- 1Center of Exact and Technological Sciences, State University of West Paraná (UNIOESTE), Cascavel, Brazil
- 2Urban Planning and Technology Center, State University of Londrina (UEL), Londrina, Brazil
The process of cassava starch extraction is associated with the generation of a large volume of liquid wastes from root washing and starch extraction (5 to 7 L.kg−1root). In this context, the aim of this study was to evaluate the treatment of cassava starch wastewater in a horizontal anaerobic fixed bed reactor to the removal of organic matter and generation of biogas. For this purpose, two fixed bed reactors filled with different types of support materials were used: bamboo rings (R1) and flexible PVC rings (R2). The reactors were constructed in polyvinyl chloride (PVC) with 90 cm length and 15 cm diameter. To evaluate the reactors were carried out 13 assays (A1 to A13) with increases in the organic loading rate (OLR, 1.7 to 15.0 g.L−1.d−1) and a decrease in the hydraulic retention time (HRT, 4.0 to 0.8 days). Biogas production followed a similar pattern in both reactors. The highest biogas productions of 1.4 L.L−1.d−1 (R1) and 1.0 L.L−1.d−1 (R2) were verified in the assay A6 in which were applied highest influent concentration (15.1g.L−1), intermediates OLR (5.6 g.L−1.d−1) and HRT (2.7 d). The chemical oxygen demand (COD) removal increased with the OLR increase resulting in COD removal values of up to 99%. Total solids removal efficiencies of 86.2 and 85.5% were achieved in R1 and R2, respectively. In both reactors, the contact surface of bamboo (132 m2.m−3) and PVC (191 m2.m−3) supported biomass attachment even in the highest OLR (15 g.L−1.d−1) and low HRT (0.8 d). Both support material provided conditions to the reactors resist the variations in operating conditions and reestablish the equilibrium after possible hydraulic and organic shocks load, constituting a robust system for the treatment of cassava starch wastewater. In these conditions the COD and solids removal remained satisfactory. After a 450-days reactor running, no changes were observed in the composition and structure of the support material, indicating that bamboo and PVC are possible cheap and efficient alternatives for biomass immobilization.
Introduction
Cassava starch is considered a product of great importance due to its functional properties which make it applicable to the most varied sectors of the economy (food, pharmaceutical, chemical, textile, paper among others). In the transformation process of cassava into starch there is wastewater generation, resulting from operations of starch extraction and root washing (5–7 m3.t−1 of root) (Leaño and Babel, 2012), which presents high COD values (12,000 mg. L−1), due to presence of starch and simple sugars (glucose and fructose) (Gomes et al., 2016).
Anaerobic treatment of cassava starch wastewater has been studied for biogas production (methane or hydrogen + methane) (Wang et al., 2011; Rosa et al., 2014; Kuczman et al., 2017), however, great part of cassava starch industries uses the lagoon system, where the produced gases (CO2, CH4, and H2S) are emitted to the atmosphere. Aiming at the energy utilization of the waste some industries cover the anaerobic lagoon with a floating, impermeable cover which is named covered lagoon digester (CLD). Although, the CLD allows the biogas valuation, this alternative presents some disadvantages as low methane productivity and continual cover maintenance (Cantrell et al., 2008).
The high-rate reactors in place of the lagoon system have been evaluated for the cassava starch wastewater treatment, since they favor biogas valuation, with shorter hydraulic retention time (HRT) and lower area requirement. However, as these wastewaters are rapidly acidified, they demand care due to acidification risks on the anaerobic reactor (Palma et al., 2018).
In high-rate anaerobic systems it is necessary to retain biomass in the reactor, which is obtained by attachment on inert support or even by maintaining biomass in sludge beds. Studies on the types of inert support in anaerobic reactors are important, since they can significantly influence the reactor performance (Garcia et al., 2008), especially in high strength wastewaters with high levels of solids such as effluents from starch and alcohol-producing industries. The support material promotes greater biomass retention in the reactor, higher substrate degradation, increases resistance to toxic substances, and shock loads (Mockaitis et al., 2014; Aquino et al., 2017).
Anaerobic digestion of cassava starch wastewater in a horizontal reactor without support material showed satisfactory performance, however under low organic loading rates (1.12 to 2.70 g.L−1.d−1) and high HRT values (16.6 to 6.6 d) (Kuczman et al., 2011). When it is applied to reactors with smaller volumes and hydraulic retention time, the use of support material may be necessary, especially when it comes to easily acidified wastewater (Palma et al., 2018).
Important aspects to be considered in selecting support materials type are: (i) ability to biomass attachment; (ii) high contact surface; (iii) low weight to do not require reactor structure increases; (iv) easiness and low obtaining costs. Thus, several materials are used as inert support in anaerobic reactors, such as polyurethane foam (Fuess et al., 2017), low-density polyethylene (Anzola-Rojas et al., 2016), plastic rings (conduit) (Santos and Oliveira, 2011), bamboo (Feng et al., 2008; Oliveira and Bruno, 2013) in addition to others more.
The utilization of bamboo support in anaerobic reactors is feasible due to your high degradation resistance and possible use of up to 10 years (Feng et al., 2008). Bamboo is a perennial herb, composed mainly of fibers (Feng et al., 2008) and widely distributed in several regions of the world, as Asia (Peng and She, 2014) and America.
Andreani et al. (2015) and Torres et al. (2017) evaluated bamboo as a support material in upflow acidogenic anaerobic reactors, destined to biohydrogen production from cassava starch wastewater. The aforementioned authors obtained satisfactory results in the reactor with bamboo support when compared to reactors with low density polyethylene support. Kunzler et al. (2013) evaluated a horizontal reactor with bamboo rings as support material in cassava starch wastewater treatment and observed a satisfactory performance until the studied organic loading rate of 5.6 g.L−1.d−1. The system performed well, demonstrating that studies could be carried out at higher organic loads to explore the reactor potential. Kuczman et al. (2017) with a pilot horizontal reactor (33.6 m3) installed in cassava starch industry also used bamboo rings as support material, however the OLR tested were low (0.55 to 9.90 g.L−1d−1) and the HRT was high (7 days).
In this context, the objective of this study was to evaluate the cassava starch wastewater treatment in a fixed bed reactor to organic matter removal and biogas production. For this purpose, two fixed bed reactors filled with two different types of support materials were used: bamboo rings and flexible PVC rings (conduit). The bamboo because it is a natural material of easy acquisition and that presents good mechanical resistance (Farid, 2017) and the flexible PVC rings because is also of easy acquisition.
Materials and Methods
Reactors and Support Material
Two horizontal anaerobic fixed bed reactors (HAFBR) were constructed in polyvinyl chloride (PVC) with length of 90 cm and diameter of 15 cm (total volume:15.9 L) as previously described by Kunzler et al. (2013). Five sampling points were installed along the reactors, with distance of 15 cm between them. The support materials used in the reactors were: (i) R1–bamboo pieces with length of 10 cm and diameter of 2.5 cm (surface area: 132 m2.m−3–working volume: 6.0 L); (ii) R2—flexible PVC rings with length of 4 cm and diameter of 2 cm (surface area:191 m2.m−3–working volume: 8.0 L). The reactors were inoculated with 30% of anaerobic sludge (84 gST.L−1) from cassava processing industry, and 70% of diluted cassava starch wastewater (dilution factor = 10).
Cassava Starch Wastewater
The cassava starch wastewater (CSWW) was collected from a full-scale starch factory located in the western region of Paraná, Brazil. The CSWW is generated during the cassava roots washing and starch extraction. In the laboratory the wastewater was homogenized and kept in a freezer (−20°C) until the use. To reproduce the industrial treatment system condition, neither dilution of CSWW nor pH adjustment was applied in the reactor influent.
Operational Conditions
In the HAFBR were carried out 13 assays (A1 to A13) in which were evaluated the increase in organic loading rate (1.7 to 15.0 g COD.L−1.d−1) and the decrease in HRT (4.0 to 0.8 days) (Table 1). The reactors were kept in a controlled temperature chamber at 26°C.
The reactors were monitored determining the following parameters pH, COD, volatile acidity, total alkalinity, total solids, fixed and volatile solids, and biogas volume. The data collection was daily and maintained for 15 days after the reactor reached the steady state. The system was considered stable when the coefficient of variation (CV) in the monitoring parameters were ≤10%. The biogas volume was measured by a liquid displacement system filled with an acidified saline solution composed by sulfuric acid (3% v/v) and sodium chloride (25% m/v), indicated to maintain all the biogas constituents (Fernandes Junior, 1995). The methane volume was estimated with basis in reactors fed with cassava wastewater and operated in similar conditions (Kuczman et al., 2014; Araujo et al., 2018; Mari, 2018).
The reactor performance indicators were: volatile acidity/ total alkalinity ratio, COD removal efficiency (%), solids removal efficiency (%), volumetric biogas production rate (Lbiogas.L−1.d−1), and methane production rate estimated (LCH4.L−1.d−1). At the end of evaluation period, samples for COD and volatile organic acids determination were taken at the sampling points along the reactor length.
Analytical Methods
The parameters pH; volatile and fixed total solids, and COD were determined according to the Standards Methods for the Examination of Water and Wastewater (APHA, 2005). The volatile acidity and total alkalinity were determined according to Silva (1977). Volatile organic acids (acetic, butyric, and propionic) were quantified by high performance liquid chromatography (HPLC Shimadzu Prominence®) on Aminex HPX-87H® column (300 × 7.8 mm), according to the methodology proposed by Lazaro et al. (2012).
Statistical Analysis
The statistical analysis was based on the split plot design in which the OLR corresponded to the main plot and the support material (bamboo and PVC) to the subplot. Analysis of variance (ANOVA) and the Tukey's test was performed at a significance level of 5% (α = 0.05) by software R version 3.4.4 with Package ExpDes.
Results and Discussion
Horizontal anaerobic fixed bed reactors (HAFBR) were monitored for 450-days. In this period, 13 assays (A1 to A13) were carried out in which the increase in the organic loading rate was due to HRT reduction and variation of organic matter (COD) in cassava starch wastewater (influent concentration). The Table 2 presents the average values to performance indicators to assays (A1 to A13) and reactors (R1 and R2).
Stability, Organic Matter, and Total Solids Removals
According to the ANOVA the interaction between OLR and reactors (support material–bamboo and PVC) were significant for VA/TA ratio (p-value ≤ 5%). Significant differences in the Tukey's test was verified in VA/TA ratios between the reactors only in assays A12 and A13 (Table 2). The average values to VA/TA ratio were 0.25 ± 0.4 (R1) and 0.2 ± 0.3 (R2). From the A8 assay there was an increase in VA/TA ratio, however, the values remained within the range of 0.1 to 0.5 in which the system is considered stable (Sánchez et al., 2005). However, in assay A12, the VA/TA ratio increase for values of 1.5±2.0 (R1) and 1.0±1.5 (R2) which may indicate reactors instability that normally occurs in response to organic or hydraulic overloads (Singh and Prerna, 2009).
The HRT reduction from 1.3 to 0.8 d leads to a disturbance in reactors, because the higher organic acids concentration generated in the system from organic matter degradation may to reduce the alkalinity. Sánchez et al. (2005), verified that the VA/TA ratio increase is directly related to HRT decrease. Although the HRT remained at 0.8 d, in assay A13 the system reestablished the dynamic equilibrium, recovering the stability after perturbation. The increase in the cell retention time in immobilized systems improves reactor resistance and promote the rapid recovery after hydraulic shock loads, the presence of inhibitory compounds and changes in substrate composition (Sánchez et al., 1994).
The interaction effect between OLR and reactors was significant (p-value ≤ 5%) for the variables: pH and COD removal efficiency. With respect to effluent pH values the Tukey test revealed that there was statistical difference between reactors only for the assays A7 and A12. The average pH values in effluent at R1 and R2 were 7.3 ± 0.4 and 7.4 ± 0.4, respectively. For the total solids removal efficiency, it was not verified significant difference in the interaction between OLR and reactors (Table 2). The average efficiency was 86.2 ± 4.6 (R1) and 85.5 ± 6.1 (R2).
For the COD removal response variable, significant differences were verified between the reactors in the assays A1 and A13. In both reactors were obtained satisfactory removal efficiency to COD (≥90%) and total solids (≥80%), with variations lower than 7% (Figures 1, 2). The influent wastewater pH was not corrected, presenting values between 3.6 and 5.7 (Table 1). In the reactors effluent, pH values remained around 7.4 with variations lower than 6%. These results demonstrate that despite the OLR increase and HRT decrease the reactors operation was stable in most of the assays as can be seen in the Figures 1 and 2.
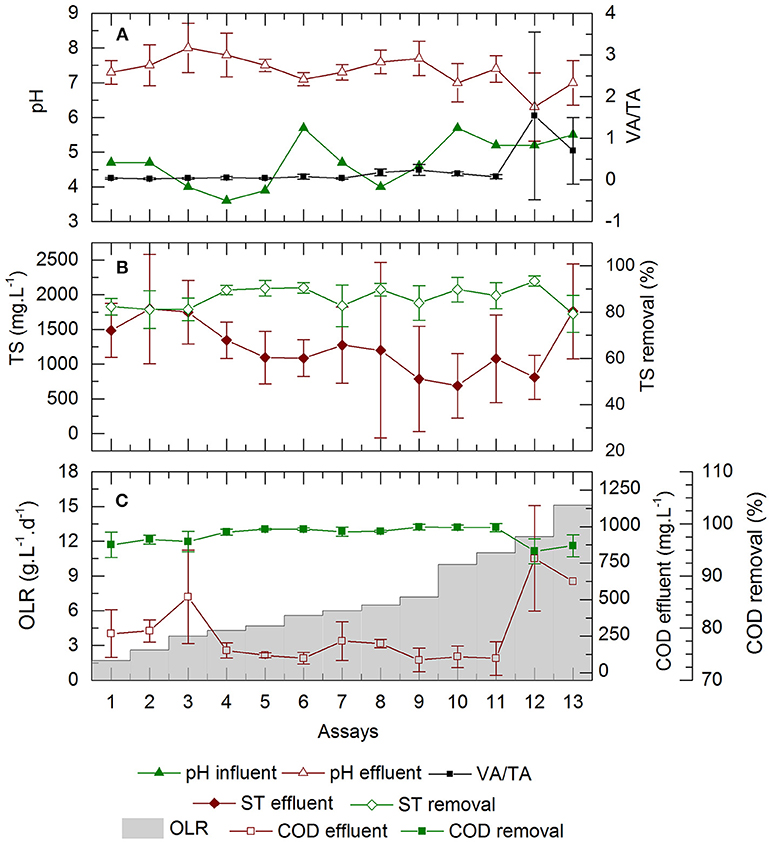
Figure 1. R1 performance: (A) pH in the effluent and VA/TA ratio; (B) total solids in the effluent and total solids removal efficiency; (C) COD in effluent and COD removal efficiency.
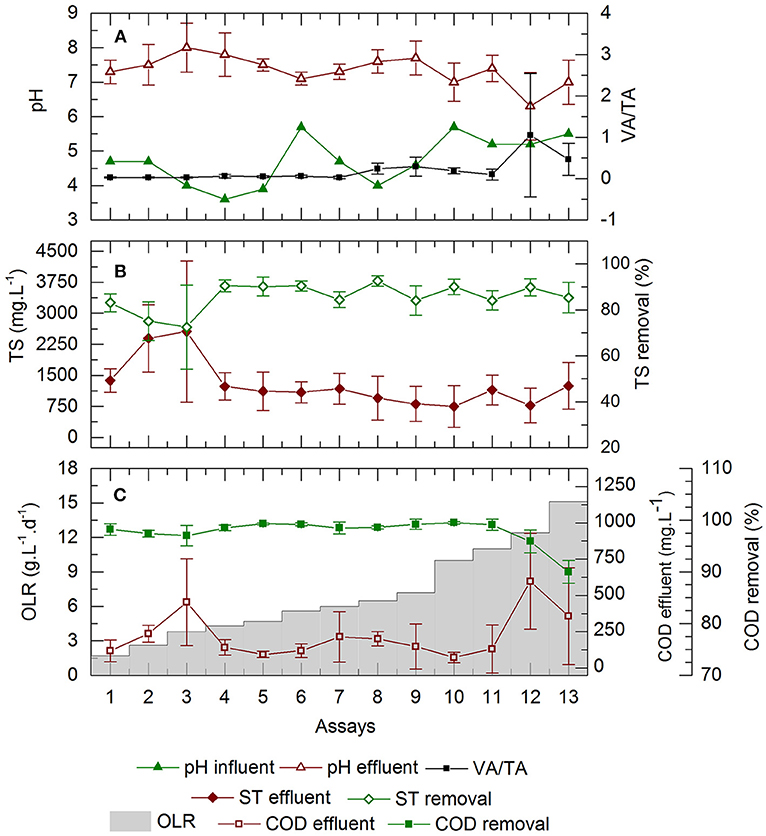
Figure 2. R2 performance: (A) pH in the effluent and VA/TA ratio; (B) total solids in the effluent and total solids removal efficiency; (C) COD in effluent and COD removal efficiency.
Aquino et al. (2017) evaluated two inert support for biomass immobilization to vinasse anaerobic treatment and observed that the arrangement influenced reactor efficiency, since it affected biomass growth. Fuess et al. (2017) compared two methanogenic reactors in vinasse treatment: (i) upflow anaerobic structured-bed reactor (ASTBR); ii) a conventional UASB reactor. The authors have verified better performance in the ASTBR, supporting high organic loading rate (30 kg m−3d−1). At the similar conditions the UASB showed performance losses for every increase in the OLR, due to inert solids accumulation in the sludge.
Degradation of Organic Matter and Intermediate Metabolites Along the Reactors Length
In order to verify the behavior of organic matter removal and intermediate metabolites in a spatial reactors profile, samples were collected at points along the R1 and R2 length (points 1 to 5) and in the reactor exit (point 6). To cover the whole experimental period the samplings were performed in the assays A1 (1.7 g.L−1.d−1); A4 (4.3 g.L−1.d−1); A5 (4.7 g.L−1.d−1); A6 (5.6 g.L−1.d−1), and A11 (11 g.L−1.d−1). The organic matter degradation along the reactors length is presented in Supplementary Material.
In all the assays (A1, A4, A5, A6, and A11), the organic matter removal occurred predominantly in the first two collection points. At R1 about 94% of the organic matter was removed at the first point, while at R2 the average removal efficiency at the same point was 79%. These high organic matter removal efficiencies indicate that the active biomass concentrated near the reactors feed zone. The presence of easily degradable substrate fractions may have favored the growth and fixation of microorganisms in this reactor area, as also observed by Schmidt et al. (2014).
The distribution of volatile organic acids along the reactors is consistent with organic matter removal efficiency. In the last sampling point of both reactors, the acetic and propionic acid concentrations decreased to values lower than 10 mg.L−1, no accumulation or higher organic acids concentrations were verified along them, indicating that organic acids were consumed in the process, which resulted in reactors stability in this assays.
Biogas Production
The variance analysis showed that the OLR and the interaction between OLR and reactors (support material) had significant effects on the biogas production (p-value ≤ 5%). According to Tukey's test statistical differences were verified between reactors R1 and R2 in the assays A4, A5, A6, A7, A8, and A9 (Table 2). To ensure the representative estimative of the methane content in the biogas, we researched works with methane content at biogas in the OLR applied range. Therefore, we adopted three average values: 70% for the assays A1 to A5 (OLR from 1.7 to 4.7 g.L−1.d−1) (Kuczman et al., 2013; Araujo et al., 2018; Mari, 2018); 73% for the assays A6 to A9 (OLR from 5.6 to 7.2 g.L−1.d−1) (Araujo et al., 2018; Mari, 2018) and 69% for the assays A10 to A13 (OLR from 10 to 15.1 g.L−1.d−1) (Araujo et al., 2018; Mari, 2018). Methane content in biogas was estimated at 70 ± 2%, with average methane production rate of 0.5 ± 0.2 and 0.4 ± 0.1 LCH4.L−1.d−1 for R1 and R2, respectively. Figure 3 shows the biogas production and methane estimated production in the thirteen assays to reactors R1 and R2.
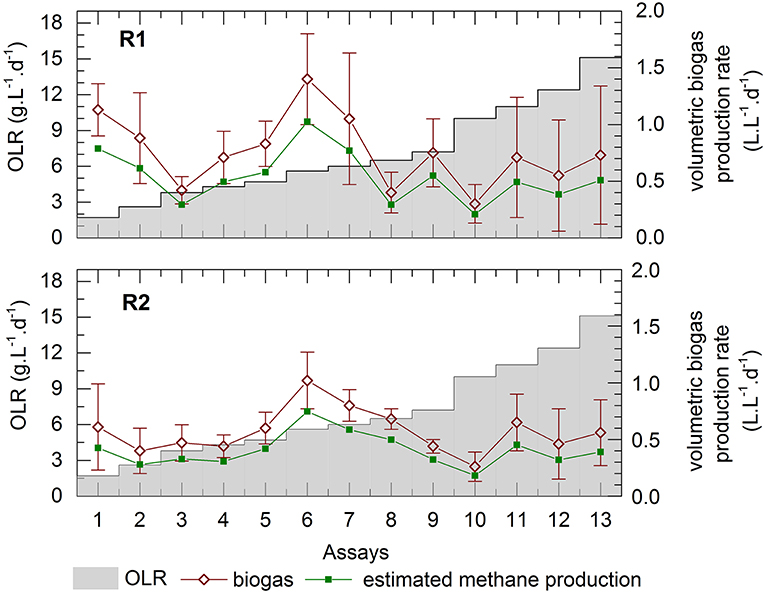
Figure 3. Volumetric biogas production rate and estimated methane production in the reactors R1 and R2.
Despite the narrow pH variability (≤6%), COD removal efficiency (≤2.6%), and total solids removal efficiency (≤6.1%), biogas production varied by around 41% in both reactors (Figure 3). In assay A6 we observed biogas production peaks of 1.4 ± 0.44 L.L−1.d−1 (R1) and 1.0 ± 0.25 L.L−1.d−1 (R2). In the following assays there was decrease in biogas volume in both reactors. In assay A10 the OLR increase (from 7.2 to 10 g.L−1.d−1) and HRT decrease (from 1.7 to 1.0 d) resulting in lowest biogas volumes (R1: 0.3 ± 0.17 L.L−1.d−1; R2: 0.25 ± 0.13 L.L−1.d−1).
Intanoo et al. (2016) observed in an upflow anaerobic sludge blanket reactor (UASB) fed with cassava wastewater from acidogenic reactor that the COD removal efficiency and biogas production were favored by OLR increased from 2 to 8 g.L−1d−1, however, the OLR increase to 10 g.L−1.d−1 leading to biogas reduction from 25 to 8 L.d−1, whereas the COD removal efficiency decreased discretely (66 to 62%), as observed in R1 and R2. According to authors the decrease in biogas volume is due to organic acids accumulation. Despite biogas reduction in R1 and R2 the profile of organic acids along the reactor no indicate your accumulation. From assay A11 there was a recovery of biogas production, which can indicate high resilience of the reactor, possibly due to biomass immobilization.
Araujo et al. (2018) evaluated the methane production in an upflow anaerobic fixed bed reactor from cassava starch wastewater and observed that the biogas production increase (0.8 to 7.56 L.d−1) was proportional to OLR increase (2.5 to 10 g.L−1.d−1), however, the methane proportion in biogas decreased from 76.8 to 66%, respectively. The increase in organic and hydraulic loads can cause disturbances in microbial community structure of the reactor. The reduction in biogas volume and in methane contents are usually related to decrease in quantity and activity of the syntrophic bacteria and methanogenic archaea (Regueiro et al., 2015).
In addition, the gas release from the liquid medium to the headspace of the reactor can also affect the biogas production. The gases solubility in liquid medium depends on the partial pressure of each gas in the atmosphere and the temperature (Metcalf and Eddy, 2016). As the solubility is inversely proportional to the temperature, at 26°C which is the temperature used in the present study, biogas dissolves more in the liquid medium when compared to optimal temperature at 35°C (Ward et al., 2008), hindered the gas release into the reactors' atmosphere. In addition to temperature, biomass growth may also contribute to biogas entrapment in the liquid phase. Palma et al. (2018) in similar operating conditions to those adopted in this study observed that the OLR increase from 3.0 to 8.5 g.L−1.d−1 and HRT decrease from 3.1 to 1.1 d lead to decreasing in the methane production from 0.29 to 0.18 LCH4.g−1COD. According to these authors the gas volume reduction may be related to its entrapment in the reactor bed due to biofilm thickening and solids retention.
Fuess et al. (2017) suggest that the biogas release to the liquid medium can be favored by adoption of the structured fixed bed, since this configuration provide increase in the empty spaces and improvement in the reactor hydrodynamic characteristics. The horizontal reactors used in this study, as well as those operated by Kunzler et al. (2013) and Palma et al. (2018) presented biogas production performance lower than that obtained by Araujo et al. (2018) in an upflow fixed bed reactor. This reactor configuration provides more efficient mixing, improving mass transfer rates between substrate and microorganisms, promoting the release of trapped gas bubbles in the liquid and preventing the particulate matter sedimentation (Ward et al., 2008).
The biomass concentration in the effluent of R1 (430 ± 214 mgVTS.L−1) and R2 (447 ± 190 mgVTS.L−1) was very close, even though there were differences between the surface areas of bamboo (132 m2.m−3) and PVC (191 m2.m−3). The reactors behavior exhibited similar patterns, indicating that the biomass attachment was effective in both supports. Good performances have been attributed to bamboo due to its porosity (R1−41%), since pore size (0.001–1,000 μm) facilitates the biomass development and the maintenance of anaerobic conditions in the support (Chen et al., 2012), in addition to improving mass transfer rates (Torres et al., 2017). Therefore, in treatment systems where wastewater composition can vary considerably, as is the case of cassava starch wastewater where we observed variations of up to 23% in organic matter concentration, immobilization of biomass in inert supports is a strategy capable of conferring robustness to the reactors.
Conclusions
Cell immobilization enabled the application of higher organic loading rate (15.1 g.L−1.d−1) and lower hydraulic retention time (0.8 days) in the reactors with bamboo (R1) and PVC (R2) support materials. In both reactors were achieved satisfactory removals of organic matter (≥90%) and total solids (≥85%). The highest biogas production of 1.4 L.L−1.d−1 (R1) and 1.0 L.L−1.d−1 (R2) were verified in assay A6 in which were applied the highest influent concentration (15.1 gCOD.L−1), intermediates organic loading rate (5.6 g.L−1.d−1) and hydraulic retention time (2.7 d). The filling of the reactor with bamboo and PVC support provided greater resistance to the possible hydraulic and organic shocks load and the ready establishment of balance after disturbances, constituting a robust system for the treatment of cassava starch wastewater. After a 450-days reactor run, no changes were observed in the support material composition and structure, indicating that bamboo and PVC are possible alternatives for biomass immobilization in cassava wastewater treatment systems.
Author Contributions
The manuscript has been written and composed by EW, CA, DL, and SG, with the assistance of OK and MT. Advanced data processing was performed by DT based on experiments made by EW and CA. All authors contributed to manuscript reviewed, read, and approved the submitted version. SG and MT supervised the project.
Conflict of Interest Statement
The authors declare that the research was conducted in the absence of any commercial or financial relationships that could be construed as a potential conflict of interest.
Acknowledgments
We authors acknowledge the financial support of National Council for Scientific and Technological Development (CNPq).
Supplementary Material
The Supplementary Material for this article can be found online at: https://www.frontiersin.org/articles/10.3389/fsufs.2019.00006/full#supplementary-material
References
Andreani, C. L., Torres, D. G. B., Schultz, L., Carvalho, K. Q., and Gomes, S. D. (2015). Hydrogen production from cassava processing wastewater in an anaerobic fixed bed reactor with bamboo as a support material. Eng Agr. 35, 578–587. doi: 10.1590/1809-4430-Eng.Agric.v35n3p578-587/2015
Anzola-Rojas, M. P., Zaiat, M., and Wever, H. (2016). Improvement of hydrogen production via ethanol-type fermentation in an anaerobic down-flow structures bed reactor. Bioresour. Technol. 202, 42–49. doi: 10.1016/j.biortech.2015.11.084
APHA (2005). Standard Methods for the Examination of Water and Wastewater. Washington, DC: American Public Health Association.
Aquino, S., Fuess, L. T., and Pires, E. C. (2017). Media arrangement impacts cell growth in anaerobic fixed-bed reactors treating sugarcane vinasse: structured vs. randomic biomass immobilization. Bioresour Technol. 235, 219–228. doi: 10.1016/j.biortech.2017.03.120
Araujo, I. R. C., Gomes, S. D., Tonello, T. U., Lucas, S. D. M., Mari, A. G., and Vargas, R. J. (2018). Methane production from cassava starch wastewater in packed-bed reactor and continuous flow. Engenharia Agrícola 38, 270–276. doi: 10.1590/1809-4430-eng.agric.v38n2p270-276/2018
Cantrell, K. B., Ducey, T., Ro, K. S., and Hunt, P. G. (2008). Livestock waste-to-bioenergy generation opportunities. Bioresour. Technol. 99, 7941–7953. doi: 10.1016/j.biortech.2008.02.061
Chen, C. J., Huang, X. X., Lei, C. X., Zhu, W. J., Chen, Y. X., and Wu, W. X. (2012). Improving anammox start-up with bamboo charcoal. Chemosphere 89, 1224–1229. doi: 10.1016/j.chemosphere.2012.07.045
Farid, M. (2017). A review on the use of bamboo for earthwork construction. MATEC Web Confer. 138, 04010. doi: 10.1051/matecconf/201713804010
Feng, H., Hu, L., Mahmood, Q., Qiu, C., Fang, C., and Shen, D. (2008). Anaerobic domestic wastewater treatment with bamboo carrier an aerobic baffled reactor. Int J Biodeterior. Biodegr. 62, 232–238. doi: 10.1016/j.ibiod.2008.01.009
Fernandes Junior, A. (1995). Digestão Anaeróbia de Manipueira Com Separação de Fases: Cinética da Fase Acidogênica.1995. Tese (Doutorado em Agronomia – Energia na Agricultura). Universidade Estadual Paulista. Botucatu.
Fuess, L. T., Kiyuna, L. S. M., Ferraz, A. D. N. J.r., Persinoti, G. F., Squina, F. M., Garcia, M. L., and Zaiat, M. (2017). Thermophilic two-phase anaerobic digestion using an innovative fixed-bed reactor for enhanced organic matter removal and bioenergy recovery from sugarcane vinasse. Appl. Energ. 189, 480–491. doi: 10.1016/j.apenergy.2016.12.071
Garcia, M. L., Lapa, K. R., Foresti, E., and Zaiat, M. (2008). Effects of bed materials on the performance of an anaerobic sequencing batch biofilm reactor treating domestic sewage. J. Environ. Manage. 88, 1471–1477. doi: 10.1016/j.jenvman.2007.07.015
Gomes, S. D., Gomes, S. D., Fuess, L.T., Mañunga, T., Gomes, P. C. F. L., and Zaiat, M. (2016). Bacteriocins of lactic acid bacteria as a hindering factor for biohydrogen production from cassava flour Wastewater in a continuous multiple tube reactor. Int. J. Hydrogen Energy 41, 8120–8131. doi: 10.1016/j.ijhydene.2015.11.186
Intanoo, P., Chaimongkol, P., and Chavadej, S. (2016). Hydrogen and methane production from cassava wastewater using two-stage upflow anaerobic sludge blanket reactors (UASB) with an emphasison maximum hydrogen production. Int J Hydrogen Energ. 41, 6107–6114. doi: 10.1016/j.ijhydene.2015.10.125
Kuczman, O., Tavares, M. H. F., Gomes, S. D., Guedes, L. P. C., and Grisotti, G. (2014). Cassava starch extraction effluent treatment in a one phase tubular horizontal pilot reactor with support medium. Eng. Agríc. 34, 1270–1282. doi: 10.1590/S0100-69162014000600021
Kuczman, O., Tavares, M. H. F., Gomes, S. D., Guedes, L. P. C., and Grisotti, G. (2017). Effects of stirring on cassava effluent treatment in an anaerobic horizontal tubular pilot reactor with support medium - A review. Renew. Sustain. Energ. Rev. 77, 984–989. doi: 10.1016/j.rser.2016.11.238
Kuczman, O., Tavares, M. H. F., Gomes, S. D., Torres, D. G. B., and Fleck, L. (2013). Influence of hydraulic retention time on the anaerobic treatment of cassava starch extraction effluent using a one-phase horizontal reactor. J. Food. Agric. Environ. 11, 1118–1120.
Kuczman, O., Torres, D. G. B., Damasceno, S. D., Tavares, M. H. F., and Alcantara, M. S. (2011). Specific biogas production from manipueira at one phase reactor. Eng. Agr. 31, 143–149. doi: 10.1590/S0100-69162011000100014
Kunzler, K. R., Gomes, S. D., Piana, P. A., Torres, D. G. B., Vilas Boas, M. A., and Tavares, M. H. F. T. (2013). Anaerobic reactors with biofilter and different diameter-length ratios in cassava starch industry wastewater treatment. Eng. Agr. 33, 612–624. doi: 10.1590/S0100-69162013000400003
Lazaro, C. Z., Vich, D. V., Hirasawa, J. S., and Varesche, M. B. A. (2012). Hydrogen production and consumption of organic acids by a phototropic microbial consortium. Int J Hydrogen Energ. 37, 11691–11700. doi: 10.1016/j.ijhydene.2012.05.088
Leaño, E. P., and Babel, S. (2012). Effects of pretreatment methods on cassava wastewater for biohydrogen production optimization. Renew. Energ. 39, 339–346. doi: 10.1016/j.renene.2011.08.030
Mari, A. G. (2018). Methane Production in AnSBBR Reactor From Previously Acidified Cassava Starch Wastewater: Performance and Economic Viability. Doctoral thesis, State University of West Paraná (Unioeste).
Metcalf, L., and Eddy, H. P. (2016). “Tratamento de Efluentes e Recuperação de Recursos,” in McGraw-Hill.-5a edição, revisada por G. Tchobanaglous, eds H. D. Stensel, R. Tsuchihashi, F. Burton, M. Abu-Orf, G. Bowden, W. Pfrang, T. I. Hespanhol, and J. C. Mierzwa (Porto Alegre: AMGH), 1980.
Mockaitis, G., Pantoja, J. L. R., Rodrigues, J. A. D., Foresti, E., and Zaiat, M. (2014). Continuous anaerobic bioreactor with a fixed-structure bed (ABFSB) for wastewater treatment with low solids and low applied organic loading content. Bioprocess Biosyst Eng. 37, 1361–1368. doi: 10.1007/s00449-013-1108-y
Oliveira, R. A., and Bruno, N. M. N. (2013). Start-up of horizontal anaerobic reactors with sludge blanket and fixed bed for wastewater treatment from coffee processing by wet method. Engenharia Agrícola 33, 353–366. doi: 10.1590/S0100-69162013000200014
Palma, D., Fuess, L. T., Lima-Model, A. N., Conceição, K. Z., Cereda, M. P., Tavares, M. H. F., et al. (2018). Using dolomitic limestone to replace conventional alkalinization in the biodigestion of rapid acidification cassava processing wastewater. J. Clean. Product. 172, 2942–2953. doi: 10.1016/j.jclepro.2017.11.118
Peng, P., and She, D. (2014). Isolation, structural characterization, and potential applications of hemicelluloses from bamboo: a review. Carbohydr. Polym. 112, 701–720 doi: 10.1016/j.carbpol.2014.06.068
Regueiro, L., Lema, J. M., and Carballa, M. (2015). Key microbial communities steering the functioning of anaerobic digesters during hydraulic and organic overloading shocks. Bioresour. Technol. 197, 208–216. doi: 10.1016/j.biortech.2015.08.076
Rosa, P. R. F., Santos, S. C., Sakamoto, I. K., Varesche, M. B. A., and Silva, E. L. (2014). The effects of seed sludge and hydraulic retention time on the production of hydrogen from a cassava processing wastewater and glucose mixture in an anaerobic fluidized bed reactor. Int. J. Hydro. Energ. 39, 13118–13127. doi: 10.1016/j.ijhydene.2014.06.152
Sánchez, E., Borja, R., Travieso, L., Martín, A., and Colmenarejo, M. F. (2005). Effect of organic loading rate on the stability, operational parameters and performance of a secondary upflow anaerobic sludge bed reactor treating piggery waste. Bioresour. Technol. 96, 335–44. doi: 10.1016/j.biortech.2004.04.003
Sánchez, E., Weiland, P., and Travieso, L. (1994). Effect of the organic volumetric loading rate on soluble COD removal in down flow anaerobic fixed bed reactors. Bioresour. Technol. 47, 173–176. doi: 10.1016/0960-8524(94)90117-1
Santos, A. C., and Oliveira, R. A. (2011). Swine waste water treatment in horizontal anaerobic reactor followed by aerobic sequencing batch reactor. Engenhar. Agrícola 31, 781–794. doi: 10.1590/S0100-69162011000400016
Schmidt, T., Ziganshin, A. M., and Nikolausz, M. (2014). Effects of the reduction of the hydraulic retention time to 1.5 days at constant organic loading in CSTR, ASBR, and fixed-bed reactors – performance and methanogenic community composition. Biomass Bioenerg. 69, 241–248. doi: 10.1016/j.biombioe.2014.07.021
Silva, M. O. S. A. (1977). Análises Físico-Químicas Para o Controle das Estações de Tratamento de Esgoto. São Paulo: Cestesb.
Singh, S. P., and Prerna, P. (2009). Review of recent advances in anaerobic packed-bed biogas reactors. Renewable Sustain. Energy Rev. 13, 1569–1575. doi: 10.1016/j.rser.2008.08.006
Torres, D. G. B., Lucas, S. D. M., Andreani, C. L., Carvalho, K. Q., Coelho, S. R. M., and Gomes, S. D. (2017). Hydrogen production and performance of anaerobic fixed-bed reactors using three support arrangements from cassava starch wastewater. Eng Agric. 37, 160–172. doi: 10.1590/1809-4430-eng.agric.v37n1p160-172/2017
Wang, W., Xie, L., Chen, J., Luo, G., and Zhou, Q. (2011). Biohydrogen and methane production by co-digestion of cassava stillage and excess sludge under thermophilic condition. Bioresour. Technol. 102, 3833–3839. doi: 10.1016/j.biortech.2010.12.012
Keywords: anaerobic reactors, bamboo, flexible PVC, agroindustrial wastewater, waste valorization
Citation: Watthier E, Andreani CL, Torres DGB, Kuczman O, Tavares MHF, Lopes DD and Gomes SD (2019) Cassava Wastewater Treatment in Fixed-Bed Reactors: Organic Matter Removal and Biogas Production. Front. Sustain. Food Syst. 3:6. doi: 10.3389/fsufs.2019.00006
Received: 08 October 2018; Accepted: 25 January 2019;
Published: 13 March 2019.
Edited by:
José Luis Garcia-Morales, University of Cádiz, SpainReviewed by:
Valeria Reginatto, University of São Paulo, BrazilBlaz Stres, University of Ljubljana, Slovenia
Copyright © 2019 Watthier, Andreani, Torres, Kuczman, Tavares, Lopes and Gomes. This is an open-access article distributed under the terms of the Creative Commons Attribution License (CC BY). The use, distribution or reproduction in other forums is permitted, provided the original author(s) and the copyright owner(s) are credited and that the original publication in this journal is cited, in accordance with accepted academic practice. No use, distribution or reproduction is permitted which does not comply with these terms.
*Correspondence: Cristiane L. Andreani, Y3Jpc3RpYW5lLmFuZHJlYW5pQGdtYWlsLmNvbQ==