH.
The remaining authors declare that the research was conducted in the absence of any commercial or financial relationships that could be construed as a potential conflict of interest.
Acknowledgments
The author DD would like to acknowledge the Graduate College at the University of Arkansas for its support through the Distinguished Academy Fellowship and the continued support from the Center for Advanced Surface Engineering, under the National Science Foundation Grant No. OIA-1457888 and the Arkansas EPSCoR Program, ASSET III. Finally, the authors would like to thank Jones-Hamilton Co. for its support and assistance in completing this research.
References
Bourassa, D. V., Lapidus, J. L., Kennedy-Smith, A. F., and Morey, A. (2019). Efficacy of neutralizing buffered peptone water for recovery of Salmonella, Campylobacter, and Enterobacteriaceae from broiler carcasses at various points along a commercial immersion chilling process with peroxyacetic acid. J. Poultry Sci. 98, 393–397. doi: 10.3382/ps/pey361
Center for Disease Control and Prevention (CDC). (2019). Listeria Outbreaks. Available online at: https://www.cdc.gov/listeria/outbreaks/index.html
Chan, Y. C., and Wiedmann, M. (2008) Physiology and genetics of Listeria monocytogenes survival and growth at cold temperatures. Critic. Rev. Food Sci. Nutr. 49, 237–253. doi: 10.1080/10408390701856272
Cheng, C., Yang, Y., Dong, Z., Wang, X., Fang, C., Yang, M., et al. (2015). Listeria monocytogenes varies among strains to maintain intracellular pH homeostasis under stresses by different acids as analyzed by a high-throughput microplate-based fluorometry. Front. Microbiol. 6:15. doi: 10.3389/fmicb.2015.00015
Devcich, D. A., Pedersen, I. K., and Petrie, K. J. (2007). You eat what you are: modern health worries and the acceptance of natural and synthetic additives in functional foods. Appetite 48, 333–337. doi: 10.1016/j.appet.2006.09.014
Dong, J., Ruan, J., Xu, N., Yang, Y., and Ai, X. (2016). In vitro, synergistic effects of fisetin and norfloxacin against aquatic isolates of Serratia marcescens. FEMS Microbiol. Lett. 363:1. doi: 10.1093/femsle/fnv220
Economic Research Service (ERS) U. S. Department of Agriculture (USDA). (2014). Cost Estimates of Foodborne Illnesses. Available online at: http://ers.usda.gov/data-products/cost-estimates-of-foodborne-illnesses.aspx
Geornaras, I., Skandamis, P. N., Belk, K. E., Scanga, J. A., Kendall, P. A., Smith, G. C., et al. (2006). Postprocess control of Listeria monocytogenes on commercial frankfurters formulated with and without antimicrobials and stored at 10°C. J. Food Protect. 69, 53–61. doi: 10.4315/0362-028X-69.1.53
Goulet, V., Hebert, M., Hedberg, C., Laurent, E., Vaillant, V., De Valk, H., et al. (2012). Incidence of listeriosis and related mortality among groups at risk of acquiring listeriosis. Clin. Infect. Dis. 54, 652–660, doi: 10.1093/cid/cir902
Kim, S. A., Knueven, C., Basel, R., and Ricke, S. C. (2018). A decontamination approach using a combination of bisulfate of soda and peracetic acid against Listeria innocua inoculated on whole apples. J. Food Control 84, 106–110. doi: 10.1016/j.foodcont.2017.07.036
Koseki, S., Mizuno, Y., and Yamamoto, K. (2007). Predictive modelling of the recovery of Listeria monocytogenes on sliced cooked ham after high pressure processing. Int. J. Food Microbiol. 119, 300–307. doi: 10.1016/j.ijfoodmicro.2007.08.025
Leverentz, B., Conway, W. S., Camp, M. J., Janisiewicz, W. J., Abuladze, T., Yang, M., et al. (2003). Biocontrol of Listeria monocytogenes on fresh-cut produce by treatment with lytic bacteriophages and a bacteriocin. Appl. Environ. Microbiol. 69, 4519–4526. doi: 10.1128/AEM.69.8.4519-4526.2003
Lourenco, A., Kamnetz, M. B., Gadotti, C., and Diez-Gonzales, F. (2016). Antimicrobial treatments to control Listeria monocytogenes in queso fresco. Food Microbiol. 64, 47–55. doi: 10.1016/j.fm.2016.12.014
Martin, E. M., Griffis, C. I., Vaughn, K. L. S., O'Bryan, C. A., Friendly, E. C., Marcy, J. A., et al. (2009). Control of Listeria monocytogenes by lauric arginate on frankfurters formulated with or without lactate/diacetate. J. Food Sci. 74, M237–M241. doi: 10.1111/j.1750-3841.2009.01196.x
Martin, E. M., O'Bryan, C. A., Lary, R. Y., Griffis, C. I., Vaughn, K. L. S. C., et al. (2010). Spray application of liquid smoke to reduce or eliminate Listeria monocytogenes surface inoculated on frankfurters. Meat Sci. 85, 640–644. doi: 10.1016/j.meatsci.2010.03.017
Mateus, T., Silva, J., Maia, R. L., and Teixeira, P. (2013). Listeriosis during pregnancy: a public health concern. ISRN Obstetrics Gynecol. 2013:851712. doi: 10.1155/2013/851712
McLauchlin, J., Mitchell, R. T., Smerdon, W. J., and Jewell, K. (2004). Listeria monocytogenes and listeriosis: a review of hazard characterization for the use in microbiological risk assessment. Int. J. Food Sci. 92, 15–33. doi: 10.1016/S0168-1605(03)00326-X
Micciche, A. C., Feye, K. M., Rubinelli, P. M., Lee, J. A., Knueven, C. J., and Ricke, S. C. (2019). Comparison of acid sanitizers on Salmonella Typhimurium inoculated commercial poultry processing reuse water. Front. Sustain. Food Syst. 2:90 doi: 10.3389/fsufs.2018.00090
Naas, H., Martinez-Dawson, R., Han, I., and Dawson, P. (2013). Effect of combining nisin with modified atmosphere packaging on inhibition of Listeria monocytogenes in ready-to-eat turkey bologna. Poultry Sci. 92, 1930–1935. doi: 10.3382/ps.2012-02141
Orsi, R. H., and Wiedmann, M. (2016). Characteristics and distribution of Listeria spp., including Listeria species newly described since 2009. Appl. Microbiol. Biotechnol. 100, 5273–5287. doi: 10.1007/s00253-016-7552-2
Papatsiros, G., and Billinis, C. (2012). “The prophylactic use of acidifiers as antibacterial agents in swine,” in Antimicrobial Agents, ed V. Bobbarala (Rijeka: InTech).
Ramaswamy, V., Cresence, V. M., Rejitha, J. S., Lekshmi, M. U., Dharsana, K. M., Prasad, S. P., et al. (2007). Listeria — review of epidemiology and pathogenesis. J. Microbiol. Immunol. Infect. 40, 4–13.
Ruiz, A., Williams, S. K., Djeri, N., Hinton, A., and Rodrick, G. E. (2010). Nisin affects the growth of Listeria monocytogenes on ready-to-eat turkey ham stored at four degrees Celsius for sixty-three days. Poultry Sci. 89, 353–358. doi: 10.3382/ps.2008-00503
Ryser, E. T., Arimi, S. M., and Donnelly, C. W. (1997). Effects of pH on distribution of Listeria ribotypes in corn, hay, and grass silage. Appl. Environ. Microbiol. 63, 3695–3697.
Ryser, E. T., and Marth, E. H. (ed.). (2007). Listeria, Listeriosis, and Food Safety- 2nd ed. New York, NY: Marcel Dekker.
Saha, M., Debnath, C., and Pramanik, A. K. (2015). Listeria monocytogenes: an emerging food borne pathogen. Int. J. Curr. Microbiol. Appl. Sci. 4, 52–72. doi: 10.3855/jidc.6616
Seman, D. L., Boler, D. D., Carr, C. C., Dikeman, M. E., Owens, C. M., Keeton, J. T., et al. (2018). Meat science lexicon. Meat Muscle Biol. 2, 1–15. doi: 10.22175/mmb2017.12.0059
Swaminathan, B., and Gerner-Smidt, P. (2007). The epidemiology of human listeriosis. Microbes Infect. 9, 1236–1243. doi: 10.1016/j.micinf.2007.05.011
Thompson, R. L., Carpenter, C. E., Martini, S., and Broadbent, J. R. (2008). Control of Listeria monocytogenes in ready-to-eat meats containing sodium levulinate, sodium lactate, or a combination of sodium lactate and sodium diacetate. J. Food Sci. 73, M239–M244. doi: 10.1111/j.1750-3841.2008.00786.x
Tienungoon, S., Ratkowsky, D. A., McMeekin, T. A., and Ross, T. (2000). Growth limits of Listeria monocytogenes as a function of temperature, pH, NaCl, and lactic acid. Appl. Environ. Microbiol. 66, 4979–4987.
Uesugi, A. R., and Moraru, C. I. (2009). Reduction of Listeria on ready-to-eat sausages after exposure to a combination of pulsed light and Nisin. J. Food Protect. 72, 347–353. doi: 10.4315/0362-028x-72.2.347
United Stated Department of Agriculture. (2015). Sodium Bisulfate - Agricultural Marketing Service. Available online at: https://www.ams.usda.gov/sites/default/files/media/Sodium%20Bi%20report%202015.pdf (accessed May 26, 2018)
United States Department of Agriculture, Food Safety and Inspection Service. (2017). FSIS Microbiological Testing Program for Ready-to-Eat (RTE) Meat and Poultry Products, 1990–2017. Available online at: https://www.fsis.usda.gov/wps/portal/fsis/topics/data-collection-and-reports/microbiology/testing-program-for-rte-meat-and-poultry-products/testing-program-rte
United States Environmental Protection Agency (EPA) (2018). Safer Chemical Ingredients List. Available online at: https://www.epa.gov/saferchoice/safer-ingredients (accessed September 25, 2018)
United States Food and Drug Administration (2018). GRAS Substances (SCOGS) Database. Available online at: https://www.fda.gov/food/ingredientspackaginglabeling/gras/scogs/default.htm (accessed August 31, 2018).
United States Food and Drug Administration, Food Safety and Inspection Service. (2016). New Neutralizing Buffered Peptone Water to Replace Current Buffered Peptone Water for Poultry Verification Sampling. FSIS Notice 41-16. Available online at: https://www.fsis.usda.gov/wps/portal/fsis/topics/regulations/fsis-notice
Wang, Y., Lu, L., Lan, R., Salazar, J. K., Liu, J., Xu, J., et al. (2017). Isolation and characterization of Listeria species from rodents in natural environments in China. Emerg. Microbes Infect. 6:e44. doi: 10.1038/emi.2017
Wei, C., Chang, S., Shien, J., Kuo, H., Chen, W., and Chou, C (2016) Synergism between two amphenicol of antibiotics, florfenicol and thiamphenicol, against Staphylococcus aureus. Veter. Rec. 178:319 doi: 10.1136/vr.103554
Yehia, H. M., Ibraheim, S. H., and Hassanein, W. A. (2016). Prevalence of Listeria species in some foods and their rapid identification. Tropic. J. Pharma. Res. 15, 1047–1052. doi: 10.4314/tjpr.v15i5.21
Zhu, M., Du, M., Cordray, J., and Uk Ahn, D. (2005). Control of Listeria monocytogenes contamination in Ready-to-Eat meat products. Comprehens. Rev. Food Sci. Food Safety 4, 34–42. doi: 10.1111/j.1541-4337.2005.tb00071.x
Keywords: nisin, bisulfate of soda, synergistic, Listeria monocytogenes, frankfurters
Citation: Bodie AR, Dittoe DK, Feye KM, Knueven CJ and Ricke SC (2019) Application of an Alternative Inorganic Acid Antimicrobial for Controlling Listeria monocytogenes in Frankfurters. Front. Sustain. Food Syst. 3:34. doi: 10.3389/fsufs.2019.00034
Received: 22 January 2019; Accepted: 25 April 2019;
Published: 14 May 2019.
Edited by:
Karl Matthews, Rutgers University, The State University of New Jersey, United StatesReviewed by:
Joseph D. Eifert, Virginia Tech, United StatesCangliang Shen, West Virginia University, United States
Copyright © 2019 Bodie, Dittoe, Feye, Knueven and Ricke. This is an open-access article distributed under the terms of the Creative Commons Attribution License (CC BY). The use, distribution or reproduction in other forums is permitted, provided the original author(s) and the copyright owner(s) are credited and that the original publication in this journal is cited, in accordance with accepted academic practice. No use, distribution or reproduction is permitted which does not comply with these terms.
*Correspondence: Steven C. Ricke, c3JpY2tlQHVhcmsuZWR1