- 1Department of Wildlife Management, College of Forestry Wildlife and Tourism, Sokoine University of Agriculture, Morogoro, Tanzania
- 2Department of Geography and Environmental Studies, Solomon Mahlangu College of Science and Education, Sokoine University of Agriculture, Morogoro, Tanzania
- 3UNESCO Chair on Ecohydrology and Transboundary Water Management, Sokoine University of Agriculture, Morogoro, Tanzania
The Lukosi River catchment plays a crucial role in the economic and ecological framework of the country, serving as a major contributor to the Great Ruaha River (GRR). This study aimed to assess the impact of human activities on the health of the Lukosi River by analyzing key water parameters. Three sampling sites across the catchment were selected, and water samples were collected and analyzed using the American Public Health Association technique. A one-sample t-test was used to measure the significance of the difference between the measured water quality parameters and the water quality criteria established by the Tanzania Bureau of Standards (TBS) and the World Health Organization (WHO). The results revealed mean values for various parameters: pH (8.67 ± 0.57), temperature (24.803 ± 1.361°C), EC (93.30 ± 3.34 mgL−1), BOD (14.85 ± 1.49 mgL−1), TDS (46.95 ± 1.55 mgL−1), total hardness (27.53 ± 1.28 mgL−1), DO (3.4 ± 0.53 mgL−1), turbidity (109.83 ± 40.99 mgL−1), NO2 (79.04 ± 0.73 mgL−1), Cu (6.07 ± 0.60 mgL−1), Ca (7.88 ± 0.36 mgL−1), Mn (0.16 ± 0.07 mgL−1), Cl (7.58 ± 3.97 mgL−1), F (0.12 ± 0.0008 mgL−1), SO4 (20.42 ± 4.12 mgL−1), Mg (1.92 ± 0.13 mgL−1), Zn (0.14 ± 0.11 mgL−1), Fe (3.56 ± 0.15 mgL−1), and NH3 (4.67 ± 0.51 mgL−1). Parameters such as DO, turbidity, NH3, NO3, and BOD significantly (p = 0.05) exceeded the permissible limits set by the TBS and the WHO. Furthermore, elements including Fe and Cu exceeded allowable limits. Moreover, the WQI was used to indicate that the water in the catchment is unsuitable for sustaining aquatic organisms and is unfit for domestic use. Household questionnaires and direct observations identified key human activities in the catchment: gardening (78.3%), agriculture (68%), livestock keeping (50%), sand mining (21%), and brick-making (21%). Less prevalent were car washing (7%) and fishing (4%). These findings underscore the urgent need for conservation efforts and sustainable management practices to safeguard the Lukosi River catchment (LRC).
1 Introduction
Globally water catchments are the sources of freshwater resources that sustain life and provide various social and economic needs (Koleka et al., 2023). However, the increasing demand for water poses significant challenges for water management (Greve et al., 2018). The destruction and misuse of natural resources as well as pollution of the aquatic environment caused by human activities have been on the rise in our planet (Uherek and Gouveia, 2014). Most of the environmental impacts associated with water catchment degradation are primarily caused by different human activities that are taking place along the catchment areas (Khatri and Tyagi, 2015). These activities affect the distribution, quantity, and chemical quality of water resources and their flow regimes (Liyanage and Yamada, 2017). Water catchment degradation affects not only stream flow regimes but also the ecology and the livelihoods of those who depend on it (Gereta et al., 2002). As a result, many countries have adopted rules to conserve the environment from anthropogenic risks and to ensure that water resources are used appropriately (Oliveira and Callisto, 2010).
Over the recent years in many African countries, considerable population growth has taken place, leading to rapid urbanization, industrialization, and expanded agricultural practices (Kanu and Achi, 2011). Consequently, this has led to a substantial increase in the discharge of various pollutants into water bodies, causing adverse impacts on aquatic ecosystems (Kanu and Achi, 2011). It is now widely recognized at national, regional, and global levels that the management and preservation of natural resources must be enhanced and substantial efforts are needed to reduce pollution resulting from human activities on a large scale (Kanu and Achi, 2011).
Water quality is assessed by examining its chemical, physical, and biological components (Lawson, 2011). The suitability of water for its intended purposes and the improvement of existing conditions hinge on the quality of these parameters (Ali et al., 2004). Key physical and chemical factors such as temperature, pH, salinity, dissolved oxygen (DO), total suspended, dissolved particles, total alkalinity, and heavy metal contaminants play a significant role in shaping the aquatic ecosystem (Lawson, 2011). These criteria, as elucidated by Lawson (2011), exert a crucial influence on the survival of aquatic flora and fauna. The composition, distribution, abundance, migrations, and variety of aquatic species are significantly influenced by the interactions between the physical and chemical properties of water (Deepak and Singh, 2014). In addition, poor water quality causes ecosystems to deteriorate, aquatic biodiversity to decline, and fisheries to become less productive (Ngor et al., 2018), which reduces local livelihood options and slows down national economic growth (Lynch et al., 2020).
Tanzania has experienced significant population growth, with its population quadrupling over the past 50 years. According to the 2022 national population and housing census, the country has 61,741,120 people [Tanzania National Bureau of Statistics (NBS), 2023]. The country relies on its surface and groundwater resources to supply clean water and livelihoods to its people. Additionally, Tanzanian surface water resources play crucial roles in tourism, agriculture, electricity generation, and industry. Moreover, they serve as vital habitats for aquatic flora and fauna. However, like most developing countries, economic activities in industry, energy production, mining, agriculture, and livestock keeping depend largely on these resources, exerting significant pressure on them, including potential contamination by various microcontaminants (Hellar-Kihampa, 2011). Only a few studies have been conducted to evaluate the quality of the water in this country, despite the harm that water pollution causes to the environment and human health. Water quality monitoring accounts for less than 2% of the total water management budget, according to a review of the 2015–2020 WSDP budget (Republic of Tanzania Ministry of Water, 2020).
The LRC is found in the Kilolo district of the Iringa region, one of the primary rivers that feed into the GRR, which is regarded as the most significant river system in Tanzania (Seeteram et al., 2019). GRR holds significant importance as a major tributary in the Rufiji Basin, Tanzania. It contributes to water flow to support various crucial functions, including hydropower production, irrigation, and sustaining livelihoods in rural areas (Gervas et al., 2019). According to Kadigi et al. (2004), hydropower production from the river accounts for 50% of Tanzania’s installed capacity. Furthermore, the GRR flows its waters into the Kilombero Ramsar Site, which sustains a diverse range of living organisms. Recently, the Kilolo district has seen a number of issues that necessitate careful examination and control. These challenges include the ongoing conversion of natural vegetation into agriculture, forest plantations, and human settlements that are allegedly having a negative impact on rainfall trends, water quality, and stream flow in the Kilolo district (Mbungu et al., 2021). There is also an increase in illegal water abstractions for various purposes, including irrigation. In addition, the country is experiencing electricity shortages and rationing as a result of the considerable reductions in river flow to the Mtera and Kidatu reservoirs brought on by the expansion of irrigated land (Gervas et al., 2019). Furthermore, population growth, livestock expansion, and poor land management practices have caused soil erosion and increased sediment load into the Lukosi River. The continuous agriculture and gardening activities along the catchment areas pose a pollution risk due to the use of fertilizers, herbicides, and pesticides. Despite these challenges, there is no current scientific information regarding the impact of human activities on the health condition of the Lukosi River. To ensure sustainable water management and planning in the LRC, it is crucial to understand the specific human activities taking place along the LRC and their impact on its health condition. Therefore, the perspective of this study was to determine the current status of the Lukosi River by examining the specific human activities taking place along the LRC and their impact on the river’s health. This study aims to provide crucial information on how these human activities affect the LRC, which is essential for sustainable water management and planning to support aquatic life that depends on the Lukosi River.
2 Materials and methods
2.1 Study area
The study was conducted in the Kilolo district of the Iringa region, situated at the north-eastern extremity, approximately 37 km from the regional administrative center (Mbungu et al., 2021) (Figure 1). This district covers an area of 7874.6 km2, hosting a population of 218,130, with geographic coordinates at 70-8030 S and 340-370 E (URT, 2012). Its elevation ranges from 900 to 2,700 m above sea level, predominantly characterized by alluvial soil. Temperatures oscillate between 15 and 27°C, while annual rainfall varies from 500 to 1,600 mm. Kilolo district is already home to a diverse array of small- and large-scale agricultural and forest plantations (PFP, 2017). The region primarily cultivates maize, legumes, fruits, and vegetables, contributing significantly to the nation’s breadbasket (URT, 2012).
2.2 Research design
The research used a cross-sectional research design, enabling the collection of data at a given time from a selected sample intended to accurately represent the entire population.
2.3 Data collection
Data were collected in the field through direct observation, household questionnaires, and a physical–chemical analysis of water parameters. The water parameter data were collected during the month of February 2023, and household questionnaires and direct observation were conducted during the month of May 2023.
2.3.1 Household questionnaire
The questionnaires were administered in Mahenge, Ruaha Mbuyuni, Mtandika “A,” and Msosa villages to selected households to gather data related to human activities taking place along the river and their impact on the resources within the river catchment. The study used a sample size of 30 respondents per sampled village, as recommended by Bailey (1994) and Yurdugul (2008). A total of 120 respondents were included from all four villages; only one member of the household was selected to represent a house.
2.3.2 Direct observation
Direct field observation was used to complement the information collected by questionnaire. The observation primarily focused on studying how individuals are involved in various human activities along the LRC, how they carry out their activities, and what type of technologies are used in their activities. Throughout the study, relevant observations were documented, and photographs were taken to provide visual evidence and support for the findings.
2.3.3 Sampling sites selection for physical–chemical analysis
The three sampling points of the study area were randomly selected using global positioning systems (GPSs) (Table 1). Sampling points were selected considering that the samples taken are representative of the different sources from which water was obtained. The choice of the stations was made to better represent the quality of the Lukosi River water. The three sampling stations in the Lukosi River were designated as follows: Ls1 (upstream), where the river enters the villages; Ls2 (midstream), with moderate human activities; and Ls3 (downstream), which experiences higher human activities and is near the place where the Lukosi River connects with the GRR.
2.3.4 Sample collection
Water samples were collected from the Lukosi River following the standard procedure described by the American Public Health Association (APHA) (1998). Each sample was collected in a pre-washed 500 mL PVC plastic container, labeled with relevant codes. Triplicate 500 mL of samples were taken from each site. The water samples were temporarily stored in an ice-packed cooler while being transported and refrigerated at 4°C in the laboratory prior to analysis, as outlined by Gangwar et al. (2012).
2.3.5 Water quality analysis
The water quality parameters were analyzed using standard analytical methods [American Public Health Association (APHA), 1998; Gebreyohannes et al., 2015; Omary et al., 2023]. Temperature, pH, electric conductivity (EC), total dissolved solids (TDSs), and DO were measured on-site using a portable multi-parameter analyzer, the HANNA HI 9829 (Hanna Instruments company, Loveland, USA), while turbidity was determined on-site using a Turner designs AquaFluor 8000-001 turbidity meter (Figure 2A). Biochemical oxygen demand (BOD) was measured in the laboratory as oxygen consumed over a 5-day test period (BOD5) at 20°C [American Public Health Association (APHA), 1998]. Using the Hach Lange kits (Hack company, Loveland, USA), nitrate (NO3) and ammonium (NH3) concentrations were measured (Gebreyohannes et al., 2015). The examination of total hardness, calcium (Ca), magnesium (Mg), manganese (Mn), zinc (Zn), iron (Fe), copper (Cu), fluorine (F), and chlorides (Cl) was also conducted in the laboratory in accordance with standard American Public Health Association (APHA) (1998) protocols (Figure 2B). A nephelometric turbidity meter was used to measure the concentrations of sulfate (SO4) (TAHP, 1999). All chemicals used were of high purity and analytical grade. In order to avoid chemical contamination, fresh reagents were used, and great care was taken throughout the experiment.
2.4 Data analysis
The data were statistically analyzed using the Statistical Package for Social Sciences (SPSS), version 20. A one-sample t-test was used to measure the significance of the difference between the measured water quality parameter and the water quality criteria established by the WHO (2017) and the TBS (2006), with significance determined at a level of p < 0.05. Microsoft Excel software was used for additional data analysis.
In order to obtain a comprehensive picture of the entire surface water quality within the catchment, the water quality index (WQI) was used to determine the overall water quality status of the catchment. The WQI is used to simplify and convey scientific water quality information by combining the influence of various water quality parameters into a single-digit score that describes the overall water quality in a watershed (Koleka et al., 2023). Policymakers and the general public can obtain scientifically based information on river quality status through the index, which provides meaningful and comprehensible information on water quality (Wong et al., 2021). The weighted arithmetic index method was used to calculate the WQI. The method categorizes water quality based on purity levels by analyzing commonly measured water quality variables (Kizar, 2018; Paun et al., 2016). This approach has been extensively utilized by researchers (Singh et al., 2013). The obtained results were assigned to the classification of water quality based on the calculated WQI (Table 2).

Table 2. Classification of water quality based on the calculated WQI (Al-Mashagbah, 2015).
2.5 WQI calculation
Step 1: Calculation of the unit weight (Wn) factors for each parameter by using the formula:
where:
Sn = Standard desirable value of the nth parameters.
On summation of all selected parameters unit weight factors, WN = 1 (unity).
Step 2: Calculation of the sub-index (QN) value by using the formula.
where:
Vn = Mean concentration of the nth parameters.
Sn = Standard desirable value of the nth parameter.
Vo = Actual value of the parameters in pure water (generally Vo = 0, for most parameters except for pH and DO).
Step 3: Combine steps 1 and 2. The WQI was calculated as follows:
3 Results and discussion
3.1 Human activities identified along the LRC
The identified human activities along the LRC were gardening, agriculture, livestock keeping, sand mining, brick-making, car washing, and fishing (Figures 3, 4). These results were consistent with Munishi et al. (2011), who found that agricultural production was practiced by over 98% of the population in the GRR basin, followed by livestock grazing and fishing. Agriculture involves the large-scale cultivation of crops and livestock for food, fiber, and other products, typically involving mechanized processes and often conducted for commercial purposes. In contrast, gardening is the cultivation of plants, typically on a smaller scale, for personal purposes. Farmers in the LRC heavily relied on inorganic fertilizers, pesticides, and irrigation to enhance their crop yields. The majority of respondents reported using inorganic fertilizers (95% in agriculture and 86% in gardening) and pesticides (91% in agriculture, 72% in gardening) (Tables 3, 4), highlighting the extensive use of these chemicals along the LRC. The chi-square test results for both agriculture and gardening activities indicated highly statistically significant associations between these activities and the use of inorganic fertilizers and pesticides (p = 0.000***). The pesticide and inorganic fertilizer applications on crops contributed to chemical deposits in the soil, which are carried through runoff and leaching into rivers, lakes, and groundwater; these bring about adverse effects to the living organisms and the environment in general (Massawe and Nyoki, 2019). Moreover, irrigation practices, which were adopted by 90% of agricultural operations and 83% of gardening activities (Table 5), were aligned well with the observations made during data collection; both pumping and scheme-based irrigation methods were observed in the field (Figure 5). Due to these practices, the water supply from the Lukosi River to the GRR either ceases or becomes minimal during the dry season, leading to the complete drying up of the GRR during the dry season over the past 10 years (Cour et al., 2005). This dryness had implications for the livelihood of the people, the economy, and significant biodiversity.
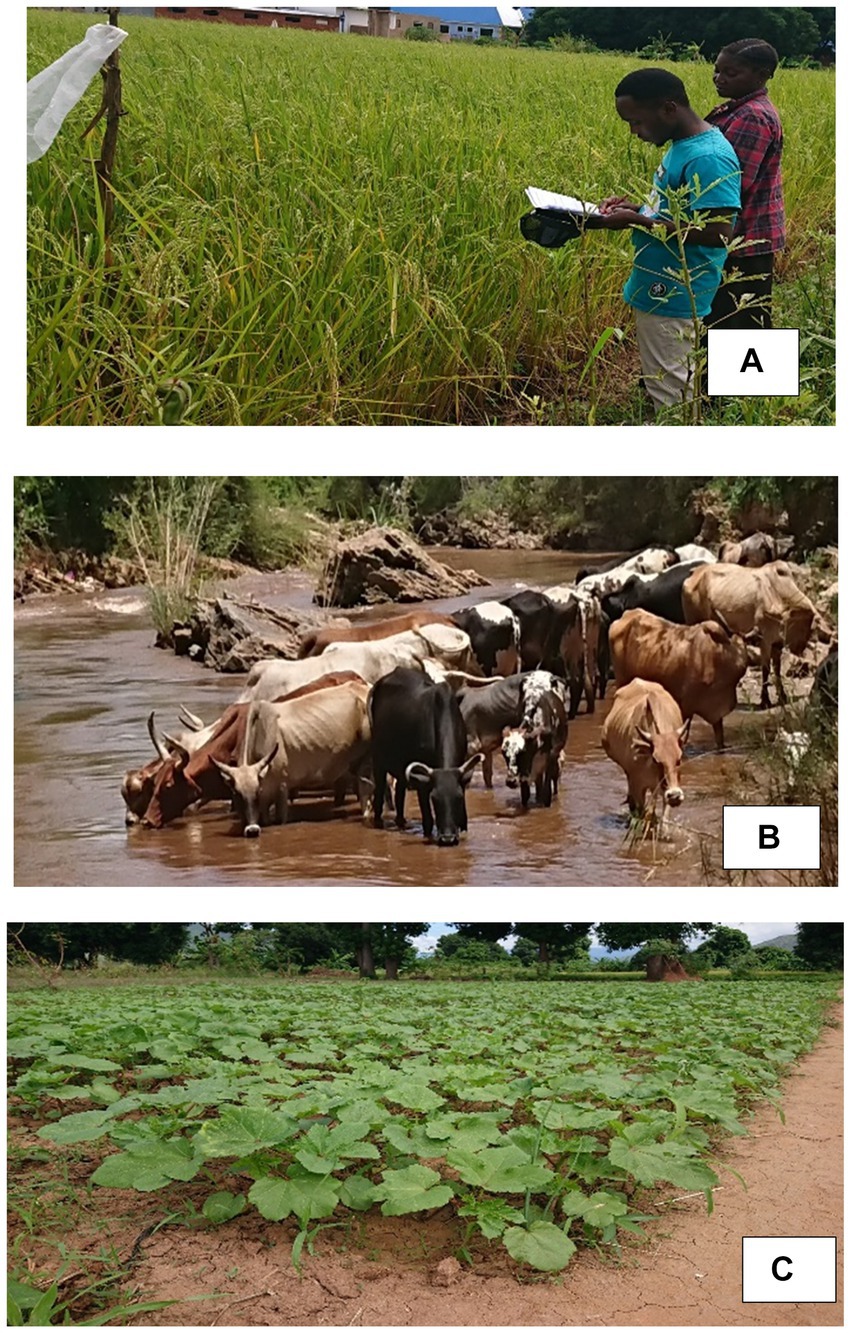
Figure 4. Some of human activities identifies along the LRC. (A) Paddy cropping; (B) Livestock grazing; (C) Vegetable gardening.

Table 3. Information on agriculture and gardening activities, specifically focusing on the usage of inorganic fertilizers.

Table 4. Information on agriculture and gardening activities, specifically focusing on the usage of pesticides.

Table 5. Information on agriculture and gardening activities, specifically focusing on irrigation practices.
Livestock keeping was also identified along the LRC, and this activity can cause different environmental challenges such as water pollution, habitat degradation, and biodiversity loss. Similar to our findings, Kumar et al. (2013) emphasized the environmental challenges posed by livestock keeping, such as water pollution and habitat degradation due to manure runoff, resulting in eutrophication and harmful algal blooms. George et al. (2004) also reported that unrestricted livestock access to rivers results in direct deposition of waste, introducing harmful pathogens, nutrients, and sedimentation, adversely impacting water quality, and aquatic health (George et al., 2004). Additionally, overgrazing practices led to soil erosion, compaction, and habitat degradation, diminishing ecosystem resilience and biodiversity (Matano et al., 2015).
Sand mining and brick-making activities were also identified along the LRC, and these activities may cause riverbank erosion, alteration of flow, and disturbance to aquatic organisms. Similar to our findings, Dufour et al. (2018) and Raphael and Makarius (2022) highlighted the environmental impacts of these activities, such as riverbank erosion, alteration of flow, and disturbance to aquatic organisms.
Car washing and fishing, though less identified, also can contribute to environmental harm through chemical use and wastewater generation. Our study identified car washing as a contributor to water pollution, which aligns with Powell et al. (2011), who noted the presence of pollutants such as petroleum hydrocarbons and heavy metals in car wash effluents.
3.2 Physico-chemical water parameters
pH serves as a critical indicator of the acidic or basic character of a solution, determined by the chemical compounds and biochemical reactions within it. In aquatic environments, pH levels hold paramount importance in governing physiological functions. These encompass vital aspects such as enzyme activity, metabolic rates, and ion exchange. Hence, maintaining an optimal pH range is crucial for ensuring the overall health and well-being of aquatic life forms. The measured mean pH values were 7.76 ± 0.006 for Ls1, 7.47 ± 0.01 for Ls2, and 7.47 ± 0.006 for Ls3. The pH values observed at all three sampling sites consistently fall within the acceptable range as recommended by both TBS and WHO standards (Table 6). This alignment indicates favorable conditions for the majority of aquatic organisms. The pH of an aquatic system is an important indicator of the water quality and the extent of pollution in the watershed areas. This finding was consistent with studies by Tembo (2009) and Turner and Chislock (2010) that emphasized the importance of pH in aquatic environments.
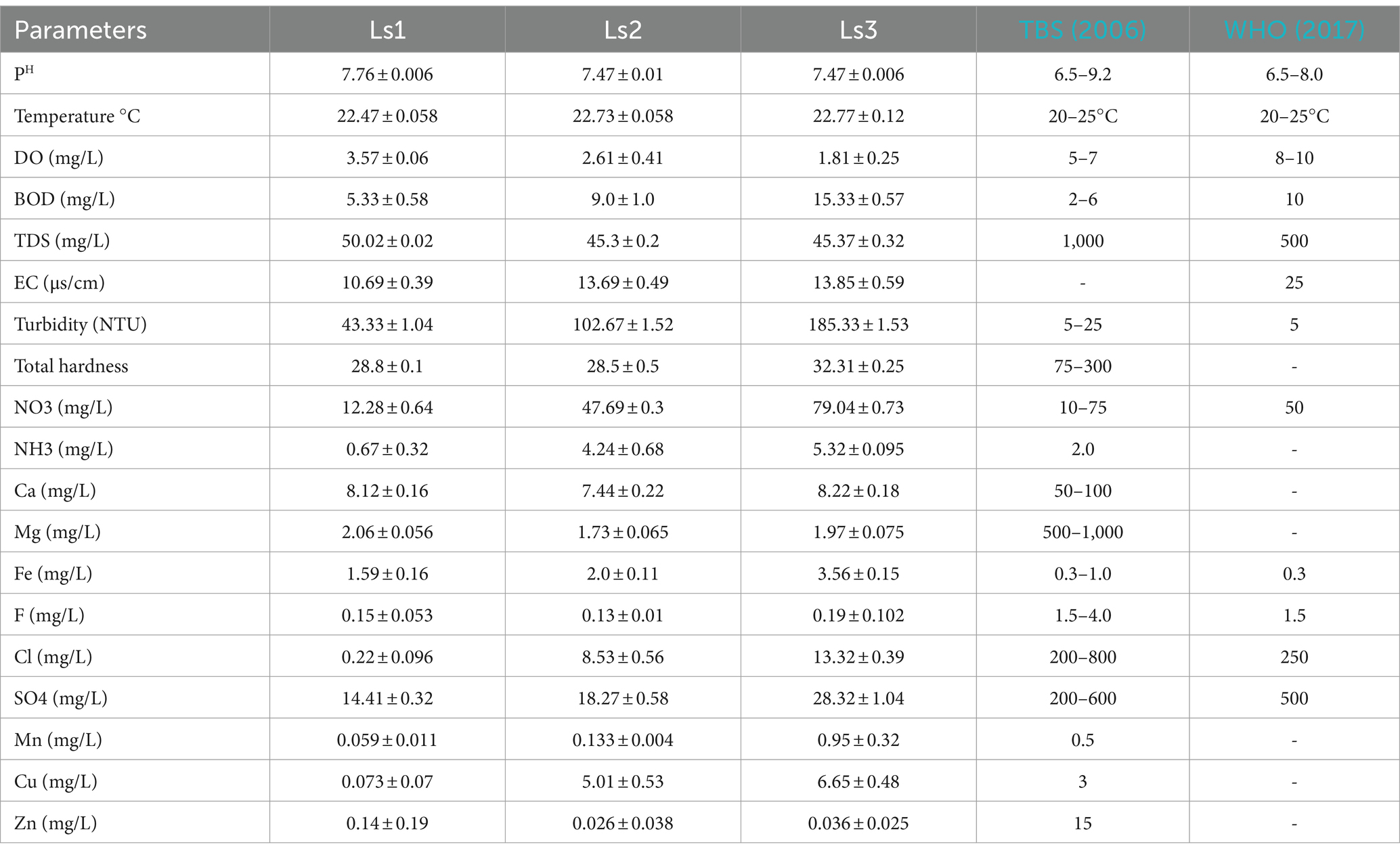
Table 6. Physicochemical water parameters recorded at three sampling points along the Lukosi River, and their respective values presented as mean ± standard deviation.
An increase in temperature brought on by climate change could alter the composition of species and lead to the extinction of endemic species (Carr and Rickwood, 2008). According to David et al. (2017), a change in temperature can alter the chemical properties of a wide range of parameters. Numerous studies (Koch et al., 2013; Pansch and Hiebenthal, 2019) reported that temperature variations were the most frequent and potent driver of changes in marine systems. The temperature measurements for Ls1, Ls2, and Ls3 were recorded as 22.47 ± 0.058°C, 22.73 ± 0.058°C, and 22.77 ± 0.12°C, respectively. The mean water temperature of the Lukosi River was within the acceptable range for aquatic life according to both TBS and WHO standards (Table 6). This indicates that the river’s temperature is suitable for supporting aquatic organisms. These findings are consistent with previous research, which reported temperature ranges of 20.5–22°C (Chiroma et al., 2012) and 19.01–23.93°C (Okweye, 2013).
Dissolved oxygen serves as a regulator of organisms’ metabolic processes, which in turn controls the metabolism of the entire biological community (Khan et al., 2012). Due to the fact that DO is essential for respiration, enough DO levels are absolutely necessary for the survival of aquatic species. The DO levels in the water samples collected from Ls1, Ls2, and Ls3 locations exhibited noticeable variations. The measured mean DO values were as follows: 3.57 ± 0.06 mg/L for Ls1, 2.61 ± 0.41 mg/L for Ls2, and 1.81 ± 0.25 mg/L for Ls3. The highest DO levels were observed in the Ls1 area, while the lowest DO levels were recorded in the Ls3 area. The obtained Do levels in all three sampling sites were found to be below the recommended limits set by both TBS and WHO, as indicated in Table 6. Our study observed DO levels below recommended limits, posing challenges for aquatic organisms. This aligns with findings by Mugidde et al. (2005) and Addo et al. (2013), who reported that low DO levels lead to hypoxia, endangering aquatic life and disrupting ecosystem dynamics. This shows that aquatic organisms may face difficulties surviving in the Lukosi River due to lower oxygen levels. The obtained DO level was due to human activities such as agriculture, livestock, and gardening activities, primarily occurring in the midstream and downstream areas of the river. In addition, according to Aiyesanmi et al. (2006), organic wastes and other nutrient inputs from industrial and sewage discharges, agricultural runoff, and urban runoff might also cause lower oxygen levels. Thus, the DO values measured in the Lukosi River were not suitable for the life of the aquatic organisms. Aquatic life is negatively impacted by DO concentrations below 5.0 mg/L (Sinha and Biswas, 2011).
The term “BOD” refers to the quantity of oxygen that bacteria need in order to decompose the degradable organic matter in water into simpler compounds (Suthar et al., 2010). Since it indicates the level of pollution, BOD is a crucial indicator for the aquatic ecosystem (Mbaruku, 2016). From the Lukosi River, the BOD levels showed variations across the sampling sites (Ls1, Ls2, and Ls3). In Ls1, a low BOD value of 5.33 ± 0.58 mg/L was observed, indicating minimal organic pollution. In Ls2, the BOD levels rose to 9.0 ± 1.0 mg/L, suggesting a moderate level of organic matter. LS3 had the highest BOD value of 15.33 ± 0.57 mg/L, indicating a high level of pollution potentially impacting water quality. The obtained values of Ls1 and Ls2 fall within the recommended values set by the WHO and TBS, while the values of Ls3 appear to be above the recommended value set by the WHO and TBS (Table 6). The high BOD value at Ls3 may be caused by agricultural operations, surface runoff, livestock keeping, and underground water movement containing leachates from a solid waste landfill located near the Lukosi River, all of which could exacerbate organic pollution (Al-badaii et al., 2013). A higher BOD indicates a more rapid depletion of oxygen in the water body, as microorganisms consume DO. Similar results were found in previous studies, which demonstrated that a high BOD level lowers the amount of DO in the river (Omary et al., 2023).
Total dissolved solid is an indicator of the salinity behavior of water (Dahiya and Kaur, 1999). The TDS measurements were conducted and resulted in an average mean value of 50.02 ± 0.02 mg/L for Ls1, 45.3 ± 0.2 mg/L for Ls2, and 45.37 ± 0.32 mg/L for Ls3. All these values were found to be lower than the permissible limits stipulated by both TBS and WHO (Table 6), which appear to be unsuitable conditions for aquatic organisms. According to Mbaruku (2016), TDS measures the capacity of water to dissolve various inorganic and certain organic minerals or salts, such as sulfates, magnesium, chlorides, bicarbonate, sodium, calcium, and potassium. Furthermore, in accordance with Mezgebu et al. (2019), elevated TDS concentrations hinder algal productivity and growth, serving as an indicator of poor water quality.
Electric conductivity is a measurement of the ability of water to carry electric current. It denotes the quantity of all dissolved salts in general. Conductivity measures the overall ionic content of water, providing an indication of whether the water is fresh or not. According to Olatayo (2014), the conductivity of water is directly related to its overall salt content. Calcium ion (Ca2+), magnesium (Mg 2+), hydrogen trioxocarbonate (HCO 3), trioxocarbonate (iv) (CO 3), trioxonitrate (v) (NO 3), and tetraoxophosphate (vi) (PO 4) are the main components of the dissolved chemicals in water (Olatayo, 2014). The measured EC values were 10.69 ± 0.39 μS/cm for Ls1, 13.69 ± 0.49 μS/cm for Ls2, and 13.85 ± 0.59 μS/cm for Ls3. The recorded EC values from all three sampling sites were below the acceptable range established by the WHO standards (Table 6), suggesting unfavorable conditions for the growth and well-being of aquatic species.
Turbidity, particularly in water, is a crucial factor in determining the quality of the water. The mean measured turbidity values from the Lukosi River were 43.33 ± 1.04 NTU for Ls1, 102.67 ± 1.52 NTU for Ls2, and 185.33 ± 1.53 NTU for Ls3. The turbidity values in all three sampling sites were higher than the recommended limits set by both WHO and TBS standards (Table 6). This suggests that the river as a whole is generally contaminated and endangering aquatic life. This may be due to the daily human activity such as washing of different vehicles, surface runoff, and other activities (Alemayehu, 2001). Aquatic species' behavior and feeding habits may be affected by this rise in turbidity, which may alter light penetration and visibility. Similar findings were reported by Joshi et al. (2009) and Murhekar (2011), who highlighted the impact of high turbidity on aquatic species’ behavior and visibility.
Total hardness is a property of water that hinders lather formation with soap and increases the boiling point of water (Mezgebe et al., 2015). It primarily depends on the presence of Ca or Mg salts, or both (Trivedy and Goel, 1986). Water is divided into three groups according to its hardness: soft water (0–75 mg/L), moderately hard water (76–150 mg/L), and hard water (151–300 mg/L). The measured total hardness values for Ls1, Ls2, and Ls3 were found to be 28.8 ± 0.1, 28.5 ± 0.5, and 32.31 ± 0.25, respectively. In all sampling sites, total hardness was found to be below the acceptable range recommended by the TBS (Table 6). The hardness values recorded for this study were within the soft classification. Standard total hardness levels are essential for the health and survival of aquatic organisms, as they provide the necessary minerals for their physiological processes. Other studies on river water similarly found lower values for total hardness (Joshi et al., 2009; Sharma et al., 2013), indicating that the water is classified as soft and suitable for aquatic organisms.
Ca and Mg are prevalent components found in natural water sources, and their respective salts significantly contribute to water hardness. In the present study, Ca concentrations were measured with average mean values of 8.12 ± 0.16 mg/L for Ls1, 7.44 ± 0.22 mg/L for Ls2, and 8.22 ± 0.18 mg/L for Ls3. Conversely, Mg levels were measured, resulting in average mean values of 2.06 ± 0.056 mg/L for Ls1, 1.73 ± 0.065 mg/L for Ls2, and 1.97 ± 0.075 mg/L for Ls3. The recorded values for both Ca and Mg were below the permissible limits set by the TBS. Although Ca and Mg are essential minerals for the development and health of aquatic organisms, the recorded levels were not suitable for their well-being.
NO3 plays a crucial role as a vital nutrient in aquatic ecosystems, promoting plant growth, while simultaneously acting as a limiting factor for algal growth (Bwalya, 2015). Nitrogen-containing elements are essential for all biotic processes in the aquatic environment (Bwalya, 2015). The recorded average mean nitrate values were 12.28 ± 0.64 mg/L for Ls1, 47.69 ± 0.3 mg/L for Ls2, and 79.04 ± 0.73 mg/L for LS3. The LS1 and Ls2 sites were within the recommended range set by both WHO and TBS; however, at the Ls3 site, the levels exceeded the limits recommended by both TBS and WHO (Table 6). According to Mwangi (2014), human activities taking place along the river cause an increase in nitrate levels. Water bodies become contaminated when runoff from agricultural activity introduces fertilizers to the watercourses during heavy rainfall. The increase in nitrate leads to excessive algal growth, which reduces oxygen levels as it decomposes and affects certain aquatic organisms that cannot withstand anaerobic conditions (Mwangi, 2014).
NH3 and SO4: NH3 levels were measured at 0.67 ± 0.32 mg/L for Ls1, 4.24 ± 0.68 mg/L for Ls2, and 5.32 ± 0.095 mg/L for Ls3. Ls1 falls below the TBS recommended range, whereas Ls2 and Ls3 exceed it (Table 6). The elevated levels of ammonia in Ls2 and Ls3 may be attributed to various human activities occurring in those regions. For instance, the increased use of fertilizers in agricultural practices within Ls2 and Ls3 could be a significant contributor to the higher ammonia levels. Monitoring and appropriately managing elevated ammonia levels is crucial, as they can pose toxicity risks to aquatic organisms. On the other hand, the sulfate (SO4) concentrations were determined to be 14.41 ± 0.32 mg/L for Ls1, 18.27 ± 0.58 mg/L for Ls2, and 28.32 ± 1.04 mg/L for Ls3. All of these measurements fall below the recommended range established by both TBS and WHO. Sulfate plays a crucial role in water chemistry, and the recorded levels were below the acceptable limit for sustaining aquatic life.
The presence of high levels of Cl in river waters may be a sign of sewage or industrial waste pollution or the entry of saltwater into freshwater systems. The Cl concentrations were measured, resulting in an average value of 0.22 ± 0.096 mg/L for Ls1, 8.53 ± 0.56 mg/L for Ls2, and 13.32 ± 0.39 mg/L for Ls3. All of these measurements fall below the allowable limit stipulated by both TBS and WHO (Table 6). The reported amounts of chloride were below acceptable ranges for the health of aquatic life, which depends on it as an important electrolyte.
Fe, F, and Mn: Fe concentrations were measured at 1.59 ± 0.16 mg/L for Ls1, 2.0 ± 0.11 mg/L for Ls2, and 3.56 ± 0.15 mg/L for Ls3. Ls1 and Ls2 values were within the TBS and WHO recommended range, but Ls3 exceeded the limit. Fe is an essential micronutrient for aquatic organisms. The elevated levels observed were likely due to soil erosion, which was exacerbated by human activities near the river, leading to bare soil and subsequent erosion of the riverbanks. It is important to assess the level of Fe to ensure it remains within the acceptable standards for the health and suitability of aquatic life. F levels were assessed, yielding mean values of 0.15 ± 0.053 mg/L for Ls1, 0.13 ± 0.01 mg/L for Ls2, and 0.19 ± 0.102 mg/L for Ls3. These measurements fall below the range recommended by both TBS and WHO. The Mn concentrations measured in Ls1, Ls2, and Ls3 were found to be 0.059 ± 0.011 mg/L, 0.133 ± 0.004 mg/L, and 0.95 ± 0.32 mg/L, respectively. These values fall within the acceptable range recommended by TBS. Mn, an essential trace element for aquatic organisms, supports their well-being, and the recorded levels provide the necessary support.
Cu and Zn: Cu levels were measured at 0.073 ± 0.07 mg/L for Ls1, 5.01 ± 0.53 mg/L for Ls2, and 6.65 ± 0.48 mg/L for Ls3. LS1 falls within the recommended TBS range, while Ls2 and LS3 exceed the recommended limit. Elevated Cu levels in aquatic environments can pose a significant threat to the health and well-being of aquatic organisms. The obtained results primarily come from agricultural runoff, especially due to the use of inorganic fertilizer, pesticide applications, and residential waste. Therefore, monitoring and effective management strategies are strongly recommended to mitigate potential harm. The Zn concentrations in all three areas (0.14 ± 0.19 mg/L for Ls1, 0.026 ± 0.038 mg/L for Ls2, and 0.036 ± 0.025 mg/L for Ls3) were below the acceptable range defined by the TBS. The obtained Zn levels were not suitable for aquatic species, where it is needed for a number of physiological processes such as enzyme activity, growth, and reproduction (Nogawa et al., 2004).
3.3 Water quality status based on calculated WQI
The calculated WQI was used to assess the overall water quality status of the entire catchment. The results revealed a WQI of 300.3252 for Ls1, 405.7578 for Ls2, and 763.4208 for Ls3 (Tables 7–9). These values indicate that the water in the catchment was unsuitable for sustaining aquatic organisms and was unfit for domestic use (Table 2).
4 Conclusion
This research provides a comprehensive assessment of the impact of human activities on the health of the LRC, utilizing selected physico-chemical parameters as indicators of water quality. The predominant agricultural and gardening practices near the river, coupled with livestock keeping, sand mining, and brick-making activities, have collectively contributed to the alteration of the water quality. Notably, the excessive use of fertilizers, pesticides, and irrigation has raised concerns about water pollution, posing a significant threat to aquatic organisms. The comprehensive analysis of the various parameters in the Lukosi River reveals concerning levels of pollution. Specifically, parameters such as DO, turbidity, BOD, NH3, and NO2, exceed the allowable limits established by both the TBS and WHO for water quality. Furthermore, metal elements, including Fe and Cu, were found to be higher than the recommended limit set by the WHO. On the other hand, TDS, EC, total hardness, Ca, Fe, F, Cl, and SO4 were found to be below the recommended limit set by both the TBS and WHO for water quality. Moreover, the WQI indicated that the water in the catchment was unsuitable for sustaining aquatic organisms and was unfit for domestic use. Our findings align with various studies in the literature, highlighting the significant impact of human activities on water quality and aquatic life along the LRC. These findings underscore the urgent need for continuous and vigilant monitoring of the Lukosi River water quality. Implementing strict protective measures is crucial to reducing pollution in the Lukosi River and ensuring compliance with standards. This proactive approach safeguards community health, supports aquatic life, and promotes long-term viability through sustained pollution control and environmental conservation efforts.
Based on the findings, the following recommendations are proposed by the authors to mitigate the impact of human activities on the health condition of the Lukosi River:
1. Control the pollution source identified: To control the identified sources of pollution, especially those responsible for excessive nutrient loading, heavy metal contamination, and organic matter discharge into the river.
2. Continuous monitoring: To establish a robust and regular water quality monitoring program to track changes and trends over time.
3. Restoration of Riparian zones: Rehabilitating and protecting the riparian zones along the Lukosi River can help stabilize the ecosystem, reduce erosion, and filter pollutants before they enter the water.
4. Community engagement and awareness: Engaging local communities and stakeholders in the conservation efforts is essential. Raising awareness about sustainable agricultural practices and the overall importance of preserving the Lukosi River will contribute significantly to long-term improvements.
5. Collaborative efforts: Encouraging collaboration between governmental bodies, non-governmental organizations, research institutions, and local communities will foster a more comprehensive and effective approach to tackling water quality issues.
Data availability statement
The original contributions presented in the study are included in the article/supplementary material, further inquiries can be directed to the corresponding author.
Ethics statement
Written informed consent was obtained from the individual(s), and minor(s)’ legal guardian/next of kin, for the publication of any potentially identifiable images or data included in this article.
Author contributions
AN: Conceptualization, Data curation, Formal analysis, Funding acquisition, Investigation, Methodology, Project administration, Resources, Software, Validation, Visualization, Writing – original draft, Writing – review & editing. VN: Conceptualization, Methodology, Project administration, Resources, Supervision, Validation, Visualization, Writing – review & editing. ML: Conceptualization, Formal analysis, Funding acquisition, Investigation, Methodology, Project administration, Resources, Supervision, Validation, Visualization, Writing – review & editing.
Funding
The author(s) declare financial support was received for the research, authorship, and/or publication of this article. This research was funded by the UNESCO Chair in Ecohydrology and Transboundary Water Management at Sokoine University of Agriculture in Tanzania.
Acknowledgments
The authors would like to express their gratitude to the Iringa Municipal Council and Kilolo District Authority for their cooperation and material support throughout the research process. Special thanks are extended to Emanus Emmanuel Kamulasi, Illuminata Kassiana, Fenitha Josephy Msafiri, and Suzana Samson Twaha from the Iringa Water Quality Laboratory office for their invaluable assistance in data collection and laboratory study.
Conflict of interest
The authors declare that the research was conducted in the absence of any commercial or financial relationships that could be construed as a potential conflict of interest.
Publisher’s note
All claims expressed in this article are solely those of the authors and do not necessarily represent those of their affiliated organizations, or those of the publisher, the editors and the reviewers. Any product that may be evaluated in this article, or claim that may be made by its manufacturer, is not guaranteed or endorsed by the publisher.
References
Addo, M. A., Darko, E. O., Gordon, C., and Nyarko, B. J. B. (2013). Water quality analysis and human health risk assessment of groundwater from open-wells in the vicinity of a cement factory at Akporkloe, Southeastern Ghana. e-J. Sci. Technol. 8, 15–30.
Aiyesanmi, A. F., Ipinmoroti, K. O., and Adeeyinwo, C. E. (2006). Baseline Water Quality Status of Rivers Within Okitipupa Southeast Belt of the Bituminous Sands Field of Nigeria. Niger. J. Sci. 40, 62–71.
Al-Badaii, F., Shuhaimi-Othman, M., and Gasim, M. B. (2013). Water Quality Assessment of the Semenyih River, Selangor, Malaysia. J. Chemother. 2013:871056. doi: 10.1155/2013/871056
Alemayehu, T. (2001). The impact of uncontrolled waste disposal on surface water quality in Addis Ababa, Ethiopia. J. Sci. 24, 93–104.
Ali, M., Salam, A., Ahmed, N., Khan, B., and Khokhar, M. Y. (2004). Monthly Variation in Physicochemical Characteristics and Metal Contents of Indus River at Ghazi Ghat, Muzaffargarh, Pakistan. Pak. J. Zool. 36, 295–300.
Al-Mashagbah, A. F. (2015). Assessment of Surface Water Quality of King Abdullah Canal, Using Physico-Chemical Characteristics and Water Quality Index, Jordan. JWARP 7, 339–352. doi: 10.4236/jwarp.2015.74027
Bwalya, E. (2015). Assessment of water quality in urban rivers: A case study of Mansa River in Luapula province of Zambia. University of Zimbabwe faculty of engineering department of Civil engineering. MSc Thesis.
Carr, G. M., and Rickwood, C. J. (2008). Water quality index for biodiversity technical development document. Report Prepared for Biodiversity Indicators Partnership, World Conservation Monitoring Center, Cambridge, 64 p.
Chiroma, T. M., Ebewele, R. O., and Hymore, F. K. (2012). Levels of Heavy Metals (Cu, Zn, Pb, Fe and Cr) in Bushgreen and Roselle Irrigated with Treated and Untreated Urban Sewage Water. Int. Res. J. Environ. Sci. 1, 50–55.
Cour, JG, Kadigi, RM, Lankford, BA, Yawson, D, and Tumbo, S. (2005). “A decision-aid for the management of water resources in the Ruaha River Basin, Tanzania” in Paper presented at the East Africa Integrated River Basin Management Conference, Sokoine University of Agriculture, Morogoro, Tanzania. 7–9 March 2005. [Vol.1]. Funded by IWMI, and others. 11p.
Dahiya, S., and Kaur, A. J. (1999). Assessment of physico-chemical characteristic of under groundwater in rural areas of Tosham subdivision, Bhiwani-Haryana. Environ. Pollut. 6, 281–288.
David, O. A., Osonubi, O., Olaiya, C. O., Agbolade, J. O., Ajiboye, A. A., Komolafe, R. J., et al. (2017). Anatomical response of wheat cultivars to drought stress. Ife J. Sci. 19, 323–331.
Deepak, S., and Singh, N. U. (2014). The Relationship between Physico-chemical Characteristics and Fish Production of Mod sagar Reservoir of Jhabua District, MP, India. Res J Recent Sci 3, 82–86.
Dufour, S., Rodríguez-González, P. M., and Laslier, M. (2018). Tracing the scientific trajectory of riparian vegetation studies: Main topics, approaches and needs in a globally changing world. Sci. Total Environ. 653, 1168–1185. doi: 10.1016/j.scitotenv.2018.10.383
Gangwar, R. K., Khare, P., Singh, J., and Singh, A. P. (2012). Assessment of physicochemical properties of water: River Ramganga at Bareilly, UP. J. Chem. Pharm. Res. 4, 4231–4234.
Gebreyohannes, F., Gebrekidan, A., Hadera, A., and Estifanos,. (2015). Investigations of physico-chemical parameters and its pollution implications. Momona Ethiop. J. Sci. 7, 240–257. doi: 10.4314/mejs.v7i2.7
George, M. R., Tate, K. W., Larsen, R. E., Gerlach, J. D., and Fulgham, K. O. (2004). Cattle grazing has varying impacts on stream-channel erosion in oak woodlands. Calif. Agric. 58, 138–143. doi: 10.3733/ca.v058n03p138
Gereta, E., Wolanski, E., Borner, M., and Serneels, S. (2002). Use of an ecohydrology model to predict the impact on the Serengeti ecosystem of deforestation, irrigation and the proposed Amala Weir Water Diversion Project in Kenya. Ecohydrol. Hydrobiol. 2, 135–142.
Gervas, R., Mulungu, D. M. M., and Nobert, J. K. (2019). Assessment of surface water resources in Great Ruaha River Sub-Basin Tanzania: Application of WEAP model for water allocation and utilization impacts analysis. TJET 38, 27–46. doi: 10.52339/tjet.v38i1.494
Greve, P., Kahil, T., and Mochizuki, J. (2018). Global assessment of water challenges under uncertainty in water scarcity projections. Nat. Sustain. 1, 486–494. doi: 10.1038/s41893-018-0134-9
Hellar-Kihampa, H. (2011). Pesticide residues in four rivers running through an intensive agricultural area, Kilimanjaro, Tanzania. J. Appl. Sci. Environ. Manag. 15, 307–316.
Joshi, D. M., Kumar, A., and Agrawal, N. (2009). Studies on physicochemical parameters to assess the water quality of river Ganga for drinking purpose in Haridwar district. Res. J. Chem. 2, 195–203.
Kadigi, R. M. J., Kashaigili, J. J., and Mdoe, N. S. (2004). The economics of irrigated paddy in Usangu Basin in Tanzania: Water utilization, productivity, income and livelihood implications. Phys. Chem. Earth 29, 1091–1100. doi: 10.1016/j.pce.2004.08.010
Kanu, I., and Achi, O. K. (2011). Industrial Effluents and their Impact on Water Quality of Receiving Rivers in Nigeria. J. Appl. Technol. Environ. Sanit. 1, 75–86.
Khan, R., Jadhav, M., and Ustad, I. (2012). Physicochemical analysis of Triveni Lake Water of Amravati District in (MS) India. Biosci. Discov. 3, 64–66.
Khatri, N., and Tyagi, S. (2015). Influences of natural and anthropogenic factors on surface and Groundwater quality in rural and urban areas. Front. Life Sci. 8, 23–39. doi: 10.1080/21553769.2014.933716
Kizar, F. M. (2018). A comparison between weighted arithmetic and Canadian methods for a drinking water quality index at selected locations in shatt al-kufa. IOP Conf. Ser: Mater. Sci. Eng. 433:012026. doi: 10.1088/1757-899X/433/1/012026
Koch, M. G., Bowes, C., Ross, X. H., and Zhang,. (2013). Climate change and ocean acidification effects on seagrasses and marine macroalgae. Glob. Chang. Biol. 19, 103–132. doi: 10.1111/j.13652486.2012.02791.x
Koleka, M., Stanley, N., and Kanyerere, T. (2023). Assessing Feasibility of Water Resource Protection Practice at Catchment Level: A Case of the Blesbokspruit River Catchment, South Africa. Water 15:2394. doi: 10.3390/w15132394
Kumar, R. R., Park, B. J., and Cho, J. Y. (2013). Application and environmental risks of livestock manure. J. Korean Soc. Appl. Biol. Chem. 56, 497–503. doi: 10.1007/s13765-013-3184-8
Lawson, E. O. (2011). Physico-Chemical Parameters and Heavy Metal Contents of Water from the Mangrove Swamps of Lagos Lagoon, Lagos, Nigeria. Adv. Biol. Res. 5, 08–21.
Liyanage, C. P., and Yamada, K. (2017). Impact of Population Growth on the Water Quality of Natural Water Bodies. Sustain. For. 9, 1–14. doi: 10.3390/su9081405
American Public Health Association (APHA) (1998) in Standard Methods for the Examination of Water and Wastewater. eds. L. S. Clesceri, A. E. Greenberg, and A. D. Eaton. 20th ed (Washington, D.C.: American Public Health Association), 1325.
Lynch, A. J., Elliott, V., Phang, S. C., Claussen, J. E., Harrison, I., Murchie, K. J., et al. (2020). Inland fish and fisheries integral to achieving the Sustainable Development Goals. Nat. Sustain. 3, 579–587. doi: 10.1038/s41893-020-0517-6
Massawe, P, and Nyoki, D. (2019). Effects of anthropogenic activities on availability of clean and safe water: A case of uluguru forest catchment areas Morogoro, Tanzania.
Matano, A., Kanangire, C., Anyona, D., Abuom, P., Gelder, F., Dida, G., et al. (2015). Effects of Land Use Change on Land Degradation Reflected by Soil Properties along Mara River, Kenya and Tanzania. Open J. Soil Sci. 5, 20–38. doi: 10.4236/ojss.2015.51003
Mbaruku, S. (2016). Assessment of River Health Using Physico-Chemical Parameter and Macro Invertebrates: A Case Study of Mungonya River in Kigoma, Tanzania. MSc Thesis. University of Zimbabwe, Harare, 102 p.
Mbungu, W. B., Balama, C. P., Maguzu, S., Sangiwa, M. J., and District, K. (2021). Spatial and Temporal Variability in Hydro-Meteorological Selected Variable in the Southern Highlands, Tanzania. Tanz. J. For. Nat. Conserv. 90, 10–23.
Mezgebe, K., Gebrekidan, A., Hadera, A., and Weldegebriel, Y. (2015). Assessment of physico-chemical parameters of Tsaeda Agam River in Mekelle City, Tigray, Ethiopia. Bull. Chem. Soc. Ethiop. 29, 377–385. doi: 10.4314/bcse.v29i3.5
Mezgebu, A., Lakew, A., and Lemma, B. (2019). Water Quality Assessment Using Benthic Macroinvertebrates as Bioindicators in Streams and Rivers around Sebeta, Ethiopia. Afr. J. Aquat. Sci. 44, 361–367. doi: 10.2989/16085914.2019.1685450
Mugidde, R, Gichuki, J, Rutagemwa, D, Ndawula, L, and Matovu, A (2005). “Status of Water Quality and Its Implication on Fishery Production” in The State of the Fisheries Resources of Lake Victoria and Their Management; pp. 106–112.
Munishi, P.K.T., Nice, N, Wilfred, J, Nshare, S, Stein, RM, Shirima, DD, et al. (2011). Valley bottom wetlands can serve for both biodiversity conservation and local livelihoods improvements, ecosystems biodiversity, PhD. ed. O Grillo. Available online at: http://www.intechopen.com/site (Accessed July 11, 2015).
Murhekar, G. H. (2011). Assessment of physico-chemical status of ground water samples in Akot city. Res. J. Chem. Sci. 1, 117–124.
Mwangi (2014). Land use practices and their impact on the water quality of the Upper Kuils River (Western Province, South Africa). MSc Thesis. University of the Western Cape.
Ngor, P. B., Legendre, P., Oberdorff, T., and Lek, S. (2018). Flow alterations by dams shaped fish assemblage dynamics in the complex Mekong-3S River system. Ecol. Indic. 88, 103–114. doi: 10.1016/j.ecolind.2018.01.023
Nogawa, K., Kobayashi, E., Okubo, Y., and Suwazono, Y. (2004). Environmental cadmium exposure, adverse effects and preventative measures in Japan. J. Biometals 17, 581–587. doi: 10.1023/B:BIOM.0000045742.81440.9c
Okweye, P. S. (2013). Seasonal Variation in physico-chemical parameters and Heavy Metals Assessment in Surface Water of North Alabama. Res. J. Chem. Environ. 17, 68–115.
Olatayo, A. A. (2014). Assessment of Physico-Chemical Parameters of Waters in Ilaje Local Government Area of Ondo State, Nigeria. Int. J. Fish. Aquat. Stud. 1, 84–92.
Oliveira, A., and Callisto, M. (2010). Benthic macroinvertebrates as bioindicators of water quality in an Atlantic Forest fragment. Ihering. Sér. Zool. 100, 291–300. doi: 10.1590/S0073-47212010000400003
Omary, R. R., Lalika, M. C. S., Nguvava, M., and Mgimwa, E. (2023). Macroinvertebrates as Bio Indicators of Water Quality in Pinyinyi River, Arusha Tanzania. J. Water Res. Protect. 15, 393–412. doi: 10.4236/jwarp.2023.158023
Pansch, C., and Hiebenthal, C. (2019). A new mesocosm system to study the effects of environmental variability on marine species and communities. Limnol. Oceanogr. Methods 17, 145–162. doi: 10.1002/lom3.10306
Paun, I, Cruceru, L, Chiriac, FL, Niculescu, M, Vasile, GG, and Marin, NM (2016) “Water quality indices—methods for evaluating the quality of drinking water” in Proceedings of the 19th INCD ECOIND International Symposium—SIMI 2016, “The Environment and the Industry”, Bucharest, Romania. October 13–14, 2016. 395–402.
PFP. (2017). Private Forest Programme. Forest Plantations Mapping of the Southern Highlands. Panda Miti Kibiashara. 73 pp.
Raphael, A, and Makarius, L. (2022). Ecohydrological Phytoregulation of Riverine Ecosystems Using Riparian Ecohydrological Phytoregulation of Riverine Ecosystems Using Riparian Vegetation. Accessed September.
Seeteram, N. A., Hyera, P. T., Kaaya, L. T., Lalika, M. C. S., and Anderson, E. P. (2019). Conserving rivers and their biodiversity in Tanzania. Water 11:2612. doi: 10.3390/w11122612
Sharma, P., Dubey, A., and Chatterjee, S. K. (2013). Physico-Chemical Analysis of Surface and Ground Water of Abhanpur Block in Raipur District, Chhattisgarh, India. Int. J. Innov. Technol. Explor. Eng. 2, 2278–3075.
Singh, P. K., Tiwari, A. K., Panigary, B. P., and Mahato, K. (2013). Water quality indices used for water resources vulnerability assessment using GIS technique: a review. Int. J. Earth Sci. Eng. 6, 1594–1600.
Sinha, S. N., and Biswas, M. (2011). Analysis of physico-chemical characteristics to study the water quality of a lake in Kalyani, West Bengal. Asian J. Exp. Biol. Sci. 2, 18–22.
Suthar, S., Sharma, J., Chabukdhara, M., and Nema, A. K. (2010). Water Quality Assessment of River Hindon at Ghaziabad, India: Impact of Industrial and Urban Wastewater. Environ. Monit. Assess. 165, 103–112. doi: 10.1007/s10661-009-0930-9
TAHP (1999). Technical Assistant Hydrology Project, Government of India & Government of the Netherlands. Standard Analytical Procedures for Water Analysis. New Delhi, India.
Tanzania National Bureau of Statistics (NBS) (2023). Census Information dissemi-nation platform 2022. Available online at. https://sensa.nbs.go.tz/ (Accessed July 7, 2023).
Tembo, R. N. (2009). The sub-lethal effects of low pH exposure on the chemoreception of Poecilia sphenops. Arch. Environ. Contam. Toxicol. 57, 157–163. doi: 10.1007/s00244-008-9255-x
Trivedy, R, and Goel, P. (1986). Chemical and Biological Methods for Water Pollution Studies, Environmental Publication, Karad: Maharastra, India.
Turner, A. M., and Chislock, M. F. (2010). Blinded by the stink: nutrient enrichment impairs the perception of predation risk by freshwater snails. Ecol. Appl. 20, 2089–2095. doi: 10.1890/10-0208.1
Uherek, C. B., and Gouveia, P. B. F. (2014). Biological monitoring using macroinvertebrates as bioindicators of water quality of maroaga stream in the Maroaga Cave System, Presidente Figueiredo, Amazon, Brazil. Int. J. Ecol. 2014:308149. doi: 10.1155/2014/308149
URT (2012). National population and housing census: General report. United Republic of Tanzania. Government Printer, Dar es Salaam, Tanzania. 264 pp.
Wong, Y. J., Shimizu, Y., and Kamiya, A. (2021). Application of artificial intelligence methods for monsoonal river classification in Selangor River basin. J. Environ. Monit. Assess. 193:438. doi: 10.1007/s10661-021-09202-y
Keywords: health condition, human activities, Lukosi River catchment, physico-chemical parameters, water quality index
Citation: Nyagongo AA, Ndibalema VG and Lalika MCS (2024) Assessing the impact of human activities on the health condition of the Lukosi River catchment using selected physico-chemical parameters as indicators of water quality. Front. Water. 6:1378849. doi: 10.3389/frwa.2024.1378849
Edited by:
Frank Onderi Masese, University of Eldoret, KenyaReviewed by:
P. Kamaraj, Bharath Institute of Science and Technology, IndiaTayssir Hamieh, Maastricht University, Netherlands
Thokozani Kanyerere, University of the Western Cape, South Africa
Copyright © 2024 Nyagongo, Ndibalema and Lalika. This is an open-access article distributed under the terms of the Creative Commons Attribution License (CC BY). The use, distribution or reproduction in other forums is permitted, provided the original author(s) and the copyright owner(s) are credited and that the original publication in this journal is cited, in accordance with accepted academic practice. No use, distribution or reproduction is permitted which does not comply with these terms.
*Correspondence: Ahmad A. Nyagongo, YWhtYWRueWFnb25nb0BnbWFpbC5jb20=