BRIEF RESEARCH REPORT
Published on 14 Oct 2024
Manipulation of DHPS activity affects dendritic morphology and expression of synaptic proteins in primary rat cortical neurons
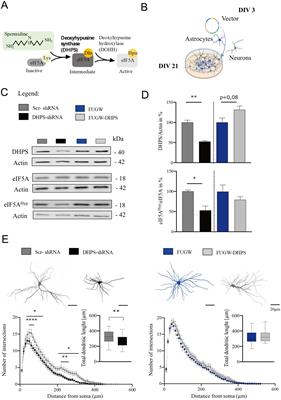
doi 10.3389/fncel.2024.1465011
- 683 views
2,460
Total downloads
15k
Total views and downloads
Select the journal/section where you want your idea to be submitted:
BRIEF RESEARCH REPORT
Published on 14 Oct 2024
ORIGINAL RESEARCH
Published on 17 Sep 2024
ORIGINAL RESEARCH
Published on 14 Dec 2023
ORIGINAL RESEARCH
Published on 18 Oct 2023
ORIGINAL RESEARCH
Published on 10 Oct 2023
REVIEW
Published on 02 Jun 2023
ORIGINAL RESEARCH
Published on 24 Feb 2023
Frontiers in Neural Circuits