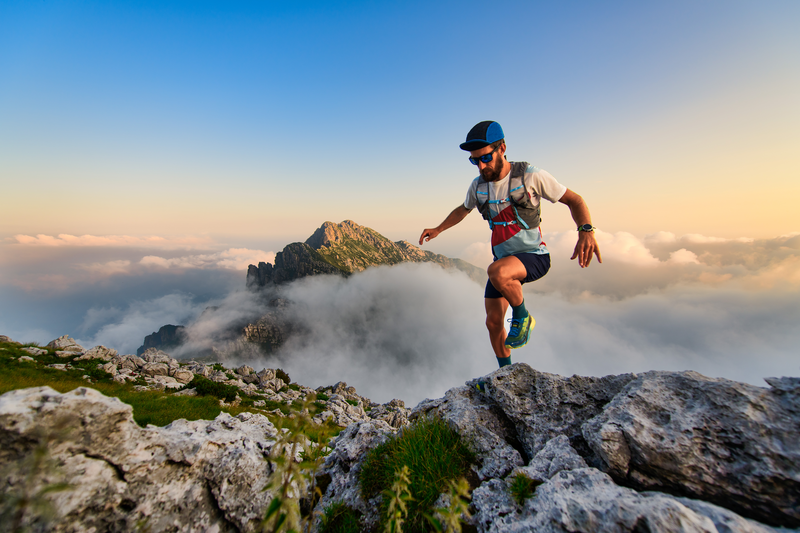
95% of researchers rate our articles as excellent or good
Learn more about the work of our research integrity team to safeguard the quality of each article we publish.
Find out more
REVIEW article
Front. Vet. Sci. , 02 April 2025
Sec. Animal Reproduction - Theriogenology
Volume 12 - 2025 | https://doi.org/10.3389/fvets.2025.1554771
This article is part of the Research Topic Innovative Strategies for Improving Sperm Lifespan during Storage View all 3 articles
Preservation of sperm significantly contributes to the advancement of assisted reproductive technologies, genetic conservation and improvement efforts, and precision breeding of livestock. This review distills knowledge from the existing information and emerging patterns in the field of buck sperm cryopreservation. The primary focus is on the challenges and opportunities associated with improving extender formulations and freezing techniques in order to enhance the vitality of sperm after thawing and to increase the potential for conception. This review assesses the efficacy and limitations of conventional extenders derived from egg yolk or soybean lecithin, and the adverse impacts of seminal plasma enzymes on sperm quality during the processes of chilling and cryopreservation. Significant progress has been made in the fields of molecular biology namely lipidomics, proteomics, metabolomics, DNA methylation providing valuable knowledge regarding the unique reactions of sperm to cryopreservation. The utilization of the “omics” technologies has shown intricate molecular transformation that occur in sperm during freezing and thawing. Moreover, detection of molecular biomarkers that indicate the quality of sperm and their ability to withstand freezing provides opportunities to choose the best sperm samples for cryopreservation. This, in turn, enhances the results of artificial insemination and genetic conservation endeavors. This review emphasizes the necessity for adopting a comprehensive approach that combines molecular and cellular knowledge with practical methods in the field of sperm cryopreservation to ensure production of goats as major food animals in the global scale.
Goats, among the first domesticated animals, have coexisted with humans for thousands of years (1). They are globally distributed due to their adaptability to various climates (2). Primarily valued for meat, milk, fiber, and skin, goats are especially crucial where land is scarce (3). They are present on all continents except Antarctica, demonstrating their adaptability (4). Their importance to small-scale farmers, particularly those in rural areas with limited resources, is significant due to their small size and adaptability. Global goat population has surpassed one billion, doubling in the past 30 years due to increased use by smallholder farmers (5, 6). Advances in genetics have improved goat farming efficiency, focusing on meat and milk production (7). Sustainable farming must integrate productivity, animal health, and food security through public-private partnerships and research (8). Therefore, enhancing these practices can significantly benefit sustainable farming. As such, goats are vital globally, and addressing challenges is crucial for improving nutrition and economic stability through food animals (9).
Buck fertility is the ability of a male goat to produce viable sperm capable of successful fertilization, which is essential for goat reproduction and affects herd productivity as well as genetic progress. Male fertility in artificial insemination programs is linked to sperm fertilizing capability and genetics. Alpine bucks are recognized for their superior traits such as better semen volume, concentration, and motility, making them ideal for breeding programs (10). Even bucks that pass fertility tests may exhibit subfertility (11), which necessitates detailed sperm structure and function analysis. Bucks also induce estrus in does, enhancing overall herd productivity (12). Artificial insemination with frozen semen has improved performance in Alpine goats, showcasing the importance of superior genetics (13). Selecting high-quality bucks is crucial for improving fertility (14). Artificial insemination with frozen-thawed semen has been successful in maintaining viable sperm and achieving good conception rates (15). It has also been effective in enhancing breeding outcomes in synchronized goats (16). Nutritional and environmental factors, such as seasonal variations and heat stress, significantly impact buck fertility. Proper nutrition with balanced protein and energy supports testicular size and sperm production (17) while heat stress can lead to DNA damage and altered gene expression, and reducing fertility (18, 19). Managing these factors and including in the buck soundness exam are critically important for maintaining fertility and productivity.
Cryobiology examines the limits of life under freezing conditions and how organisms can be preserved for revival. The critical method of cryopreservation involves preserving biological materials, including organelles, for long periods of time at low temperatures. This technique is essential in fields such as artificial insemination, organ transplantation, and long-term cell storage (20–22). Cryoprotectants prevent formation and damaging effects of ice crystals on molecular and cellular anatomy of sperm during freezing and thawing (23), with natural examples seen in species such as frogs that produce cryoprotectants to survive winter (24, 25). Alaska wood frogs, for instance, synthesize higher amounts of cryoprotectants to survive freeze-thaw cycles (26). Cryopreservation is also used as a conservation tool for endangered species such as the Louisiana pine snake, where sperm viability post-thaw has been studied (27). Cryopreservation is also utilized for microbial organisms in teaching, research, and industry (28). Sperm cryopreservation allows superior donor genetics to be used in artificial insemination programs (29), with slow freezing and vitrification as common methods. New cryoprotectants such as trehalose enhance cell preservation (23, 30). Age affects sperm quality during cryopreservation, with older bulls showing reduced motility and oxidative damage (31). Cryoprotectants combined with antioxidants preserve sperm integrity (32–34), and advanced computational tools help improve post-thaw sperm quality (35, 36). Studying the presence of gene products and protein expression in cryopreserved sperm provides insights into fertility pathways and the potential effects of cryopreservation on gene expression after fertilization (37, 38). Supplementing extenders with trehalose improves buck sperm freeze-thawing tolerance (39, 40), and shows promise for improving sperm cryosurvival in bulls, bucks, and rams (41, 42).
Semen extenders are vital for sperm preservation, supporting fertilization by maintaining sperm metabolism, regulating pH, preventing bacterial contamination, and reducing cryogenic damage (43, 44). They regulate pH (45), serve as an energy source (46), provide antioxidant support (47), contain antibiotics to prevent contamination (48), and help mitigate freezing shock (49). Extenders are used for both short-term chilling and long-term cryopreservation (50), with common ingredients such as egg yolk, skimmed milk (51), and plant-based alternatives like soybean lecithin (52). Egg yolk extenders form complexes with Bovine Seminal Plasma proteins (BSP proteins) to preserve sperm motility (53) while skimmed milk regulates pH and chelates heavy metals (54). Honey, due to its hyperosmotic properties, enhances sperm motility and reduces abnormalities (55, 56). Fish oil, incorporated into extenders, improves semen performance during freeze-thawing and artificial insemination (43). Soybean lecithin is a sanitary alternative, protecting against freezing shock (57).
Cryopreservation stimulates Reactive Oxygen Species (ROS) generation, such as superoxide anions (), hydrogen peroxide (H2O2), and hydroxyl radicals (OH•) that trigger lipid peroxidation (58, 59) and cause irreversible damage to sperm membranes, mitochondria, and DNA. Superoxide dismutase (SOD), a defense enzyme, catalyzes the conversion of to H2O2 and O2 to avert mitochondrial dysfunction and premature capacitation (60). Yet, in the event of no neutralization, H2O2 is implicated in the Fenton reaction, forming highly reactive OH• in the presence of Fe2+ that initiate lipid peroxidation by targeting polyunsaturated fatty acids (PUFAs) such as docosahexaenoic acid (DHA) in sperm membranes, resulting in loss of membrane integrity, impaired motility, and DNA fragmentation (61, 62).
At the molecular level, antioxidants such as Epididimal Glutathione Peroxidase 5 (GPx5) play a critical role in detoxifying reactive intermediates and protecting DNA, lipids, and proteins from oxidative damage (63). At the cellular level, enzymatic antioxidants (e.g., SOD, catalase) and non-enzymatic antioxidants (e.g., vitamins C and E) help preserve mitochondrial function, essential for ATP production and motility (64, 65). To counter the accumulation of H2O2 two of the main intracellular protective enzymes, catalase (CAT) and glutathione peroxidase (GPx), are able to degrade H2O2 into water and O2 (66) or utilize glutathione (GSH) to reduce both H2O2 and lipid hydroperoxides (LOOH), thus successfully targeting the peroxidation chain reaction. SOD is the first line of defense against ROS, specifically radicals. It catalyzes the dismutation of into H2O2 and molecular oxygen, thereby reducing the potential for cellular damage caused by these radicals (67). Adding SOD to semen extenders enhances sperm quality across species in a concentration-dependent manner. In bulls, 100–200 IU/mL SOD improves post-thaw motility and viability (68). In rams, 1–2 mM/mL SOD enhances sperm quality in both frozen and chilled semen (69). In boars, 150–300 IU/mL SOD, alone or with catalase, increase motility, viability, and embryo production while reducing ROS (70). CAT complements the action of SOD by converting H2O2, a byproduct of dismutation, into water and oxygen. This enzymatic activity is vital in preventing oxidative damage to sperm membranes and DNA. Research indicates that CAT, along with SOD, is crucial for maintaining sperm motility and viability under oxidative stress conditions (71). Adding CAT to semen extenders improves sperm quality in a concentration-dependent manner across species. In bulls, 100–200 IU/mL CAT in tris-egg yolk extenders enhances sperm viability post-thaw, while no significant effect is observed in citrate-egg yolk extenders (72). In boars, 400 U/mL CAT has been reported to improve total sperm motility, while 200–400 U/mL reduces H2O2 levels (73). In rams, 50 mM trehalose alone or in combination with 400 μg CAT has been reported to improve post-thaw sperm motility (74). In humans, 200–400 IU/mL CAT has been reported to reduce ROS levels and protect sperm viability, motility, mitochondrial membrane potential, and DNA integrity during cryopreservation (75). Following this, CAT and GPx further detoxify H2O2, converting it into water and oxygen. CAT is particularly effective at high concentrations of H2O2, while GPx utilizes glutathione to reduce H2O2 and lipid hydroperoxides, thus preventing lipid peroxidation (76). Adding GPx to semen extenders enhances sperm quality in a species- and concentration-dependent manner. In stallions, 1–5 IU/mL GPx improves acrosome integrity post-thaw but has no significant effect on motility parameters (77). During chilled storage, supplementation with 15 IU/mL GPx, along with SOD and CAT, preserves motility and viability while reducing caspase-3 activation and DNA fragmentation (78). In bulls, 1.0 mM GPx in a nano lecithin-based extender improves plasma membrane integrity, reduces lipid peroxidation, and enhances blastocyst formation in vitro (47). The interplay between these enzymes is crucial for maintaining cellular redox balance, especially in spermatozoa, which are particularly susceptible to oxidative stress due to their high polyunsaturated fatty acid content in membranes (79). Collectively, these enzymes work synergistically to preserve the sperm's redox balance while preventing oxidative damage and maintaining acrosomal integrity, mitochondrial activity, and fertilization ability (80, 81). Since cryopreservation significantly depletes endogenous antioxidant activity, potent antioxidant supplementation in extenders could provide a breakthrough in improving buck sperm cryosurvival and post-thaw fertilization potential (82, 83).
Vitamins C and E have also been shown to donate some electrons and provide powerful antioxidant effects by neutralizing free radicals. Selenium and zinc are equally essential as vitamins in maintaining the antioxidant defense system and facilitating the action of enzymatic antioxidants. Such minerals act as coenzymes in biochemical cascade reactions of multiple metabolic enzymatic reactions and play a significant role in the overall antioxidant defense system. Beta-carotene and lycopene are also parts of this family, providing strong antioxidant activity in protecting lipids from peroxidation. In addition, flavonoids, which are largely available in fruits and vegetables, possess antioxidant properties and lead to reduced oxidative stress (84). Adding antioxidants to semen extenders enhances post-thaw sperm quality in a species- and concentration-dependent manner. In stallions, α-tocopherol (0.5–2 mM) reduces lipid peroxidation, while ascorbic acid (0.9–1.8 g/L) improves membrane integrity and stability (85). In Bhadawari bulls, vitamin E (5 mM) and vitamin C (5 mM) individually improve post-thaw sperm parameters, with their combination (5 mM + 5 mM) providing the highest protection against oxidative stress and cold shock (86). In rams, 0.3 mM α-Tocopherol significantly improves post-thaw motility, viability, normal sperm percentage, and functional integrity, while low-dose vitamin C (0.1 mM) also enhances sperm quality but leads to more secondary abnormalities (87).
These mechanisms also protect the lipid-rich sperm membrane, composed of polyunsaturated fatty acids, from oxidative damage caused by lipid peroxidation, thereby ensuring the integrity and functionality of sperm (88). Commercial extenders such as Triladyl®, Ovipro®, AndroMed®, and Steridyl® are widely used, with plant-based extenders minimizing contamination risk (89–91). Nanoparticles improve sperm cryopreservation by mitigating oxidative stress through their antioxidant properties, scavenging ROS, and stabilizing cell membranes (92, 93). Metal-based nanoparticles, such as zinc oxide (ZnO) and selenium oxide (SeO), enhance sperm motility, plasma membrane integrity, and DNA stability while reducing oxidative damage markers such as malondialdehyde (94, 95).
Lipid-based nanoparticles further improve post-thaw sperm quality by providing a protective barrier and stabilizing cellular membranes (96). Additionally, nanoparticles facilitate sperm purification and advanced techniques such as chromatin protection and selective sperm population enrichment, supporting innovative reproductive applications (97, 98). Liposomes, as phospholipid bilayer vesicles, enhance sperm cryopreservation by encapsulating antioxidants and cryoprotectants, thereby reducing oxidative stress, stabilizing plasma membranes, and improving post-thaw sperm viability (92, 99). They also offer alternatives to animal-derived extenders such as egg yolk, addressing biosecurity and ethical concerns, while liposome-based formulations with trehalose further improve the rheological properties of cryopreservation media (100, 101). These multifaceted roles position liposomes as a key innovation in reproductive biotechnology, enhancing sperm functionality across species (102, 103).
Success of sperm cryopreservation is significantly influenced by genetic variations, as molecular markers have been linked to semen freezability. For instance, in boars, specific genetic markers have been associated with variations in post-thaw semen quality, underscoring the role of genetics in cryopreservation outcomes (104). The composition of sperm membranes is also critical, as changes during cryopreservation, such as the loss of membrane sterols, can compromise sperm structural integrity and fertilizing potential (105–107). The use of cryoprotectants during freezing helps minimize cryoinjury and cold damage, improving motility after thawing (108). Molecular markers such as heat shock proteins 70 (HSP70) and peroxiredoxin 6 (PRDX6) have been proposed as indicators of sperm freezability, with higher levels correlating with improved semen quality and fertility (109, 110).
Metabolomic approaches revealed seminal plasma metabolites associated with sperm cryo-tolerance, offering potential for optimizing cryopreservation techniques (111). Sperm morphology, particularly flagellum size, has been linked to freezability, with larger flagella being associated with reduced cryosurvival (112). Cryopreservation impacts sperm cellular anatomy and physiology, including changes in sperm characteristics and mRNA downregulation, which are vital for fertilization and early embryo development (95, 113). Additionally, the utilization of supervised learning methods has been suggested for characterizing sperm population structures related to freezability, providing insights into the factors influencing sperm cryo-survival (114). Supervised learning, a branch of artificial intelligence, relies on training algorithms with labeled data to detect patterns and predict outcomes from new datasets. Techniques such as deep learning models, such as convolutional neural networks (CNNs), have been applied to sperm analysis, providing more accurate and consistent evaluations of morphology and motility compared to traditional methods (115–117). Furthermore, Artificial intelligence-powered systems enable rapid analysis of large datasets, facilitating predictions of sperm fertilization potential and identifying factors affecting sperm cryo-survivability (114, 118, 119). In sperm cryopreservation, supervised learning algorithms predict post-thaw motility and viability by leveraging large datasets, which helps refine freezing protocols and improve success rates in artificial insemination (116, 120).
Cryopreservation significantly impacts sperm physiology, including motility, viability, capacitation, acrosome reaction, and fertilization potential in mammals. Motility is critical for sperm function in the female reproductive tract, and it can decrease from 90 to 95% pre-cryopreservation to around 75% post-thaw in bulls (121). This process also compromises acrosome integrity and reduces fertilization success due to impaired acrosome reactions humans and alpacas (122, 123). Cryopreservation induces oxidative stress, DNA damage, and changes in gene expression, which reduce embryo quality and development (124, 125). These changes can influence gene expression that may compromise the quality and developmental potential of embryos fertilized with cryopreserved sperm. The negative impact can potentially be due to modifications in sperm proteins induced by the cryopreservation process as well (126). Furthermore, the use of cryopreserved sperm can reduce fertilization rates and blastocyst formation in in vitro fertilization (IVF) and intracytoplasmic sperm injection (ICSI) procedures (127).
Premature capacitation and increased DNA fragmentation are linked to oxidative damage and mitochondrial dysfunction (128, 129). Structural integrity of the sperm plasma membrane is significantly compromised during the freeze-thaw cycles, primarily due to osmotic stress, which causes mechanical strain and destabilization of the membrane. Although cryoprotectants such as glycerol provide partial protection against these damages by reducing ice crystal formation and osmotic imbalances, they are not entirely effective in preserving the full functionality and structural integrity of the membranes (130–133). Additionally, cryopreservation alters sperm's epigenetic landscape, including DNA methylation, potentially affecting gene regulation in embryos (134). These disruptions highlight the need for improved cryopreservation protocols to maintain sperm function and embryo development in Assisted Reproductive Technologies (ARTs).
Spermatogenesis is a complex and essential process for sexual reproduction, where diploid spermatogonia undergo proliferative divisions and differentiate into mature haploid spermatozoa through meiotic phases and sperm cell maturation (135). This transformation occurs within the seminiferous epithelium in regulated cycles in the buck testes. Initially, round spermatids are formed and then elongated along Sertoli cells to produce elongated spermatids. This process results from coordinated interactions among Sertoli cells, type A and B spermatogonia, and primary spermatocytes, which eventually develop into haploid spermatids. Spermatogenesis in bucks typically spans 47.7 days, culminating in the release of elongated spermatids into the seminiferous tubules, where histones are replaced by protamines (136). Buck sperm exhibit unique molecular characteristics, including distinct lipid compositions and proteomic profiles, which influence cryopreservation outcomes, motility, and viability (111, 137, 138). The sperm morphology, particularly the dimensions of the head and midpiece, differs from those of other mammals (Table 1) (139, 140). Regulation of gene expression during spermatogenesis is controlled by transcriptional, post-transcriptional, and epigenetic mechanisms, which are essential for sperm maturation and function (141).
Cryopreservation-induced sperm damage extends beyond oxidative stress and membrane destabilization, significantly compromising DNA integrity through multiple mechanisms. The primary causes of DNA fragmentation in buck sperm include species-specific genetic characteristics, osmotic stress, and apoptotic-like pathways, differing in severity from human and cattle sperm (142, 143). Buck sperm is particularly vulnerable due to its high PUFA content, which makes it more susceptible to lipid peroxidation-derived aldehydes such as malondialdehyde (MDA), further exacerbating DNA fragmentation (144, 145). Additionally, buck sperm chromatin is less compact and retains more histones than human and bovine sperm, increasing its susceptibility to shearing forces during freezing-thawing (146, 147). Osmotic stress during cryopreservation induces torsional strain on DNA, leading to increased single- and double-strand breaks (148, 149). Moreover, mitochondrial apoptotic pathways may be triggered, where excessive ROS accumulation activates caspases and endonucleases, further degrading DNA—an effect more pronounced in bucks due to their higher metabolic activity and mitochondrial ROS production (150, 151). These vulnerabilities necessitate cryoprotectant strategies focused on DNA preservation, incorporating antioxidants such as GSH, vitamin E, polyphenols, seleno-organic molecules, and flavanoids which have been shown to mitigate cryo-induced genetic damage (152–156). Given these unique susceptibilities, buck sperm cryopreservation requires distinct DNA optimization strategies compared to human and bovine sperm.
Buck sperm exhibits distinct physiological characteristics compared to bull sperm, particularly in proteomic composition, motility, and reproductive performance (8). Zhu et al. (147) identified 238 differentially abundant proteins in buck sperm, involved in energy production and oxidative stress mitigation. Cryopreservation significantly reduces buck sperm motility and viability, primarily due to diminished membrane integrity and mitochondrial dysfunction, which impair energy production and flagellar movement (157, 158). Capacitation and acrosome reactions in buck sperm differ from that of bull sperm in that they occur more rapidly, are more sensitive to environmental conditions such as pH and ionic changes, show greater vulnerability to oxidative stress, and experience increased plasma membrane damage during cryopreservation, affecting fertilization success. Because of these differences, extenders as well as the processes of freezing and thawing might require refined compositions and methods to optimize outcomes (147). Nutritional factors, including dietary supplementation, further influence buck sperm quality, improving motility and membrane integrity (157). As such, further research is needed to understand better the unique physiological and molecular adaptations of buck sperm, particularly in response to oxidative stress, cryopreservation, and environmental factors affecting fertilization success.
Researchers employing proteomic and lipidomic analyses have identified key molecules affecting goat sperm viability after cryopreservation (138, 159). Heat shock proteins such as HSP70 and HSP90 prevent protein denaturation during freezing, while antioxidant enzymes such as SOD and catalase protect against oxidative stress (160, 161). Proteins such as proline dehydrogenase (PRODH) enhance membrane stability by scavenging ROS and supporting cellular structures, while ubiquinone (coenzyme Q10) acts as an antioxidant to reduce oxidative stress and preserve mitochondrial function, significantly improving motility and membrane integrity (29, 162). Differentially expressed proteins (DEPs) linked to energy metabolism and oxidative stress response further contribute to sperm cryotolerance (138). The fluidity of goat sperm plasma membranes is greatly decreased after epididymal maturation, which is characterized by higher lipid phase fluidity in caput (immature) than in cauda (mature) sperm membranes, as measured with pyrene and 1,6-Diphenyl-1,3,5-Hexatriene (DPH) lipid descriptors. Cholesterol also enhances membrane stability and reduces cryoinjury, supporting sperm viability post-thaw (163). Future research should quantify specific alterations in lipid and protein profiles to refine cryopreservation protocols and optimize reproductive outcomes in goats.
Cryopreservation of buck sperm is absolutely important for both fundamental and applied animal reproduction science (Figure 1), allowing long-term storage and transport of the genetic material. Semen collection methods significantly impact sperm cryoresistance, with different techniques influencing sperm survival during cryopreservation (164). Extenders such as Tris-egg yolk-glucose and non-fat dried skimmed milk are commonly used to protect sperm during freezing and thawing (165). Tris-egg yolk-glucose provides essential nutrients and energy, while non-fat dried skimmed milk supplies proteins and lipids necessary for maintaining sperm viability. Glycerol prevents ice crystal formation, which is the key to preserving cellular integrity during freezing (166). Cholesterol-loaded cyclodextrins improve membrane integrity, increasing both viability and motility in buck and bull sperm (167). Fruit juices, such as pineapple (Ananas comosus), orange (Citrus sinensis), and cucumber (Cucumis sativus), contain antioxidants like carotenoids, vitamins (C and E), phenolic compounds, and flavonoids, which have been shown to enhance motility and reduce sperm abnormalities by mitigating oxidative stress (168). The success of buck sperm cryopreservation largely depends not only on the extender composition, as each component plays a crucial role in protecting sperm cells, but also on the characteristics of the ejaculate itself, including the presence of seminal plasma and seasonal variations in sperm freezability throughout the year (169, 170). For instance, cholesterol-loaded cyclodextrins improve membrane integrity, leading to better post-thaw viability (167). Metabolomics studies reveal that trehalose supplementation (60–100 mM) enhances post-thaw sperm motility, viability, mitochondrial activity, and DNA integrity when combined with low concentrations of cryoprotectants (36, 171). Trehalose exerts its cryoprotective effects by stabilizing sperm plasma membrane phospholipids, preventing cellular dehydration, and reducing lipid peroxidation, thereby minimizing oxidative stress and preserving sperm function after cryopreservation (172).
Figure 1. Sperm cryopreservation protocol. The cryopreservation process of semen typically begins with the collection of semen using an artificial vagina. However, electroejaculation may be employed in cases where elite male animals are unable to mount due to conditions such as lameness, shyness, or limb abnormalities. The collected ejaculate is then evaluated for key quality parameters such as motility and morphology. Following this assessment, semen is diluted with appropriate extenders. The diluted semen is loaded into 0.25 or 0.5 mL straws and cooled to 5°C, followed by an equilibration at this temperature for ~3 h. During the freezing stage, temperature reduction occurs in distinct phases to minimize cellular damage and osmotic stress. Initially, the straws are cooled to −5 to −7°C to induce extracellular ice nucleation, preventing intracellular ice formation. This is followed by a controlled cooling phase, during which the temperature is gradually reduced at a rate of ~1°C per min until reaching −35°C. This step allows the cells to adapt to the temperature decrease, minimizing intracellular crystallization and membrane damage. The final phase involves rapid cooling to −196°C, which transitions the sperm cells into a vitrified state, halting all metabolic activities and preserving cellular integrity (204). Two main methods are employed during the freezing stage of cryopreservation. The first, conventional method involves placing the straws in a styrofoam box, 4–8 cm above the liquid nitrogen surface (with a nitrogen level of 5 cm) to be frozen in the nitrogen vapor for 15 min (Method A). The second method utilizes automatic freezing systems. These systems offer a wide range of temperatures, from +40°C to −180°C, and operate with precise cooling rates between 0.01°C and 60°C/min (Method B). Both methods aim to ensure the gradual adaptation of sperm cells to temperature changes, thus preserving their viability and motility. Following cryopreservation, the straws are transferred into liquid nitrogen (−196°C) for long-term storage.
Cryopreservation of buck sperm presents challenges in maintaining motility, plasma and acrosomal membrane integrity, mitochondrial membrane potential, and reducing ROS generation. Additionally, it leads to proteomic and metabolomic alterations due to structural damage, ultimately lowering sperm viability (171) (Figure 2). The choice of extender is a critical factor, as different extenders affect sperm quality in various ways (Table 2) (173). Sperm quality is also influenced by age, and supplementation with antioxidants has shown potential in improving these parameters (174). Re-adding seminal plasma post-thaw has had limited success, indicating the need for novel approaches (175). Innovative additives such as Mito-TEMPO have shown promise in improving sperm cryopreservation (176). Cholesterol supplementation has also been effective in enhancing cryosurvival (177). Factors such as semen collection methods, extender choice, and centrifugation all influence post-thaw sperm quality, emphasizing the need for standardized protocols (165). Antioxidant supplementation, such as cysteine, improves motility and viability, highlighting its potential in enhancing cryosurvival (178). Studies on tris and egg yolk concentrations further emphasize the importance of extender composition for post-thaw viability (179). Soy-based extenders have shown promise in improving semen freezability (180).
Figure 2. Sperm evaluation. (A) CASA (Computer-Assisted Sperm Analysis). (B) Acrosomal Membrane Integrity, B1: Intact acrosomal membrane, B2: Damaged acrosomal membrane. (C) Plasma Membrane Integrity, C1: Intact plasma membrane, C2: Damaged plasma membrane. (D) Mitochondrial membrane potential, D1: High mitochondrial membrane potential, D2: Low mitochondrial membrane potential, D3: No mitochondrial membrane potential. (E) Immunolocalization of Histones in Bovine Sperm Cells, E1: Negative control sperm cell incubated with only the secondary antibody, E2: Sperm cell with a medium level of histone fluorescence, E3: Sperm cell with a high level of histone fluorescence. (F) Reactive Oxygen Species (H2O2) and Viability, F1: Viable sperm without ROS, F2: Dead sperm with ROS, F3: Viable sperm with ROS. (G) Mitochondrial generation, G1: Live cells without stain, G2: Dead cells with green stain, G3: positive mitochondrial generation, G4: Dead and positive mitochondrial generation. (H) Proteomics. (I) Metabolomics.
Cryoprotectants such as trehalose play a protective role in preserving membrane integrity during freezing and thawing (181), and using soybean lecithin instead of egg yolk provides a safer alternative for buck semen cryopreservation (182). In Markhoz goats, 50–70 mM trehalose, alone or in combination with 3–6 mM pentoxifylline (PTX), has been reported to improve post-thaw sperm motility, viability, and chromatin integrity during cryopreservation (183). In Angora bucks, 50–75 mM trehalose has been reported to improve post-thaw sperm motility, while 50 mM trehalose resulted in the lowest percentage of total abnormalities (184). Further research is needed to optimize the techniques and antioxidants to better protect sperm during and after cryopreservation (32). Removing seminal plasma through centrifugation has improved sperm quality during cryopreservation (185), and supplementation with seminal plasma later in the cryopreservation process has enhanced post-thaw sperm quality (186). Washing procedures during cryopreservation affect sperm quality, underscoring the need for standardized protocols (166). Egg yolk concentration also influences sperm quality during cryopreservation, reinforcing the importance of extender composition (187). Natural additives, such as fulvic acids (188) and black cumin seed extract (189) have shown promise in improving motility and reducing oxidative stress in buck sperm. The cryoprotective effects of fruit juices on sperm viability further suggest their potential as natural cryoprotectants (168). Morphological changes post-cryopreservation emphasize the need for careful sperm selection for successful fertilization (10). Overcoming the challenges in buck sperm cryopreservation requires optimizing extender composition, antioxidant treatments, cryoprotectants, and semen processing techniques. By improving these factors, the success of cryopreservation can be increased, providing a valuable tool for genetic preservation in breeding programs.
Sperm cryopreservation causes molecular changes effecting sperm structure, function, and fertility. These effects vary by species due to unique sperm characteristics such as size, morphology, and membrane composition (190, 191). Factors affecting cryopreservation efficacy include cooling and thawing rates, sperm origin (ejaculate or epididymal), and individual variations (192–194). Cryopreservation lowers sperm viability and motility while increasing acrosome reaction rates (195). Proteomic analyses have identified biomarkers that influence sperm cryopreservation recovery, suggesting that deeper insights into these molecular species are crucial for understanding functional preservation in frozen-thawed sperms. Cryopreservation impacts proteins involved in sperm motility, viability, acrosomal integrity, ATP content, and capacitation. The use of “omics” technologies, especially proteomics, aids in optimizing freezing-thawing protocols to maintain sperm function and fertility (145, 147, 171). Different extenders used during cryopreservation contain cryoprotectants, antioxidants, and other agents to maintain sperm viability and function (190). However, while some freezing protocols yield satisfactory post-thaw sperm survival, others may result in reduced sperm viability due to suboptimal cryoprotectant composition or freezing and thawing conditions (196).
Lipidomics has been significant for studying lipid profiles, providing insights into how variations in lipid composition affect sperm quality and cryopreservation outcomes across different species. Lipidomic analysis can help predict cryopreservation success in ruminant sperm (145, 197, 198). Targeted lipidomics has been applied to uncover semen cryotolerance-related lipid profiles in Mediterranean Buffalo bulls, demonstrating its potential in evaluating sperm quality (199). The link between lipidomics and sperm fertility has been explored, focusing on cryotolerance and semen quality, identifying potential biomarkers in the spermatozoa lipidome that could be used for selecting high-fertility doses before freezing (200). Lipidomics has revealed the lipid composition of sperm cells from various species, highlighting the role of fatty acids in sperm function (201). Recent lipidomic studies have identified significant qualitative and quantitative differences in sperm membranes among ejaculates that led to pregnancy vs. those that did not (202), providing additional evidence for this approach's potential for characterizing gamete function associated with fertility outcomes. Lipidomics has also been proposed as a method to assess the spermatozoa and seminal plasma of males for fertility prediction (203).
There is a need to enhance sperm cryopreservation techniques to improve sperm survival during freezing and thawing processes and ensure successful fertilization across various species, thereby contributing to the progress of reproductive biotechnologies and conservation initiatives. Advances in molecular biology, lipidomics, proteomics, metabolomics, and DNA methylation are helpful in better understanding the male gamete during freezing and thawing, and in improving extender formulations and freezing techniques in buck sperm cryopreservation. Future advancements are expected to focus on minimizing the detrimental effects of seminal plasma enzymes through the improvement of extenders, as part of broader strategies to enhance sperm quality and viability post-cryopreservation. Furthermore, detection of biomarkers that indicate the quality of sperm and their ability to withstand freezing will aid in choosing the most suitable sperm samples for freezing. This will ultimately enhance the effectiveness of artificial insemination and genetic conservation initiatives in goats.
MB: Conceptualization, Data curation, Formal analysis, Investigation, Methodology, Project administration, Resources, Supervision, Validation, Visualization, Writing – original draft, Writing – review & editing. MH: Conceptualization, Data curation, Formal analysis, Investigation, Methodology, Resources, Supervision, Validation, Visualization, Writing – original draft, Writing – review & editing. OG: Formal analysis, Visualization, Writing – original draft, Writing – review & editing. RM: Formal analysis, Investigation, Visualization, Writing – original draft, Writing – review & editing. EM: Conceptualization, Data curation, Formal analysis, Funding acquisition, Investigation, Methodology, Project administration, Resources, Supervision, Validation, Writing – original draft, Writing – review & editing.
The author(s) declare that financial support was received for the research and/or publication of this article. This study was funded by the USDA-National Institute of Food and Agriculture, Grant Number: 2024-38821-42115 and Evans Allen Grant Number: to MH and EM. MB was funded through a competitive international postdoctoral fellowship by the Scientific and Technological Research Council of Türkiye (TUBITAK -1059B192200797).
The facilities and support necessary for conducting this research were provided by the International Goat Research Center, Prairie View A&M University, TX, United States.
The authors declare that the research was conducted in the absence of any commercial or financial relationships that could be construed as a potential conflict of interest.
The author(s) declare that no Gen AI was used in the creation of this manuscript.
All claims expressed in this article are solely those of the authors and do not necessarily represent those of their affiliated organizations, or those of the publisher, the editors and the reviewers. Any product that may be evaluated in this article, or claim that may be made by its manufacturer, is not guaranteed or endorsed by the publisher.
1. Monteiro AC, Lima MJ, Costa JM. Goat system productions: advantages and disadvantages to the animal, environment and farmer. In:Kukovics S, , editor. Goat Science. Rijeka: IntechOpen (2017). p. 351–66.
2. Aziz MA. Present status of the world goat populations and their productivity. Lohmann Inform. (2010) 45:42–52.
3. Sinn R, Rudenberg PG. Raising Goats for Milk and Meat. Arkansas: Heifer Project International (1983).
4. Hirst K. The History of the Domestication of Goats (Capra Hircus). New York, NY: Dotdash Meredith, Thought Co., (2019).
5. FAO. Fao Statistical Yearbook 2023. Rome: Food and Agriculture Organization of the United Nations (2023). doi: 10.4060/cc8166en
7. Rexroad C, Vallet J, Matukumalli LK, Reecy J, Bickhart D, Blackburn H, et al. Genome to phenome: improving animal health, production, and well-being—a new usda blueprint for animal genome research 2018–2027. Front Genet. (2019) 10:e00327. doi: 10.3389/fgene.2019.00327
8. Bodu M, Hitit M, Memili E. Harnessing the value of fertility biomarkers in bull sperm for buck sperm. Anim Reprod Sci. (2025) 272:107643. doi: 10.1016/j.anireprosci.2024.107643
9. Minnig A, Zufferey R, Thomann B, Zwygart S, Keil N, Schüpbach-Regula G, et al. Animal-based indicators for on-farm welfare assessment in goats. Animals. (2021) 11:3138. doi: 10.3390/ani11113138
10. Bogdaniuk A, Garkavii V, Petrushko M. Reproductive characteristics of saanen and alpine bucks. Anim Biol. (2023) 25:19. doi: 10.15407/animbiol25.03.019
11. Banwarth MR, DeAtley KL, Gifford CA, Schohr TK, McFarlane ZD. Bull selection and management in extensive rangeland production systems of California: a producer survey. Transl Anim Sci. (2022) 6:txac138. doi: 10.1093/tas/txac138
12. Gutierrez VA, Sánchez-Dávila F, Ledezma-Torres RA, Peterson S, Brenner EG, Luna-Palomera C, et al. The use of oxytocin to cause cervical dilation for transcervical insemination in nulliparous goats: improving pregnancy and kidding rates. Reprod Domest Anim. (2022) 57:886–92. doi: 10.1111/rda.14135
13. Agossou DJ, Koluman N. The effects of natural mating and artificial insemination using cryopreserved buck semen on reproductive performance in alpine goats. Arch Anim Breed. (2018) 61:459–61. doi: 10.5194/aab-61-459-2018
14. Nyaupane N, Gillespie J, McMillin K, Harrison R, Sitienei I. Selection of breeding stock by US meat goat producers. J Agric Appl Econ. (2017) 49:416–37. doi: 10.1017/aae.2017.6
15. Susilowati S, Triana IN, Sardjito T, Suprayogi TW, Wurlina W, Mustofa I. Effect of simmental bull seminal plasma protein in egg yolk-citrate extender on kacang buck semen fertility. Cryobiology. (2020) 97:20–7. doi: 10.1016/j.cryobiol.2020.10.013
16. Sharma A, Sood P. Cryopreservation and fertility of frozen thawed chegu goat semen. Indian J Anim Res. (2019) 53:1414–9. doi: 10.18805/ijar.B-3696
17. Guan Y, Malecki IA, Hawken PA, Linden MD, Martin GB. Under-nutrition reduces spermatogenic efficiency and sperm velocity, and increases sperm DNA damage in sexually mature male sheep. Anim Reprod Sci. (2014) 149:163–72. doi: 10.1016/j.anireprosci.2014.07.014
18. Delgadillo JA, Chemineau P. Abolition of the seasonal release of luteinizing hormone and testosterone in alpine male goats (Capra Hircus) by short photoperiodic cycles. Reproduction. (1992) 94:45–55. doi: 10.1530/jrf.0.0940045
19. Nardone A, Ronchi B, Lacetera N, Ranieri MS, Bernabucci U. Effects of climate changes on animal production and sustainability of livestock systems. Livest Sci. (2010) 130:57–69. doi: 10.1016/j.livsci.2010.02.011
20. Gao D, Critser JK. Mechanisms of cryoinjury in living cells. ILAR J. (2000) 41:187–96. doi: 10.1093/ilar.41.4.187
21. Sambu S. A Bayesian approach to optimizing cryopreservation protocols. PeerJ. (2015) 3:e1039. doi: 10.7717/peerj.1039
22. Chang T, Zhao G. Ice inhibition for cryopreservation: materials, strategies, and challenges. Adv Sci. (2021) 8:2002425. doi: 10.1002/advs.202002425
23. Yang J, Pan C, Zhang J, Sui X, Zhu Y, Wen C, et al. Exploring the potential of biocompatible osmoprotectants as highly efficient cryoprotectants. ACS Appl Mater Interfaces. (2017) 9:42516–24. doi: 10.1021/acsami.7b12189
24. Yang T, Zhang Y, Guo L, Li D, Liu A, Bilal M, et al. Antifreeze polysaccharides from wheat bran: the structural characterization and antifreeze mechanism. Biomacromolecules. (2024) 25:3877–92. doi: 10.1021/acs.biomac.3c00958
25. Churchill TA, Storey KB. Dehydration tolerance in wood frogs: a new perspective on development of amphibian freeze tolerance. Am J Physiol Regul Integr Comp Physiol. (1993) 265:R1324–R32. doi: 10.1152/ajpregu.1993.265.6.R1324
26. Larson DJ, Middle L, Vu H, Zhang W, Serianni AS, Duman J, et al. Wood frog adaptations to overwintering in Alaska: new limits to freezing tolerance. J Exp Biol. (2014) 217:2193–200. doi: 10.1242/jeb.101931
27. Sandfoss MR, Cantrell J, Roberts BM, Reichling S. Cryopreservation of sperm from an endangered snake with tests of post-thaw incubation in caffeine. Animals. (2022) 12:1824. doi: 10.3390/ani12141824
28. Prakash O, Nimonkar Y, Shouche YS. Practice and prospects of microbial preservation. FEMS Microbiol Lett. (2013) 339:1–9. doi: 10.1111/1574-6968.12034
29. Zhang W, Min L, Li Y, Lang Y, Hoque SAM, Adetunji AO, et al. Beneficial effect of proline supplementation on goat spermatozoa quality during cryopreservation. Animals. (2022) 12:2626. doi: 10.3390/ani12192626
30. Hu Y, Liu X, Liu F, Xie J, Zhu Q, Tan S. Trehalose in biomedical cryopreservation–properties, mechanisms, delivery methods, applications, benefits, and problems. ACS Biomater Sci Eng. (2023) 9:1190–204. doi: 10.1021/acsbiomaterials.2c01225
31. Trevizan JT, Carreira JT, Carvalho IR, Kipper BH, Nagata WB, Perri SHV, et al. Does lipid peroxidation and oxidative DNA damage differ in cryopreserved semen samples from young, adult and aged nellore bulls? Anim Reprod Sci. (2018) 195:8–15. doi: 10.1016/j.anireprosci.2018.04.071
32. Güngör S, Ata A, Inanc ME, Kastelic JP. Effect of various antioxidants and their combinations on bull semen cryopreservation. Turk J Vet Anim Sci. (2019) 43:590–5. doi: 10.3906/vet-1907-39
33. Bucak MN, Akalin PP, Keskin N, Bodu M, Öztürk AE, Ili P, et al. Combination of fetuin and trehalose in presence of low glycerol has beneficial effects on Freeze-Thawed Ram spermatozoa. Andrology. (2021) 9:1000–9. doi: 10.1111/andr.12974
34. Bucak MN, Keskin N, Bodu M, Bülbül B, Kirbaş M, Öztürk AE, et al. Combination of trehalose and low boron in presence of decreased glycerol improves post-Thawed Ram sperm parameters: a model study in boron research. Andrology. (2022) 10:585–94. doi: 10.1111/andr.13130
35. Tu F, Bhat M, Blondin P, Vincent P, Sharafi M, Benson JD. Machine learning and hypothesis driven optimization of bull semen cryopreservation media. Sci Rep. (2022) 12:22328. doi: 10.1038/s41598-022-25104-6
36. Öztürk AE, Bodu M, Bucak MN, Agir V, Özcan A, Keskin N, et al. The synergistic effect of trehalose and low concentrations of cryoprotectants can improve post-thaw ram sperm parameters. Cryobiology. (2020) 95:157–63. doi: 10.1016/j.cryobiol.2020.03.008
37. Ebenezer Samuel King JP, Sinha MK, Kumaresan A, Nag P, Das Gupta M, Arul Prakash M, et al. Cryopreservation process alters the expression of genes involved in pathways associated with the fertility of bull spermatozoa. Front Genet. (2022) 13:1025004. doi: 10.3389/fgene.2022.1025004
38. Gomes FP, Park R, Viana AG, Fernandez-Costa C, Topper E, Kaya A, et al. Protein signatures of seminal plasma from bulls with contrasting frozen-thawed sperm viability. Sci Rep. (2020) 10:14661. doi: 10.1038/s41598-020-71015-9
39. Ryu D-Y, Song W-H, Pang W-K, Yoon S-J, Rahman MS, Pang M-G. Freezability biomarkers in bull epididymal spermatozoa. Sci Rep. (2019) 9:12797. doi: 10.1038/s41598-019-49378-5
40. Karunakaran M, Konyak P, Mandal A, Mondal M, Bhakat C, Rai S, et al. Effect of trehalose-an impermeant cryoprotectant on cryopreservation of black bengal buck semen. Indian J Anim Res. (2019) 53:37–40. doi: 10.18805/ijar.B-3470
41. Posado R, García J, Gómez-Fernández J, Tomás C, Gómez-Izquierdo E, de Mercado E. Effect of the egta (ethylene glycol tetraacetic acid) supplementation in the freezing extender on quality of cryopreserved bull sperm. Int J Vet Sci Res. (2017) 3:18–22. doi: 10.17352/ijvsr.000017
42. Mocé E, Graham JK. Cholesterol-loaded cyclodextrins added to fresh bull ejaculates improve sperm cryosurvival. J Anim Sci. (2006) 84:826–33. doi: 10.2527/2006.844826x
43. Malik A, Jaelani A, Widaningsih N, Ni'mah GK, Raviani, Sakiman, et al. Effect of different concentration of fish oil in skim milk-egg yolk extenders on post- thawed semen qualities of kalang swamp buffalo bull. Asian Pac J Reprod. (2018) 7:233576. doi: 10.4103/2305-0500.233576
44. Raheja N, Choudhary S, Grewal S, Sharma N, Kumar N. A review on semen extenders and additives used in cattle and buffalo bull semen preservation. J Entomol Zool Stud. (2018) 6:239–45.
45. Liu C-H, Dong H-B, Ma D-L, Li Y-W, Han D, Luo M-J, et al. Effects of Ph during liquid storage of goat semen on sperm viability and fertilizing potential. Anim Reprod Sci. (2016) 164:47–56. doi: 10.1016/j.anireprosci.2015.11.011
46. Mohamed M, AM AE-H, Shaarawy A. Influence of adding different energy sources to the bull and ram spermatozoa exposed to different refrigerating times. Egyp J Sheep Goats Sci. (2019) 14:1–18. doi: 10.21608/ejsgs.2019.56661
47. Mousavi SM, Towhidi A, Zhandi M, Amoabediny G, Mohammadi-Sangcheshmeh A, Sharafi M, et al. Comparison of two different antioxidants in a nano lecithin-based extender for bull sperm cryopreservation. Anim Reprod Sci. (2019) 209:106171. doi: 10.1016/j.anireprosci.2019.106171
48. Schulze M, Nitsche-Melkus E, Hensel B, Jung M, Jakop U. Antibiotics and their alternatives in artificial breeding in livestock. Anim Reprod Sci. (2020) 220:106284. doi: 10.1016/j.anireprosci.2020.106284
49. Tariq A, Ahmad M, Iqbal S, Riaz MI, Tahir MZ, Ghafoor A, et al. Effect of carboxylated poly L-lysine as a cryoprotectant on post-thaw quality and in vivo fertility of nili ravi buffalo (Bubalus Bubalis) bull semen. Theriogenology. (2020) 144:8–15. doi: 10.1016/j.theriogenology.2019.12.012
50. Johnston SD, Zee YP, López-Fernández C, Gosálvez J. The effect of chilled storage and cryopreservation on the sperm DNA fragmentation dynamics of a captive population of koalas. J Androl. (2012) 33:1007–15. doi: 10.2164/jandrol.111.015248
51. Bustamante Filho I, Pederzolli C, Sgaravatti A, Gregory R, Dutra Filho C, Jobim M, et al. Skim milk-egg yolk based semen extender compensates for non-enzymatic antioxidant activity loss during equine semen cryopreservation. Anim Reprod. (2018) 6:392–9.
52. Layek SS, Mohanty TK, Kumaresan A, Parks JE. Cryopreservation of bull semen: evolution from egg yolk based to soybean based extenders. Anim Reprod Sci. (2016) 172:1–9. doi: 10.1016/j.anireprosci.2016.04.013
53. Bergeron A, Manjunath P. New insights towards understanding the mechanisms of sperm protection by egg yolk and milk. Mol Reprod Dev. (2006) 73:1338–44. doi: 10.1002/mrd.20565
54. Foote RH, Brockett CC, Kaproth MT. Motility and fertility of bull sperm in whole milk extender containing antioxidants. Anim Reprod Sci. (2002) 71:13–23. doi: 10.1016/S0378-4320(02)00018-0
55. Malik A. Effects of honey supplementation into the extender on the motility, abnormality and viability of frozen thawed of bali bull spermatozoa. Asian J Anim Vet Sci. (2019) 13:109–13. doi: 10.3923/ajava.2018.109.113
56. Fakhrildin M-BM, Alsaadi RA. Honey supplementation to semen-freezing medium improveshuman sperm parameters post-thawing. J Fam Reprod Health. (2014) 8:27.
57. El-Sisy GA, El-Nattat WS, El-Sheshtawy RI, Abo El-Maaty AM. Substitution of egg yolk with different concentrations of soybean lecithin in tris-based extender during bulls' semen preservability. Asian Pac J Reprod. (2016) 5:514–8. doi: 10.1016/j.apjr.2016.10.011
58. Agarwal A, Virk G, Ong C, du Plessis SS. Effect of oxidative stress on male reproduction. World J Mens Health. (2014) 32:1–17. doi: 10.5534/wjmh.2014.32.1.1
59. Shi Z, Yu M, Guo T, Sui Y, Tian Z, Ni X, et al. Micrornas in spermatogenesis dysfunction and male infertility: clinical phenotypes, mechanisms and potential diagnostic biomarkers. Front Endocrinol. (2024) 15:1293368. doi: 10.3389/fendo.2024.1293368
60. Bansal AK, Bilaspuri GS. Impacts of oxidative stress and antioxidants on semen functions. Vet Med Int. (2010) 2010:686137. doi: 10.4061/2011/686137
61. Lenzi A, Gandini L, Picardo M, Tramer F, Sandri G, Panfili E. Lipoperoxidation damage of spermatozoa polyunsaturated fatty acids (Pufa): scavenger mechanisms and possible scavenger therapies. Front Biosci. (2000) 5:E1–E15. doi: 10.2741/lenzi
62. Aitken RJ, Baker MA. Oxidative stress and male reproductive biology. Reprod Fertil Dev. (2004) 16:581–8. doi: 10.1071/RD03089
63. Li L, Jin T, Chen S, Cao H, Ma Y, Fang W, et al. Exploring novel function of Gpx5 antioxidant activity: assisting epididymal cells secrete functional extracellular vesicles. J Cell Physiol. (2024) 239:e31273. doi: 10.1002/jcp.31273
64. Bahmyari R, Zare M, Sharma R, Agarwal A, Halvaei I. The efficacy of antioxidants in sperm parameters and production of reactive oxygen species levels during the freeze-thaw process: a systematic review and meta-analysis. Andrologia. (2020) 52:e13514. doi: 10.1111/and.13514
65. Losano JDA, Angrimani DSR, Dalmazzo A, Rocha CC, Brito MM, Perez EGA, et al. Effect of vitamin E and polyunsaturated fatty acids on cryopreserved sperm quality in Bos taurus bulls under testicular heat stress. Anim Biotechnol. (2018) 29:100–9. doi: 10.1080/10495398.2017.1322973
66. Kankofer M, Kolm G, Aurich J, Aurich C. Activity of glutathione peroxidase, superoxide dismutase and catalase and lipid peroxidation intensity in stallion semen during storage at 5 degrees C. Theriogenology. (2005) 63:1354–65. doi: 10.1016/j.theriogenology.2004.07.005
67. Li J, Barranco I, Tvarijonaviciute A, Molina MF, Martinez EA, Rodriguez-Martinez H, et al. Seminal plasma antioxidants are directly involved in boar sperm cryotolerance. Theriogenology. (2018) 107:27–35. doi: 10.1016/j.theriogenology.2017.10.035
68. Murtaza A, Ahmad M, Zubair M, Umar S, Mushtaq A, Gul AHST, et al. Comparative effects of addition of superoxide dismutase and reduced glutathione on cryopreservation of sahiwal bull semen. J Hellenic Vet Med Soc. (2019) 69:1291–6. doi: 10.12681/jhvms.19619
69. Zeitoun MM, Al-Damegh MA. Effect of nonenzymatic antioxidants on sperm motility and survival relative to free radicals and antioxidant enzymes of chilled-stored ram semen. Open J Anim Sci. (2014) 5:50–8. doi: 10.4236/ojas.2015.51007
70. Roca J, Rodríguez MJ, Gil MA, Carvajal G, Garcia EM, Cuello C, et al. Survival and in vitro fertility of boar spermatozoa frozen in the presence of superoxide dismutase and/or catalase. J Androl. (2005) 26:15–24. doi: 10.1002/j.1939-4640.2005.tb02867.x
71. Nakamura BN, Lawson G, Chan JY, Banuelos J, Cortés MM, Hoang YD, et al. Knockout of the transcription factor Nrf2 disrupts spermatogenesis in an age-dependent manner. Free Rad Biol Med. (2010) 49:1368–79. doi: 10.1016/j.freeradbiomed.2010.07.019
72. Asadpour R, Jafari R, Tayefi-Nasrabadi H. Effect of various levels of catalase antioxidant in semen extenders on lipid peroxidation and semen quality after the freeze-thawing bull semen. Vet Res Forum. (2011) 2:218–21.
73. Kim S-H, Lee Y-J, Kang T-W, Kim Y-J. The reduction of hydrogen peroxide in viable boar sperm cryopreserved in the presence of catalase. J Vet Clin. (2011) 28:13–9.
74. Gungor S, Ata A, Inanc ME. Effects of trehalose and catalase on the viability and kinetic parameters of cryopreserved ram sperm. Acta Sci Vet. (2018) 46:7. doi: 10.22456/1679-9216.83865
75. Li Z, Lin Q, Liu R, Xiao W, Liu W. Protective effects of ascorbate and catalase on human spermatozoa during cryopreservation. J Androl. (2010) 31:437–44. doi: 10.2164/jandrol.109.007849
76. Drevet JR. The antioxidant glutathione peroxidase family and spermatozoa: a complex story. Mol Cell Endocrinol. (2006) 250:70–9. doi: 10.1016/j.mce.2005.12.027
77. Barros L, Silva S, Almeida F, Silva E, Carneiro G, Guerra M. Effect of addition of acetyl-cysteine and glutathione peroxidase in freezing extender of stallion semen. J Equine Vet Sci. (2012) 32:478. doi: 10.1016/j.jevs.2012.06.026
78. Del Prete C, Stout T, Montagnaro S, Pagnini U, Uccello M, Florio P, et al. Combined addition of superoxide dismutase, catalase and glutathione peroxidase improves quality of cooled stored stallion semen. Anim Reprod Sci. (2019) 210:106195. doi: 10.1016/j.anireprosci.2019.106195
79. Aydemir B, Onaran I, Kiziler AR, Alici B, Akyolcu MC. Increased oxidative damage of sperm and seminal plasma in men with idiopathic infertility is higher in patients with glutathione S-transferase Mu-1 null genotype. Asian J Androl. (2007) 9:108–15. doi: 10.1111/j.1745-7262.2007.00237.x
80. Uribe P, Treulen F, Boguen R, Sánchez R, Villegas JV. Nitrosative stress by peroxynitrite impairs Atp production in human spermatozoa. Andrologia. (2017) 49:12615. doi: 10.1111/and.12615
81. Dorostghoal M, Kazeminejad SR, Shahbazian N, Pourmehdi M, Jabbari A. Oxidative stress status and sperm DNA fragmentation in fertile and infertile men. Andrologia. (2017) 49:e12762. doi: 10.1111/and.12762
82. Kumar A, Prasad JK, Kumar N, Anand M, Verma S, Dhariya R, et al. Quercetin in semen extender curtails reactive oxygen and nitrogen species and improves functional attributes of cryopreserved buck semen. Cryobiology. (2024) 116:104931. doi: 10.1016/j.cryobiol.2024.104931
83. Batool I, Fayyaz MH, Hameed A, Andrabi SMH, Kausar R, Shahzad M, et al. Quercetin in semen extender improves frozen-thawed spermatozoa quality and in-vivo fertility in crossbred kamori goats. Front Vet Sci. (2024) 11:1385642. doi: 10.3389/fvets.2024.1385642
84. Lobo V, Patil A, Phatak A, Chandra N. Free radicals, antioxidants and functional foods: impact on human health. Pharmacogn Rev. (2010) 4:118–26. doi: 10.4103/0973-7847.70902
85. Vasconcelos Franco JS, Chaveiro A, Góis A, Moreira da Silva F. Effects of A-tocopherol and ascorbic acid on equine semen quality after cryopreservation. J Equine Vet Sci. (2013) 33:787–93. doi: 10.1016/j.jevs.2012.12.012
86. Mittal PK, Anand M, Madan A, Yadav S, Kumar J. Antioxidative capacity of vitamin E, vitamin C and their combination in cryopreserved bhadavari bull semen. Vet World. (2014) 7:1131. doi: 10.14202/vetworld.2014.1127-1131
87. El-Hamid I, Khalifa M, Shedeed H, Rateb S. Cryosurvival of ram spermatozoa after supplementing the diluent with L-ascorbic acid or A-tocopherol. Int. J. Vet. Sci. (2018) 7:197–204.
88. Rashki Ghaleno L, Alizadeh A, Drevet JR, Shahverdi A, Valojerdi MR. Oxidation of sperm DNA and male infertility. Antioxidants. (2021) 10:97. doi: 10.3390/antiox10010097
89. Hegedušová Z, Štolc L, Louda F, Cunát L, Vejnar J. Effect of different extenders on ram sperm traits during storage. Acta Univ Agric et Silvic Mend Brun. (2012) 60:111–6. doi: 10.11118/actaun201260060111
90. Rehman F, Zhao C, Shah M, Qureshi M, Wang X. Semen extenders and artificial insemination in ruminants. Veterinaria. (2013) 1:1–8.
91. Jiménez-Rabadán P, García-Álvarez O, Vidal A, Maroto-Morales A, Iniesta-Cuerda M, Ramón M, et al. Effects of vitrification on ram spermatozoa using free-egg yolk extenders. Cryobiology. (2015) 71:85–90. doi: 10.1016/j.cryobiol.2015.05.004
92. Saadeldin IM, Khalil WA, Alharbi MG, Lee SH. The current trends in using nanoparticles, liposomes, and exosomes for semen cryopreservation. Animals. (2020) 10:2281. doi: 10.3390/ani10122281
93. Saleh NT, Hosseinzadeh Z, Banadkuki NG, Novin MS, Zaman SS, Gardeshi TM. The protective effect of zinc oxide and selenium oxide nanoparticles on the functional parameters of rat sperm during vitrification. Clin Exp Reprod Med. (2024) 51:20–7. doi: 10.5653/cerm.2023.06079
94. Sultan T, Chaudhary AK, Mehta J, Mehra R, Manuja A, Talluri T, et al. Cryoprotective effect of zinc and gold nanoparticles during cooling and freeze-thawing on marwari stallion sperm parameters and reactive oxygen species production. Cryoletters. (2024) 45:361–9. doi: 10.54680/fr24610110212
95. Castellini C, Placidi M, Barbonetti A, Tatone C, Di Emidio G. Mechanisms underlying human sperm cryodamage: the role of reactive oxygen species (ros) and antioxidants. RIVER J. (2024) 1:3–9. doi: 10.57582/RIVER.240101.003
96. Abdel-Ghani MA, El-Sharawy ME, Zaid EK, Shehabeldin AM, Dessouki SM, Moustapha ME, et al. Low concentrations of soybean lecithin nanoparticles had a positive impact on holstein bulls' cryopreserved semen. Reprod Dom Anim. (2024) 59:e14613. doi: 10.1111/rda.14613
97. Lone F. Nanotechnology based semen purification: a panacea. Vet Med Open J. (2016) 1:e1–2. doi: 10.17140/VMOJ-1-e001
98. Moradi M, Hajarian H, Karamishabankareh H, Soltani L, Soleymani B. Pre-treatment of ram semen extender with magnetic nanoparticles on freeze-thawed spermatozoa. Vet Med Sci. (2022) 8:792–8. doi: 10.1002/vms3.689
99. Najafi A, Taheri RA, Mehdipour M, Martínez-Pastor F, Rouhollahi AA, Nourani MR. Improvement of post-thawed sperm quality in broiler breeder roosters by ellagic acid-loaded liposomes. Poult Sci. (2019) 98:440–6. doi: 10.3382/ps/pey353
100. Mendoza-Viveros CD, Gutiérrez-Pérez O, Bernad-Bernad MJ, Medina-Torres L, Monroy-Barreto M, Gimeno M, et al. Boar semen cryopreserved with trehalose-containing liposomes: disaccharide determination and rheological behaviour. Zygote. (2022) 30:895–902. doi: 10.1017/S0967199422000442
101. Mutalik S, Salian SR, Avadhani K, Menon J, Joshi H, Hegde AR, et al. Liposome encapsulated soy lecithin and cholesterol can efficiently replace chicken egg yolk in human semen cryopreservation medium. Syst Biol Reprod Med. (2014) 60:183–8. doi: 10.3109/19396368.2014.902521
102. Medina-León AZ, Domínguez-Mancera B, Cazalez-Penino N, Cervantes-Acosta P, Jácome-Sosa E, Romero-Salas D, et al. Cryopreservation of horse semen with a liposome and trehalose added extender. Aust J Vet Sci. (2019) 51:119–23. doi: 10.4067/S0719-81322019000300119
103. Taouzinet L, Fatmi S, Khellouf A, Lahiani-Skiba M, Skiba M, Iguer-Ouada M. Drug release, stability and efficiency of vitamin E loaded in liposomes for bovine sperm protection in cryopreservation medium. CryoLetters. (2022) 43:50–7. doi: 10.54680/fr22110110612
104. Fraser L. A novel approach to assess semen freezability. Vet Med Open J. (2016) 1:e5–6. doi: 10.17140/VMOJ-1-e003
105. Hermann G, Zabludovsky N, Steinberger Y. Preliminary study: a new approach for improving the cryopreservation of mammalian sperm. Open J Anim Sci. (2019) 9:13. doi: 10.4236/ojas.2019.94032
106. Pardede BP, Agil M, Yudi Y, Supriatna I. Relationship of frozen-thawed semen quality with the fertility rate after being distributed in the brahman cross breeding program. Vet World. (2020) 13:2649–57. doi: 10.14202/vetworld.2020.2649-2657
107. Craig LB, Brush RS, Sullivan MT, Zavy MT, Agbaga M-P, Anderson RE. Decreased very long chain polyunsaturated fatty acids in sperm correlates with sperm quantity and quality. J Assist Reprod Genet. (2019) 36:1379–85. doi: 10.1007/s10815-019-01464-3
108. Ma L, Kim D-H, Jung E-J, Lee W-J, Hwang J-M, Bae J-W, et al. Effect of glycerol addition time on the cryopreserved korean native brindle cattle (chikso) sperm quality. Anim Reprod. (2022) 19:e20210058. doi: 10.1590/1984-3143-ar2021-0058
109. Pardede BP, Kusumawati A, Pangestu M, Purwantara B. Bovine sperm Hsp-70 molecules: a potential cryo-tolerance marker associated with semen quality and fertility rate. Front Vet Sci. (2023) 10:1167594. doi: 10.3389/fvets.2023.1167594
110. Luo X, Liang M, Huang S, Xue Q, Ren X, Li Y, et al. Itraq-based comparative proteomics reveal an enhancing role of Prdx6 in the freezability of mediterranean buffalo sperm. BMC Genom. (2023) 24:245. doi: 10.1186/s12864-023-09329-x
111. Xu B, Bai X, Zhang J, Li B, Zhang Y, Su R, et al. Metabolomic analysis of seminal plasma to identify goat semen freezability markers. Front Vet Sci. (2023) 10:1132373. doi: 10.3389/fvets.2023.1132373
112. Ros-Santaella JL, Domínguez-Rebolledo ÁE, Garde JJ. Sperm flagellum volume determines freezability in red deer spermatozoa. PLoS ONE. (2014) 9:e112382. doi: 10.1371/journal.pone.0112382
113. Hitit M, Ugur MR, Dinh TTN, Sajeev D, Kaya A, Topper E, et al. Cellular and functional physiopathology of bull sperm with altered sperm freezability. Front Vet Sci. (2020) 7:581137. doi: 10.3389/fvets.2020.581137
114. Ramón M, Martínez-Pastor F, García-Álvarez O, Maroto-Morales A, Soler AJ, Jiménez-Rabadán P, et al. Taking advantage of the use of supervised learning methods for characterization of sperm population structure related with freezability in the iberian red deer. Theriogenology. (2012) 77:1661–72. doi: 10.1016/j.theriogenology.2011.12.011
115. Thambawita V, Hicks SA, Storås AM, Nguyen T, Andersen JM, Witczak O, et al. Visem-tracking, a human spermatozoa tracking dataset. Sci Data. (2023) 10:260. doi: 10.1038/s41597-023-02173-4
116. Hicks SA, Andersen JM, Witczak O, Thambawita V, Halvorsen P, Hammer HL, et al. Machine learning-based analysis of sperm videos and participant data for male fertility prediction. Sci Rep. (2019) 9:16770. doi: 10.1038/s41598-019-53217-y
117. Leung TY, Lee CL, Chiu PCN. P−064 application of an artificial intelligence model for morphologic prediction of fertilization-competent human spermatozoa. Hum Reprod. (2021) 36(Suppl. 1):e063. doi: 10.1093/humrep/deab130.063
118. Campanholi SP, Garcia Neto S, Pinheiro GM, Nogueira MFG, Rocha JC, Losano JDdA, et al. Can in vitro embryo production be estimated from semen variables in senepol breed by using artificial intelligence? Front Vet Sci. (2023) 10:1254940. doi: 10.3389/fvets.2023.1254940
119. Lafuente R, López Granollers G, Hernando A, Carballo E, Canals J, Pujol A, et al. O-308 Is ai the future of sperm quality assessment? A comparative study of ai-enhanced and conventional semen analysis systems. Hum Reprod. (2024) 39(Suppl. 1):deae108.365. doi: 10.1093/humrep/deae108.365
120. Ottl S, Amiriparian S, Gerczuk M, Schuller B. A machine learning framework for automatic prediction of human semen motility. arXiv preprint arXiv:210908049. (2021). doi: 10.1016/j.isci.2022.104644
121. Miller ME, Kemski M, Grayson JD, Towne K, Dabrowski K. Yellow perch sperm motility, cryopreservation, and viability of resulting larvae and juveniles. N Am J Aquac. (2018) 80:3–12. doi: 10.1002/naaq.10001
122. Esteves SC, Spaine DM, Cedenho AP. Effects of pentoxifylline treatment before freezing on motility, viability and acrosome status of poor quality human spermatozoa cryopreserved by the liquid nitrogen vapor method. Braz J Med Biol Res. (2007) 40:985–92. doi: 10.1590/S0100-879X2006005000118
123. Santiani A, Juárez J, Allauca P, Roman B, Ugarelli A, Evangelista-Vargas S. Cryopreservation effect on sperm viability, mitochondrial membrane potential, acrosome integrity and sperm capacitation of alpaca spermatozoa detected by imaging flow cytometry. Reprod Dom Anim. (2023) 58:560–3. doi: 10.1111/rda.14318
124. Ortiz-Rodriguez JM, Ortega-Ferrusola C, Gil MC, Martín-Cano FE, Gaitskell-Phillips G, Rodríguez-Martínez H, et al. Transcriptome analysis reveals that fertilization with cryopreserved sperm downregulates genes relevant for early embryo development in the horse. PLoS ONE. (2019) 14:e0213420. doi: 10.1371/journal.pone.0213420
125. Figueroa E, Lee-Estévez M, Valdebenito I, Farías JG, Romero J. Potential biomarkers of DNA quality in cryopreserved fish sperm: impact on gene expression and embryonic development. Rev Aquacult. (2020) 12:382–91. doi: 10.1111/raq.12323
126. Ortiz-Rodríguez JM, Martín-Cano FE FE, Gaitskell-Phillips G, Barrientos AÁ, Rodríguez-Martínez H, Gil MC, et al. Sperm cryopreservation impacts the early development of equine embryos by downregulating specific transcription factors. BioRxiv. (2021) 2021.05.12.443855. doi: 10.1101/2021.05.12.443855
127. Xu X, Li W, Zhang L, Ji Y, Qin J, Wang L, et al. Effect of sperm cryopreservation on mirna expression and early embryonic development. Front Cell Dev Biol. (2021) 9:749486. doi: 10.3389/fcell.2021.749486
128. Paoli D, Lombardo F, Lenzi A, Gandini L. Sperm cryopreservation: effects on chromatin structure. In:Baldi E, Muratori M, , editors. Genetic Damage in Human Spermatozoa. New York, NY: Springer New York (2014). p. 137–50.
129. Irvine DS, Twigg JP, Gordon EL, Fulton N, Milne PA, Aitken RJ, et al. Integrity in human spermatozoa: relationships with semen quality. J Androl. (2000) 21:33–44. doi: 10.1002/j.1939-4640.2000.tb03273.x
130. Holt WV, North RD. Effects of temperature and restoration of osmotic equilibrium during thawing on the induction of plasma membrane damage in cryopreserved ram spermatozoa. Biol Reprod. (1994) 51:414–24. doi: 10.1095/biolreprod51.3.414
131. Oldenhof H, Gojowsky M, Wang S, Henke S, Yu C, Rohn K, et al. Osmotic stress and membrane phase changes during freezing of stallion sperm: mode of action of cryoprotective agents. Biol Reprod. (2013) 88:104661. doi: 10.1095/biolreprod.112.104661
132. De Leeuw FE, De Leeuw AM, Den Daas JHG, Colenbrander B, Verkleij AJ. Effects of various cryoprotective agents and membrane-stabilizing compounds on bull sperm membrane integrity after cooling and freezing. Cryobiology. (1993) 30:32–44. doi: 10.1006/cryo.1993.1005
133. Watson PF. The causes of reduced fertility with cryopreserved semen. Anim Reprod Sci. (2000) 60:481–92. doi: 10.1016/S0378-4320(00)00099-3
134. Khosravizadeh Z, Khodamoradi K, Rashidi Z, Jahromi M, Shiri E, Salehi E, et al. Sperm cryopreservation and DNA methylation: possible implications for art success and the health of offspring. J Assist Reprod Genet. (2022) 39:1815–24. doi: 10.1007/s10815-022-02545-6
135. França LR, Godinho CL. Testis morphometry, seminiferous epithelium cycle length, and daily sperm production in domestic cats (Felis Catus). Biol Reprod. (2003) 68:1554–61. doi: 10.1095/biolreprod.102.010652
136. França L, Becker-Silva S, Chiarini-Garcia H. The length of the cycle of seminiferous epithelium in goats (Capra Hircus). Tissue Cell. (1999) 31:274–80. doi: 10.1054/tice.1999.0044
137. Gu N-H, Zhao W-L, Wang G-S, Sun F. Comparative analysis of mammalian sperm ultrastructure reveals relationships between sperm morphology, mitochondrial functions and motility. Reprod Biol Endocrinol. (2019) 17:66. doi: 10.1186/s12958-019-0510-y
138. Sun P, Zhang G, Xian M, Zhang G, Wen F, Hu Z, et al. Proteomic analysis of frozen–thawed spermatozoa with different levels of freezability in dairy goats. Int J Mol Sci. (2023) 24:15550. doi: 10.3390/ijms242115550
139. Vicente-Fiel S, Palacín I, Santolaria P, Hidalgo CO, Silvestre MA, Arrebola F, et al. Comparative study of the sperm nuclear morphometry in cattle, goat, sheep, and pigs using a new computer-assisted method (casma-F). Theriogenology. (2013) 79:436–42. doi: 10.1016/j.theriogenology.2012.10.015
140. Andraszek K, Banaszewska D, Szostek M, Wójcik E, Czubaszek M, Walczak-Jedrzejowska R. Use of silver nitrate for the assessment of sperm measurements in selected farm and free-living animal species. J Vet Res. (2014) 58:487–94. doi: 10.2478/bvip-2014-0074
141. Murat F, Mbengue N, Winge SB, Trefzer T, Leushkin E, Sepp M, et al. The molecular evolution of spermatogenesis across mammals. Nature. (2023) 613:308–16. doi: 10.1038/s41586-022-05547-7
142. Kadirvel G, Kumar S, Kumaresan A. Lipid peroxidation, mitochondrial membrane potential and DNA integrity of spermatozoa in relation to intracellular reactive oxygen species in liquid and frozen-thawed buffalo semen. Anim Reprod Sci. (2009) 114:125–34. doi: 10.1016/j.anireprosci.2008.10.002
143. Hai E, Li B, Zhang J, Zhang J. Sperm freezing damage: the role of regulated cell death. Cell Death Discov. (2024) 10:239. doi: 10.1038/s41420-024-02013-3
144. Angrimani DSR, Silva ROC, Losano JDA, Dalmazzo A, Tsunoda RH, Perez EGA, et al. Extender supplementation with antioxidants selected after the evaluation of sperm susceptibility to oxidative challenges in goats. Anim Biotechnol. (2019) 30:21–9. doi: 10.1080/10495398.2018.1423992
145. Xu B, Wang R, Wang Z, Liu H, Wang Z, Zhang W, et al. Evaluation of lipidomic change in goat sperm after cryopreservation. Front Vet Sci. (2022) 9:1004683. doi: 10.3389/fvets.2022.1004683
146. De Iuliis GN, Thomson LK, Mitchell LA, Finnie JM, Koppers AJ, Hedges A, et al. DNA damage in human spermatozoa is highly correlated with the efficiency of chromatin remodeling and the formation of 8-hydroxy-2′-deoxyguanosine, a marker of oxidative stress. Biol Reprod. (2009) 81:517–24. doi: 10.1095/biolreprod.109.076836
147. Zhu W, Cheng X, Ren C, Chen J, Zhang Y, Chen Y, et al. Proteomic characterization and comparison of ram (Ovis Aries) and buck (Capra Hircus) spermatozoa proteome using a data independent acquisition mass spectometry (Dia-Ms) approach. PLoS ONE. (2020) 15:e0228656. doi: 10.1371/journal.pone.0228656
148. Cankut S, Dinc T, Cincik M, Ozturk G, Selam B. Evaluation of sperm DNA fragmentation via halosperm technique and tunel assay before and after cryopreservation. Reprod Sci. (2019) 26:1575–81. doi: 10.1177/1933719119828096
149. Rochdi C, Allai L, Bellajdel I, Taheri H, Saadi H, Mimouni A, et al. Evaluation of sperm DNA fragmentation using halosperm technique after the freezing-thawing process in men: a study on the validation of the Scd protocol. J Reprod Infertil. (2024) 25:12–9. doi: 10.18502/jri.v25i1.15194
150. Mateo-Otero Y, Llavanera M, Torres-Garrido M, Yeste M. Embryo development is impaired by sperm mitochondrial-derived ros. Biol Res. (2024) 57:5. doi: 10.1186/s40659-024-00483-4
151. Said TM, Fischer-Hammadeh C, Hamad M, Refaat K, Hammadeh ME. Oxidative stress, DNA damage, and apoptosis in male infertility. In:Agarwal A, Aitken RJ, Alvarez JG, , editors. Studies on Men's Health and Fertility. Totowa, NJ: Humana Press (2012). p. 433–48.
152. Penitente-Filho JM, Oliveira FA, Jimenez CR, Carrascal E, Dias JC, Oliveira GD, et al. Association of vitamin E with rapid thawing on goat semen. ScientificWorldJournal. (2014) 2014:964172. doi: 10.1155/2014/964172
153. Zou J, Wei L, Li D, Zhang Y, Wang G, Zhang L, et al. Effect of glutathione on sperm quality in guanzhong dairy goat sperm during cryopreservation. Front Vet Sci. (2021) 8:771440. doi: 10.3389/fvets.2021.771440
154. Aitken RJ, Muscio L, Whiting S, Connaughton HS, Fraser BA, Nixon B, et al. Analysis of the effects of polyphenols on human spermatozoa reveals unexpected impacts on mitochondrial membrane potential, oxidative stress and DNA integrity; implications for assisted reproductive technology. Biochem Pharmacol. (2016) 121:78–96. doi: 10.1016/j.bcp.2016.09.015
155. Ömür AD, Kaynar Ö, Ileritürk M, Özkaraca M, Öztürk AE, Bodu M, et al. Immunofluorescence and biochemical investigation of the protective effects of naringin and diosmin on the freezability of merino ram semen. CryoLetters. (2024) 45:309–19. doi: 10.54680/fr24510110412
156. Bucak MN, Bodu M, Başpinar N, Güngör S, Ili P, Acibaeva B, et al. Influence of ellagic acid and ebselen on sperm and oxidative stress parameters during liquid preservation of ram semen. Cell J. (2019) 21:7–13. doi: 10.22074/cellj.2019.5593
157. Nurul I, Nuryadi N, Eko N, editors. Supplementation of mangosteen (Garcinia Mangostana) pericarp filtrate in tris-egg yolk-based diluent on buck sperm membrane integrity. In: Proceedings of the 1st International Conference in One Health (ICOH 2017). Atlantis Press (2017).
158. Kumar R, Chandra P, Konyak P, Karunakaran M, Santra A, Das SK. In vitro development of preimplantation caprine embryo using cryopreserved black bengal buck semen. Indian J Anim Res. (2020) 54:1210–3. doi: 10.18805/ijar.B-3886
159. Hitit M, Memili E. Sperm signatures of fertility and freezability. Anim Reprod Sci. (2022) 247:107147. doi: 10.1016/j.anireprosci.2022.107147
160. Nikbin S, Panandam JM, Yaakub H, Murugaiyah M, Sazili AQ. Novel Snps in heat shock protein 70 gene and their association with sperm quality traits of boer goats and boer crosses. Anim Reprod Sci. (2014) 146:176–81. doi: 10.1016/j.anireprosci.2014.03.001
161. Hu W, Fang M, Yang Y, Ye T, Liu B, Zheng W. Detection of heat shock protein 27, 70, 90 expressions in primary parenchymatous organs of goats after transport stress by real-time Pcr and elisa. Vet Med Sci. (2020) 6:788–95. doi: 10.1002/vms3.327
162. Hernawati T, Susilowati S, Mulyati S, Oktanella Y. Optimizing equilibration protocols through ubiquinone supplementation in goat frozen semen diluent. Interciencia. (2024) 404:65–83. doi: 10.59671/gvis9
163. Rana APS, Majumder GC, Misra S, Ghosh A. Lipid changes of goat sperm plasma membrane during epididymal maturation. Biochim Biophys Acta Biomemb. (1991) 1061:185–96. doi: 10.1016/0005-2736(91)90284-F
164. Jiménez-Rabadán P, Soler AJ, Ramón M, García-Álvarez O, Maroto-Morales A, Iniesta-Cuerda M, et al. Influence of semen collection method on sperm cryoresistance in small ruminants. Anim Reprod Sci. (2016) 167:103–8. doi: 10.1016/j.anireprosci.2016.02.013
165. Jiménez-Rabadán P, Ramón M, García-Álvarez O, Maroto-Morales A., del Olmo E, Pérez-Guzmán MD, et al. Effect of semen collection method (artificial vagina vs electroejaculation), extender and centrifugation on post-thaw sperm quality of blanca-celtibérica buck ejaculates. Anim Reprod Sci. (2012) 132:88–95. doi: 10.1016/j.anireprosci.2012.04.005
166. Anand M, Baghel G, Yadav S. Effect of egg yolk concentration and washing on sperm quality following cryopreservation in barbari buck semen. J Appl Anim Res. (2017) 45:560–5. doi: 10.1080/09712119.2016.1232265
167. Mocé E, Tomás C, Blanch E, Graham JK. Effect of cholesterol-loaded cyclodextrins on bull and goat sperm processed with fast or slow cryopreservation protocols. Animal. (2014) 8:771–6. doi: 10.1017/S1751731114000226
168. Daramola J, Adekunle E, Onagbesan O, Oke O, Ladokun A, Abiona J, et al. Protective effects of fruit-juices on sperm viability of west African dwarf goat bucks during cryopreservation. Anim Reprod. (2018) 13:7–13. doi: 10.4322/1984-3143-AR726
169. Cabrera F, González F, Batista M, Calero P, Medrano A, Gracia A. The effect of removal of seminal plasma, egg yolk level and season on sperm freezability of canary buck (Capra Hircus). Reprod Dom Anim. (2005) 40:191–5. doi: 10.1111/j.1439-0531.2005.00544.x
170. Cardenas-Padilla AJ, Jimenez-Trejo F, Cerbon M, Chavez-Garcia A, Cruz-Cano NB, Martinez-Torres M, et al. Sperm melatonin receptors, seminal plasma melatonin and semen freezability in goats. Theriogenology. (2024) 225:98–106. doi: 10.1016/j.theriogenology.2024.05.034
171. Xu B, Wang Z, Wang R, Song G, Zhang Y, Su R, et al. Metabolomics analysis of buck semen cryopreserved with trehalose. Front Genet. (2022) 13:938622. doi: 10.3389/fgene.2022.938622
172. Öztürk AE, Bucak MN, Bodu M, Başpinar N, Çelik I, Shu Z, et al. Cryobiology and cryopreservation of sperm. Cryopreserv IntechOpen. (2019).
173. Morrell JM, Malaluang P, Ntallaris T, Johannisson A. Practical method for freezing buck semen. Animals. (2022) 12:352. doi: 10.3390/ani12030352
174. Nasr NH, Abu Elnaga NA, AbdRabou MA, Ghanem N. Age-dependent computer-assisted sperm analysis of buck semen supplemented with different antioxidants. Egyp J Hosp Med. (2023) 90:994–1000. doi: 10.21608/ejhm.2023.280195
175. Ohaneje UL, Osuagwuh UI, Alvarez-Rodríguez M, Yánez-Ortiz I, Tabarez A, Palomo MJ. The re-addition of seminal plasma after thawing does not improve buck sperm quality parameters. Animals. (2021) 11:3452. doi: 10.3390/ani11123452
176. Barfourooshi HJ, Esmaeilkhanian S, Davachi ND, Asadzadeh N, Masoudi R. Effect of mito-tempo on post-thawed semen quality in goats. Iran J Vet Med. (2023) 17:393–400. doi: 10.32598/ijvm.17.4.1005346
177. Behera S, Harshan HM, Bhai KL, Ghosh KN. Effect of cholesterol supplementation on cryosurvival of goat spermatozoa. Vet World. (2015) 8:1386–91. doi: 10.14202/vetworld.2015.1386-1391
178. Metwaly A, El-Siefy E, El-Halawany R, EL-Behnsawy B. Effect of cysteine supplementation on freezability of baladie goat spermatozoa. J Sustain Agricul Sci. (2016) 42:120–30. doi: 10.21608/jsas.2016.3030
179. Kamal MM, Alam ME, Islam MA, Gofur MR, Kabir A. Effects of tris (hydroxymethyl) aminomethane and egg yolk on the cryopreservation of buck semen. J Adv Vet Anim Res. (2022) 9:676–83. doi: 10.5455/javar.2022.i636
180. Soltan W, El-Siefy E, Abd El-Razek I, El-Shamaa I. Efficacy of using soybean milk-based semen extender for freezability of buck spermatozoa. J Agric Res Kafr El-Sheikh Univ. (2016) 42:131–43. doi: 10.21608/jsas.2016.3031
181. Kamal MM, Alam ME, Das SK, Yeasmin MS, Ahmed S, Rahman MA, et al. Effects of glucose and trehalose on tris-citric acid-egg yolk-fructose diluents for semen cryopreservation in goat. J Adv Vet Anim Res. (2023) 10:169–77. doi: 10.5455/javar.2023.j666
182. Konyak P, Mandal A, Mondal M, Bhakat C, Das S, Rai S, et al. Preservation of black bengal buck semen in soybean lecithin based chemically defined extender. Indian J Anim Res. (2018) 52:1151–4. doi: 10.18805/ijar.B-3335
183. Nazari P, Farshad A, Hosseini Y. Protective effects of trehalose and pentoxifylline on goat sperm exposed to chilling-freezing process. Biopreserv Biobank. (2022) 20:540–50. doi: 10.1089/bio.2021.0081
184. Tuncer PB, Taşdemir U, Büyükleblebici S, Özgürtaş T, Coşkun E, Erol H, et al. Effects of different doses of trehalose supplementation in egg yolk extender in frozen–thawed angora buck semen. Small Rumin Res. (2013) 113:383–9. doi: 10.1016/j.smallrumres.2013.04.012
185. Daramola JO. Effect of centrifugation on motility, sperm capacitation and acrosome reaction in soy bean and avocado seed milk extenders of cryopreserved goat spermatozoa. Agricul Trop Subtrop. (2017) 50:13–8. doi: 10.1515/ats-2017-0002
186. Das A, Uddin AM, Uddin MB, Rahman ANMA, Hossain MK, Atikuzzaman M. Seminal plasma contains Tgf-B and Cxcl10 but sperm washing before cryopreservation is beneficial for post-thawing sperm motility in black bengal goats (Capra Hircus). Am J Reprod Immunol. (2020) 84:e13301. doi: 10.1111/aji.13301
187. Mutlak NK. The impact of adding different levels of egg yolk on the motility and morphology pre and post thaw cryopreservation of goat semen. Al-Anbar J Vet Sci. (2019) 12:107–15. doi: 10.37940/AJVS.2019.12.1.12
188. Xiao Y, Wu Z, Wang M. Effects of fulvic acids on goat sperm. Zygote. (2018) 26:220–3. doi: 10.1017/S0967199418000126
189. Kardani A, Modi L, Chaudhari N, Singh V, Modi F, Bhut D. Effect of black cumin (Nigella Sativa) seeds extract in tris egg yolk citrate extender on oxidative stress parameters of cryopreserved surti buck semen. Int J Adv Biochem Res. (2024) 8:777–81. doi: 10.33545/26174693.2024.v8.i3Sj.880
190. Peris-Frau P, Soler AJ, Iniesta-Cuerda M, Martín-Maestro A, Sánchez-Ajofrín I, Medina-Chávez DA, et al. Sperm cryodamage in ruminants: understanding the molecular changes induced by the cryopreservation process to optimize sperm quality. Int J Mol Sci. (2020) 21:2781. doi: 10.3390/ijms21082781
191. Allai L, Benmoula A, Marciane da Silva M, Nasser B, El Amiri B. Supplementation of ram semen extender to improve seminal quality and fertility rate. Anim Reprod Sci. (2018) 192:6–17. doi: 10.1016/j.anireprosci.2018.03.019
192. Roca J, Martínez E, Sánchez-Valverde MA, Ruiz S, Vázquez JM. Seasonal variations of semen quality in male goats: study of sperm abnormalities. Theriogenology. (1992) 38:115–25. doi: 10.1016/0093-691X(92)90223-E
193. Danso F, Iddrisu L, Lungu SE, Zhou G, Ju X. Effects of heat stress on goat production and mitigating strategies: a review. Animals. (2024) 14:ani14121793. doi: 10.3390/ani14121793
194. Lukusa K, Kabuba J. Semen collection methods and cooling rates affect post-thaw sperm motility and kinematic parameters of saanen goat. Asian Pac J Reprod. (2020) 9:239–46. doi: 10.4103/2305-0500.294666
195. Yoon SJ, Rahman MS, Kwon WS Ryu DY, Park YJ, Pang MG. Proteomic identification of cryostress in epididymal spermatozoa. J Anim Sci Biotechnol. (2016) 7:67. doi: 10.1186/s40104-016-0128-2
196. Medrano A, Terrazas A, Soto R. Principles and perspectives for the conservation of goat buck spermatozoa. Small Rumin Res. (2010) 89:140–3. doi: 10.1016/j.smallrumres.2009.12.036
197. Evans HC, Dinh TTN, Ugur MR, Hitit M, Sajeev D, Kaya A, et al. Lipidomic markers of sperm cryotolerance in cattle. Sci Rep. (2020) 10:20192. doi: 10.1038/s41598-020-77089-9
198. An Z, Shi L, Zhou H, Hou G, Xun W. Exploratory metabolomics and lipidomics profiling contributes to understanding how curcumin improves quality of goat semen stored at 16°C in tropical areas. Int J Mol Sci. (2024) 25:10200. doi: 10.3390/ijms251810200
199. Luo X, Ren X, Huang S, Li Y, Xue Q, Shi D, et al. Seminal plasma lipid profiles of differential cryotolerance of semen in mediterranean buffalo bulls. Reprod Dom Anim. (2023) 58:481–5. doi: 10.1111/rda.14308
200. de Andrade AF. 175 Analysis of cryopreserved semen quality: with the tools available, what is possible to be interpreted. J Anim Sci. (2021) 99(Suppl. 1):112–3. doi: 10.1093/jas/skab054.185
201. Evans HC, Dinh TTN, Hardcastle ML, Gilmore AA, Ugur MR, Hitit M, et al. Advancing semen evaluation using lipidomics. Front Vet Sci. (2021) 8:601794. doi: 10.3389/fvets.2021.601794
202. Rivera-Egea R, Garrido N, Sota N, Meseguer M, Remohí J, Dominguez F. Sperm lipidic profiles differ significantly between ejaculates resulting in pregnancy or not following intracytoplasmic sperm injection. J Assist Reprod Genet. (2018) 35:1973–85. doi: 10.1007/s10815-018-1284-4
203. Klein EK, Swegen A, Gunn AJ, Stephen CP, Aitken RJ. Gibb Z. The future of assessing bull fertility: can the ‘omics fields identify usable biomarkers? Biol Reprod. (2022) 106:854–64. doi: 10.1093/biolre/ioac031
204. Mazur P. Freezing of living cells: mechanisms and implications. Am J Physiol Cell Physiol. (1984) 247:C125–C42. doi: 10.1152/ajpcell.1984.247.3.C125
205. NCBI. Bos taurus. Available online at: https://www.ncbi.nlm.nih.gov/datasets/taxonomy/9925/ (accessed December 4, 2024).
206. NCBI. Capra hircus. Available online at: https://www.ncbi.nlm.nih.gov/datasets/taxonomy/9913/ (accessed December 4, 2024).
207. NCBI. Ovis aries. Available online at: https://www.ncbi.nlm.nih.gov/datasets/taxonomy/9940/ (accessed December 4, 2024).
208. NCBI. Sus scrofa. Available online at: https://www.ncbi.nlm.nih.gov/datasets/taxonomy/9823/ (accessed December 4, 2024).
209. NCBI. Equus caballus. Available online at: https://www.ncbi.nlm.nih.gov/datasets/taxonomy/9796/ (accessed December 4, 2024).
210. NCBI. Homo sapiens. Available online at: https://www.ncbi.nlm.nih.gov/datasets/taxonomy/9606/ (accessed December 4, 2024).
211. McGowan M, Holland MK, Boe-Hansen G. Review: ontology and endocrinology of the reproductive system of bulls from fetus to maturity. Animal. (2018) 12:s19–26. doi: 10.1017/S1751731118000460
213. Reiland S. Growth and skeletal development of the pig. Acta Radiologica Supplementum. (1978) 358:15–22.
215. Alotaibi MF. Physiology of puberty in boys and girls and pathological disorders affecting its onset. J Adolesc. (2019) 71:63–71. doi: 10.1016/j.adolescence.2018.12.007
216. Knight TW, Lindsay DR. Short-and long-term effects of oxytocin on quality and quantity of semen from rams. Reproduction. (1970) 21:523–9. doi: 10.1530/jrf.0.0210523
217. França LR, Avelar GF, Almeida FFL. Spermatogenesis and sperm transit through the epididymis in mammals with emphasis on pigs. Theriogenology. (2005) 63:300–18. doi: 10.1016/j.theriogenology.2004.09.014
218. Wong WY, Merkus HMWM, Thomas CMG, Menkveld R, Zielhuis GA, Steegers-Theunissen RPM. Effects of folic acid and zinc sulfate on male factor subfertility: a double-blind, randomized, placebo-controlled trial. Fertil Steril. (2002) 77:491–8. doi: 10.1016/S0015-0282(01)03229-0
219. Hochereau-de Reviers MT, Perreau C, Pisselet C, Pelletier J. Effect of a 2-month light cycle regimen on testicular parameters of adult Ile-De-France rams. Micros Res Techn. (1992) 20:268–73. doi: 10.1002/jemt.1070200306
220. Heller CG, Clermont Y. Spermatogenesis in man: an estimate of its duration. Science. (1963) 140:184–6. doi: 10.1126/science.140.3563.184
221. Haris FZ, Ondho YS, Samsudewa D, editors. Effect of vitamin E addition to frozen simmental bull semen extender on post-thawing quality. In: E3S Web of Conferences. Jember: EDP Sciences (2020).
222. Rostini T, Zakir I, Biyatmoko D. The effect of zinc-biocomplex and vitamin E supplementation on local kacang goats semen. Am J Anim Vet Sci. (2020) 15:175. doi: 10.3844/ajavsp.2020.169.175
223. Chakurkar EB, Naik SS, Barbuddhe SB, Karunakaran M, Naik PK, Singh NP. Seminal attributes and sperm morphology of agonda goan pigs. J Appl Anim Res. (2016) 44:130–4. doi: 10.1080/09712119.2015.1021807
224. Sultan MA, Abd El-Razek EM, Zaghloul AH, Genedy TM, Elbaz HT. Some parameters of stallion semen ejaculates during different seasons. J Curr Vet Res. (2023) 5:283–90. doi: 10.21608/jcvr.2023.296182
225. Jeyendran RS, Karuhn RF, Van Der Ven HH, Perez-Pelaez M. Volumetric analysis of human spermatozoa. Andrologia. (1987) 19:54–7. doi: 10.1111/j.1439-0272.1987.tb01859.x
226. Lemma A, Shemsu T. Effect of age and breed on semen quality and breeding soundness evaluation of pre-service young bulls. J Reprod Infertil. (2015) 6:35–40. doi: 10.5829/idosi.jri.2015.6.2.94131
227. Sultana F, Husain S, Khatun A, Apu A, Khandoker M. Study on buck evaluation based on semen quality and fertility. Banglad J Anim Sci. (2013) 42:101–8. doi: 10.3329/bjas.v42i2.18487
228. Al-Juwari MF, Al-Shaar NM, Ibrahim MS. Study of some reproductive parameters and sexual behavior in awassi rams: a review. Mesopot J Agric. (2022) 50:1196. doi: 10.33899/magrj.2022.135812.1196
229. Miroshnikova OS, Naumenko SV. Comparative assessment of reproductive capacity of boar-sires depending on the breed and season. Ukrain J Vet Agric Sci. (2022) 5:23–8. doi: 10.32718/ujvas5-3.05
230. Janett F, Thun R, Niederer K, Burger D, Hässig M. Seasonal changes in semen quality and freezability in the warmblood stallion. Theriogenology. (2003) 60:453–61. doi: 10.1016/S0093-691X(03)00046-3
231. Cooper TG, Noonan E, von Eckardstein S, Auger J, Baker HWG, Behre HM, et al. World health organization reference values for human semen characteristics. Hum Reprod Update. (2009) 16:231–45. doi: 10.1093/humupd/dmp048
232. Gravance CG, Liu IKM, Davis RO, Hughes JP, Casey PJ. Quantification of normal head morphometry of stallion spermatozoa. Reproduction. (1996) 108:41–6. doi: 10.1530/jrf.0.1080041
233. Maree L, du Plessis SS, Menkveld R, van der Horst G. Morphometric dimensions of the human sperm head depend on the staining method used. Hum Reprod. (2010) 25:1369–82. doi: 10.1093/humrep/deq075
234. Wibowo S, Setiatin E, Kurnianto E. The relationship between sperm morphometry and sperm competition in local goats of central java, Indonesia. Media Peternakan Fakultas Peternakan Institut Pertanian Bogor. (2013) 36:179–84. doi: 10.5398/medpet.2013.36.3.179
235. Jiang H, Kwon J-w, Lee S, Jo Y-J, Namgoong S, Yao X-r, et al. Reconstruction of bovine spermatozoa substances distribution and morphological differences between Holstein and Korean native cattle using three-dimensional refractive index tomography. Sci Rep. (2019) 9:8774. doi: 10.1038/s41598-019-45174-3
236. Waheed MM, Ghoneim IM, Abdou MSS. Morphometric characteristics of spermatozoa in the arabian horse with regard to season, age, sperm concentration, and fertility. J Equine Vet Sci. (2015) 35:244–9. doi: 10.1016/j.jevs.2015.01.005
237. Rajashri M, Reddy KR, Kumari GA, Kumari NN, Kesharwani S, Srinivas G. Qualitative ultrastructural changes and morphometry of deccani sheep spermatozoa preserved with egg yolk citrate extender. J Appl Nat Sci. (2017) 9:1515–21. doi: 10.31018/jans.v9i3.1394
238. Kondracki S, Banaszewska D, Wysokińska A, Iwanina M. The effect of sperm concentration in the ejaculate on morphological traits of bull spermatozoa. Folia Biol. (2012) 60:85–91. doi: 10.3409/fb60_1-2.85-91
239. Lodovico P, Graciela Estela C, Marco F. New advances in intracytoplasmic sperm injection (Icsi). In:Bin W, , editor. Advances in Embryo Transfer. Rijeka: IntechOpen (2012). p. Ch. 7.
240. Stewart J. Calculus: Early Transcendentals. 7th ed. California: Brooks/Cole Cengage Learning (2012).
241. Yániz JL, Capistrós S, Vicente-Fiel S, Hidalgo CO, Santolaria P. A comparative study of the morphometry of sperm head components in cattle, sheep, and pigs with a computer-assisted fluorescence method. Asian J Androl. (2016) 18:840–3. doi: 10.4103/1008-682X.186877
242. Banaszewska D, Andraszek K, Szostek M, Wojcik E, Danilewicz A, Walczak-Jedrzejowska R. Analysis of stallion semiologic semen parameters. Med Weterynaryjna. (2015) 71.
243. Menkveld R, Franken DR, Kruger TF, Oehninger S, Hodgen GD. Sperm selection capacity of the human zona pellucida. Mol Reprod Dev. (1991) 30:346–52. doi: 10.1002/mrd.1080300409
244. Suwor F, Tongpae S, Nakharuthai C, Ponchunchoovong S. Effect of Cryoprotectants, Antioxidants and DNA Methylation on the Frozen Semen Quality of Anglo-Nubian Goat (2024). Available online at: https://ssrncom/abstract=4751175 (accessed April 3, 2024).
245. Ali N, Memon A, Kaka A, Mirani A, Panhwar M, Solangi N, et al. In-vitro and in-vivo fertilizing potential of kamohri buck semen frozen in tris-based egg yolk extender supplemented with selenium. J Anim Health Prod. (2024) 12:64–70. doi: 10.17582/journal.jahp/2024/12.1.64.70
246. Bucak MN, Karaşör ÖF, Sari A, Bodu M, Ili P, Narliçay S, et al. Lipid mixtures (from a liposome kit) and melatonin improve post-thawed angora goat sperm parameters. Cryobiology. (2024) 115:104897. doi: 10.1016/j.cryobiol.2024.104897
247. Salama MS, Shehabeldin AM, Ashour MA, Al-Ghadi MQ, Marghani BH, El-kon I, Shukry M. Effect of the addition of platelet-rich plasma to boer buck semen on sperm quality and antioxidant activity before and after cryopreservation and in vivo fertility. Small Rumin Res. (2024) 230:107167. doi: 10.1016/j.smallrumres.2023.107167
248. Khalique MA, Andrabi SMH, Majeed KA, Yousaf MS, Ahmad N, Tahir SK, et al. Cerium oxide nanoparticles improve the post-thaw quality and in-vivo fertility of beetal buck spermatozoa. Theriogenology. (2024) 214:166–72. doi: 10.1016/j.theriogenology.2023.10.022
249. Saratsi A, Samartzi F, Panagiotidis I, Basioura A, Tsiokos D, Ligda C, et al. Post-thaw parameters of buck semen quality after soy lecithin extender supplementation with fumaric acid. Vet Sci. (2023) 10:569. doi: 10.3390/vetsci10090569
250. Galián S, Peinado B, Almela L, Poto Á, Ruiz S. Post-thaw quality of spermatozoa frozen with three different extenders in the murciano granadina goat breed. Animals. (2023) 13:309. doi: 10.3390/ani13020309
251. Shah SAH, Haider MS, Ahmed H, Fayyaz MH, Andrabi SMH. Triladyl® improves the cryopreserved quality and in vivo fertilization potential of beetal buck (Capra Hircus) spermatozoa. Small Rumin Res. (2023) 221:106938. doi: 10.1016/j.smallrumres.2023.106938
252. Ghanem N, Nasr NH, Abu Elnaga NA, Abou-Hashim F, Kamel S, Warda M, et al. Molecular and physiochemical evaluation of buck semen cryopreserved with antioxidants. Reprod Dom Anim. (2023) 58:813–22. doi: 10.1111/rda.14354
253. Liang J, Larbi A, Lv C, Ali S, Wu G, Quan G. Fertility results after exocervical insemination using goat semen cryopreserved with extenders based on egg yolk, skim milk, or soybean lecithin. Reprod Dom Anim. (2023) 58:431–42. doi: 10.1111/rda.14304
254. Caamaño JN, Santiago-Moreno J, Martínez-Pastor F, Tamargo C, Salman A, Fernández Á, et al. Use of the flavonoid taxifolin for sperm cryopreservation from the threatened bermeya goat breed. Theriogenology. (2023) 206:18–27. doi: 10.1016/j.theriogenology.2023.05.004
255. Abedin SN, Baruah A, Baruah KK, Kadirvel G, Katiyar R, Khargharia G, et al. In vitro and in vivo studies on the efficacy of zinc-oxide and selenium nanoparticle in cryopreserved goat (Capra Hircus) spermatozoa. Biol Trace Elem Res. (2023) 201:4726–45. doi: 10.1007/s12011-022-03551-6
256. Esmaeilkhanian S, Asadzadeh N, Masoudi R. Flow cytometry study of post-thawed buck spermatozoa: mito-tempo improves cryopreservation performance by controlling apoptosis rate, DNA fragmentation and ros production. Cryobiology. (2023) 110:108–10. doi: 10.1016/j.cryobiol.2022.11.238
257. Akhondzadeh S, Farshad A, Rostamzadeh J, Sharafi M. Effects of antifreeze protein type I and glycerol in diluents on cryopreserved goat epididymal sperm. Biopreserv Biobank. (2022) 21:65–73. doi: 10.1089/bio.2021.0150
258. Karaşör ÖF, Bucak MN, Cenariu M, Bodu M, Taşpinar M, Taşpinar F. The effects of different doses of rock inhibitor, antifreeze protein III, and boron added to semen extender on semen freezeability of ankara bucks. Molecules. (2022) 27:8070. doi: 10.3390/molecules27228070
259. Dhara S, Gupta HP, Kumar S, Sharma RK, Thakur S. Effects of heterologous bovine seminal plasma-supplemented egg yolk-based extender on cryosurvivability of pantja buck semen. Biopreserv Biobank. (2022) 21:336–45. doi: 10.1089/bio.2022.0013
260. El-Khawagah ARM, Rawash ZM, El-Badry DA, Kandiel MM. Influence of butylated hydroxytoluene addition to cryodiluents on freezability and DNA integrity of boer and zaraibi buck spermatozoa. Asian Pac J Reprod. (2020) 9:96–103. doi: 10.4103/2305-0500.281079
261. Sun L, Fan W, Wu C, Zhang S, Dai J, Zhang D. Effect of substituting different concentrations of soybean lecithin and egg yolk in tris-based extender on goat semen cryopreservation. Cryobiology. (2020) 92:146–50. doi: 10.1016/j.cryobiol.2019.12.004
262. Igbokwe AA, Iyasere OS, Sobayo RA, Iyasere S, Animashaun RI, Balogun FA, et al. Comparative effect of slow and rapid freezing on sperm functional attributes and oxidative stress parameters of goat spermatozoa cryopreserved with tiger nut milk extender. Reprod Dom Anim. (2019) 54:551–9. doi: 10.1111/rda.13393
263. Lv C, Larbi A, Wu G, Hong Q, Quan G. Improving the quality of cryopreserved goat semen with a commercial bull extender supplemented with resveratrol. Anim Reprod Sci. (2019) 208:106127. doi: 10.1016/j.anireprosci.2019.106127
264. Gororo E, Zulu PT, Chatiza FP, Mhuka C. Effects of different extenders and storage temperatures on longevity of small east african goat (Capra Hircus) semen. Small Rumin Res. (2019) 175:83–9. doi: 10.1016/j.smallrumres.2019.04.013
265. Pawshe M, Harshan H, John A, Kurein M, Sunanda C. Evaluation of extenders for cryopreservation of malabari buck semen. Ind J Anim Reprod. (2018) 39:52–4.
266. Swelum AA-A, Saadeldin IM, Alanazi MB, Ba-Awadh H, Afifi M, Alowaimer AN. Effects of adding egg yolks of different avian species to tris glycerol extender on the post-thawing quality of buck semen. Anim Reprod Sci. (2018) 195:345–54. doi: 10.1016/j.anireprosci.2018.06.016
267. El-Battawy K, El-Nattat W. Cryopreservation of buck semen with emphasis on its chilling and its characteristics after some amino acids supplementation. Curr Sci Int. (2018) 7:731–6. doi: 10.3390/ani12233271
268. Yousefian I, Emamverdi M, Karamzadeh-Dehaghani A, Sabzian-Melei R, Zhandi M, Zare-Shahneh A. Attenuation of cryopreservation-induced oxidative stress by antioxidant: impact of coenzyme Q10 on the quality of post-thawed buck spermatozoa. Cryobiology. (2018) 81:88–93. doi: 10.1016/j.cryobiol.2018.02.005
269. Narwade B, Mohanty TK, Bhakat M, Rahim A. Goat semen cryopreservation using egg yolk and soya based extenders containing trehalose. Ind J Anim Sci. (2017) 87:851–5. doi: 10.56093/ijans.v87i7.72229
270. Seifi-Jamadi A, Ahmad E, Ansari M, Kohram H. Antioxidant effect of quercetin in an extender containing dma or glycerol on freezing capacity of goat semen. Cryobiology. (2017) 75:15–20. doi: 10.1016/j.cryobiol.2017.03.002
Keywords: sperm, cryopreservation, extender, antioxidants, omics, sustainable goat production
Citation: Bodu M, Hitit M, Greenwood OC, Murray RD and Memili E (2025) Extender development for optimal cryopreservation of buck sperm to increase reproductive efficiency of goats. Front. Vet. Sci. 12:1554771. doi: 10.3389/fvets.2025.1554771
Received: 02 January 2025; Accepted: 27 February 2025;
Published: 02 April 2025.
Edited by:
Eliana Pintus, Czech University of Life Sciences Prague, CzechiaReviewed by:
Alfredo Medrano, National Autonomous University of Mexico, MexicoCopyright © 2025 Bodu, Hitit, Greenwood, Murray and Memili. This is an open-access article distributed under the terms of the Creative Commons Attribution License (CC BY). The use, distribution or reproduction in other forums is permitted, provided the original author(s) and the copyright owner(s) are credited and that the original publication in this journal is cited, in accordance with accepted academic practice. No use, distribution or reproduction is permitted which does not comply with these terms.
*Correspondence: Mustafa Bodu, bWJvZHVAc2VsY3VrLmVkdS50cg==; Mustafa Hitit, bXVoaXRpdEBwdmFtdS5lZHU=; Erdogan Memili, ZXJtZW1pbGlAcHZhbXUuZWR1
Disclaimer: All claims expressed in this article are solely those of the authors and do not necessarily represent those of their affiliated organizations, or those of the publisher, the editors and the reviewers. Any product that may be evaluated in this article or claim that may be made by its manufacturer is not guaranteed or endorsed by the publisher.
Research integrity at Frontiers
Learn more about the work of our research integrity team to safeguard the quality of each article we publish.