- 1Stockholm Resilience Centre, Stockholm University, Stockholm, Sweden
- 2Faculty of Engineering and Sustainable Development, University of Gävle, Gavle, Sweden
There is a need for new tools in urban planning, design, and management (urban PDM) of green-blue infrastructure (GBI) to better support resilience and sustainable development. Virtual Reality (VR) emerges as a potential tool in this context. GBI provides a diversity of ecosystem services that increase the capacity of urban environments to absorb change while continuing to develop (adaptive capacity), a key factor in resilience and sustainability. However, there is a lack of tools capable of effectively capturing and communicating the designs, functions, and uses of GBI. Despite this need, research on the contributions of VR as a tool in urban PDM of GBI remains limited. To address this gap, an exploratory experiment using inductive qualitative data analysis was conducted. Participants of different ages and with different expertise viewed 360-degree video clips from urban areas through VR, completed a questionnaire, and participated in interviews. The results showed that the 360-degree video VR experience enhanced participants’ understanding of the viewed sites. Specifically, participants paid attention to details, captured the context, responded by generating emotional engagement, identified site development potential, and described an immersive experience. These results align with key principles for the sustainable management of ecosystem services, such as participation, learning, diversity, place-making, and stewardship; and adaptive capacity aspects such as processes and results grounded in complexity-embracing creativity. Our analysis shows that 360-degree video VR can increase the understanding of complexities, connections, and nuances in the urban environment. We conclude that 360-degree video VR could serve as a valuable complement to traditional tools in urban PDM, enriching decision-making with information that can support adaptive capacity, resilience, and sustainability.
1 Introduction
Today’s interplay of biodiversity, climate, and social challenges is increasing the need to ensure sustainability and resilience of urban areas (Pascual et al., 2022). A vital aspect for supporting urban sustainability and resilience is the urban green (natural and semi-natural land) and blue (water) infrastructure, which in this article is referred to as GBI (Gill et al., 2007; Puppim de Oliveira et al., 2022). However, the usual tools applied in urban planning, design, and management (urban PDM) are limited in their capacity to deal with the complexity of urban GBI. Tools typically used include blueprints, photos, written documents, prototypes, online maps, and CAD programs (Bott et al., 2019; Zheng et al., 2017). These instruments lack the ability to include crucial details, functions, and different values of natural landscape objects. Presentations tend to mainly consist of selected visual information, and omit other vital information necessary for experiencing a place, e.g., the soundscape (Sällström, 2000). Expert knowledge is often required for using the tools. Meanwhile, co-creation and different perceptions in decision-making concerning GBI is widely regarded as important for GBI design, access, and interactions (Derkzen et al., 2017; van der Jagt et al., 2019; Zhang and Tan, 2023).
Novel, creative, and integrated approaches are needed to address the complexity of urban challenges, overcome the limitations of traditional tools, and meet requirements for sustainable development. New, systemic ways of thinking about urban PDM are essential (Chang et al., 2018; Elmqvist et al., 2018). In this study, urban areas are regarded as complex, adaptive, social-ecological-technological systems (urban SETS) (Wallhagen, 2016). The SETS continuously co-evolve and adapt to internal and external changes and stressors (Grimm et al., 2015). Planning, design, and management can be understood as processes that together and separately influence the characteristics, functions, and sustainability of the urban SETS.
Emerging digital information and communication technologies (digital ICTs) enable new opportunities for supporting sustainability in urban PDM (Nitoslawski et al., 2021). However, a systems perspective on these opportunities remains an under-studied field (e.g., Colding et al., 2020). Virtual Reality (VR) is a digital ICT that has been discussed as a promising complement to more traditional tools in urban PDM (Sanchez-Sepulveda M. et al., 2019; Suneson et al., 2008). In any VR session, the user wears a headset that enables full immersion in a 3D, 360-degree, multisensory experience (Sutherland, 1968). It is this sensory immersion that typically distinguishes VR from other, non-immersive technologies. For example, in Augmented Reality, another emerging digital ICT, digital objects are displayed overlaying the real world, in real time, on an external screen. This paper focuses on a subtype of VR, 360-degree video VR, which is created by special cameras that capture the entire, 360-degree, environment. The 360-degree video VR presents non-interactive, real-world video footage and audio. This VR differs from 3D VR, which presents digitally created, artificial, and sometimes interactive environments (Bowman and McMahan, 2007).
Both research and policy have increasingly highlighted the importance of considering resilience in urban PDM (Alberti et al., 2018; Elmqvist et al., 2021). In sustainability science, resilience is the capacity of a system to deal with change, and adapt or transform and continue to develop (Biggs et al., 2015; Holling, 1973). Resilience and sustainability of urban SETS can be supported by urban adaptive capacity. Adaptive capacity refers to the agility and reflexibility in governance, technology, and physical structures to continuously learn about, and respond to complex social and environmental changes (Chester and Allenby, 2021; Matthews et al., 2015).
The concept of ecosystem services refers to the benefits people derive from the world’s ecosystems. Ecosystem services provide the foundation for human existence and wellbeing (Bratman et al., 2019; MA, 2005), can increase the capacity to adapt to climate change (Gaffin et al., 2012), and build local and regional ecological resilience (Sassen, 2010). Urban GBI (e.g., trees, forests, lawns, flowerbeds, waterways, and wetlands), can provide ecosystem services such as air purification, temperature regulation, noise reduction, aesthetics, habitats, and spaces for recreation. Ecosystem services are also vital for securing and supporting biodiversity (Andersson et al., 2019; Bolund & Hunhammar, 1999; Ives et al., 2016). Multifunctional GBI refers to spaces and elements that can provide several different types of ecosystem services (Manning et al., 2018).
The concept of ecosystem services was included in the EU Biodiversity Strategy 2030 (European Commission and Directorate-General for Environment, 2021) and the preceding Strategy for 2020 (European Commission, 2011). The Swedish government also recognised the need for better integration of nature in cities to increase the prevalence of urban ecosystem services. The government’s Strategy for living cities – politics for sustainable urban development, Objective 2.2.1, mandates that “a majority of the municipalities, by 2025, safeguard and integrate urban nature and ecosystem services in planning, construction, and management in cities and densely built-up areas” (Regeringskansliet, 2018).
Urban PDM need new tools for supporting this mandate. These new tools must address multifunctional GBI, biodiversity, and the provisioning of ecosystem services (Seifert et al., 2020; Vainio, 2016). Thus, tools need to be capable of dealing with complexity. New tools also must be practical, locally adaptable (Khoshkar et al., 2020), and capable of involving stakeholders across sectors and between levels (Kabisch et al., 2016). Moreover, tools need to facilitate inclusion of groups whose participation in the processes and in urban landscapes is typically underrepresented and overlooked: women, children, and disadvantaged groups (Bosman et al., 2017; Maalsen et al., 2023).
Therefore, this study explores the question: How can 360-degree video VR as a tool support planning, design, and management of urban GBI and the provisioning of ecosystem services? It applies a complex adaptive social-ecological-technological systems approach (Grimm et al., 2015; Levin et al., 2012) and principles for enhancing ecosystem services (Berkes et al., 1998; Biggs et al., 2015).
2 Methods
An exploratory, inductive, qualitative study was conducted to gain insights into how VR can influence the understanding of ecosystem services and urban SETS (Figure 1). An experiment was conducted in which participants used VR headsets. They watched 360-degree video clips of two housing areas with differing characteristics in the city of Gävle, Sweden. The participants were interviewed, observed, and asked to answer a questionnaire.
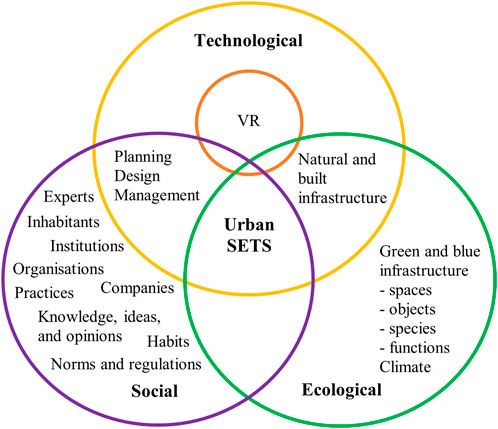
Figure 1. Illustration of the position of VR as a tool in planning, design, and management of urban natural and built infrastructure. The three parts of SETS are each presented with examples of their constituting elements.
A total of 25 professionals and students were selected and invited to participate in the experiment. The participants worked with or studied issues related to urban planning, and were connected to the City of Gävle, the University, or the then-ongoing project Augmented Urbans (Augmented Urbans, 2018). The participants in this study could be categorised as belonging to two groups. One group consisted of experts from academia, architecture, and urban planning with a background in sustainability studies, architecture, landscape architecture, biology, and urban planning. The other group comprised students from the University of Gävle participating in a bachelor’s level course in the Industrial Design program. The students were involved in design tasks linked to the Augmented Urbans project through the program. Seven of the participants were born between 1952 and 1969, five were born between 1970 and 1989, and 13 were born between 1990 and 1998. Ten were women and 15 were men. The expert group included six women and six men. The student group included four women and nine men. The responses from both groups are presented in the text.
The Augmented Urbans project developed Extended Reality (XR) applications and tested their capacities to support participatory planning for urban resilience in seven cities in four countries in the Central Baltic region. The project inspired the experiment.
2.1 Experiment design
The experiment was performed on two different occasions during the autumn season: the first with the group of experts, and the second with the students a month later. Participation was voluntary, and all participants were given information about the experiment and signed a consent form. The participants watched the clips in a room with groups of three to five people at a time. The experiment was sound- and video recorded, to capture how the participants reacted verbally and physically to the experience of using VR. After watching, the participants filled out questionnaires about their perceptions of the experience and how they could imagine using VR in their own work or studies.
2.2 The 360-degree videos and the areas
The 360-degree video clips (Supplementary Material Appendix A) were filmed in 5K pixel resolution, and non-interactive. The clips were filmed during the late summer, using a GoPro Fusion 360 video camera (GoPro, 2021). The camera was small enough to fit in one hand and lightweight, and thus easily portable. The 5K pixel resolution was at the higher end before approaching professional level equipment. A tripod was used for the camera during filming to stabilise the videos, ensure high-quality recording, and make the VR experience more comfortable for the participants in the experiment. The production included filming the 360-degree video clips and uploading them to a server, which required some technological expertise. Two Oculus Go headsets (Meta Technologies LLC, 2021) with an inserted Samsung Galaxy S5 mobile phone were utilised during the experiment. The headsets had buttons and a touch function for navigating the options in the view, and a wheel for adjusting the focus. Operating the headsets required some technological expertise. Each clip lasted approximately 30 s and was played in a loop. Each participant viewed between three and five different point locations.
The 360-degree video clips showed two different sites in Gävle, Hemsta (Figure 2) and Ulvsätersvägen (Figure 3). They were selected to represent two common Swedish housing area and greenspace typologies. The areas consist of blocks of flats built in different stages from the 1960s to the 1990s and associated shared greenspaces, such as courtyards and lawns. The company that owns the buildings had previously conducted a survey to local inhabitants. The results of the survey showed that the greenspaces could be improved for functionality, biodiversity, and aesthetic appeal. The greenspaces thus represented suitable sites for the experiment.
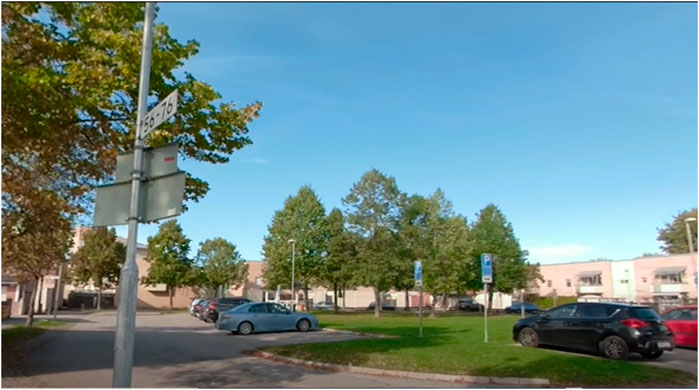
Figure 2. Screenshot from a 360 video clip of Hemsta, facing west. The communal greenspace is laid to grass and surrounded by car parks. The two-storey blocks of flats are in the background.
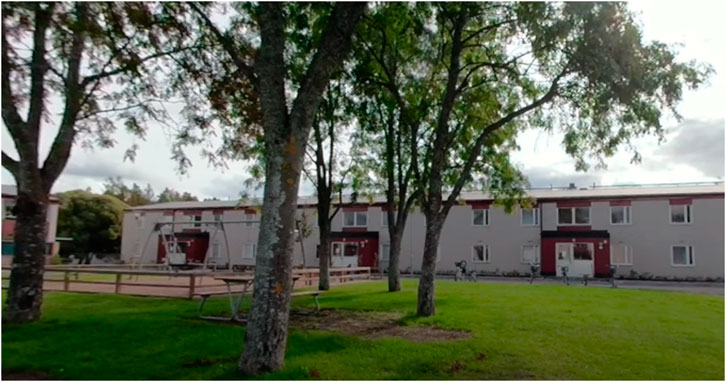
Figure 3. Screenshot from a 360 video clip of Ulvsätersvägen, facing south-south-east. The communal greenspace is mainly laid to grass, also hosting a tree grove and a playground with swings. The two-storey blocks of flats are seen in the background, separated from the greenspace by a paved surface.
2.3 The experiment questionnaire
The questionnaire (Supplementary Material Appendix B) entailed nine questions. The first set of three open-ended questions asked:
a) how the participants experienced using 360-degree video VR,
b) what tools they normally used to plan or get to know an area, and
c) how 360-degree video VR compared to the tools the participants normally used. The subsequent three open-ended questions asked:
d) what possibilities the participants could identify with using 360-degree video VR,
e) what challenges or limitations the participants could identify with using 360-degree video VR, and
f) if they could envision using it in their own work. The questionnaire further entailed three questions about the participants’ age, gender, and occupation.
2.4 Transcriptions and qualitative content analysis
The written questionnaire responses and the recorded verbal comments are from here on jointly referred to as the responses. The responses provided by the participants during the exploratory experiment were processed in an inductive qualitative data analysis (Graneheim and Lundman, 2004). The recorded videos from the experiment were put on a secure server, and the audio files from the interviews were transcribed. The responses were condensed into extracted meaning units, i.e., sentences and words that constituted the units of analysis (Elo et al., 2014). The meaning units were then translated to English, and coded into sub-themes and themes. Thematic saturation, when new information produced little or no change to the codebook (Guest et al., 2006), was reached when approximately 75% of the meaning units had been coded into sub-themes. The sub-themes were grouped into five themes designed to capture and describe common patterns and topics in the responses. A calculation of the distribution of meaning units across the themes was performed. The themes, sub-themes, and extracted meaning units were developed in dialogue between the authors and two additional senior researchers.
3 Results
The results showed that all expert participants and most student participants saw potential in using 360-degree video VR for a variety of purposes (see Section 3.6) in urban planning, design, and management (urban PDM). Purposes included documentation, presentations, and communication of ideas, proposals, and preferences. No participant was against the idea of using 360-degree video VR.
Five over-arching themes were identified in the participants’ written and verbal responses: Attention to detail, Capturing the context, Generating emotional engagement, Identifying site development potential, and Providing an immersive experience. The distribution of responses associated with each theme is presented in Figure 4. This section presents each theme.
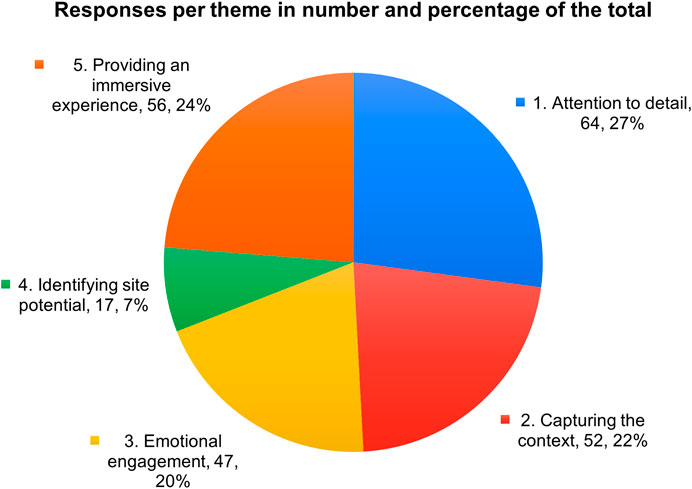
Figure 4. The numerical and percentage distribution of meaning units associated with each theme. The data labels include each theme’s number and name, the number of associated meaning units, and the proportion they represent out of the total 236 meaning units. Some meaning units are linked to multiple themes.
3.1 Attention to detail
The theme Attention to detail garnered the largest number of responses. The 64 individual responses accounted for 27% of the total of 236 responses. Nearly all participants noted numerous details while viewing the 360-degree video clips in VR (Table 1). Responses included identifications of ongoing activities on-site, such as: “There’s someone walking a dog,” and of individual objects: “A fence, a small swing, a barbeque.” Responses also included the colours and condition of objects: “This wood here has not been doing so well. The façade and such made of wood and planks. There was some growth on them, and you can see that here” and object details: “I’m standing with a streetlight right in front of me, where 5,676 is written.” Responses also included identification of the materials of objects: “Here’s bricks, with bright sealant. A concrete staircase and then a slightly longer building, with a pent roof.”
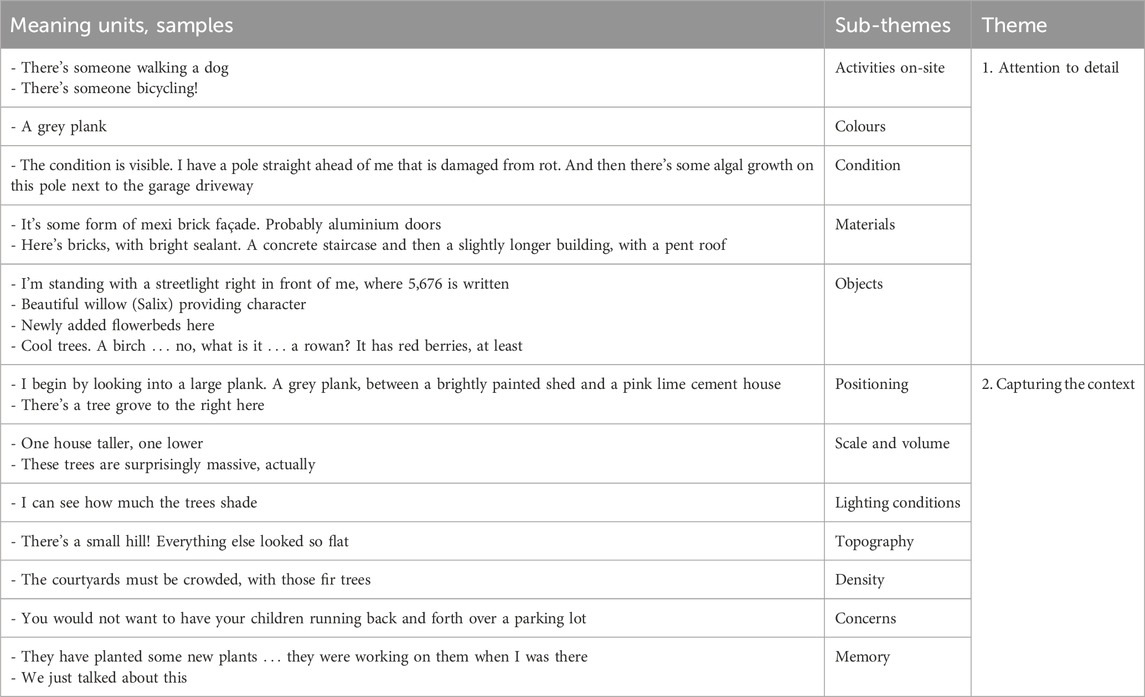
Table 1. Overview of Theme 1, Attention to detail, and Theme 2, Capturing the context. Samples of responses transcribed, translated, and condensed into meaning units, sub-themes, and themes.
Other responses pertained to the green infrastructure. They included identification of objects: “Newly added flowerbeds there,” species: “Beautiful willow, providing character,” and object details: “Cool trees. A birch what is it a rowan? It has red berries, at least.”
3.2 Capturing the context
The responses showed that the VR experience captured the context of the site (Table 1). The theme Capturing the context garnered 52 responses, representing 22% of the total of 236 responses. Sub-themes pertained to the physical properties of the sites, and to planning and design processes associated with the sites. Responses pertained to the physical context of the sites. They concerned objects’ individual and relative positioning, such as: “I begin by looking into a large plank. A grey plank, between a brightly painted shed and a pink lime cement house,” and scale and volume: “There’s one house taller, one lower,” and “These trees are surprisingly massive, actually.” Responses also concerned the lighting conditions: “I can see how much the trees shade,” the landscape topography: “There’s a small hill! Everything else was so flat,” and the density: “The courtyards must be crowded, with those fir trees.” Viewers noted that the VR experience provided them with a holistic understanding of the site, for example, by expressing that “this is incredibly good for generating context.”
Concerns raised in the responses focused on the design and intended use of sites: “It is clear how the lawn and the parking space destroy the entirety. This potential park is destroyed by the parking spaces.” Others focused on safety: “You would not want to have your children running back and forth over a parking lot.”
For some participants, the visual experience triggered memories from planning, design, and management dialogues: “We just talked about this” and of previous visits to the sites: “They were working on [the flowerbeds] when I was there.” The visual experience also triggered mental associations to ongoing planning and design processes: “These crowded outdoor places, we want to make them greener.” Suggestions were presented: “if digital objects, such as buildings or trees, could be inserted into a VR presentation, it might help with understanding suggestions for potential changes in the current, local context.”
3.3 Generating emotional engagement
Most participants reacted with emotional responses and subsequent inspiration to act (Table 2), when viewing the 360-degree videos in VR. The theme Generating emotional engagement garnered 47 responses or 20% of the total of 236 responses. Assessments of the aesthetics of the sites were made, such as: “That’s the most unwelcoming barbecue site I’ve seen,” and “the outdoors environment is sad looking.” Trees were deemed “beautiful,” a playground “prison-like,” and walls “large, empty” and “horribly ugly.”
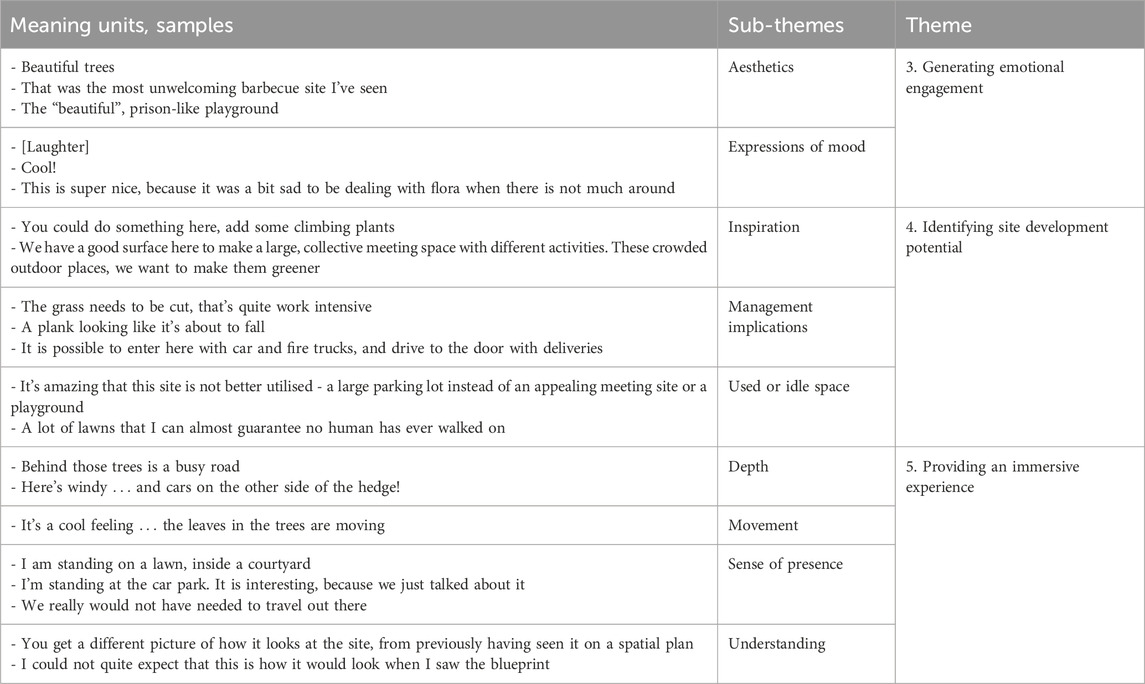
Table 2. Overview of Theme 3, Generating emotional engagement, Theme 4, Identifying site development potential, and Theme 5, Providing an immersive experience. Samples of responses transcribed, translated, and condensed to meaning units, sub-themes, and themes.
During the experiment, while wearing the headset, participants expressed mood by exclaiming words, “Cool!,” “Great!,” and bursting out in surprised or excited laughter. Following the expressions of emotional responses, a range of ideas for taking action were generated, which are presented in the next theme.
3.4 Identifying site development potential
The results included responses regarding how VR could be used to Identify site development potential (Table 2). This theme garnered 17 responses or 7% of the total of 236 responses. Issues were spotted, e.g., impressions of dense outdoor spaces. Suggestions on adding GBI were presented: both for creating more appealing sites, such as: “You could do something here, put some climbing plants on it,” and designing for different purposes: “We have a pretty good surface here to make a large, collective meeting space with different activities.”
Responses associated with Identifying site development potential also pertained to the use of VR for presenting sites during different seasons, or for showing what sites could have looked like in the past. Some suggested that the VR experience could be further developed to help communicating ideas, e.g., by allowing for digital objects to be inserted in the 360-degree video view: “If I make a bench or something, I could put it there.”
Some made observations regarding the age and condition of materials and vegetation, potentially associated with management implications like repair needs: “I have a pole right in front of me that’s damaged from rot” and “a plank that looks like it is about to fall.” Other observations pertained to management requirements more directly: “The grass needs to be cut, that’s quite work intensive.”
Responses included identifications of the intended use of sites: “Ah, there is a pétanque field.” Idle sites that were not used were identified: “A lot of lawns that I can almost guarantee that no human has ever walked on.” Responses identified issues with existing green infrastructure and provided hints at how they could be improved: “There’s very little colour in the area, it is only green. There are no flowers anywhere.” Some suggested how sites could be redesigned: “It is amazing that this site is not better utilised, I think - a large parking lot instead of an appealing meeting site or a small playground.”
3.5 Providing an immersive experience
The results from the experiment showed that VR was Providing an immersive experience (Table 2). The theme garnered 56 responses, or 24% of the total of 236 responses. Responses simultaneously expressed emotions and observations of movement, such as: “It is a cool feeling … the leaves are moving in the trees.” They described a sense of depth: “Behind those trees is a busy road” and “Here’s windy, and cars on the other side of the hedge!.” Responses further highlighted the multisensory experience provided by the VR headset as sound in addition to the 360-degree video view: “I can hear traffic and the wind.”
While wearing the VR headset and viewing the 360-degree videos, several participants expressed a sense of presence at the site: “I’m standing on a lawn, inside a courtyard” and “I feel like I am at the site.” A couple of participants expressed that the VR experience could replace on-site visits: “We really would not have needed to travel out there to look.”
In one of the video clips, a person was recorded walking up towards the camera. A participant who was wearing the VR headset commented on the perspective: “I can imagine it is how cats and dogs see people, before they get picked up.” Another participant stated: “I feel like I stand higher up than I do in real life.”
Participants noted a flexibility in the viewer perspective when using VR: “I can look up at the underside of the roof beams that protrude in the eaves.” As for the 360-degree video’s 5K resolution, two of the responses were: “You see what it looks like … except for the resolution, which is better in real life” and “It is a bit grainy.” The possibility of experiencing a site in VR during a different season than what’s shown in the video, i.e., temporal flexibility was highlighted in the responses: “This is super nice, because it was a bit sad to be dealing with flora when there was not much around.”
The VR experience was described as providing a different, and possibly deeper, understanding of the site compared to other tools: “You get a different picture of how it looks at the site, from previously having seen it on a spatial plan” and “When we were [in the other room], we sat there with a blueprint, a coloured plan, and a photo. Here we got so much more.”
3.6 Respondents’ views on potential contributions and limitations of the 360-degree video VR as a tool
The written questionnaire provided the participants with an opportunity to further elaborate on their impressions of the experiment. The responses showed that a mix of digital and non-digital tools (Supplementary Material Appendix C) was normally used. The 360-degree video VR was commonly described as easy to use and easily providing a three-dimensional understanding of a site. All except two participants was trying VR for the first time. One of the participants who had tried VR before stated that the 360-degree video VR experience in the experiment was grainier than in gaming VR, but provided more depth than, e.g., Google Maps. The 360-degree video VR was described as differing from photos, for instance, and was suggested as a potentially suitable tool specifically in urban planning and design. Suggestions on uses of 360-degree video VR in the participants’ own professions included: for presentations, to facilitate dialogues, to generate an understanding, and to spark curiosity (Table 3).
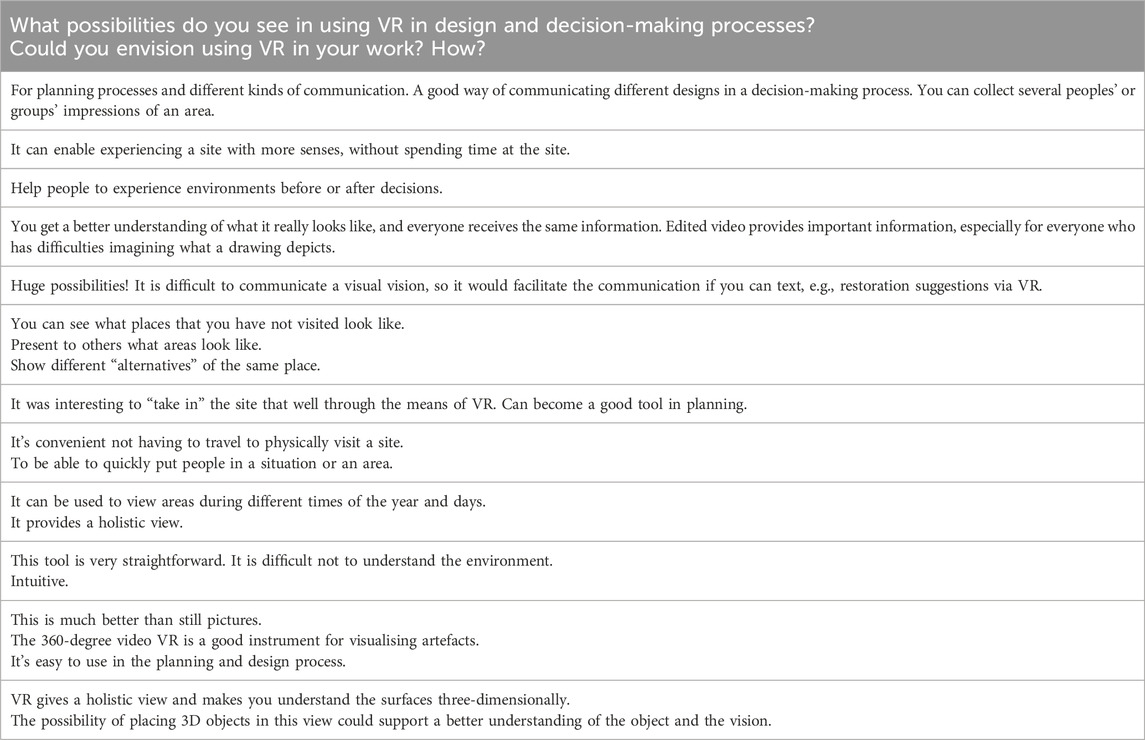
Table 3. Samples of responses to the questions of potential contributions of using 360-degree video VR in design and decision-making, and if and how participants could envision using VR as a tool in their own work.
Ideas on uses of 360-degree video VR further included: to present sites to others, to “visit” other sites, and to experience a site during different seasons and times of the day. A couple of participants responded that 360-degree video VR could minimise the need for physical visits, which was regarded as a possibility and a risk, respectively. The idea of combining 360-degree video VR with other digital tools, e.g., GIS and CAD, was presented. The aim would be to visualise design proposals in their environment and to enable rapid re-designs, but without the resources, e.g., time, skills, and money, needed in experiments that involve physical prototypes. Suggestions also included modifications to the experience: to enable written feedback in the VR view, create scenarios, present before and after-scenarios, and recreate historic environments.
In their written responses, 21 participants specified their perceived limitations of the 360-degree video VR (Table 4). Each perceived limitation occurred between one to four times. Perceived limitations pertained to the technology, encompassing the hardware and the production. Specifically, using the headset requires technological expertise, filming is required prior to using the VR headset, production and equipment could be costly, and the experience is limited to the extent of the battery life. At least one participant responded that the use process was time consuming. Other limitations pertained to the presentation of the video clips: the view was perceived as less detailed compared to real-life visits, and a couple of responses even described it as blurry. A couple of participants responded that repeat visits over time is crucial for understanding how sites change over time through, e.g., seasons and weather. However, the video clips only showed the sites from one occasion. Perceived limitations also pertained to the user experience. These included feelings of dizziness and discomfort, and that users needed time to get used to the headset before they could absorb the presented information. The experience was described as individual, which could prevent discussions, the 360-degree video VR could prevent on-site visits. Additionally, one respondent expressed concern that the screen’s close proximity to the eyes might cause eye damage.
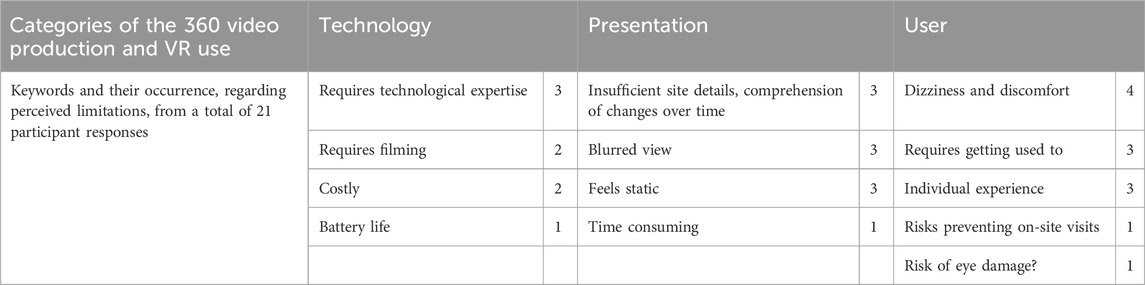
Table 4. Keywords from the responses to the question: “What limitations, obstacles, or difficulties associated with using the VR technology do you perceive?” The answers are grouped and presented in categories that reflect aspects of the VR process, from production to presentation.
It took between 1.5 and 4 min for each user to put on the headset, get used to operating it, and navigating the playlist. Around 15% of the users accidently left the playlist and ended up elsewhere on YouTube or in the Oculus VR app library. A couple of the participants expressed in their responses that it was valuable to have a skilled VR person present during the trials to facilitate with the technology.
4 Discussion
4.1 VR has potential to mitigate known limitations of traditional tools
We set out to explore the question: How can VR as a tool support planning, design, and management of urban GBI and the provisioning of ecosystem services? The results from our explorative, qualitative study show that 360-degree video VR technology can complement the usual tools traditionally used in urban planning, design, and management (urban PDM) (blueprints, physical models, texts, photos, online maps, CAD, etc.). The results further show that 360-degree video VR provided a feeling of immersion and supported absorption of multi-sensory information through a combination of audio and visual input. The technology was described as intuitive to use, and the presented information meaningful and easy to absorb. Results show that 360-degree video VR has the capacity to capture and present: important characteristics of the built environment, human-nature interactions, and the complex, continuously evolving, dynamic, multi-scalar networks of different living organisms that produce ecosystem services. The capacity can increase the understanding of the social-ecological complexities and nuances at a site, and as such support informed decision-making associated with urban GBI, an area where research has identified existing limitations (Cook et al., 2024).
Traditional tools in urban PDM tend to be limited in their capacity to embrace the complexity of urban challenges. Physical and digital models present selected information, largely lacking context and detailed information on vegetation or animal life, and can limit designs to simple, rectilinear shapes that are easy to copy and replicate. Understanding written documents and blueprints typically require specialised expert knowledge. Such requirements give rise to the participation paradox, in which access to information in the early stages of planning, when important decisions are made, is limited to selected experts (Wolf et al., 2020). Furthermore, urban PDM processes have been found to risk exacerbating social inequalities, and to be dominated by and limited to primarily male participation and perspectives (Maalsen et al., 2023; Meerow et al., 2019). In our study, we found that the 360-degree video VR showed potential to address and mitigate such limitations.
4.2 Implications for building urban adaptive capacity
Earlier research has indicated that VR might strengthen adaptive capacity aspects of planning, design, and management processes, focusing on capacities like empathy building, flexibility in perspective taking, collaboration, and learning (Barbot and Kaufman, 2020; Hanzl, 2007). The following sections discuss how the results may be understood in terms of support for adaptive capacity in the processes, and in built-up and natural urban landscapes. The discussion is structured according to the themes and sub-themes.
4.2.1 Attention to detail
The theme Attention to detail includes identification of activities on-site, and the colours, conditions, and materials of built infrastructure objects. The theme also encompasses the condition, location, and design of green infrastructure objects such as flowerbeds, lawns, and trees, and plant and animal species. The properties of 360-degree video VR that enable users to identify these elements link to several of the principles for supporting PDM of urban ecosystem services as identified by Biggs et al. (2015). Examples of principles include: maintaining diversity and redundancy, encouraging learning, and broadening participation.
The presentation of objects through visual and audio data, and with high spatial accuracy, can be useful for a range of users. For example, planners, designers, managers, and others, who cannot travel to a physical site, are experts on different tools or topics, or are new to a site. Attention to detail enabled by 360-degree video VR could support planners and designers with preparations for different scenarios, and suggestions on alternative designs or management plans. It could also inform managers on the volumes, materials, and colours of buildings that need maintenance work and so on. As planning, design, and management processes are increasingly digitalised, the presentation of details could also be useful if 360-degree video VR were combined with data in augmented information models to visualise projected local changes, e.g., temperature, rain, or drought, new or invasive species, or population density.
4.2.2 Capturing the context
Where Attention to detail focused on individual objects within an area, Capturing the context connects different objects and their surroundings. Capturing the context facilitates the contextual understanding of an area, e.g., how urban objects, both built and natural, relate to each other in terms of scale, volume, and positioning; and how they relate to topography, light, and shade conditions.
VR-enabled contextual understanding could inform planners, designers, and managers in design and decision-making on heights and densities of spatial objects, and the combinations of materials, textures, colours and so on in the area. It could also inform on the shapes, extents, and functions of vegetation, and the assemblages of plant and animal species in the local and the surrounding area. By providing this kind of understanding of the properties of the urban infrastructure and SETS, 360-degree video VR can help decision-making on suitable heights, shapes, and placements of new constructions to maintain flows of ecosystem services, for instance, to allow for sunlight to enter, and to prevent wind corridors. Contextual understanding could facilitate identification of social concerns such as safety, light pollution, and noise issues. It could also inform on how differently people experience and perceive contexts, and how their capacities of utilising an area might be impaired.
Other studies on VR support the result in our analysis that 360-degree video VR could prompt participants’ memories of places and experiences (e.g., Subramanian et al., 2017). Prompting memories can generate feelings of attachment to places, assign meanings, and support place-based agency and long-term management (Stedman, 2002). In urban PDM, prompting memories could contribute to dialogues: with new ideas, spatially exact information about a site, and relevant knowledge from other places K regarding species, their use, or ecological management practices (e.g., Tengö et al., 2014).
Capturing the context also relates to fostering complex adaptive systems thinking. Complex adaptive systems refer to people’s capacity (and that of our governing institutions) to understand ourselves and the world around us as linked, continuously evolving, and adapting systems. The 360-degree video VR shows promise in both capturing and revealing the complexity of urban SETS. This capacity could support adaptive systems understanding and inform decision-makers on prerequisites, needs, and potentials for GBI in the local SETS context.
4.2.3 Generating emotional engagement
The capacity of VR to generate emotional engagement was demonstrated by the participants as expressions of mood, i.e., laughing, amazement and, for some, slight discomfort. Emotional engagement was also expressed as immediate responses to the viewed information, pertaining, for instance., to aesthetic qualities of trees and buildings as pleasant, beautiful, or ugly. The result supports those in other studies, where a closely related concept to emotional engagement, empathy, is identified as a strong feature in VR use (Sanchez-Sepulveda M. V. et al., 2019; Stauskis, 2014). Emotional engagement has been found to increase creativity, a key aspect of innovations, even lasting past the period of immersion (Houzangbe et al., 2022). It is also important for spurring motivation to act to maintain or enhance valued attributes of a site (Stedman, 2002), by participating in activities in the landscape or in planning and design processes.
Engaging participants and inspiring responses between and within groups of experts and non-experts, can facilitate inclusion and combinations of multiple fields of expertise and knowledge: Inclusion of diverse sources of knowledge is a key element in building adaptive capacity (Kabisch et al., 2016). It can deepen the understanding of prerequisites, challenges, needs, and interests nested in different groups and individuals, and contribute to creative ideas for new solutions.
Emotional engagement is also a fundamental step towards feeling a sense of responsibility for a site and taking action to support its wellbeing and functions. In resilience research, this connection is known as stewardship (Andersson et al., 2014; Chapin III et al., 2010) and sense of place (Masterson et al., 2017). Urban planners often work with other experts, e.g., internationally renowned architectural firms, who can be in other countries and lack prior experience of the sites. Emotional engagement through 360-degree video VR can be one way of strengthening experts’ emotional connection to a site and their sense of responsibility towards the planning and design processes.
4.2.4 Identifying site development potential
In our study, participants were inspired to present new ideas regarding site designs, e.g., turning a parking space into a green site offering a meeting space for different activities.
This result shows how the 360-degree video VR experience could help in identifying used or idle space by capturing activities (e.g., dog walking), details (e.g., graffiti), or a lack of activities (e.g., unkempt spaces). The identification could guide decision-makers to suitable sites for interventions; prioritising where interventions could be needed to improve ecosystem services or reduce maintenance costs, e.g., an empty lawn; and identifying appropriate types of intervention. Collaboration, creativity, and experimentation are important elements in planning, design, and long-term management of ecosystem services-based solutions, and in building urban adaptive capacity (Elmqvist et al., 2018).
Identifying site development potential connects to problem identification and creative solution ideation, processes that are known to benefit from multi-stakeholder collaborations. This theme also connects to two approaches in urban PDM, known as safe-to-fail, and urban tinkering (Ahern, 2011; Elmqvist et al., 2018). Focus is on design that allows for failure, of supporting creativity, including different sources of knowledge, and enabling experimentation in the processes of designing resilient and potentially multifunctional solutions, e.g., GBI that can deal with flooding and drought conditions.
4.2.5 Providing an immersive experience
The multisensory experience provided when both audio and visual information are received created a feeling of immersion, as observed in other studies (e.g., Slater, 2009). Participants felt as though they were physically present at the site displayed in the VR view and were engaged with the experience. For a planner or designer, the immersive experience can provide important information in bundles, e.g., the sounds, lights, reflections, and wind conditions at a site; details like what butterflies and other insects move through an area; and the speeds at which people travel as pedestrians, cyclists, and drivers. Participants responded that the 360-degree video VR provided them with an understanding of the depths at the site, which they had not experienced with other tools. For example, they could hear the sound of cars behind hedges, and notice movements, e.g., people walking their dog. The effects contributed to deepen the understanding of the site.
Filming from different heights and locations can represent the perspectives of different people, such as children and individuals with health conditions or impairments (Götzelmann and Kreimeier, 2020) and of animals (Steel et al., 2015). Representation of different perspectives could support social inclusion and diversity in planning, design, and management, and support ecosystem-based management. In both cases, heights, distances, obstacles, users and uses can be better identified, expressed, and understood. The immersive experience in 360-degree video VR could furthermore support better understanding of other important aspects that influence greenspace usage, such as perceptions of greenspace safety, resource quality (Rigolon, 2016), and comfort (Vukmirovic et al., 2019).
The VR headsets are easily transported, enabling users to experience geographical sites without having to travel. Users can experience a site as it was when the 360-degree video was recorded, at any season of the year or any time of the day. Potential benefits include experts in different cities or countries sharing knowledge or ideas for a site, saving on travel-related costs, carbon emissions, and other environmental impacts.
For urban GBI managers, the 360-degree video VR could provide detailed, spatially accurate information on the changing state of the GBI, informing about when, where, and which vegetation might appear; or when and where interventions should be done or avoided. Many other studies establish that efforts to enhance the adaptive capacity of urban social-ecological systems must be supported by continuous learning and experimentation (e.g., Biggs et al., 2015; Elmqvist et al., 2018).
4.3 Limitations
Although this study shows the 360-degree video VR experience has widespread application, the technology relies predominantly on the user’s ocular capacities. It could thus be of limited use for the visually impaired. The risk of discomfort or nausea, as some participants experienced, could limit or exclude participation by people with vestibular issues. The need for data storage could also constrain the usefulness of 360-degree video VR: at around 0.5 gigabyte per 30 s, the 360-degree video files required ample data storage resources.
The study was conducted in Sweden, but it could be replicated in different SETS contexts in terms of ecosystems, technological resources, governance systems, demographics, urbanisation rates, projected climate change effects and so on. The study explored aspects related to the potential use of VR and did not include an analysis of why VR was not already being used. The study was conducted as an experiment, in which each participant tried the VR experience once in a spatial and social context that was not part of their usual work or study environment. All participants had backgrounds within urban PDM. A different emphasis in responses might be expected from participants specialised in the development or use of digital ICTs. Similarly, if conducting a more extensive study, it could preferably engage an even more socially diverse group of participants to better align with sustainability commitments as outlined in, for example, the Sustainable Development Goal 11, Sustainable Cities and Communities, and Agenda 2030 (United Nations, 2015).
4.4 Recommendations for use of VR, and suggested topics for research
Based on the results of this study, we have formulated the following recommendations for the use of 360-degree video VR in urban planning, design, and management:
- Complement other tools with 360-degree video VR. The 360-degree video VR should be regarded as a complement to other tools, providing capacities to accessibly present rich, specific, and accurate information. The capacities can facilitate dialogues, knowledge sharing, and creative ideation between experts in different fields, and between experts and non-experts. Dialogues are important for supporting informed decision-making in complex systems, and for identifying novel solutions for dealing with often escalating and unpredictable change.
- Consciously design equitable use processes. Planned VR use is a process in which each step can influence participation and equitability: the identification and invitations of participants, selection of location, the information and response provisioning and processing, and transparency in decision-making and implementation. Participation is a vital aspect of building agency, a strong contributor to sustainable long-term GBI management. Consciously designing the steps of the VR use can widen the inclusion and meaningful participation of groups, knowledge, and ideas.
- Keep exploring potential digital evolution pathways. As digital ICTs are developing, the digitalisation of urban PDM proceeds, and challenges to urban areas grow in complexity, there are many opportunities to explore how as a tool and its use can evolve to support dealing with SETS complexities.
Our suggestions on future research on VR and other digital ICTs as tools in planning, design, and management of urban ecosystem services and resilience include:
- What other ICT tools and frameworks, beyond VR and adaptive capacity, could be useful for assessing resilience of urban landscapes?
- How can VR be used to visualise scenarios of projected future turbulence in and of urban SETS caused by climate change?
- How can the visualisation capacities of VR be combined with the computational powers and artistic capacities of Artificial Intelligence? Specifically, to present augmented digital scenarios of greener urban futures, or models of the effects of GBI on the local provisioning of ecosystem services?
- What factors are enabling or limiting the use of 360-degree video VR in urban PDM in different contexts, e.g., in developing and in developed urbanising areas?
5 Conclusion
This study demonstrates that the capabilities of 360-degree video VR make it a potential complement to existing tools in urban planning, design, and management (urban PDM) for supporting the ecosystem services provided by green-blue infrastructure (GBI). Urban GBI and its ecosystem services are considered part of continuously developing, complex adaptive social-ecological-technological systems (SETS) and are crucial for urban resilience and sustainability. However, traditional planning, design, and management tools are often limited in their capacity to capture the details, functions, and uses of GBI. An exploratory experiment and inductive qualitative data analysis identified five themes linked to 360-degree video VR capabilities: 1) Attention to detail, 2) Capturing the context, 3) Generating emotional engagement, 4) Identifying site development potential, and 5) Providing an immersive experience. The results showed that 360-degree video VR provided participants with a detailed and nuanced understanding of the sites, and participants were overall positive about using 360-degree video VR in their work. Potential contributions of 360-degree video VR include enabling spatially precise and contextually rich input; inspiring dialogue among experts across different cities or countries; fostering communication between experts and non-experts; facilitating the inclusion of citizen groups that use GBI but traditionally play a minor role in urban decision-making; and supporting decisions related to GBI management interventions and long-term planning. The results relate to key aspects of social-ecological adaptive capacity theory, particularly in promoting experimentation, creativity, and the multifunctionality of GBI. The findings also align with established principles for building resilience in SETS, such as promoting diversity (both biodiversity and inclusion of different sources of knowledge), stewardship, sense of place, participation, and learning. We conclude that 360-degree video VR displays promising potential as a tool in urban PDM for managing GBI as part of complex adaptive systems.
Data availability statement
The raw data supporting the conclusions of this article will be made available by the authors, without undue reservation.
Ethics statement
Ethical approval was not required for the studies involving humans because no participants who could be considered vulnerable participated. The article contains no personally identifiable information. No information that could be regarded as sensitive was requested, e.g., regarding the participants’ ethnicity, sexuality, religion or union affiliation. The studies were conducted in accordance with the local legislation and institutional requirements. The participants provided their written informed consent to participate in this study.
Author contributions
MS: Conceptualization, Data curation, Formal Analysis, Funding acquisition, Investigation, Project administration, Validation, Visualization, Writing–original draft, Writing–review and editing. MW: Conceptualization, Funding acquisition, Investigation, Methodology, Supervision, Validation, Writing–review and editing.
Funding
The author(s) declare that financial support was received for the research, authorship, and/or publication of this article. The University of Gävle and Stockholm University provided funding for the research and publishing.
Acknowledgments
We thank Fredrik Moberg, Ph.D., and Prof. Erik Andersson for reflections and comments. We also express our gratitude to the experiment participants, and to the course leaders for supporting the students’ participation.
Conflict of interest
The authors declare that the research was conducted in the absence of any commercial or financial relationships that could be construed as a potential conflict of interest.
Publisher’s note
All claims expressed in this article are solely those of the authors and do not necessarily represent those of their affiliated organizations, or those of the publisher, the editors and the reviewers. Any product that may be evaluated in this article, or claim that may be made by its manufacturer, is not guaranteed or endorsed by the publisher.
Supplementary material
The Supplementary Material for this article can be found online at: https://www.frontiersin.org/articles/10.3389/frvir.2024.1432556/full#supplementary-material
References
Ahern, J. (2011). From fail-safe to safe-to-fail: sustainability and resilience in the new urban world. Landsc. Urban Plan. 100 (4), 341–343. doi:10.1016/j.landurbplan.2011.02.021
Alberti, M., McPhearson, T., and Gonzalez, A. (2018). “Embracing urban complexity,” in Urban planet. Editors T. Elmqvist, X. Bai, N. Frantzeskaki, C. Griffith, D. Maddox, T. McPhearsonet al. (Cambridge University Press), 45–67. doi:10.1017/9781316647554.004
Andersson, E., Barthel, S., Borgström, S., Colding, J., Elmqvist, T., Folke, C., et al. (2014). Reconnecting cities to the biosphere: stewardship of green infrastructure and urban ecosystem services. Ambio 43, 445–453. doi:10.1007/s13280-014-0506-y
Andersson, E., Langemeyer, J., Borgström, S., McPhearson, T., Haase, D., Kronenberg, J., et al. (2019). Enabling green and blue infrastructure to improve contributions to human well-being and equity in urban systems. BioScience 69 (7), 566–574. doi:10.1093/biosci/biz058
Augmented Urbans (2018). Helsinki, Finland: Metropolia University. Available at: www.augmentedurbans.eu (Accessed February, 2021).
Barbot, B., and Kaufman, J. C. (2020). What makes immersive virtual reality the ultimate empathy machine? Discerning the underlying mechanisms of change. Comput. Hum. Behav. 111 (February), 106431. doi:10.1016/j.chb.2020.106431
Berkes, F., Folke, C., and Colding, J. (1998). Linking social and ecological systems—management practices and social mechanisms for building resilience. 1st ed. Cambridge University Press. Available at: https://www.cambridge.org/vi/universitypress/subjects/life-sciences/ecology-and-conservation/linking-social-and-ecological-systems-management-practices-and-social-mechanisms-building-resilience?format=HB (Accessed October 20, 2023).
R. Biggs, M. Schlüter, and M. Schoon (2015). Principles for building resilience: sustaining ecosystem services in social-ecological systems (Cambridge, England: Cambridge University Press).
Bolund, P., and Hunhammar, S. (1999). Ecosystem services in urban areas. Ecol. Econ. 29 (2), 293–301. doi:10.1016/S0921-8009(99)00013-0
Bosman, C., Grant-Smith, D., and Osborne, N. (2017). Women in planning in the twenty-first century. Aust. Plan. 54 (1), 1–5. doi:10.1080/07293682.2017.1297321
Bott, H., Grassl, G. C., and Anders, S. (2019). Sustainable urban planning—vibrant neighbourhoods—smart cities—resilience. DETAIL Bus. Inf. GmbH. Available at: https://www.detail.de/en/de_en/sustainable-urban-planning (Accessed April 04 2024).
Bowman, D. A., and McMahan, R. P. (2007). Virtual reality: how much immersion is enough? Computer 40 (7), 36–43. doi:10.1109/MC.2007.257
Bratman, G. N., Anderson, C. B., Berman, M. G., Cochran, B., De Vries, S., Flanders, J., et al. (2019). Nature and mental health: an ecosystem service perspective. Sci. Adv. 5, eaax0903–927. doi:10.1126/sciadv.aax0903
Chang, D. L., Sabatini-Marques, J., da Costa, E. M., Selig, P. M., and Yigitcanlar, T. (2018). Knowledge-based, smart and sustainable cities: a provocation for a conceptual framework. J. Open Innovation Technol. Mark. Complex. 4 (1), 1–17. doi:10.1186/s40852-018-0087-2
Chapin III, F. S., Carpenter, S. R., Kofinas, G. P., Folke, C., Abel, N., Clark, W. C., et al. (2010). Ecosystem stewardship: sustainability strategies for a rapidly changing planet. Trends Ecol. and Evol. 25 (4), 241–249. doi:10.1016/j.tree.2009.10.008
Chester, M. V., and Allenby, B. (2021). Toward adaptive infrastructure: the Fifth Discipline. Sustain. Resilient Infrastructure 6 (5), 334–338. doi:10.1080/23789689.2020.1762045
Colding, J., Wallhagen, M., Sörqvist, P., Marcus, L., Hillman, K., Samuelsson, K., et al. (2020). Applying a systems perspective on the notion of the smart city. Smart Cities 3 (22), 420–429. doi:10.3390/smartcities3020022
Cook, L. M., Good, K. D., Moretti, M., Kremer, P., Wadzuk, B., Traver, R., et al. (2024). Towards the intentional multifunctionality of urban green infrastructure: a paradox of choice? Npj Urban Sustain. 4 (1), 12. doi:10.1038/s42949-024-00145-0
Derkzen, M. L., van Teeffelen, A. J. A., and Verburg, P. H. (2017). Green infrastructure for urban climate adaptation: how do residents’ views on climate impacts and green infrastructure shape adaptation preferences? Landsc. Urban Plan. 157, 106–130. doi:10.1016/j.landurbplan.2016.05.027
Elmqvist, T., Andersson, E., McPhearson, T., Bai, X., Bettencourt, L., Brondizio, E., et al. (2021). Urbanization in and for the anthropocene. Nat. Urban Sustain. 1, 6. doi:10.1038/s42949-021-00018-w
Elmqvist, T., Siri, J., Andersson, E., Anderson, P., Bai, X., Das, P. K., et al. (2018). Urban tinkering. Sustain. Sci. 13 (6), 1549–1564. doi:10.1007/s11625-018-0611-0
Elo, S., Kääriäinen, M., Kanste, O., Pölkki, T., Utriainen, K., and Kyngäs, H. (2014). Qualitative content analysis: a focus on trustworthiness. SAGE Open 4 (1), 215824401452263. doi:10.1177/2158244014522633
European Commission (2011). The EU biodiversity Strategy to 2020. Luxembourg: Publications Office of the European Union, 1–28.
European Commission, and Directorate-General for Environment (2021). “EU Biodiversity Strategy for 2030—bringing nature back into our lives,” in Communication from the commission to the European parliament, the council, the European economic and social committee and the committee of the regions (Luxembourg: Publications Office of the European Union), 23.
Gaffin, S. R., Rosenzweig, C., and Kong, A. Y. Y. (2012). Adapting to climate change through urban green infrastructure. Nat. Clim. Change 2, 704. doi:10.1038/nclimate1685
Gill, S. E., Handley, J. F., Ennos, A. R., and Pauleit, S. (2007). Adapting cities for climate change: the role of the green infrastructure. Built Environ. 33 (1), 115–133. doi:10.2148/benv.33.1.115
GoPro (2021). GoPro Fusion | the most versatile 360 action camera ever. Available at: https://gopro.com/en/hk/shop/cameras/fusion/CHDHZ-103-FW.html (Accessed April 30 2024).
Götzelmann, T., and Kreimeier, J. (2020). Towards the inclusion of wheelchair users in smart city planning through virtual reality simulation. ACM Int. Conf. Proceeding Ser., 440–446. doi:10.1145/3389189.3398008
Graneheim, U. H., and Lundman, B. (2004). Qualitative content analysis in nursing research: concepts, procedures and measures to achieve trustworthiness. Nurse Educ. Today 24 (2), 105–112. doi:10.1016/j.nedt.2003.10.001
Grimm, N. B., Cook, E. M., Hale, R. L., and Iwaniec, D. M. (2015). “A broader framing of ecosystem services in cities,” in The routledge handbook of urbanization and global environmental change. 1st Edn (England, United Kingdom: Routledge Handbooks Online), 202–212.
Guest, G., Bunce, A., and Johnson, L. (2006). How many interviews are enough? an experiment with data saturation and variability. Field Methods 18 (1), 59–82. doi:10.1177/1525822X05279903
Hanzl, M. (2007). Information technology as a tool for public participation in urban planning: a review of experiments and potentials. Des. Stud. 28 (3), 289–307. doi:10.1016/j.destud.2007.02.003
Holling, C. S. (1973). Resilience and stability of ecological systems. Annu. Rev. Ecol. Syst. 4, 1–23. doi:10.1146/annurev.es.04.110173.000245
Houzangbe, S., Masson, D., Fleury, S., Gómez Jáuregui, D. A., Legardeur, J., Richir, S., et al. (2022). Is virtual reality the solution? A comparison between 3D and 2D creative sketching tools in the early design process. Front. Virtual Real. 3. doi:10.3389/frvir.2022.958223
Ives, C. D., Lentini, P. E., Threlfall, C. G., Ikin, K., Shanahan, D. F., Garrard, G. E., et al. (2016). Cities are hotspots for threatened species. Glob. Ecol. Biogeogr. 25 (1), 117–126. doi:10.1111/geb.12404
Kabisch, N., Frantzeskaki, N., Pauleit, S., Naumann, S., Davis, M., Artmann, M., et al. (2016). Nature-based solutions to climate change mitigation and adaptation in urban areas: perspectives on indicators, knowledge gaps, barriers, and opportunities for action. Ecol. Soc. 21 (2), art39. doi:10.5751/ES-08373-210239
Khoshkar, S., Hammer, M., Borgström, S., and Balfors, B. (2020). Ways forward for advancing ecosystem services in municipal planning—experiences from Stockholm county. Land 9 (9), 296. doi:10.3390/land9090296
Levin, S., Xepapadeas, T., Crépin, A.-S., Norberg, J., De Zeeuw, A., Folke, C., et al. (2012). Social-ecological systems as complex adaptive systems: modeling and policy implications. Environ. Dev. Econ. 18, 111–132. doi:10.1017/S1355770X12000460
MA (2005). “Millennium ecosystem assessment—ecosystems and human wellbeing: synthesis,”. United Nations: Island Press. Available at: https://www.millenniumassessment.org/documents/document.356.aspx.pdf (Accessed November 06 2023).
Maalsen, S., Wolifson, P., and Dowling, R. (2023). Gender in the Australian innovation ecosystem: planning smart cities for men. Gend. Place Cult. 30 (2), 299–320. doi:10.1080/0966369X.2022.2053068
Manning, P., van der Plas, F., Soliveres, S., Allan, E., Maestre, F. T., Mace, G., et al. (2018). Redefining ecosystem multifunctionality. Nat. Ecol. and Evol. 2 (3), 427–436. doi:10.1038/s41559-017-0461-7
Masterson, V., Tengö, M., and Spierenburg, M. (2017). Competing Place Meanings in Complex Landscapes: A Social–Ecological Approach to Unpacking Community Conservation Outcomes on the Wild Coast, South Africa. Soc. Nat. Resour. 30, 1442–1457. doi:10.1080/08941920.2017.1347975
Matthews, T., Lo, A. Y., and Byrne, J. A. (2015). Reconceptualizing green infrastructure for climate change adaptation: barriers to adoption and drivers for uptake by spatial planners. Landsc. Urban Plan. 138, 155–163. doi:10.1016/j.landurbplan.2015.02.010
Meerow, S., Pajouhesh, P., and Miller, T. R. (2019). Social equity in urban resilience planning. Local Environ. 24 (9), 793–808. doi:10.1080/13549839.2019.1645103
Meta Technologies LLC (2021). Oculus Go: stand-alone VR headset | Oculus. Available at: https://www.oculus.com/go/ (Accessed April 30 2023).
Nitoslawski, S. A., Wong-Stevens, K., Steenberg, J. W. N., Witherspoon, K., Nesbitt, L., and Konijnendijk van den Bosch, C. C. (2021). The digital forest: mapping a decade of knowledge on technological applications for forest ecosystems. Earth’s Future 9 (8), e2021EF002123. doi:10.1029/2021EF002123
Pascual, U., McElwee, P. D., Diamond, S. E., Ngo, H. T., Bai, X., Cheung, W. W. L., et al. (2022). Governing for transformative change across the biodiversity–climate–society nexus. BioScience 72 (7), 684–704. doi:10.1093/biosci/biac031
Puppim de Oliveira, J. A., Bellezoni, R. A., Shih, W.-Y., and Bayulken, B. (2022). Innovations in urban green and blue infrastructure: tackling local and global challenges in cities. J. Clean. Prod. 362, 132355. doi:10.1016/j.jclepro.2022.132355
Regeringskansliet (2018). Strategi för Levande städer – politik för en hållbar stadsutveckling Regeringens skrivelse 2017/18. Available at: https://www.regeringen.se/contentassets/b5640fd317d04929990610e1a20a5383/171823000webb.pdf (Accessed February 09 2024).230, 1–63.
Rigolon, A. (2016). A complex landscape of inequity in access to urban parks: a literature review. Landsc. Urban Plan. 153, 160–169. doi:10.1016/j.landurbplan.2016.05.017
Sällström, P. M. (2000). Att studera ljud och arkitektur. Nordic Archit. Res. Assoc. 13 (1–2), 79–89.
Sanchez-Sepulveda, M., Fonseca, D., Franquesa, J., and Redondo, E. (2019a). Virtual interactive innovations applied for digital urban transformations. Mixed approach. Future Gener. Comput. Syst. 91, 371–381. doi:10.1016/J.FUTURE.2018.08.016
Sanchez-Sepulveda, M. V., Torres-Kompen, R., Fonseca, D., and Franquesa-Sanchez, J. (2019b). Methodologies of learning served by virtual reality: a case study in urban interventions. Appl. Sci. 9 (23), 5161. doi:10.3390/app9235161
Sassen, S. (2010). Cities are at the center of our environmental future. S. A. P. I. E.N.S 2 (3), 72–83. doi:10.16924/revinge.31.8
Seifert, N., Mühlhaus, M., and Petzold, F. (2020). Urban strategy playground: rethinking the urban planner’s toolbox. Int. J. Archit. Comput. 18 (1), 20–40. doi:10.1177/1478077119894477
Slater, M. (2009). Place illusion and plausibility can lead to realistic behaviour in immersive virtual environments. Philosophical Trans. R. Soc. B Biol. Sci. 364 (1535), 3549–3557. doi:10.1098/rstb.2009.0138
Stauskis, G. (2014). Development of methods and practices of virtual reality as a tool for participatory urban planning: a case study of Vilnius City as an example for improving environmental, social and energy sustainability. Energy, Sustain. Soc. 4, 7. doi:10.1186/2192-0567-4-7
Stedman, R. C. (2002). Toward a social psychology of place—predicting behavior from place-based cognitions, attitude, and identity. Environ. Behav. 34 (5), 561–581. doi:10.1177/0013916502034005001
Steel, B., Ersin, E. H., and McNicholas, R. (2015). In the eyes of the animal. MIT – Docubase. Available at: https://docubase.mit.edu/project/in-the-eyes-of-the-animal/ (Accessed November 10 2023).
Subramanian, A., Barnes, J., Vemulapalli, N., and Chhawri, S. (2017). “Virtual reality museum of consumer technologies,” in Advances in human factors, business management, training and education. Editors J. I. Kantola, T. Barath, S. Nazir, and T. Andre (Springer, Cham: Springer International Publishing), 549–560.
Suneson, K., Allwood, C. M., Paulin, D., Heldal, I., Roupé, M., Johansson, M., et al. (2008). Virtual reality as a new tool in the city planning process. Tsinghua Sci. Technol. 13 (S1), 255–260. doi:10.1016/s1007-0214(08)70158-5
Sutherland, I. E. (1968). “A head-mounted three dimensional display,” in AFIPS ’68 (Fall, Part I): Proceedings of the December 9-11, 1968, Fall Joint Computer Conference, Part I, San Francisco California, December 09, 1968 (New York, NY: ACM Press), 757–764.33
Tengö, M., Brondizio, E. S., Elmqvist, T., Malmer, P., and Spierenburg, M. (2014). Connecting diverse knowledge systems for enhanced ecosystem governance: the multiple evidence base approach. Ambio 43 (5), 579–591. doi:10.1007/s13280-014-0501-3
United Nations (2015). Transforming our world: the 2030 agenda for sustainable development A/RES/70/1. New York: UN General Assembly, 3–40. Available at: https://sdgs.un.org/sites/default/files/publications/21252030%20Agenda%20for%20Sustainable%20Development%20web.pdf (Accessed September 25, 2015).
Vainio, T. (2016). Motivations, results and the role of technology in participatory design research during 2000’s – a review in the field of architecture and urban planning. Archit. Urban Plan. 11 (1), 14–18. doi:10.1515/aup-2016-0002
van der Jagt, A. P. N., Smith, M., Ambrose-Oji, B., Konijnendijk, C. C., Giannico, V., Haase, D., et al. (2019). Co-creating urban green infrastructure connecting people and nature: a guiding framework and approach. J. Environ. Manag. 233, 757–767. doi:10.1016/j.jenvman.2018.09.083
Vukmirovic, M., Gavrilovic, S., and Stojanovic, D. (2019). The improvement of the comfort of public spaces as a local initiative in coping with climate change. Sustain. Switz. 11 (23), 6546. doi:10.3390/su11236546
Wallhagen, M. (2016). “Environmental assessment tools for neighbourhoods and buildings in relation to environment, architecture, and architects,”. Stockholm, Sweden: KTH Royal Institute of Technology. PhD thesis.
Wolf, M., Söbke, H., and Wehking, F. (2020). “Mixed reality media-enabled public participation in urban planning,” in Augmented reality and virtual reality: changing realities in a dynamic world. Editors T. Jung, M. C. tom Dieck, and P. A. Rauschnabel (Springer, Cham: Springer International Publishing), 125–138.
Zhang, J., and Tan, P. Y. (2023). Assessment of spatial equity of urban park distribution from the perspective of supply-demand interactions. Urban For. and Urban Green. 80, 127827. doi:10.1016/j.ufug.2022.127827
Keywords: virtual reality, urban ecosystem services, green and blue infrastructure, urban planning, urban design and management, adaptive capacity, urban resilience, urban sustainability
Citation: Schewenius M and Wallhagen M (2024) Virtual Reality in planning, design, and management of urban green and blue infrastructure. Front. Virtual Real. 5:1432556. doi: 10.3389/frvir.2024.1432556
Received: 14 May 2024; Accepted: 23 August 2024;
Published: 09 September 2024.
Edited by:
Kay Marie Stanney, Design Interactive, United StatesReviewed by:
Peter Eachus, University of Salford, United KingdomRachel Cooper, Lancaster University, United Kingdom
Copyright © 2024 Schewenius and Wallhagen. This is an open-access article distributed under the terms of the Creative Commons Attribution License (CC BY). The use, distribution or reproduction in other forums is permitted, provided the original author(s) and the copyright owner(s) are credited and that the original publication in this journal is cited, in accordance with accepted academic practice. No use, distribution or reproduction is permitted which does not comply with these terms.
*Correspondence: Maria Schewenius, bWFyaWEuc2NoZXdlbml1c0BzdS5zZQ==