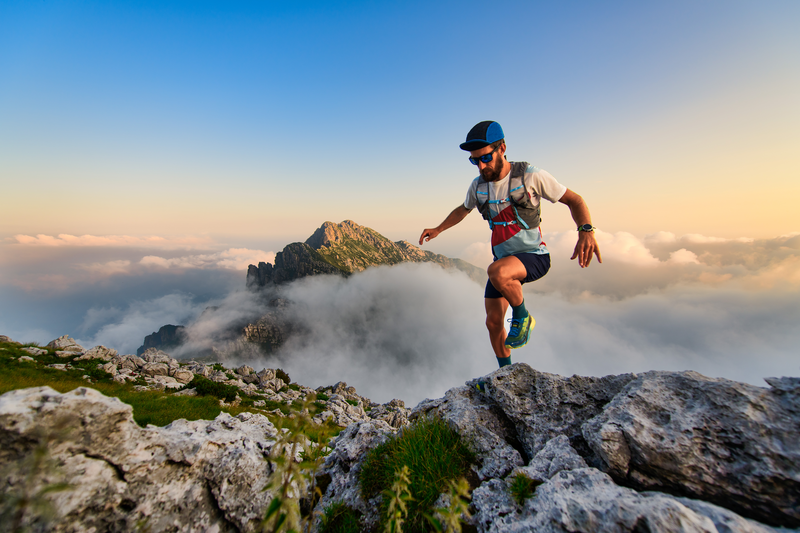
94% of researchers rate our articles as excellent or good
Learn more about the work of our research integrity team to safeguard the quality of each article we publish.
Find out more
ORIGINAL RESEARCH article
Front. Water , 27 February 2024
Sec. Water and Human Systems
Volume 6 - 2024 | https://doi.org/10.3389/frwa.2024.1253921
Water, energy, and food and their interactions (commonly referred to as the WEF nexus) are critical pillars to resolving the intractable global challenges such as poverty, hunger, malnutrition, poor sanitation, climate, and health crises. The nexus approach, practices, and innovations at the household level are critical determinants of whether resource use efficiency, co-benefits, basic rights to water and food, and sustainability governance are attained. In particular, smart WEF innovations can contribute to the current generations' economic, social, and environmental needs without compromising the needs of the future generation. The study aimed to identify smart innovations, practices, and factors influencing their adoption to inform policy and decision-making processes. The study intends to support scaling up the adoption of innovations and practices that enhance sustainability and resource security in support of the sustainable development goals (SDGs). Semi-structured interviews and key informant interviews (KII) supplemented with observational checklists were used to identify the WEF nexus smart technologies, innovations, and practices in Vhembe District Municipality, Limpopo Province, South Africa. Data were collected from a sample size of 128 households in the study area. Our findings revealed synergistic smart innovation practices across WEF resource use and management practices. Though indigenous knowledge (IK) practices were widely evident in the study area, non-existent WEF smart knowledge support systems existed in the study area. Indigenous knowledge practices were the most elicited innovation by 99.2% of households, suggesting it is critical to advancing WEF smart innovations and practices and needs to be integrated into any policy and governance interventions. A proportion of households recycle water (27%), whilst 53% use untreated water. Furthermore, the knowledge systems on smart WEF innovations were fragmented despite their potential to synergize sustainability objectives. Exploring innovation platforms (IPs) as vehicles for dissemination, innovation, and extension and advisory service delivery, as well as validation of Indigenous Knowledge Systems (IKS), has the potential to contribute to the diffusion, uptake, and scaling of existing innovation and practices with significant spill-over effects on WEF resource security and sustainability outcomes both at local and extra local scales.
The Water-Energy-Food nexus, hereinafter referred to as WEF, focuses on the interrelationships and conflicts among system components, notably supply and demand dynamics in energy, water, and food, as well as the environmental footprints of these relationships (Rasul and Sharma, 2016; Zhang and Vesselinov, 2017; Mpandeli et al., 2018; Vahabzadeh et al., 2023). Simpson and Jewitt (2019a,b) express various WEF interaction typologies as “water for food and food for water, energy for water and water for energy, and food for energy and energy for food.” In a nutshell, the nexus approach advances the conceptual and analytical approaches for framing interactable relations between WEF resources (Harwood, 2018). As an analytical framework, the WEF nexus is critical to addressing global challenges such as increasing populations and risk of malnutrition, biodiversity loss, and climate change crises. In particular, it is critical in mitigating the adverse impacts of climate change on crop yield and productivity as well as the nutritional value of edible plant-based diets (FAO, 20211). WEF thinking is largely informed by the need to address (a) growing resource scarcities, (b) resource supply crises, and (c) failures of silo WEF governance strategies (Cash and Swatuk, 2017). In essence, integrated approaches such as the WEF nexus have the potential to support a wide range of decision- and policymaking such as resource planning and coordination while offsetting potential negative externalities (Albrecht et al., 2018).
Implementing WEF nexus innovations and practices, where two or the three components of the nexus are integrated as inputs to each other, not only enhances resource efficiency but also expands the available natural resource base with synergetic contribution to the sustainability and security of the Water, Energy, and Food sub-sectors (Halalsheh et al., 2018). A system analysis of WEF in South Asia (Putra et al., 2020) also provided evidence for considering the WEF security nexus as an integrated system rather than just a combination of three different sectors or securities. The utility of the nexus further goes beyond the three WEF sub-sectors. For example, Fernández-Ríos et al. (2021) applied index-based approaches to link nutrient flows to health outcomes, while Janssen et al. (2020) integrated the WEF nexus with land resource constraint in the identification of innovations for sustainable adaptation and development under changing climate conditions.
The surge in urbanization and population growth rates with multiplier pathways on WEF resource securities, demand, and extraction in particular increases the urgency toward coordinated efforts that minimize conflicts while maximizing synergies among WEF sub-systems (Sarkar et al., 2020). The WEF nexus is accordingly highlighted as critical to achieving the Sustainable Development Goals (SDGs), African Union Agenda 2063, as well as national goals envisioned in various planning blueprints such as the Republic of South Africa's National Development Vision 2030 (Biggs et al., 2015; Butler et al., 2016; Rasul and Sharma, 2016; Mpandeli et al., 2018; Nightingale et al., 2020; Naidoo et al., 2021). Mitigating the negative footprints requires practices and innovations that reduce the environmental footprint and equally reduce cost, social equity, or generally efficiency measures collectively referred to as smart WEF innovations. For instance, direct resource consumption in the food sub-system, indirect operations in the energy sub-system, adverse environmental footprints have been established (Li et al., 2023).
Innovation is an idea, good, service, process, or procedure considered to be novel in solving a given problem or exploiting an opportunity (Nair et al., 2016). Innovation covers a broad spectrum of dimensions across technological, institutional, behavioral changes, and responsive social, cultural, and indigenous systems, which in turn influence the effectiveness of smart technologies (Steffen et al., 2020). Innovations thus encompass changes in processes, practices, structures, and institutions at the individual, organizational, and technological levels, including at various spatial levels (Otto et al., 2020; Leventon, 2021). It is the embodiment, combination, or synthesis of knowledge in its original form which is relevant and valued. WEF innovation and practices are thus vital in addressing the intractable challenges of poverty, human wellbeing, and sustainable development (Dennehy et al., 2021). The uptake of innovations is one of the strategies employed by economic agents to adjust to shocks such as climate change and weather variability (Zilberman et al., 2012; Williams et al., 2017).
WEF nexus innovations and practices across one or more of the WEF nexus input-output interactions not only enhance resource efficiency but also expand the natural resource base with significant contributions to sustainability and security objectives in WEF discourse (Halalsheh et al., 2018). This study adopted the definition of smart innovation as the ability to create new opportunities for end users, fostering ideas and their operationalization to increase the efficiency, survival, and thriving of individuals and communities in the face of global and environmental changes (Kumar, 2023). Smart innovations in this regard refer to ideas or practices, new or existing, that address WEF security risks (availability, cost, stability, and access to WEF resources, as well as reduction in environmental footprints). In a nutshell, smart innovations have the potential or actual capability to contribute to the current generations' economic, social, and environmental objectives without compromising the needs of the future generation, hence the attainment of SDGs.
Renewable energy, improving energy efficiency, conservation agriculture, water recycling, and wastewater reuse are just a few examples of nexus practices that transcend the three WEF resources (Halalsheh et al., 2018). Such technological options and solutions are being implemented in South Africa, though to varying degrees, and contribute to efficiency objectives (Mabhaudhi et al., 2018). However, these innovations need to be expanded to meet the goals of the nexus-relevant SDGs and the Paris Climate Summit of 2015. In the energy sector, smart energy systems focus on entire energy systems, including infrastructure designs and operation strategies that result in to the most effective and least-cost solutions through sub-sector integration (Østergaard et al., 2019). Such an integrated and holistic approach diversifies the energy mix (electricity sources and various uses such as heating and cooling in the industry, buildings, and transportation) and facilitates the identification of more feasible renewable and sustainable energy options (The World Bank, 2021).
The assessment of WEF nexus innovations and practices implementation at the household level can be considered through its link to WEF environmental securities and livelihoods of the people (Biggs et al., 2015), hence the need to integrate both internal and external factors influencing adoption and use at scale and household level. Such an attempt has the potential for mapping and thereafter scaling up, scaling out, and scaling deep interventions that address wicked sustainability and environmental governance challenges at the household level (Riddell and Moore, 2015). The study thus attempts to narrow the knowledge gaps through mapping practices and innovations, barriers, and enablers, and informing local and extra-local policy as well as decision-making processes on the existence of promising nexus innovations and practices. This paper extends the solution space on the wicked sustainability and resource WEF security challenges. Due to biophysical and social vulnerability and the semi-arid climate, the Nzhelele and Luvuvhu river catchments provide a rich typology of socio-economic context that captures intractable WEF complexity and contexts in sustainability transitions. The study did not, however, consider the full spectrum of WEF resources and security lenses. The article is organized into several sections. Section 2 discusses the materials and methods, Section 3 provides the findings, while Section 4 provides a discussion of the results. Section 5 provides the concluding remarks and directions for future research.
The study was conducted in Vhembe District Municipality (VDM), which is one of the five districts of the Limpopo Province of South Africa. The VDM is the country's northernmost district and shares its northern border with the Beitbridge district in Matabeleland South, Zimbabwe, and on the east with Gaza Province in Mozambique. It is a Category C Municipality, established in the year 2000 in terms of the Local Government Municipal Structures Act No. 117 of 1998. The district covers 27,969,148 km2 of land with a total population of 1,393,949, according to Stats SA, 2016 Community Survey. The district is divided into four local municipalities: Makhado, Thulamela, Musina, and Collins Chabane, which are category B municipalities. The study area is predominantly rural settlements with low-income, fast-growing peri-urban centers and small-scale farmers in the Nzhelele and Luvuvhu river catchment areas. The specific villages where data were collected are Siloam, Khalavha, and Phadzima villages in the Nzhelele River catchment and Sambandou, Tshakhuma, and Maluvuwe villages in the Luvuvhu River catchment (Figure 1).
The scope of the study involved identifying and quantifying the WEF nexus smart technologies, innovations, and practices in the study area. In this case, the use of the concept of “technologies” is inclusive of innovations and practice. Co-creation of solutions with household participants was the underpinning principle. The main output from this activity is a refined list of promising WEF nexus technologies relevant to the Nzhelele and Luvuvhu river catchment areas from the co-created typology of promising technologies. Noteworthy, the study is limited to identifying and recommending these promising technologies and not implementation thereof.
Resource insecurities and climate change are some of the existential risks faced by humanity. Since risk is one reason for innovation in an organization and social system (Nair et al., 2016), fast and frugal heuristic logic (Hafenbrädl et al., 2016) in sampling and sample size determination was adopted. Where evidence suggests risk disposition consensus, fast and frugal heuristics logic is normally adopted by selecting few representative cases as it does not compromise accuracy in the analysis of the obtained data (Hafenbrädl et al., 2016; Volenzo and Odiyo, 2020). Noteworthy, the sample size of 200 households was large enough to allow the generalization and exploration of risk from innovation lenses.
Due to security advisories, semi-structured questionnaires were only administered to volunteering households among the randomly included households at a central location. The team adhered to a schedule agreed upon by the local administration and selected household representatives. Only those households who were available as scheduled (128 out of a possible 200) were interviewed. The information from household surveys was triangulated through key informant interviews (KII) and focus group discussions (FGDs). The development of the semi-structured questionnaire (Supplementary Appendix 1) was developed based on a mixed research approach. This involved gathering both quantitative data, including aspects like biogas digesters, gas/Liquefied Petroleum Gas (LPG) usage, solar photovoltaic (PV), wind energy, and other indigenous innovations and practices and qualitative data. The qualitative data encompassed information on household typology, household water uses, energy uses, farming systems, typology of food security and food sufficiency, water, energy, agriculture and food technological innovations and practices. Additionally, it covered details about access to and use of water, energy, and food (WEF) resources, challenges faced, and reasons for choosing a particular water, energy, or food technology innovation. The collection of quantitative data is necessary in order to test and objectively examine relationships (Mavodza, 2022).
The study employed Fisher's (1983) formula in the determination of sample size as given by Equation (1). Only those households who were available as scheduled (128 out of a possible 200 households from the sampling frame initially selected through random sampling) were interviewed. WEF resource insecurities and climate change are some of the risks experienced by households in Vhembe District Municipality. The pilot study by the research team revealed that only about 40% of the households were involved in agriculture. Further, the households implemented similar WEF innovations and practices. Hence, we adopted fast and frugal heuristics logic (Hafenbrädl et al., 2016). In risk studies, the adoption of fast and frugal heuristics logic allows for sampling and generalization from few representatives as it does not compromise accuracy in the analysis of the obtained data (Hafenbrädl et al., 2016; Volenzo and Odiyo, 2020).
where n, desired sample size; Z, standard normal deviate at 95% level of confidence = 1.96; P, proportion of the target population estimated to have the characteristic under investigation (40% or 0.4) to maximize sample size (precision); q, proportion of the target population without the characteristic (1-p = 60% or 0.6); d, level of precision corresponding to the statistical significance level of 0.05 or 5%.
Substituting for the values
n = Z2 ((p q)/d2) = 1.962 (0.5*0.5)/(0.05)2 = 3.8416 (0.25)/0.0025 = 188.16, hence 189 households.
Taking cognizance of non-response, we targeted 200 households but only 128 were available due to the aforementioned security challenges. In the context of diagnostic risk assessment, we considered a sample size of 128 households large enough to allow for generalization and exploration of risk from innovation lenses adequate enough, given that the social and economic structure and farming systems (mostly home gardens) are more homogeneous. Further, information from household surveys was triangulated through key informant interviews (KII) and focus group discussions (FGDs). The semi-structured questionnaire was developed based on a mixed research approach, i.e., gathering quantitative and qualitative data. Collecting quantitative data is necessary to test and objectively examine relationships (Mavodza, 2022). The distribution of households interviewed is given in Table 1.
A pilot study was the initial part of the research that sought to test the data instruments and their effectiveness in the research field. As such, the pilot study employed the research instruments on a selected sample of participants. Pilot surveys were conducted at Siloam, Khalavha, Phadzima, Sambandou, and Malavuwe villages. The pilot assisted in refining the questionnaire instrument and ensured there was no ambiguity in the questions. It sought to establish the time taken in each interview, the clarity of the question asked, and how the respondents responded to the question. Once the researchers were satisfied, the data collection process then commenced.
After this, 128 questionnaires were administered for the main study. Final survey data were collected in Siloam, Khalavha, Phadzima, Sambandou, Malavuwe, and Tshakhuma. Although six villages were initially purposed for the study, one village could not be accessed due to the community leaders' unwillingness to participate. This unwillingness was triggered by acute water scarcity and their insufficient municipal water supply. Questionnaires were further administered in Siloam, Khalavha, Phadzima, Sambandou, and Malavuwe villages. This was supplemented with FGD and KII. Quantitative story telling (QST) (Saltelli and Giampietro, 2017) was applied to check the coherence of community narratives against mainstream scientific narratives, synthesizing and clarifying issues under investigation (Di Felice et al., 2021).
Descriptive statistics were used to analyze data on innovations and practices identified by the respondents. The findings were triangulated through key informant and focus group discussion findings. The data were analyzed using the IBMR SPSSR Statistics version 27 statistical package. Results are presented as tables and charts. Qualitative data were analyzed using thematic analysis. Thematic data analysis involved identifying, analyzing, and reporting patterns (themes) within data (Braun and Clarke, 2006; Bryman, 2016). Data analysis using thematic analysis accords the researcher's flexibility and it fits different or multiple contexts. The study was able to compare its findings with the findings or conclusions drawn from other studies which were premised on the literature review from FGDs and KIIs.
The study interviewed 128 respondents from households with age profiles presented in Table 2. The classification of respondents by age groupings revealed that 29% were above 60 years of age, while cumulatively, only 42.2% were below 51 years of age. Noteworthily, the sample had a fair representation of all age categories.
The education levels of respondents were recorded to determine their literacy level. At least 46% of the respondents had secondary education, 14% had technical and vocational education and training (TVET)/college qualifications, and about 3% had a university education. Noteworthily, the literacy level could be considered high given that only 10% of respondents had no schooling. With regard to employment status, at least 49% of the respondents had some form of employment, and 39% were unemployed. Of the employed respondents, 10% had formal employment, whereas 36% were informally employed. The income levels, including remittances, are summarized in Table 3, indicating the majority of respondents (64.1%) had a total household income of R4,000 or less.
Awareness or knowledge is an integral part of the diffusion and adoption process (Rogers, 2004), with cognitive factors being essential for linking technical nexus analyses to actual decision-making and policy processes (Balick et al., 2016). Accordingly, the study assessed ownership of information and communications technology (ICT) resources (Table 4), access, and usage in the study area. The majority of respondents owned a radio (92.2%), cell phone (89.8%), and television (81.3%). Landlines and newspapers were the least reported communication tools, with 5.5% and 13.3%, respectively. Electronic media remains the most ubiquitous platform in the study area and has great significance in the dissemination of knowledge, especially WEF smart technologies.
Noticeably, the majority (91.9%) of the respondents owned hand hoes, which are the basic form of farm implements/machinery, with about 4% owning ox-drawn plows (Table 5). A small proportion owned a tractor and bakkie at 2.7% each. This could suggest that most farmers hire heavy farm machinery or cultivate small farms.
In South Africa, agriculture is the largest water user at 61% of total water use, followed by municipal use at 27% (including industrial and commercial users provided by municipal systems), with power generation, mining and bulk industrial use, livestock, and conservation and afforestation jointly making up the remaining 12% (DWA, 2013). The level of assurance at which agricultural water is supplied is lower than that of the other sectors (90%). Respondents had diverse community water resource assets, with about 23 and 27% obtaining water from rainfall and river sources, respectively. About 29% of the respondents used communal municipal taps, with about 11% using boreholes (Table 6). Moreover, the least source of water source were community-owned waterpoints and springs at about 1 and 10%, respectively. The preeminence of communal/collective water assets could significantly impact efficiency measures and the adoption of water-smart technologies at the household level.
South Africa is a water-scarce country with water resources critically impacting economic, political, and social welfare. The majority (about 38%) of the households had access to water resources within the household, 34% within 1 km, and about 29% beyond 1 km of the household. However, the majority of the households either received water less than three times a month or never received it at all. The main uses of water at the household level are given in Table 7. Other than consumption (potable use) sources, about 70% of the respondents used water for irrigation, followed by about 43% for livestock keeping. The multiple uses of water at the household level across the WEF nexus aptly signify the importance of an integrated approach to their management, especially concerning smart innovation interventions.
There were several practices and innovations around water use in the study area. Most of the innovations cut across the WEF spectrum, suggesting that the silo approach to addressing is untenable at the household level. Only 27% of the households (Table 8) recycled water. The water treatment practices and innovations in the study area unpack several lessons. Noticeably, most households (18%) used Jik as the water treatment method compared to 15% who used boiling. The safety and health risk of such a method is uncertain. About 53% of the households did not treat the water. Only 3% used other modern methods such as filtration chambers.
Reducing both the impacts and drivers of climate change is premised on major resource use and reuse shifts, especially the limited water resources. Even though often overlooked, water resources are an essential part of the solution to climate change. A significant amount of water is required to generate energy. Also, direct resource consumption in the food sub-system and indirect operations in the energy sub-system cause environmental impacts (Li et al., 2023). Reducing the environmental footprint on water could be achieved through many means. These include developing alternative water sources, reducing losses, use of technology, smart metering, and recycling. About 71% of the households did not recycle water in any way. Most of the water recycling was on vegetable gardening at 14% and household sanitation at about 3%. Generally, there was low use of water-smart technologies at the community/household level, with harvesting and use of rainwater for irrigation and household use being the most prominent approach at about 32%.
Water smart technologies either exploit alternative sources, reduce energy use in its exploitation, or reduce cost. The most identified factor was reliability (26%), followed by finance (21%), then cost saving (17%). These factors already influence or are likely to influence the adoption of water smart technologies in Table 8.
Drip irrigation is one of the smart water innovations with the potential to substantially reduce the amount of water applied and losses through evapotranspiration (Ranjan et al., 2022). However, the low practice of innovation in the study area was attributed to high initial costs and lack of knowledge by the respondents. Rainwater harvesting and its use for irrigation was one of the major innovations with the potential to reduce the environmental footprints of agricultural production systems on the environment. However, quantification of the environmental footprints is beyond the scope of this paper.
There was a myriad of energy sources identified in the study area. About 53% of the respondents used electricity as the main energy source, while 3.1% had renewable resources (solar) as the energy mix in the household (Table 9). Paraffin and LPG, with 2.6% each, were the least used energy sources. Alternative cost-effective energy sources not only address climate change but also contribute to other multiple objectives on sustainability and human wellbeing (Bhave et al., 2018; Karan et al., 2018). Though other innovations, such as sun drying, are common in the community, this section mainly focuses on electricity and solar energy and their uses. Increasing the use of biomass also emerged as an economic innovation in the context of load shedding and escalating electricity costs. Noticeably, solar energy is used for heating and pumping water for irrigation. Most households stated they were unaware of solar energy as an alternative. Some of the challenges they faced in using solar energy include the theft of solar panels and the high initial cost. However, those using it could identify its utility in terms of reliable energy supply, especially for lighting and powering TVs and radios. Most FGDs suggested solar energy as one of the potential solutions to frequent load shedding in the community. The main uses for solar energy in the community were lighting, TV, and accessories. However, most households stated that there was a lack of knowledge on the required capacity to meet their needs.
The energy mix at the household level reflects the policy and economic lock-ins yet is an eye opener to the renewable energy solutions that could play a critical role in addressing social, economic, and environmental challenges at the household and national levels. Solar energy could mitigate the current biting load shedding (outages) experienced at the household level and contribute to overall energy security. Table 10 summarizes the main energy uses at the household level. The major uses of energy are for cooking (95.3%), lighting (86.7%), and energy uses for appliances (84.4%). Moderate energy use for refrigeration was 63.3%. About 72% of the respondents reported the use of energy for heating. Notably, the preeminence of non-renewable energy sources in households reflects the policy and economic lock-ins on energy resources and use at the national level.
Food systems have been identified as critical drivers in the transition to carbon-neutral growth. Agriculture, forestry, and land use (AFOLU), which currently accounts for at least 30% of global emissions, have emerged as a focal sector for intervention. Climate smart agriculture (CSA) is seen as a significant approach toward enhancing adaptation-resilience synergies and mitigation co-benefits [UNFCCC (United Nations Framework Convention on Climate Change), 2021]. CSA presupposes integrating climate change into the planning and implementation of sustainable agriculture practices. Since emission intensities are agricultural mitigation indicators [UNFCCC (United Nations Framework Convention on Climate Change), 2016], it is adopted as a strategic vision in integrating emissions from agricultural activities into the climate action agenda (UNEP, 2019; Hohne et al., 2020). The adopted ecoefficiency, hence, the CSA approach biases innovation toward greenhouse gas emission mitigation. Climate smart agricultural practices include crop-livestock integration, sustainable intensification, precision agriculture, and conservation agriculture (FAO, 2016). As one of the integrated approaches to managing landscapes such as cropland, livestock, forests, and fisheries and accounting for interlinked challenges of food security and climate change (The World Bank, 2021), CSA aims to simultaneously achieve increased productivity, enhanced resilience, and reduced emissions (FAO, 2010, 2016; IPCC, 2018, 2019; Aguilera et al., 2021; Bonner and Biglan, 2021).
Table 11 provides the main uses of solar energy at the household level. According to the KII and FGDs, initial cost remained the biggest barrier to the adoption of this technology. Households using solar alternatives cited load shedding and unreliability of the national grid system as the main incentive for adopting the solar alternatives. Solar energy was mainly used for house lighting, with some used for powering appliances such as televisions (TV).
Table 12 summarizes the various smart agricultural practices mapped in the area. One of the most prevalent agriculture smart innovations and practices was mulching, used by about 49%. About 52% of those studied practiced conservation agriculture, broadly defined as an agriculture resource use system that minimizes external resource inputs such as inorganic fertilizers and/or minimizes soil moisture loss by minimizing soil disturbance. Other practices were to deliberately allow the field to rest for a season before replanting (24%) and growing agroforestry (combinations of trees and/or legumes with livestock in an agricultural system which has the potential to mitigate Green House Gas (GHG) emission, as well as capture and store carbon from the atmosphere) species at about (3%). The rate of using livestock manure, practicing zero tillage, and growing crops season after season without a fallow period between crops (relay cropping) was about 7% each. Zero/minimum tillage as a form of conservation agriculture was mentioned by about 7% of the households. Drip irrigation, multipurpose agroforestry, and compositing were practiced by 11%, 4%, and 3% of households in the study area, respectively.
The main food preservation innovations and practices in the study area are summarized in Table 13. The most common preservation method was sun drying (about 36%), followed by refrigeration/freezing (28.1%). However, 25% of the respondents did not use any kind of preservation methods. Fumigation, a chemical preservation method, was mentioned by 5.5%. The use of ash, an indigenous method against pest infestation for cereals/pulses, was mentioned by about 3% of the respondents.
Further, during FGDs, the community captured other methods such as smoking, salting, and fermentation for food products such as milk. Food preservation methods are closely linked to existing infrastructure, knowledge systems, cultural disposition, and household socioeconomic status. Sun drying and freezing have practical implications on energy consumption and its impact chain.
Food storage methods and infrastructure used at the household and community levels are indicated in Table 14. Fridges were the most common food storage infrastructure at the household level at about 26% of the respondents, followed by various types of bags at about 22%. About 9% of the households stored their food in a spare room, with about 8% storing in drums. Food storage has implications for energy use and offers an important entry point for WEF security interventions. Given fridges are the common storage infrastructure, all actors need to promote more energy-efficient fridge models at the household level.
The following are some of the indigenous innovations that were identified in the community.
• Winter-summer rotational cropping systems between vegetables and cereals (irrigated vegetables during winter, such as mustard spinach, to take advantage of ambient moisture, and plant cereals during summer, such as African millet, to take advantage of relatively high rainfall amounts received).
• Locally adapted breeds, including indigenous cattle, free-range piggery, and poultry, reduce the need for external inputs and/or the number of external inputs required.
• Low external input agriculture whereby there is minimal reliance on externally acquired inputs (inorganic fertilizers) but increased reliance on internal inputs such as manure and compositing, among other nutrient recycling practices in the farming system. At the household level, these practices reduce costs (increase profitability), reduce emission footprint, and increase resilience, hence contributing to objectives envisaged under climate-smart agriculture.
• Conservation agriculture, including fallow strips and growing of agroforestry species.
• Food preservation sun drying, as one of the ubiquitous technologies used in vegetable drying and preservation, reduces the need for refrigeration.
• Dryland agriculture—including the planting of short-maturing crop varieties and drought-tolerant crops such as sorghums.
• Water storage—rainwater harvesting is practiced by the majority of households, reducing the pressure on conventional water extraction sources such as boreholes and tap water. This is mainly an adaptation, hence innovation to governance challenges on communal water supply.
• Non-conventional foods such as Mopani worms and termites increase the resilience of communities to droughts/shocks as well as address environmental footprints along the value chain as compared to conventional food systems that require elaborate transport, storage, and refrigeration systems that increase various environmental footprints.
Greenhouse gas (GHG) emission footprint is a major indicator of innovation smartness and, in turn, depends on the number of resources consumed and the pollution load into the environment. Energy efficiency (and/or wastage) takes prominence in the GHG emission footprint as it is the major driver in resource transformation. It was noted that the smartness of identified innovations is also related to water and energy saving. Smart agricultural practices identified in the study area include avocado orchards which are some of the most common fruit trees and which perform multipurpose functions such as food, shade, and windbreaks. Based on the existing literature, the criteria for smartness in our study thus includes the perceived chain impact on the environmental footprints of identified WEF innovations and practices. The smartness is attributed to several impact pathways, as outlined.
• Minimized energy intensities in food production and preservation using organic practices such as manuring and sun drying. The use of solar pumps and panels for various uses such as lighting, cooking, charging accessories, and pumping household water reduces embedded energy levels and emission footprints.
• Groundwater is increasingly emerging as an adaptation measure yet increasing the risk of over-extraction and serious ecological imbalances. Reducing the environmental footprint of water extraction was evident through roof harvesting by the majority of households.
• Increasing crop and water productivity through irrigation during winter while depending on rainfed agriculture to grow cereals during summer reduces evapotranspiration losses and the ecological footprint on water resources (reduces the need for irrigation, hence groundwater extraction of water resources for irrigation).
• Using local seed varieties and/or short-maturing cereals crops such as sorghum reduces the water footprint.
• Diversification in agricultural enterprises, including mixed farming, allows for the exchange of nutrients to increase synergies and build the resilience of the household to weather shocks and market price risks, especially following the disruption in the fertilizer value chain following the Ukraine-Russia War.
• Recycling of nutrients between livestock (poultry and cattle) and crops reduces the need for external inputs, hence contributing to sustainable agricultural value chains and food systems (reducing emissions of GHG that would otherwise be generated through the transport of inorganic inputs and as well as embedded energy in inorganic inputs).
• Regenerative agricultural practices or all farming practices that positively impact topsoil regeneration, enhance biodiversity, improve the water cycle, enhance ecosystem services, and, in the process, increase resilience to climate change and strengthen the health and vitality of farms, i.e., conservation agriculture (CA).
• Multiple-purpose fruit trees reduce the space needed for cultivation and substantially reduce the environmental footprint on water resources as well as emissions.
The uptake of any technology is influenced by both the technology characteristics as well as social economic profiles of individuals and communities (Rogers, 2004). Such changes in the environment and stimuli or signals by a suite of agents and spill-overs are drivers of innovation in a social system (Nair et al., 2016). In most cases, extra local institutions influence carbon transition visions or lock-ins (Sovacool et al., 2020). Lock-ins, in turn, inform and justify the technological, institutional, policy, and behavioral choices (Seto et al., 2016; Buschmann and Oels, 2019). The effect of lock-ins is thus multifaceted. First, it could undermine innovation and bias policy choice efforts on generic yet locally irrelevant alternatives. This section identifies various informational (communicative), organizational, and individual factors that mediate smart innovations and practices.
Renewable energy and energy efficiencies are among the most critical determinants of the economy and decarbonization initiatives. Table 15 summarizes the factors influencing the uptake of smart energy innovations and practices. Knowledge (about 62%), reliability (53%), and cost (45%) as well as finance (48%) were major influencing factors. A 28% value for the environment suggests public awareness may be critical. Social networks, family spill-overs, and peers together account for about 46% and hence could inform the exploration of IPs. The factors influencing the uptake of energy smart technologies cut across the informational, regulatory, and organizational spectrum of WEF governance.
Smart agriculture technologies such as composting and the use of manures increase productivity and income and also reduce greenhouse gas emissions. Table 16 summarizes the factors influencing smart agriculture practices. Informational interventions, at about 63%, take preeminence in influencing the adoption of smart agriculture innovations and practices. The cost and financing are not mentioned, probably because of the small vegetable gardens that dominate the landscape.
Only about 16% (20 in number) of the respondents were cognitive of the factors influencing smart water technologies. The reasons for the dismal cognition include lack of information, predominant subsidized water schemes as well as poor extension services. This is despite water being of great concern in the community. Of those cognizant of water smart technologies, about 33% and 25% cited social networks and value for the environment as the most critical and leading factors influencing adoption. The results suggest innovation platforms (IPs) as a potential arena for addressing low cognition (Table 17).
The role of institutionally mediated human agency in facilitating or constraining WEF outcomes is often ignored (Villamayor-tomas et al., 2015). For example, the perception of effective transitions largely revolves around risk perception and incentives (Cash and Swatuk, 2017; Smith and Mayer, 2018). We posit that cognition plays an acritical role in human agency cognition plays an important role in innovation and diffusion processes. Due to the low cognition level of options on smart water and smart agriculture pathways, the team further elicited specific awareness of various sector technological options. Table 18 summarizes the awareness level of various water smart options. Indigenous knowledge (IK) was the most elicited innovation by 99.2%, suggesting it is critical to advancing WEF smart innovations and practices and needs to be integrated into any policy and governance interventions. Water cycling and water loss reduction options were cited by about 30% and 7% of the respondents, respectively. The observation affirms low awareness of water smart technologies in the study area. Only about 9% and about 3% of the respondents, respectively, were aware of water smart practices through CA and sustainable intensification (i.e., hydroponics).
Table 19 summarizes the awareness level of various agriculture smart options. As in water smart technologies, IK was the most elicited innovation by 100% of the respondents, suggesting it is critical to advancing WEF smart innovations and practices. Water innovations toward smart agriculture (water cycling and water loss reduction and harvesting) options were cited by about 20% of respondents collectively. The observation affirms low awareness of agriculture smart technologies in the study area. Less than 1 and 2% of the respondents, respectively, were aware of agriculture smart practices through CA and sustainable intensification (i.e., hydroponics).
Innovations and practices play a critical role in addressing the water, energy, and food challenges. The introduction of new and appropriate innovations and practices can improve resource efficiency in the water, energy, and food sectors and contribute to their security and sustainability. For instance, introducing renewable energy and improving energy efficiency, conservation agriculture, water recycling, and wastewater reuse are just a few examples of such driving forces between the nexus of the three components and technology (Holmatov et al., 2023). Indigenous knowledge could play a critical role as a smart WEF innovation and practice alternative, hence reducing energy intensity and pollutant load into the environment.
Narratives encompass divergent viewpoints such as opinions and their interpretations, expert and lay judgement, expert knowledge, as well as various social-cultural contexts and epistemologies underlying them (Mewes et al., 2016). Quantitative story telling (QST) has emerged as a multiscale nexus assessment and biophysically grounded method for assessing the co-production of knowledge (Saltelli and Giampietro, 2017), as well as checking the coherence of already existing or new narratives (Di Felice et al., 2021). QST inspects the relationships between the narratives used to frame sustainability issues and the evidence on those issues. Though QST is a nexus assessment method that uses both numbers and narratives, it may not be instrumental in directly inducing policy change, but it is a valuable means to initiating discussions on innovations outside the dominant imaginaries. From the current study, we have identified narratives on conventional as well as indigenous knowledge innovations and practices with the potential to synergize sustainability outcomes in WEF resource use. These include livelihood diversification practices, such as multipurpose orchard patterns (food forests), indigenous seasonal cropping patterns that mitigate water withdrawals for irrigation, and water use innovations, including rainwater harvesting and use of drought-tolerant crops such as sorghums, millets, and root crops. The various narratives underpinning them at the household and community level qualify them as innovations with the potential to address WEF resource insecurities.
Since GHG footprints provide evidence of environmental footprints and thus the smartness of a given WEF innovation and practice, their role in WEF security and sustainability transitions cannot be overemphasized. Sound selection of technology and proper policies across the nexus could ensure holistic water, energy, and food security. Policymakers should be mindful of the fact that single-sector efficiency may sometimes lead to a negative impact in the other two sectors, as in the case of water subsides in irrigation and the promotion of the use of solar pumps, which both led to over-abstraction of groundwater as in the case of India (Kimmich, 2013). Save for widely practiced IK, few smart innovations as well as non-existent WEF smart knowledge innovation systems in the study area undermine sustainability governance and transitions in the study area. The fragmented knowledge systems on smart WEF innovations in the study area further undermine collective action pathways to synergize sustainability objectives.
Targeting incentives toward nexus smart investment, such as renewable energy and technologies that decouple energy and water intensification in food production, as well as removing barriers, are key to mainstreaming nexus in development planning and implementation of the WEF policy framework (Rasul, 2016). The effectiveness is increased when paired with capacity enhancement, learning, and innovative approaches to support farmers' decision-making (Westermann et al., 2015). Addressing fragmentation in the knowledge and innovation systems is one of the priority areas for transformation toward sustainable governance, especially in food systems (Dinesh et al., 2021). One of the solutions exists in the use of innovation platforms. Climate smart villages, akin to IPs, are highly effective mechanisms for bringing climate-smart options to scale and forging links from the local level to national policy processes (Aggarwal et al., 2018). Implicitly, social learning plays a critical role in policy experimentation and learning. Presumption of understanding and, therefore, framing cycles in discourse such as sustainability transitions on social and ecological aspects, especially during the climate change scenarios (Scholz and Methner, 2020 and Epstein et al., 2015) is assumed to be social learning. However, the sufficient and necessary conditions in social learning (Reed et al., 2010) are beyond the scope of this paper.
The uptake of smart WEF innovation has a critical role in sustainability and environmental governance. However, they are constrained by governance and institutional and household social-economic dynamics. These include cultural, economic, and historical lock-ins. Creating synergy at the implementation level, the household is thus critical if envisaged sustainability and resource security objectives in WEF framing are to be optimized at the local level and hopefully at a regional to global scale. Indigenous knowledge innovations and practices could play a critical role in innovation for resilience building around the WEF resources. Innovation platforms (IPs) are one such opportunity for scaling promising WEF innovations and practices. Innovation platforms could be a driver toward the legitimacy of technology and innovation (Klerkx et al., 2010), hence the critical integration of IKs practices in policy and social learning. Indeed, IPs could be instrumental in the validation of the ubiquitous IK smart innovations and practices in the study area, thus contributing to the environmental and sustainability governance agenda.
Understanding institutional human behavior interplay (Seto et al., 2016) is critical in overcoming lock-ins and fostering alternative innovative net zero carbon development pathways (Buschmann and Oels, 2019). Multistakeholder platforms and policy-making networks are key to effective up-scaling and are particularly critical. Therefore, cross-sector linkages and membership in community institutions become critical parts of the innovation ecosystem as they both mediate capabilities and accumulation of knowledge and diffusion of ideas (Aggarwal et al., 2018). WEF-focused IPs are thus recommended in scaling up the identified smart innovations in order to synergize and deepen the environmental, social, and economic benefits envisioned by WEF discourse. The nexus approach considers the interactions, synergies, and conflicts around WEF management can increase overall resource use efficiency and co-benefits, including the realization of basic rights to water and food.
The study identified smart WEF innovations and practices in Vhembe District Municipality, Limpopo Province, South Africa. There exist several innovations and practices across the WEF resource sectors, with IKS accounting for the majority of innovations and practices across the WEF ecosystem, yet these are not receiving the necessary attention. Mainstreaming IKS innovations and practices into the policy interventions could thus increase the suite of WEF smart innovations and practices and policy options for tackling the wicked sustainability challenges across scales. A life cycle assessment and social-technical assessment of particular innovations and practices are critical in quantifying actual environmental footprints and prioritization for scaling up and scaling out the identified practices and innovations. Though economic, social, and environmental criteria guide the smartness of WEF practices and innovations, their realization at the household level is lacking. The interconnectedness of potential impacts at the household level further illustrates the need for integrated planning and targeting of policy interventions and interventions if WEF synergies are to be optimized. The greater impact can be achieved through:
• “Scaling out,” which is about impacting greater numbers through replication and dissemination, increasing the number of people or communities impacted.
• Aligning institutions, policy, and regulations, especially the cultural and economic lock-ins and cognition factors that tend to constrain the “scaling up” of promising IKs, smart practices, and innovations. Integration of IK could be critical in this direction.
• Holistic consideration of the interaction and effect of informational, procedural, and organizational instruments in WEF innovation and transformational agenda.
• IPs could be cost-effective vehicles for addressing synergies, fragmentation, and validation of IKs. This could be tested through multistakeholder WEF-IP platforms in policy experimentation and social learning.
Smart WEF innovations potentially could make a significant contribution toward sustainability transitions and WEF resource securities amid accelerated climate change. The study has provided baseline data on existing smart WEF innovations and practices at the household level, Vhembe District Municipality. To advance the sustainability transition agenda and policy and social learning, a detailed life cycle and evaluation impact assessment are recommended. This task was beyond the scope of the study. Though innovations and practices are found to be working within the local context and are promising and can be taken to scale, policy experiments are likely to inform the formulation of responsive governance and institutional mechanisms for scaling the identified innovations and practices. WEF IPs across the smart innovation and practice options could provide the forum for the nurturing, validating, and scaling of such innovations.
The original contributions presented in the study are included in the article/Supplementary material, further inquiries can be directed to the corresponding author.
Ethical review and approval was not required for the study on human participants in accordance with the local legislation and institutional requirements. Written informed consent from the participants was not required to participate in this study in accordance with the national legislation and the institutional requirements.
KM: Conceptualization, Project administration, Writing – original draft, Methodology. EM: Investigation, Writing – review & editing. TM: Methodology, Project administration, Writing – review & editing. RM: Investigation, Methodology, Project administration, Writing – review & editing. TV: Data curation, Formal analysis, Investigation, Writing – review & editing. NM: Methodology, Resources, Writing – review & editing. KN: Funding acquisition, Investigation, Methodology, Project administration, Writing – review & editing.
This project was funded by the Water Research Commission. Project No. WRC C2020/2021-0039: integrating water-energy-food nexus innovations and practices into policy, governance, and institutional frameworks for sustainable development in Vhembe District, Limpopo Province, South Africa.
We thank the Nzhelele and Luvuvhu River catchment villages for participation in this project. We are grateful to the traditional leaders for permitting us to conduct the survey. Special thanks go to all respondents of this project.
EM was employed by Clovita Consulting Services. TM was employed by FANRPAN.
The remaining authors declare that the research was conducted in the absence of any commercial or financial relationships that could be construed as a potential conflict of interest.
All claims expressed in this article are solely those of the authors and do not necessarily represent those of their affiliated organizations, or those of the publisher, the editors and the reviewers. Any product that may be evaluated in this article, or claim that may be made by its manufacturer, is not guaranteed or endorsed by the publisher.
The Supplementary Material for this article can be found online at: https://www.frontiersin.org/articles/10.3389/frwa.2024.1253921/full#supplementary-material
Aggarwal, P. K., Jarvis, A., Campbell, B. M., Zougmoré, R. B., Khatri-chhetri, A., and Vermeulen, S. J. (2018). The climate-smart village approach: framework of an integrative strategy. Ecol. Soc. 23:14. doi: 10.5751/ES-09844-230114
Aguilera, E., Reyes-Palomo, C., Díaz-Gaona, C., Sanz-Cobena, A., Smith, P., García-Laureano, R., et al. (2021). Greenhouse gas emissions from Mediterranean agriculture: evidence of unbalanced research efforts and knowledge gaps. Glob. Environ. Change 69:102319. doi: 10.1016/j.gloenvcha.2021.102319
Albrecht, T. R., Crootof, A., and Scott, C. A. (2018). The water-energy-food nexus: a systematic review of methods for nexus assessment. Environ. Res. Lett. 13:043002. doi: 10.1088/1748-9326/aaa9c6
Balick, A., Weitz, P., Neary, J. T., Crow, T., Alkon, D. L., Sutherland, M., et al. (2016). Identifying poorly met demand: the impact of product beliefs on attribute importance. J. Market. Res. 11.
Bhave, A. G., Conway, D., Dessai, S., and Stainforth, D. (2018). Water resource planning under future climate and socioeconomic uncertainty in the Cauvery River Basin in Karnataka, India. Water. Res. Res. 54, 708–728. doi: 10.1002/2017WR020970
Biggs, E. M., Bruce, E., Boruff, B., Duncan, J. M. A., Horsley, J., Pauli, N., et al. (2015). Sustainable development and the water-energy-food nexus: a perspective on livelihoods. Environ. Sci. Policy 54, 389–397. doi: 10.1016/j.envsci.2015.08.002
Braun, V., and Clarke, V. (2006). Using thematic analysis in psychology. Qual. Res. Psychol. 2, 77–101. doi: 10.1191/1478088706qp063oa
Bonner, A. C., and Biglan, A. (2021). Rebooting behavioral science to reduce greenhouse gas emissions. Behav. Soc. Issues 30. doi: 10.1007/s42822-021-00058-y
Buschmann, P., and Oels, A. (2019). The overlooked role of discourse in breaking carbon lock-in: the case of the German energy transition. WIREs Clim. Change 10:e574. doi: 10.1002/wcc.574
Butler, J. R. A., Bohensky, E. L., Suadnya, W., Yanuartati, Y., Handayani, T., Habibi, P., et al. (2016). Scenario planning to leap-frog the sustainable development goals: an adaptation pathways approach. Clim. Risk Manag. 12, 83–99. doi: 10.1016/j.crm.2015.11.003
Cash, C., and Swatuk, L. A. (2017). Water, Energy, Food and People Across the Global South: ‘The Nexus' in an Era of Climate Change. Springer International Publishing.
Dennehy, D., Pappas, I. O., Wamba, S. F., and Michael, K. (2021). Guest editorial. Inform. Technol. People 34. doi: 10.1108/ITP-10-2021.100306-871
Di Felice, J. L., Cabello, V., Ripa, M., and Madrid-lopez, C. (2021). Quantitative storytelling: science, narratives, and uncertainty in nexus innovations. Sci. Technol. Hum. Values 1. doi: 10.1177/01622439211053819
Dinesh, D., Hegger, D. L., Klerkx, L., Vervoort, J., Campbell, B. M., Driessen, P. P. J., et al. (2021). Enacting theories of change for food systems transformation under climate change. Glob. Food Secur. 31:100583. doi: 10.1016/j.gfs.2021.100583
DWA (2013). National Water Resource Strategy, 2nd Edn. Available online at: https://www.scirp.org/reference/referencespapers?referenceid=2231817
Epstein, G., Pittman, J., Alexander, S. M., Berdej, S., Dyck, T., Kreitmair, U., et al. (2015). Institutional fit and the sustainability of social-ecological systems. Curr. Opin. Environ. Sustain. 14, 34–40. doi: 10.1016/j.cosust.2015.03.005
FAO (2010). “Climate-Smart” Agriculture: Policies, Practices and Financing for Food Security, Adaptation and Mitigation.
FAO (2016). The Agriculture sectors in the Intended Nationally Determined Contributions: Analysis (No. 62; Environment and Natural Resources). Rome: Italy.
Fernández-Ríos, A., Laso, J., Campos, C., Ruiz-Salmón, I., Hoehn, D., Cristóbal, J., et al. (2021). Towards a water-energy-food (WEF) nexus index: a review of nutrient profile models as a fundamental pillar of food and nutrition security. Sci. Total Environ. 789:147936. doi: 10.1016/j.scitotenv.2021.147936
Hafenbrädl, S., Haven, N., States, U., Waeger, D., and Marewski, J. N. (2016). Applied decision making with fast-and-frugal heuristics. J. Appl. Res. Mem. Cogn. 5, 215–231. doi: 10.1016/j.jarmac.2016.04.011
Halalsheh, M., Ouarda, T., and Al-Jayousi, O. (2018). The Water-Energy-Food Nexus in the Arab Region. Nexus Technology and Innovation Case Studies; Policy Brief No. 6. Available online at: https://uploads.water-energy-food.org/legacy/policy_briefs_6_english.pdf
Harwood, S. A. (2018). In search of a (WEF) nexus approach. Environ. Sci. Policy 83, 79–85. doi: 10.1016/j.envsci.2018.01.020
Hohne, N., Elzen, M. D., Rogelj, J., Metz, B., Fransen, T., Kuramochi, T., et al. (2020). Emissions: world has four times the work or one third of the time. Nat. Clim. Chang. 579, 25–28. doi: 10.1038/d41586-020-00571-x
Holmatov, B., Lautze, J., and Uhlenbrook, S. (2023). The nexus across water, energy and food (WEF): learning from research, building on evidence, strengthening practice. Nat. Resour. Forum 47, 817–841. doi: 10.1111/1477-8947.12352
IPCC (2018). Global Warming of 1.5dc. An IPCC Special Report on the Impacts of Global Warming of 1.5dc Above Preindustrial Levels and Related Global Greenhouse Gas Emission Pathways in the Context of Strengthening Global Response to the Threat of Climate Change in Sustainability (Switzerland).
IPCC (2019). Climate Change and Land: An IPCC Special Report on Climate Change, Desertification and Land Degradation, Sustainable Land Management, Food Security and Greenhouse Gas Fluxes in Terrestrial Ecosystems. Available online at: https://www.ipcc.ch/srccl/
Janssen, D. N. G., Ramos, E. P., Linderhof, V., Polman, N., Laspidou, C., Fokkinga, D., et al. (2020). The climate, land, energy, water and food nexus challenge in a land scarce country: innovations in the Netherlands. Sustainability 12:10491. doi: 10.3390/su122410491
Karan, E., Asadi, S., Mohtar, R., and Baawain, M. (2018). Towards the optimization of sustainable food-energy-water systems: a stochastic approach. J. Clean. Prod. 171, 662–674. doi: 10.1016/j.jclepro.2017.10.051
Kimmich, C. (2013). Linking action situations: coordination, conflicts, and evolution in electricity provision for irrigation in andhra pradesh, india. Ecol. Econ. 90, 150–158. doi: 10.1016/j.ecolecon.2013.03.017
Klerkx, L., Aarts, N., and Leeuwis, C. (2010). Adaptive management in agricultural innovation systems: the interactions between innovation networks and their environment. Agric. Syst. 103, 390–400. doi: 10.1016/j.agsy.2010.03.012
Leventon, J. (2021). Scaling behaviour change for a 1.5 degree world: transformations and systems thinking. Glob. Sustain. 4, e27. doi: 10.1017/sus.2021.27
Li, J., Ma, Z., Sun, H., and Chen, W. (2023). Driving factor analysis and dynamic forecast of industrial carbon emissions in resource-dependent cities: a case study of Ordos, China. Environ. Sci. Pollut. Res. 30, 92146–92161. doi: 10.1007/s11356-023-28872-4
Mabhaudhi, T., Simpson, G., Badenhorst, J., Mohammed, M., Motongera, T., Jewitt, A., et al. (2018). Assessing the State of the Water-Energy-Food (WEF) Nexus in South Africa?: Report to the Water Research Commission (Issue July). Available online at: https://www.wrc.org.za/wp-content/uploads/mdocs/KV365-18.pdf
Mewes, R., Kowarsch, L., Reinacher, H., and Nater, U. M. (2016). Barrieren und Chancen zur psychotherapeutischen Versorgung von Asylsuchenden-Eine Befragung niedergelassener Psychotherapeuten in Mittel- und Nordhessen = Obstacles and opportunities for the psychotherapeutic treatment of asylum seekers. Psychother. Psychosom. Med. Psychol. 66, 36–368. doi: 10.1055/s-0042-111314
Mpandeli, S., Naidoo, D., Mabhaudhi, T., Nhemachena, C., Nhamo, L., Liphadzi, S., et al. (2018). Climate change adaptation through the water-energy-food nexus in Southern Africa. Int. J. Res. Public Health 15, 1–19. doi: 10.3390/ijerph15102306
Naidoo, D., Nhamo, L., Mpandeli, S., Sobratee, N., Senzanje, A., Liphadzi, S., et al. (2021). Operationalising the water-energy-food nexus through the theory of change. Renew. Sustain. Energy Rev. 149:111416. doi: 10.1016/j.rser.2021.111416
Nair, A., Tingting, Y., Young, K. R. O., Adegoke, O., Todd, H. C., Lee, S.-Y., et al. (2016). How environmental innovations emerge and proliferate in supply networks: a complex adaptive system perspective. Environ. Innov. Supply Netw. 52, 66–86. doi: 10.1111/jscm.12102
Nightingale, A. J., Eriksen, S., Taylor, M., Forsyth, T., Pelling, M., Newsham, A., et al. (2020). Beyond technical fixes: climate solutions and the great derangement. Clim. Dev. 12, 343–352. doi: 10.1080/17565529.2019.1624495
Østergaard, P. A., Lund, H., and Mathiesen, B. V. (2019). Developments in 4th generation district heating. Int. J. Sustain. Energy Plan. Manage. 20. doi: 10.5278/ijsepm.2019.20.1
Otto, I. M., Donges, J. F., Cremades, R., Bhowmik, A., and Hewitt, R. J. (2020). Social tipping dynamics for stabilizing Earth' s climate by 2050. Proc. Natl. Acad. Sci. U.S.A. 117, 2354–2365. doi: 10.1073/pnas.1900577117
Putra, M. P. I. F., Pradhan, P., and Kropp, J. P. (2020). A systematic analysis of water-energy-food security nexus: a South Asian case study. Sci. Total Environ. 728:138451. doi: 10.1016/j.scitotenv.2020.138451
Ranjan, S., Sow, S., Kumar, S., and Dutta, S. K. (2022). Influence of integrated nutrient management in rice (Oryza sativa) on performance of wheat (Triticum aestivum) under long term rice-wheat cropping system. Ecol. Environ. Conserv. 28, 802–805. doi: 10.53550/eec.2022.v28i02.034
Rasul, G. (2016). Managing the food, water, and energy nexus for achieving the sustainable development goals in South Asia. Environ. Dev. 18, 14–25. doi: 10.1016/j.envdev.2015.12.001
Rasul, G., and Sharma, B. (2016). The nexus approach to water – energy – food security: an option for adaptation to climate change an option for adaptation to climate change. Clim. Policy 16, 682–702. doi: 10.1080/14693062.2015.1029865
Reed, M. S., Evely, A. C., Cundill, G., Fazey, I., Glass, J., Laing, A., et al. (2010). What is social learning? Ecol. Soc. 15:r1. doi: 10.5751/ES-03564-1504r01
Riddell, D., and Moore, M. (2015). Scaling out, scaling up, scaling deep: advancing systemic social innovation and the learning processes to support it. J. Corpor. Citizensh. 1. doi: 10.9774/GLEAF.4700.2015.ju.00009
Rogers, E. M. E. (2004). A prospective and retrospective look at the diffusion model. J. Health Commun. 9, 13–19. doi: 10.1080/10810730490271449
Saltelli, A., and Giampietro, M. (2017). What is wrong with evidence based policy, and how can it be improved? Futures 91, 62–71. doi: 10.1016/j.futures.2016.11.012
Sarkar, P., Salami, M., Githiora, Y., Vieira, R., Navarro, A., Clavijo, D., et al. (2020). A conceptual model to understand the drivers of change in tropical wetlands: a comparative assessment in India and Brazil. Biota Neotrop. 20. doi: 10.1590/1676-0611-bn-2019-0913
Scholz, G., and Methner, N. (2020). A social learning and transition perspective on a climate change project in South Africa. Environ. Innov. Soc. Transit. 34, 322–335. doi: 10.1016/j.eist.2019.10.011
Seto, K. C., Davis, S. J., Mitchell, R. B., Stokes, E. C., Unruh, G., Ürge-Vorsatz, D., et al. (2016). Carbon lock-in: types, causes, and policy implications. Annu. Rev. Environ. Resour. 41, 425–452. doi: 10.1146/annurev-environ-110615-085934
Simpson, G. B., and Jewitt, G. P. (2019a). The water-energy-food nexus in the anthropocene: moving from ‘nexus thinking' to ‘nexus action'. Curr. Opin. Environ. Sustain. 40, 117–123. doi: 10.1016/j.cosust.2019.10.007
Simpson, G. B., and Jewitt, G. P. W. (2019b). The development of the water-energy-food nexus as a framework for achieving resource security: a review. Front. Environ. Sci. 7:8. doi: 10.3389/fenvs.2019.00008
Smith, E. K., and Mayer, A. (2018). A social trap for the climate? Collective action, trust and climate change risk perception in 35 countries. Glob. Environ. Change 49, 140–153. doi: 10.1016/j.gloenvcha.2018.02.014
Sovacool, B. K., Bergman, N., Hopkins, D., and Hielscher, S. (2020). Imagining sustainable energy and mobility transitions: valence, temporality, and radicalism in 38 visions of a low-carbon future. Soc. Stud. Sci. 50, 642–679. doi: 10.1177/0306312720915283
Steffen, B., Egli, F., Pahle, M., and Schmidt, T. S. (2020). Navigating the clean energy transition in the COVID-19 crisis. Joule 4, 1137–1141. doi: 10.1016/j.joule.2020.04.011
UNEP (2019). Emissions Gap Report 2019. Available online at: http://www.unenvironment.org/emissionsgap
UNFCCC (United Nations Framework Convention on Climate Change) (2016). Report of the Conference of the Parties on its twenty-First Sessionheld in Paris from 30 November to 13 December 2015 (Vol. 01192). Bonn: UNFCCC.
UNFCCC (United Nations Framework Convention on Climate Change) (2021). Nationally Determined Contributions under the Paris Agreement: Synthesis Report (Vol. 02674).
Vahabzadeh, M., Afshar, A., Molajou, A., Parnoon, K., and Ashrafi, S. M. (2023). A comprehensive energy simulation model for energy-water-food nexus system analysis: a case study of the great Karun water resources system. J. Clean. Prod. 418:137977. doi: 10.1016/j.jclepro.2023.137977
Villamayor-tomas, S., Grundmann, P., Epstein, G., Evans, T., and Kimmich, C. (2015). The water-energy-food security nexus through the lenses of the value chain and the institutional analysis and development frameworks. Water Altern. 8, 735–755.
Volenzo, T., and Odiyo, J. O. (2020). Perception of environmental spillovers across scale in climate change adaptation planning: the case of small-scale farmers' irrigation strategies, Kenya. Climate 8. doi: 10.20944/preprints201910.0289.v1
Westermann, O., Thornton, P., and Förch, W. (2015). Reaching More Farmers – Innovative Approaches to Scaling up Climate Smart Agriculture (135; CCAFS Working Paper, Issue 135).
Williams, P. D., Alexander, M. J., Barnes, E. A., Butler, A. H., Davies, H. C., Garfinkel, C. I., et al. (2017). A census of atmospheric variability from seconds to decades. Geophys. Res. Lett. 44, 11201–11211. doi: 10.1002/2017GL075483
Zhang, X., and Vesselinov, V. V. (2017). Integrated modeling approach for optimal management of water, energy and food security nexus. Adv. Water Resour. 101, 1–10. doi: 10.1016/j.advwatres.2016.12.017
Keywords: WEF nexus, smart innovations, indigenous knowledge, innovation platforms, sustainability transitions, resource security
Citation: Musetsho KD, Mwendera E, Madzivhandila T, Makungo R, Volenzo TE, Mamphweli NS and Nephawe KA (2024) Assessing and mapping water-energy-food nexus smart innovations and practices in Vhembe District Municipality, Limpopo Province, South Africa. Front. Water 6:1253921. doi: 10.3389/frwa.2024.1253921
Received: 07 July 2023; Accepted: 17 January 2024;
Published: 27 February 2024.
Edited by:
Yizi Shang, China Institute of Water Resources and Hydropower Research, ChinaReviewed by:
Dhaarna Dhaarna, National Institute of Construction Management and Research, IndiaCopyright © 2024 Musetsho, Mwendera, Madzivhandila, Makungo, Volenzo, Mamphweli and Nephawe. This is an open-access article distributed under the terms of the Creative Commons Attribution License (CC BY). The use, distribution or reproduction in other forums is permitted, provided the original author(s) and the copyright owner(s) are credited and that the original publication in this journal is cited, in accordance with accepted academic practice. No use, distribution or reproduction is permitted which does not comply with these terms.
*Correspondence: Khangwelo D. Musetsho, ZG11c2V0c2hvQG5hbGVkemkuY28uemE=
Disclaimer: All claims expressed in this article are solely those of the authors and do not necessarily represent those of their affiliated organizations, or those of the publisher, the editors and the reviewers. Any product that may be evaluated in this article or claim that may be made by its manufacturer is not guaranteed or endorsed by the publisher.
Research integrity at Frontiers
Learn more about the work of our research integrity team to safeguard the quality of each article we publish.