- 1International Iberian Nanotechnology Laboratory, Braga, Portugal
- 2Centre of Biological Engineering, University of Minho, Braga, Portugal
- 3Instituto de Biologia Experimental e Tecnológica, Oeiras, Portugal
The present work aims at developing and characterizing a modified galactomannan for the production of nano-sized micelles through the solvent diffusion method and its evaluation as a new carrier for hydrophobic compounds. Guar gum galactomannan was acetylated and a new polymer with different degrees of acetylation was obtained. The acetylation and substitution degree of the polymer was determined and characterized by means of Fourier transform infrared spectroscopy and thermogravimetric analysis. Galactomannan acetate (GA) was evaluated for development of micelles through the solvent diffusion method. Critical micellar concentration (CMC) was evaluated through dynamic light scattering where the effect of a wide range of GA concentrations in the micelle formation was studied. Results showed that CMC is around 0.2 mg mL−1 and an increase of GA concentration leads to the formation of monodisperse micelles with low values of polydispersity. The influence of the degree of acetylation was evaluated in terms of size average, polydispersity (PdI) and zeta potential. Transmission electron microscopy was used to evaluate micelles' morphology. It was shown that GA can be used to form micelles for the encapsulation of curcumin (used as model compound) with an encapsulation efficiency higher than 99% and a loading capacity ranging from 5.5 to 7.7%. Results showed that the degree of acetylation can be used to control particle size: micelles produced from GA with the lowest degree of acetylation have a diameter which is around 25% smaller than that of micelles produced from GA with the highest degree of acetylation. This study provides information concerning the preparation of polymeric micelles based on a modified natural galactomannan for application in the food and pharmaceutical industry. To our knowledge, it is the first work dealing with galactomannans' modification.
Introduction
Polymeric micelles formed by the self-assembly of amphiphilic polymers in aqueous solution have received a great attention in the last 15 years. Such polymers are composed by block copolymers or hydrophobically modified water-soluble polymers. They present as main advantage the fact that self-aggregates are formed without a cross-linker and the incorporation of the bioactive compound can be achieved without degradation. Normally, the obtained polymeric micelles are composed by a hydrophobic core, which can act as a reservoir for hydrophobic compounds, and a hydrophilic outer shell that is responsible by the dispersion of the micelles in an aqueous ambient (Duval-Terrié et al., 2003; Hassani et al., 2012; Yang et al., 2018a). The hydrophilic outer shell can form hydrogen bonds with the aqueous surrounding medium forming a shell around the micellar core, while the hydrophobic compounds are entrapped in the core and transported at concentrations that can exceed their intrinsic water solubility.
Recently, naturally derived biodegradable materials have attracted more attention for polymeric micelles production due to their unique characteristics (e.g., non-toxicity, biocompatibility). Modification of natural polysaccharides is a new strategy for preparing environmentally friendly biomaterials and biopolymers with special properties which can extend their applications (Zhang et al., 2009). Polysaccharides are a good alternative to synthetic compounds for the formation of micelles due to their biocompatibility, biodegradability, hydrophilicity and protective properties, together with a high micelle stability achieved (Duval-Terrié et al., 2003; Hassani et al., 2012).
Galactomannans are neutral, water-soluble polysaccharides that due to their economical, biocompatible, non-toxic, biodegradable features, have been used as functional biopolymers for applications in papermaking, food and pharmaceutical industry (Vendruscolo et al., 2005; Scaman et al., 2006; Cerqueira et al., 2009, 2011a). However, to our knowledge until now never a chemically-modified amphiphilic galactomannan has been developed with the objective of improving its hydrophobicity aiming at the development of polymeric micelles for hydrophobic compounds encapsulation.
Functional compounds with potential to be applied in the pharmaceutical and food industries often have poor solubility in aqueous solution, being this the major barrier for their industrial application, influencing bioavailability and efficacy (Kuan et al., 2011; Onwulata, 2012). Curcumin, a polyphenol present in perennial herb Curcuma longa L. has been one of the most studied nutraceuticals. It has been shown a great range of interesting properties such as: antioxidant, anti-inflammatory and antimicrobial capacity as well as the ability to suppress the proliferation of a wide variety of tumor cells (Menon and Sudheer, 2007; Jiao et al., 2009; Kilian et al., 2012). Curcumin is hydrophobic in nature, being insoluble in water but soluble in ethanol, dimethylsulfoxide and acetone. One of the main barriers in its bioavailability and clinical efficacy is its poor water-solubility and rapid metabolism (Anand et al., 2007; Silva et al., 2018).
Attempts have been made to increase its solubility and bioavailability through encapsulation in nanoemulsions, liposomes, polymeric nanoparticles, biodegradable microspheres, cyclodextrin, and hydrogels (Das et al., 2010; Li et al., 2012; Yu et al., 2012; Tikekar et al., 2013; Silva et al., 2018).
The demand for new structures targeted at the delivery of functional compounds is increasing, where the utilization of new modified natural polymers with this purpose is one of the challenges (Jacob et al., 2018; Shishir et al., 2018). In this work, galactomannan acetate (GA) with different acetylation degrees was used for the development of micelles, that were characterized in terms of average size, polydispersity (PdI) and zeta potential; and were used as a vehicle for the encapsulation of curcumin (hydrophobic model compound), being the encapsulation efficiency and loading capacity determined.
Materials and Methods
Materials
Guar gum galactomannan, dimethyl sulfoxide (DMSO) and curcumin (≥94% of curcuminoid and ≥80% of curcumin) were purchased from Sigma-Aldrich (USA). Formamide (99.5%) and acetic anhydride (≥97%) were obtained from Acros Organics (Belgium). Sodium hydroxide, hydrochloric acid (37%), potassium bromide, ethanol (≥97%) and pyridine were obtained from Panreac Química (Spain).
Synthesis of Galactomannan Acetate With Different Degrees of Acetylation
Galactomannan acetate (GA) was synthesized as described by Motozato et al. (1986) with some modifications. Briefly, 2 g of galactomannan were dissolved in 50 mL of formamide at 54°C. Then 12 mL of pyridine and three different volumes of acetic anhydride (10, 20, and 30 mL) were added and the mixture was stirred at 54°C for 48 h. A dark brown precipitate was obtained and was then purified by precipitation with two volumes of 500 mL of distilled water. The solid material was dried for 24 h at 35°C and a white powder was obtained. The samples were stored in the absence of light until further utilization. The samples were denominated GA1, GA2, and GA3 when treated with 10, 20, and 30 mL of acetic anhydride, respectively. Two replicates were performed for each sample.
Fourier Transform-Infrared (FTIR) Spectroscopy
Fourier transform-infrared (FTIR) spectroscopy was used to confirm the acetylation of modified galactomannan (GA). IR spectra of the galactomannan and of GA were determined using a Fourier transform infrared spectrometer (Perkin-Elmer 16 PC spectrometer, Boston, USA). The material was ground with spectroscopic grade potassium bromide powder and then pressed into 1 mm pellets. FTIR measurement was performed in the wavenumber range of 400 and 4,000 cm−1 using 16 scans. Each spectrum was baseline corrected and the absorbance was normalized between 0 and 1. Two replicates were performed for each sample.
Degree of Acetylation and Substitution of Galactomannan Acetate
The degree of acetylation was determined by titration based in the ASTM D871-96 (2019) with some modifications as suggested by Garcia et al. (2008). Briefly, 1 g of the GA was dried at 105°C during 3 h, mixed with 40 mL of ethanol (75%) and heated at 50°C during 30 min. Then 40 mL of 0.5 mol L−1 NaOH was added and the mixture heated again at 50°C during 15 min. The samples were left during 72 h at room temperature and then titrated with 0.5 mol L−1 HCl using phenolphthalein as the indicator. After this first titration an excess of 1 mL of 0.5 g mol−1 HCl was added and the solutions were left overnight at room temperature. The degree of acetylation (%Acetyl) and substitution (DS) were determined using Equations 1 and 2, where Va and Vb represents volume of HCl for the blank and for the sample, respectively, NHCl is the normality of HCl, MacetyI is molecular weight of the acetyl group, and msample is the weight of the sample in grams (Laignel et al., 1997). At least two replicates were performed for each sample.
Thermogravimetric Analyses
Thermogravimetric analyses (TGA) were carried out using a Shimadzu TGA-50 (Shimadzu Corporation, Kyoto, Japan) in N2 atmosphere and calibrated with Indium as standard. Analyses were started at 20°C and continued up to 580°C, with a linear increase of 10°C min−1. Samples were weighed (approximately 5–10 mg of dry matter) in aluminum pans (Al crimp Pan C.201-52943) being the empty pans used as a reference. Data were treated using TASYS software (Shimadzu Corporation, Kyoto, Japan). At least two replicates were performed for each sample.
Preparation of Galactomannan Acetate Micelles (GAM)
The solvent diffusion method was used for micelles formation according to methodology suggested by Zhang et al. (2009). Briefly, GA was weighted and dissolved in DMSO with agitation during 12 h. Then, the solution was added to an aqueous solution using a syringe pump (NE: 1000, USA) at a flow rate of 0.2 mL min−1, using a 1 mL syringe with a needle of 0.45 mm of diameter.
Critical Micellar Concentration (CMC) of Acetate Galactomannan
Critical micellar concentration (CMC) was evaluated through dynamic light scattering (DLS), measuring the intensity of scattered light using a Zetasizer, Nano ZS (Malvern Instruments, UK) (Rangelov et al., 2004; Minimol et al., 2013). Different concentrations (ranging between 0.001 and 1 mg mL−1) of GA were prepared in DMSO and were used for micelle formation in water [please see section Preparation of Galactomannan Acetate Micelles (GAM)]. The evaluation of intensity data was based on the detected scattering; for concentrations below the CMC scattering was similar to that of distilled water.
Size Average, Zeta Potential, and Polydispersity Index
The size average, zeta potential (Zp) and polydispersity index (PdI) were determined by DLS (Zetasizer Nano ZS, Malvern Instruments, UK). Each sample was analyzed in a folded capillary cell. The measurements were made in triplicate, with three readings for each of them. The results are given as the average of the nine values obtained.
Encapsulation of Curcumin in Galactomannan Acetate Micelle
Curcumin-Ioaded galactomannan acetate micelles (GAM) were prepared by adding different concentrations of curcumin (between 2 and 0.5 mg mL−1) to GA dissolved in DMSO. In order to form GAM by the solvent diffusion method [please see section Preparation of Galactomannan Acetate Micelles (GAM)]a pre-prepared solution was added to an aqueous solution.
Encapsulation efficiency (EE) and loading capacity (LC) of curcumin in micelles were determined by measuring the amount of curcumin that was not encapsulated in the micelles. Briefly, after the preparation of the curcumin-loaded GAM, 0.5 mL of the solution was placed in Eppendorf Amicon® Ultra 0.5 centrifugal filters with a cut-off of 10 kDa (Millipore, USA) and centrifuged at 9,500 g for 10 min. This process allows separating the encapsulated curcumin from free curcumin in the supernatant. The amount of curcumin in the supernatant was determined by UV/Vis absorbance at 427 nm, being the EE and LC calculated by the following equations:
where [curcum]i represents the initial concentration of curcumin and [curcum]f the final concentration in the supernatant. Three replicates were performed for each sample.
Transmission Electron Microscopy
The morphology of nanoparticles was observed by transmission electron microscopy (EM 902nd, Zeiss, Germany). A drop of solution containing 2% (w/v) of phosphotungstic acid was placed on copper grids for observation. The samples were air-dried before observation.
Statistical Analyses
Statistical analyses were performed using the analysis of variance procedure with SigmaPlot 11.0 software for Windows (free trial version). Tukey's test was applied to detect differences of means, and p < 0.01 was considered to be statistically significant.
Results and Discussion
Galactomannan Acetate Synthesis and Characterization
Table 1 shows the values of degree of acetylation (%acetyl) and degree of substitution (DS) of samples GA1, GA2, and GA3, which represent the modified galactomannan obtained with the utilization of acetic anhydride volumes of 10, 20, and 30 mL, respectively. The replacement of hydroxyl groups present in the galactomannan structure occurs during the acetylation by acetate groups, so it is expected that higher volumes of acetic anhydride lead to a higher substitution of hydroxyl groups by acetate groups. The obtained results are in agreement with the ones reported by other authors using pullulan, where DS values of 3.00, 2.84, and 2.71 were obtained when the polysaccharide was treated with 15, 10, and 7.5 mL of acetic anhydride, respectively (Zhang et al., 2009).
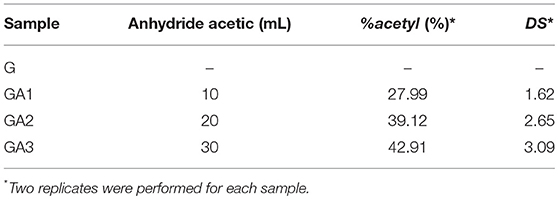
Table 1. Acetylation degree (%acetyl), degree of substitution (DS), for unmodified galactomannan (G) and galactomannan treated with different volumes of anhydride acetic (GA1, GA2, and GA3).
Successful acetylation of the galactomannan was confirmed by FTIR spectroscopy. Figure 1 shows FTIR spectra for unmodified galactomannan and galactomannan acetate (GA) with different degrees of acetylation. The spectrum of unmodified galactomannans shows broad bands ranging between 3,500–3,100 and 3,000–2,800 cm−1 that are attributed to OH stretching and to C–H stretching vibration, respectively (Cerqueira et al., 2011b). The broad band between 1,134 and 983 cm−1 is a characteristic contribution of C–OH bending, while the peak at 1,152 cm−1 corresponds to bending vibrational modes of C–O, present in the pyranose ring. The broad band between 1,198 and 983 cm−1 is characteristic of the stretching vibration of C–O in C–OH bonds, which is related with glycosidic bonds from the galactomannans' sugar composition. The presence of anomeric configurations and glycosidic linkages, attributed to α-D-galactopyranose and β-D–mannopyranose units are represented by peaks at 815 and 872 cm−1, respectively (Cerqueira et al., 2011b).
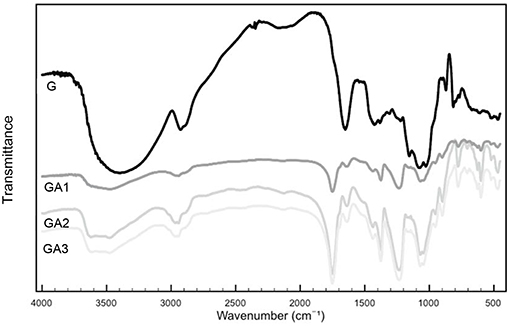
Figure 1. Fourier transform infrared spectra in the spectral region of 400 and 4,000 cm−1 of unmodified galactomannan (G) and galactomannan acetate treated with 10 mL (GA1), 20 mL (GA2), and 30 mL (GA3) of acetic anhydride. Two replicates were performed for each sample.
The chemical modification where the hydroxyl groups of galactomannan's structure are replaced by acetyl groups, led to changes in IR spectra. The modifications are confirmed by the presence of the characteristic bands for acetyl group at 1,752 cm−1 (C = O), 1,375 cm−1 (CH3), and 598 cm−1 (O–C = O). Also the broad band between 3,500 and 3,100 cm−1 of GA became weak when compared with unmodified galactomannan, which is related with the replacement of hydroxyl groups by acetyl groups. Moreover, the peak at 1,752 cm−1, related with ester groups, increases for samples with higher acetylation degrees (Zhang et al., 2009). The differences between spectra of samples GA1, GA2, and GA3 are in agreement with the values obtained for degrees of acetylation (%acetyl) and substitution (DS), where the increase from GA1 to GA3 goes from 27.99 and 1.62 to 42.91 and 3.09, respectively, corresponds to a higher intensity of the characteristic peaks in modified galactomannan spectra.
Thermal properties of galactomannan acetate were studied in order to evaluate the effect of acetylation in the thermal stability of galactomannan's structure. Figure 2 allows evaluating the thermal stability of the galactomannan before and after modification of its structure. It can be seen that the thermal stability of galactomannan acetate (GA) samples is higher than the one obtained for unmodified galactomannan (G) sample, which presents a lower degradation temperature. G sample shows two decomposition stages, one near 100°C attributed to the loss of adsorbed and structural water (associated to the hydrophilic nature of the polysaccharide that results in a weight loss of ca. 11%) and a second mass loss event due to the polysaccharide's thermal decomposition at approximately 305.47°C (resulting in a weight loss of ca. 58%). For all GA samples it was observed only one mass loss event, corresponding to the polysaccharide's thermal decomposition, and the increase of acetylation degree did not change the thermal degradation properties, being the peak value ca. 390°C for all samples (resulting in a weight loss of ca. 80%). Results confirm that the substitution of the hydroxyl by acetyl groups in the galactomannan structure influenced the thermal stability, where the galactomannan acetate presents a more stable structure. This stability increase can be explained by the presence of acetyl groups which replaced the hydroxyl end groups. Acetyl groups prevented the first degradation at lower temperatures, which facilitates the depolymerization of the other functional groups. Therefore, the initial blocking of the hydroxyl end group blocks to some extent the second degradation process (Persenaire et al., 2001). Works with other polymers also showed that their acetylation lead to an increase of the thermal stability of the modified polymer (Hung et al., 2017; Yang et al., 2018b).
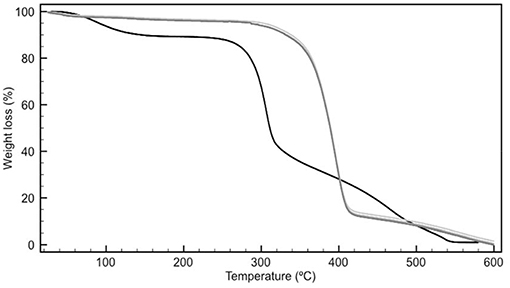
Figure 2. Thermogravimetric analysis of unmodified galactomannan (G —) and galactomannan acetate treated with 10 mL (GA1 —), 20 mL (GA2 —) and 30 mL (GA3 —) of acetic anhydride. Two replicates were performed for each sample.
Galactomannan Acetate Micelles
The development of micelles based in amphiphilic polysaccharides has been shown to produce well-defined, nano-sized micelles, spheres and particles (Zhang et al., 2009; Guo et al., 2012; Zhu et al., 2013). Most of the published works use phase separation-dialysis, ultrasounds or emulsification as production methods (Liu et al., 2011), however Zhang et al. (2009) showed that the solvent diffusion method could be used to form nanoparticles using amphiphilic polysaccharides, opening the possibility for a large scale production. Based on this, in the present work nano-sized micelles were formed when hydrophobized GA, soluble in a solvent (DMSO), were added to an aqueous solution promoting the solvation of the GA and the formation of micelles. This phenomenon is governed by the Marangoni effect that is related with interfacial turbulences that happen at the interface between solvent and non-solvent and are influenced, among other factors, by surface tension differences (Fessi et al., 1989). This phenomenon will condition micelles' formation, which is influenced by the galactomannan-solvent and water-solvent interactions that promote the formation of the core of the micelle (the hydrophobic part), being the hydrophilic part oriented out to the water phase. This process is highly influenced by the critical micellar concentration (CMC) that establishes the concentration of GA that leads to the formation of micelles.
Galactomannan acetate CMC was evaluated by DLS, where the data were related with the intensity of scattered light and the formation of micelles. At low concentrations of GA, the formation of micelles was not possible (lower scattering values, high PdI values, bi-modal and non-narrow size distribution) (results not shown), which can be explained by the fact that at low concentrations GA molecules were not associated, and the micelles were thus not formed. The CMC value obtained for GA was 0.2 mg mL−1. This value is within the same order of magnitude of values found in studies with other amphiphilic polymers that presented values around 0.1 mg mL−1 (Gonçalves et al., 2007; Guo et al., 2012).
Figure 3 shows that an increase of GA concentration leads to lower PdI values, indicating a more stable structure and a more monodisperse solution. As the concentration of GA increases, attractive and repulsive forces between the molecules are higher, causing self-aggregation that results in the formation of micelles. This phenomenon can be explained by the favored hydrophobic domain with the increase of GA concentrations, that gives rise to the formation of micelles with a hydrophobic core. These results are in line with findings for micelle nano-structures of octenyl succinic anhydride, where the increase of octenyl succinic anhydride concentration leads to more uniform and monodisperse micelles (Zhu et al., 2013). Table 2 shows the parameters obtained by fitting the data (the same data used to plot Figure 3) to a power-law model (Equation 5):
where κ and α are the parameters of the model.
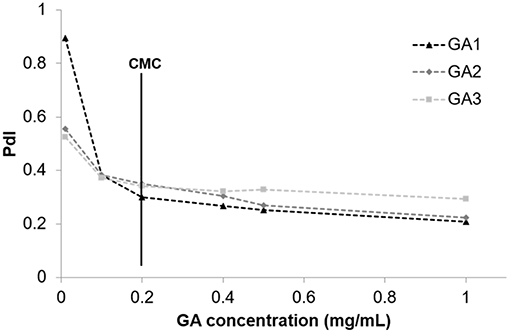
Figure 3. Polydispersity (PdI) of the micelles for increasing concentrations of galactomannan acetate (GA) with different acetylation degrees (GA1, GA2, and GA3).
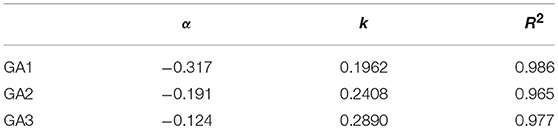
Table 2. Estimated power-law parameters for the variation of polydispersity with galactomannan acetate concentration for GA1, GA2, and GA3.
Results are in agreement with other published works where the relation between the aggregation factor (in this case given by PdI) and polymer concentration were fitted with a power-law model (Lee et al., 2002) and where it was shown that for higher polymer concentrations a lower aggregation of micelles and lower micelle size were obtained (Ranganathan et al., 2000). The obtained parameters show a relationship between the acetylation degree and the constant factor (κ) and the exponential factor (α), indicating that for low acetylation degree the GA concentration presents a more pronounced action in micelles' formation, while the utilization of a higher acetylation degree leads to the formation of micelles with lower concentrations of GA.
Based on the determination of CMC and according to the results presented, it was established that for concentrations higher than CMC it is possible to form stable micelles. Being so, a concentration of 1 mg mL−1 was used to study the influence of acetylation degree on zeta potential, size average, stability, encapsulation efficiency, and loading capacity of micelles.
Table 3 shows the values of size average and PdI of micelles produced using GA with different acetylation degrees. All micelles are in the nanometric range with PdI below 0.281. Results show that the increase of acetylation degree leads to higher values of size average, which can be explained by the hydrophobicity of the polymer (higher %acetyl and DS) that confers a more hydrophobic pattern to the GA structure (decreasing the ratio between head group and “tail”), thus influencing its solubility in aqueous solution and its aggregation behavior.
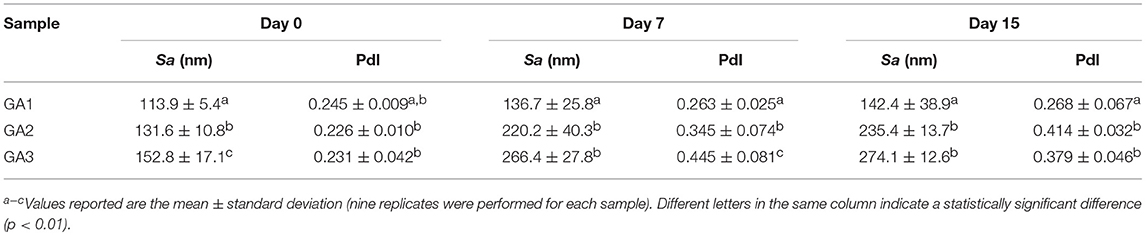
Table 3. Size average (Sa) and polydispersity (PdI) of micelles produced with galactomannan acetate with increasing acetylation degrees (GA1, GA2, and GA3) during storage.
Micelles presented a negative charge with values of zeta potential ranging between −14.8 and −18.1, and no statistically significant differences (p > 0.05) were found between micelles prepared with GA with different %acetyl (results not shown). The values obtained are in agreement (i.e., negative and lower than −30 mV) with the results presented by Gonçalves and Gama (2008) that presented values around −5.0 mV for hydrophobized dextrin. The low-negative zeta potential of micelles can be useful according to the application; some authors mentioned that low-negative zeta potential of PEGylated starch acetate nanoparticles could be desirable for particle uptake and drug absorption (Minimol et al., 2013). Moreover, the electrostatic interaction allows a repulsive force between micelles, resulting in a more stable system during storage. In fact, it is well-known that for the maintenance of the stability of nanoparticles the values of zeta potential should be ±30 mV, which is not the case of the developed micelles. Therefore, it is expected that over time some aggregation could happen, with the consequent increase of PDI values.
In order to evaluate this possibility, micelles' stability was evaluated during 15 days at 25°C. Table 3 shows the values of size average and PdI for micelles produced with GA1, GA2, and GA3. After 7 and 15 days of storage the values of size average and PdI increased for all samples, however for micelles prepared with GA presenting higher %acetyl (GA2 and GA3) this increase is higher comparing to the values obtained for micelles prepared with GA1. These results indicate that %acetyl and the DS influence micelles stability during storage.
Particle uptake and drug absorption are of high importance when the compound that is encapsulated presents low bioavailability, as is the case of curcumin. Curcumin is not soluble in water forming insoluble aggregates, however it is soluble in other solvents such as DMSO. One of the ways to improve curcumin solubility in aqueous solutions is the formation of micelles that encapsulate curcumin in a hydrophobic core whereas the external shell is water-soluble. In order to evaluate the suitability of GA micelles to encapsulate curcumin, the procedure used for micelle formation was used for curcumin encapsulation; the curcumin-loaded GA micelles were subsequently evaluated in terms of size average, PdI and their encapsulation efficiency (EE) and loading capacity (LC) were determined.
Table 4 shows values of size average, PdI, EE, and LC of micelles produced using GA for increasing %acetyl. Curcumin-loaded GA micelles present similar (p > 0.01) size average and PdI when compared with unloaded micelles (please compare Tables 3, 4), indicating that curcumin-loaded and unloaded micelles have similar behaviors.
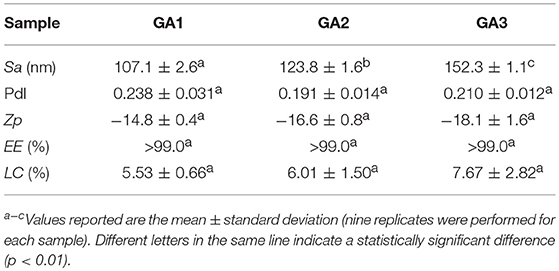
Table 4. Size average (Sa), polydispersity (PdI), zeta potential (Zp), efficiency of encapsulation (EE) and loading capacity (LC) of curcumin-loaded micelles produced with galactomannan acetate with increasing acetylation degrees (GA1, GA2, and GA3).
All micelles present EE values higher than 99%. Regarding LC, the values ranged between 5.53 and 7.67% (Table 4). The highly hydrophobic character of GA micelles and curcumin led to a significant hydrophobic interaction between the compounds thus increasing LC. Results showed that GA micelles are good systems for curcumin encapsulation when compared with other nanostructures such as alginate-chitosan-pluronic nanoparticles, that present EE values between 10 and 15% (Das et al., 2010). Micellar aggregates of cross-linked and random copolymers of N-isopropylacrylamide (NIPAAM), with N-vinyl-2-pyrrolidone (VP) and poly(ethyleneglycol)monoacrylate (PEG-A) showed EE values higher than 90% for curcumin (Bisht et al., 2007). Other amphiphilic polymers were used to encapsulate epirubicin and clonazepam and showed values for EE of 64.8 and 70.3% and LC of 6.09 and 23.2%, respectively (Jung et al., 2003; Jung et al., 2004.)
Figure 4 shows TEM photographs of unloaded and curcumin-loaded micelles produced using GA1. Micelles present a spherical shape with sizes ranging between 50 and 100 nm, which is in the range of the size distribution measured by DLS. However, micelles aggregation can be observed, which are very probably related with sample preparation procedures and with evaporation of the solvent that happens during TEM analyses, where a dried sample is needed.
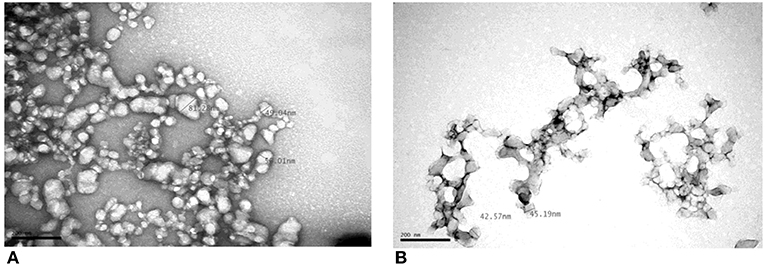
Figure 4. Transmission electron microscopy (TEM) photographs of unloaded (A) and curcumin-loaded (B) micelles from GA1.
Conclusion
The chemical modification of a galactomannan through the incorporation of acetyl groups was successfully preformed for the first time using a simple methodology, resulting in the development of an amphiphilic polymer with different acetylation degrees. This procedure can be used in the development of different chemical and physical functionalities in natural polysaccharides (e.g., hydrophobicity, solubility in organic solvents and good thermal behavior). The availability of galactomannan acetate with different acetylation degrees opens the possibility of utilization of these materials for the development of other new structures, e.g., for encapsulation of hydrophobic compounds. This was demonstrated in the present work, resulting in the formation of micelles at the nanoscale with very high values of encapsulation efficiency.
Data Availability
The datasets generated for this study are available on request to the corresponding author.
Author Contributions
The authors MC and AP did the experimental work and contributed to conception, design, writing, and revision of the manuscript. LP and AV contributed to conception, writing, and revision of the manuscript. All the authors read and approved the submitted version of the manuscript.
Conflict of Interest Statement
The authors declare that the research was conducted in the absence of any commercial or financial relationships that could be construed as a potential conflict of interest.
Acknowledgments
AP is recipient of a fellowship (SFRH/BPD/101181/2014), supported by Fundação para a Ciência e Tecnologia (FCT, Portugal). This work was supported by the Portuguese Foundation for Science and Technology (FCT) under the scope of the strategic funding of UID/BIO/04469/2013 unit and COMPETE 2020 (POCI-01-0145-FEDER-006684) and by BioTecNorte operation (NORTE-01-0145-FEDER-000004) funded by the European Regional Development Fund under the scope of Norte2020–Programa Operacional Regional do Norte. The authors would like to acknowledge to Rui Fernandes from IBMC, University of Porto for assistance in taking the TEM pictures.
References
Anand, P., Kunnumakkara, A. B., Newman, R. A., and Aggarwal, B. B. (2007). Bioavailability of curcumin: problems and promises. Mol. Pharm. 4, 807–818. doi: 10.1021/mp700113r
ASTM D871-96 (2019). Standard Test Methods of Testing Cellulose Acetate (West Conshohocken, PA: ASTM International), 1–12. Available online at: www.astm.org.
Bisht, S., Feldmann, G., Soni, S., Ravi, R., Karikar, C., Maitra, A., et al. (2007). Polymeric nanoparticle-encapsulated curcumin (“nanocurcumin”): a novel strategy for human cancer therapy. J. Nanobiotechnol. 5:3. doi: 10.1186/1477-3155-5-3
Cerqueira, M. A., Bourbon, A. I., Pinheiro, A. C., Martins, J. T., Souza, B. W. S., Teixeira, J. A., et al. (2011a). Galactomannans use in the development of edible films/coatings for food applications. Trends Food Sci. Technol. 22, 662–671. doi: 10.1016/j.tifs.2011.07.002
Cerqueira, M. A., Pinheiro, A. C., Souza, B. W. S., Lima, A. M., Ribeiro, C., Miranda, C., et al. (2009). Extraction, purification and characterization of galactomannans from non-traditional sources. Carbohydr. Polym. 75, 408–414. doi: 10.1016/j.carbpol.2008.07.036
Cerqueira, M. A., Souza, B. W. S., Simões, J., Teixeira, J. A., Domingues, M. R., Coimbra, M. A., et al. (2011b). Structural and thermal characterization of galactomannans from non-conventional sources. Carbohydr. Polym. 83, 179–185. doi: 10.1016/j.carbpol.2010.07.036
Das, R. K., Kasoju, N., and Bora, U. (2010). Encapsulation of curcumin in alginate-chitosan-pluronic composite nanoparticles for delivery to cancer cells. Nanomedicine 6, 153–160. doi: 10.1016/j.nano.2009.05.009
Duval-Terrié, C., Huguet, J., and Muller, G. (2003). Self-assembly and hydrophobic clusters of amphiphilic polysaccharides. Coll. Surfaces A Physicochem. Eng. Aspects 220, 105–115. doi: 10.1016/S0927-7757(03)00062-1
Fessi, H., Puisieux, F., Devissaguet, J., Ph., Ammoury, N., and Benita, S. (1989). Nanocapsule formation by interfacial polymer deposition following solvent displacement. Int. J. Pharm. 55, R1–R4. doi: 10.1016/0378-5173(89)90281-0
Garcia, R. B., Da Silva, D. L. P., and Costa, M. (2008). Avaliação de géis obtidos a partir da acetilação da quitosana em meio heterogêneo. Química Nova 31, 486–492. doi: 10.1590/S0100-40422008000300004
Gonçalves, C., and Gama, F. M. (2008). Characterization of the self-assembly process of hydrophobically modified dextrin. Eur. Polym. J. 44, 3529–3534. doi: 10.1016/j.eurpolymj.2008.08.034
Gonçalves, C., Martins, J. A., and Gama, F. M. (2007). Self-assembled nanoparticles of dextrin substituted with hexadecanethiol. Biomacromolecules 8, 392–398. doi: 10.1021/bm060993e
Guo, Y., Wang, X., Shu, X., Shen, Z., and Sun, R.-C. (2012). Self-assembly and paclitaxel loading capacity of cellulose-graftpoly(lactide) nanomicelles. J. Agric. Food Chem. 60, 3900–3908 doi: 10.1021/jf3001873
Hassani, L. N., Hendra, F., and Bouchemal, K. (2012). Auto-associative amphiphilic polysaccharides as drug delivery systems. Drug Discov. Today 17, 608–614. doi: 10.1016/j.drudis.2012.01.016
Hung, K.-C., Yang, C.-N., Yang, T.-C., Wu, T.-L., Chen, Y.-L., and Wu, J.-H. (2017). Characterization and thermal stability of acetylated slicewood production by alkali-catalyzed esterification. Materials 10:393 doi: 10.3390/ma10040393
Jacob, J., Haponiuk, J. T., Thomas, S., and Gopi, S. (2018). Biopolymer based nanomaterials in drug delivery systems: a review. Material. Today Chem. 9, 43–55. doi: 10.1016/j.mtchem.2018.05.002
Jiao, Y., Wilkinson, J., Di, X., Wang, W., and Hatcher, H., et al. (2009). Curcumin, a cancer chemopreventive and chemotherapeutic agent, is a biologically active iron chelator. Blood 113, 462–469. doi: 10.1182/blood-2008-05-155952
Jung, S.-W., Jeong, Y.-I., and Kim, S.-H. (2003). Characterization of hydrophobized pullulan with various hydrophobicities. Int. J. Pharm. 254, 109–121. doi: 10.1016/S0378-5173(03)00006-1
Jung, S.-W., Jeong, Y.-I., Kim, Y.-H., and Kim, S.-H. (2004). Self-assembled polymeric nanoparticles of poly(ethylene glycol) grafted pullulan acetate as a novel drug carrier. Arch. Pharm. Res. 5, 562–569. doi: 10.1007/BF02980132
Kilian, P. H., Kronski, E., Michalik, K. M., and Barbieri, O., et al. (2012). Curcumin inhibits prostate cancer metastasis in vivo by targeting the inflammatory cytokines CXCL1 and −2. Carcinogenesis 33, 2507–2519. doi: 10.1093/carcin/bgs312
Kuan, C.-Y., Yee-Fung, W., Yuen, K.-H., and Liong, M.-T. (2011). Nanotech: propensity in foods and bioactives. Crit. Rev. Food Sci. Nutr. 52, 55–71. doi: 10.1080/10408398.2010.494259
Laignel, B., Bliard, C., Massiot, G., and Nuzillard, J. M. (1997). Proton NMR spectroscopy assignment of o-glucose residues in highly acetylated starch. Carbohydr. Res. 298, 251–260. doi: 10.1016/S0008-6215(96)00314-X
Lee, A. S., Bütün, V., Vamvakaki, M., Armes, S. P., Pople, J. A., and Gast, A. P. (2002). Structure of pH-dependent block copolymer micelles: charge and ionic strength dependence. Macromolecules 35, 8540–8551. doi: 10.1021/ma0114842
Li, X. Y., Chen, S., Zhang, B., Li, M., Diao, K., Zhang, Z., et al. (2012). In situ injectable nano-composite hydrogel composed of curcumin, N,O-carboxymethyl chitosan and oxidized alginate for wound healing application. Int. J. Pharm. 437, 110–119. doi: 10.1016/j.ijpharm.2012.08.001
Liu, Y., Sun, J., Zhang, P., and He, Z. (2011). Amphiphilic polysaccharide-hydrophobicized graft polymeric micelles for drug delivery nanosystems. Curr. Med. Chem. 18, 2638–2648. doi: 10.2174/092986711795933696
Menon, V. P., and Sudheer, A. R. (2007). Antioxidant and anti-inflammatory properties of curcumin. Adv. Exp. Med. Biol. 595, 105–125. doi: 10.1007/978-0-387-46401-5_3
Minimol, P. F., Paul, W., and Sharma, C. P. (2013). PEGylated starch acetate nanoparticles and its potential use for oral insulin delivery. Carbohydr. Polym. 95, 1–8. doi: 10.1016/j.carbpol.2013.02.021
Motozato, Y., Ihara, H., Tomoda, T., and Hirayama, C. (1986). Preparation and gel permeation chromatographic properties of pululan spheres. J. Chromatogr. 355, 434–437. doi: 10.1016/S0021-9673(01)97349-2
Onwulata, C. I. (2012). Encapsulation of new active ingredients. Annu. Rev. Food Sci. Technol. 3, 183–202. doi: 10.1146/annurev-food-022811-101140
Persenaire, O., Alexandre, M., Degée, P., and Dubois, P. (2001). Mechanisms and kinetics of thermal degradation of poly(ε-caprolactone). Biomacromolecules 2, 288–294 doi: 10.1021/bm0056310
Ranganathan, R., Tran, L., and Bales, B. L. (2000). Surfactant- and salt-induced growth of normal sodium alkyl sulfate micelles well above their critical micelle concentrations. J. Phys. Chem. B 104, 2260–2264. doi: 10.1021/jp993917x
Rangelov, S., Petrov, P., Berlinova, I., and Tsvetanov, C. (2004). Association properties of a high molecular weight poly(propy1ene oxide-b-ethylene oxide) diblock copolymer in aqueous solution. Polym. Bull. 52, 155–161. doi: 10.1007/s00289-004-0266-0
Scaman, C., Nakai, S., and Aminlari, M. (2006). Effect of pH, temperature and sodium bisulfite or cysteine on the level of Maillard-based conjugation of lysozyme with dextran, galactomannan and mannan. Food Chem. 99, 368–380. doi: 10.1016/j.foodchem.2005.08.003
Shishir, M. R. I., Xie, L., Sun, C., Zheng, X., and Chen, W. (2018). Advances in micro and nano-encapsulation of bioactive compounds using biopolymer and lipid-based transporters. Trends Food Sci. Technol. 78, 34–60. doi: 10.1016/j.tifs.2018.05.018
Silva, H. D., Poejo, J., Pinheiro, A. C., Dons,ì, F., Serra, A. T., Duarte, C. M. M., et al. (2018). Evaluating the behaviour of curcumin nanoemulsions and multilayer nanoemulsions during dynamic in vitro digestion. J. Funct. Foods 48, 605–613 doi: 10.1016/j.jff.2018.08.002
Tikekar, R. V., Pan, Y., and Nitin, N. (2013). Fate of curcumin encapsulated in silica nanoparticle stabilized Pickering emulsion during storage and simulated digestion. Food Res. Int. 51, 370–377. doi: 10.1016/j.foodres.2012.12.027
Vendruscolo, C. W., Andreazza, I. F., Ganter, J. L. M. S., Ferrero, C., and Bresolin, T. M. B. (2005). Xanthan and galactomannan (from M. scabrella) matrix tablets for oral controlled delivery of theophylline. Int. J. Pharm. 296, 1–11. doi: 10.1016/j.ijpharm.2005.02.007
Yang, S., Xie, Q., Liu, X., Wang, S., and Song, X. (2018b). Acetylation improves thermal stability and transmittance in FOLED substrates based on nanocellulose films. RSC Adv. 8, 3619–3625. doi: 10.1039/C7RA11134G
Yang, X., Shi, X., D'arcy, R., Tirelli, N., and Zhai, G. (2018a). Amphiphilic polysaccharides as building blocks for self-assembled nanosystems: molecular design and application in cancer and inflammatory diseases. J. Control. Release 272, 114–144. doi: 10.1016/j.jconrel.2017.12.033
Yu, H., Shi, K., Liu, D., and Huang, Q. (2012). Development of a food-grade organogel with high bioaccessibility and loading of curcuminoids. Food Chem. 131, 48–54. doi: 10.1016/j.foodchem.2011.08.027
Zhang, H., Gao, F., Liu, L., Li, X., Zhou, Z., Yang, X., et al. (2009). Pullulan acetate nanoparticles prepared by solvent diffusion method for epirubicin chemotherapy. Colloids Surf. B 71 19–26. doi: 10.1016/j.colsurfb.2008.12.039
Keywords: Cyamopsis tetragonolobo, guar gum, self-assembly, nanomicelles, nanoencapsulation
Citation: Cerqueira MA, Pinheiro AC, Pastrana LM and Vicente AA (2019) Amphiphilic Modified Galactomannan as a Novel Potential Carrier for Hydrophobic Compounds. Front. Sustain. Food Syst. 3:17. doi: 10.3389/fsufs.2019.00017
Received: 27 October 2018; Accepted: 01 March 2019;
Published: 26 March 2019.
Edited by:
Cristobal N. Aguilar, Universidad Autónoma de Coahuila, MexicoReviewed by:
Guillermo Alfredo Picó, Institute of Biotechnological and Chemical Processes Rosario (IPROBYQ), ArgentinaAna Cristina de Oliveira Monteiro-Moreira, University of Fortaleza, Brazil
Copyright © 2019 Cerqueira, Pinheiro, Pastrana and Vicente. This is an open-access article distributed under the terms of the Creative Commons Attribution License (CC BY). The use, distribution or reproduction in other forums is permitted, provided the original author(s) and the copyright owner(s) are credited and that the original publication in this journal is cited, in accordance with accepted academic practice. No use, distribution or reproduction is permitted which does not comply with these terms.
*Correspondence: Miguel A. Cerqueira, miguel.cerqueira@inl.int