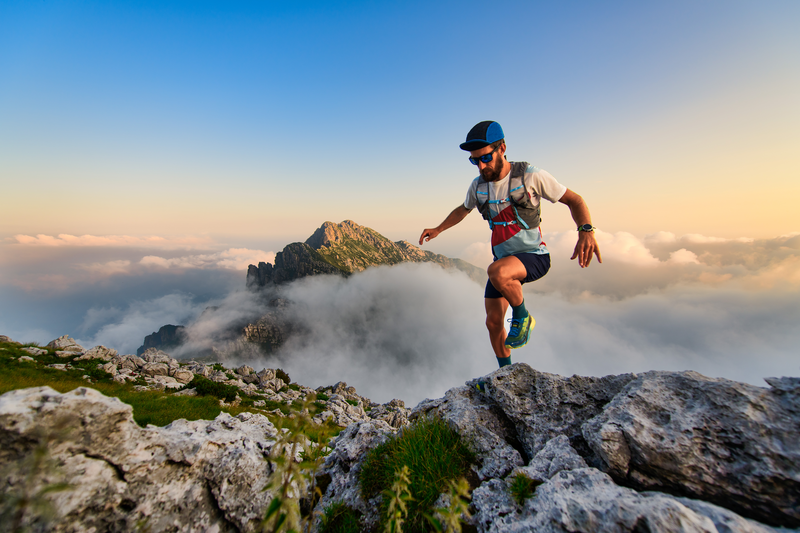
94% of researchers rate our articles as excellent or good
Learn more about the work of our research integrity team to safeguard the quality of each article we publish.
Find out more
ORIGINAL RESEARCH article
Front. Vet. Sci. , 06 March 2025
Sec. Animal Behavior and Welfare
Volume 12 - 2025 | https://doi.org/10.3389/fvets.2025.1538195
This article is part of the Research Topic Advances in the Application of Technology for Monitoring Horse Welfare and Health View all 8 articles
Introduction: Several studies have explored alternatives to enhance the performance, health, and safety of sports horses. One promising method involves the use of vibrating platforms (VP), which offer passive exercise stimulation via mechanical oscillations distributed throughout the body. This type of exercise is referred to as whole-body vibration (WBV) and is an emerging strategy for accelerating muscle recovery. This study examined the dynamics of proteins responsible for transporting monocarboxylates (MCT1 and MCT4), and their relationship with lactatemia and acid-base balance in connection with WBV recovery following intense treadmill exercise in horses.
Methods: Eight crossbred horses underwent the standardized exercise test on the treadmill to determine the velocity corresponding to the lactate threshold. This velocity was used to prescribe the external load of the acute intense exercise bout (AIEB), which was performed to recruit rapidly fatigable type II muscle fibers and induce hyperlactatemia and metabolic acidosis. The horses were assigned to three experimental groups in a crossover design, with a 7-day washout period. The treadmill group (TG) actively recovered through low-intensity treadmill walking. The WBV group (WBVG) followed a stepwise recovery protocol on VP, with each step lasting 2 min and the frequencies decreasing in a specific order: 76, 66, 55, 46, and 32 Hz. The sham group (SG) was designated for horses with the VP turned off. All groups experienced a uniform recovery strategy duration of 10 min. Heart rate (HR), rectal temperature (RT), lactatemia, glycemia, acid-base status and electrolytes, strong ion difference (SID), and muscle monocarboxylate transporters (MCT1 and MCT4), were assessed.
Results: AIEB induced positive chronotropic effects, hyperlactatemia and moderate metabolic acidosis in all experimental groups. All groups also showed transitory hyperthermia, hyperglycemia, hypernatremia, hyperchloremia, hyperkalemia and SID reduction. HR was higher in TG than in the WBVG and SG immediately after the recovery procedures. Between the groups, there was no change in RT, lactatemia, glycemia and MCT1 and MCT4 content. Regardless of groups, the MCT4 content decreased 3 and 6 h after recovery strategies.
Discussion: It was concluded that a single whole-body vibration session did not enhance recovery of lactatemia or acid-base balance in horses after intense treadmill exercise.
Improving the quality and safety of athletic performance is an important scientific topic for humans and other animal species. Equestrian associations aim to enhance the athletic ability, health and wellbeing of horses involved in sports. This field encompasses various research areas, including whole-body vibration (WBV). WBV is considered a non-invasive and non-pharmacological rehabilitation and recovery strategy, which uses vibrating platforms that produce mechanical vibrations, exposing the organism to a mechanotransduction process, and has been studied in humans (1–10) and in rodents (11–15) as a training device, to improve involuntary neuromuscular activation, biomechanics, and physiological variables related to performance (16–18).
It is vital to understand that improper use of vibration therapy can lead to adverse effects in men, including skin erythema, edema, pain (19), hematuria (20), knee discomfort (21, 22), and fatigue (21). Furthermore, research has shown progressive intervertebral disc degeneration in mice (23) and worsened chronic lameness in horses after extended WBV sessions (24) and intensified thoracolumbar pain with short-term use (25). Customizing vibration parameters such as amplitude, frequency, and oscillation magnitude is essential, as individual responses vary (16).
Recent studies demonstrate the benefits of WBV in addressing obesity and enhancing physical performance. One study found that WBV decreased lactate, ammonia, and serum creatine kinase activity, while increasing blood glucose in rats subjected to swimming and WBV, indicating its potential to alleviate fatigue (11). Another study on middle-aged mice revealed that WBV improved aerobic fitness and reduced fatigue (12). Research on combining dehydroepiandrosterone supplementation with WBV in mice showed remarkable results, including enhanced exercise performance, increased serum testosterone and blood glucose, and higher liver and muscle glycogen (14). These findings further emphasize WBV's role in boosting fitness and overall wellbeing.
Isometric squat training for healthy active males on a vibrating platform effectively increases time to exhaustion and reduces neuromuscular fatigue in knee extensor muscles (8). Research indicates that combining physical exercise with whole-body vibration (WBV) lowers lactate levels, heart rate (HR), and blood pressure (2, 4, 5). However, a study on recreational runners using WBV and drop jumps as warm-ups found no significant changes in ankle dorsiflexion or running times (10). Similarly, a study on horses assessed the effects of WBV as a warm-up strategy on various physiological variables and found no significant results (26), underscoring the complexity of WBV's effectiveness in different contexts.
Methods to restore homeostasis after intense exercise, particularly in terms of body temperature recovery, hyperlactatemia, and metabolic acidosis, are gaining attention in equine studies (27–33). In the horse industry, WBV is proposed as a technique to accelerate recovery post-exercise. While manufacturers endorse this method (34), its use in equestrian centers often lacks scientific evidence, at least to our knowledge. We hypothesize that WBV enhances lactate clearance through monocarboxylate transporters, facilitating the transport of L-lactate across the sarcolemma and restoring acid-base balance after vigorous exertion. It should be highlighted that this paper seeks to improve the abilities of veterinarians, researchers, and horse owners in maintaining horses' health and wellbeing by bridging the gap between experimental research on WBV and real-world applications.
The study was approved by the Ethics Committee on the Use of Animals (CEUA) of the School of Agricultural and Veterinarian Sciences UNESP-Jaboticabal Campus (protocol no. 08373/19) and carried out during October and November 2022 at GPS coordinates-21.245408 latitude and−48.299415 longitude.
Eight crossbreed horses were used (one gelding and seven females), with an average body mass of 406 ± 33 kg and aged between 7 and 19 years. The horses belonged to the didactic herd of the Equine Exercise Physiology and Pharmacology Laboratory (LAFEQ), Department of Animal Morphology and Physiology, School of Agricultural and Veterinarian Sciences, São Paulo State University (FCAV/UNESP), Jaboticabal, São Paulo, Brazil. The horses were included in the study after ~2 months of adaptation to the experimental conditions, such as handling and feeding, treadmill (Galloper G 5500, Sahinco, Palmital, SP) and vibrating platform (TheraPlate®, Weatherford, TX, USA). The horses were kept in paddocks and fed with Tifton 85 hay, in addition to 0.2% of body mass in concentrate once a day. Mineralized salt and water were provided ad libitum. They were regularly hoofed, and, during the experimental period, they did not use horseshoes. Before the start of the experimental stages, the horses underwent clinical and hematological examinations to determine their healthiness and were previously vaccinated and treated with anthelmintics.
A standardized exercise test (SET) was conducted on all horses to determine the velocity corresponding to the lactate threshold (VLT), which was used to prescribe the external load for an acute intense exercise bout (AIEB). The SET protocol was adapted from Lamprecht and Williams (35) and performed on a treadmill. The warming-up consisted of 3 min of walking at a speed of 1.5 m/s with a surface inclination of 0%, followed by 2 min of trotting at 2.5 m/s and 5% positive slope. The SET started at 4.0 m/s, with the speed increasing by 1.0 m/s at 2-min intervals. The horses underwent a 2-min active recovery period at 1.5 m/s between each speed step. A surface inclination of 5% was maintained throughout the incremental phase. To ensure the horses' safety, the SET concluded when the horses reached a heart rate of 200 bpm or at the end of the speed stage of 9 m/s. At the end of the SET, the horses cooled down by walking at a comfortable speed for everyone, with an inclination of 0% (Supplementary Table S1).
Two complementary methods were used to determine the velocities corresponding to the lactate threshold: visual (VLTV) and bi-segmented (VLTBI). During the SET, the inflection point of the lactate-velocity curve was determined by analyzing the plasma lactate concentrations of each horse, indicating a non-linear increase. Four exercise physiology experts established this point. They then associated this point with the corresponding speed (x-axis) to establish the velocity corresponding to the visual lactate threshold (VLTV). This method was refined by applying bi-segmented linear regression (VLTBI). Two regression lines from the ordinary least squares regression were used, and the VLTV obtained was considered. By equating the values of the two equations related to the y-values, the speed (x) was obtained. To ensure that the external load of AIEB would recruit rapidly fatigable type II muscle fibers and induce physiologic hyperlactatemia and metabolic acidosis, the highest speed obtained between VLTV and VLTBI for everyone was used, and the values are presented in Supplementary Table S2.
All horses performed AIEB with an external load above the VLT. Initially, the horses were subjected to a warming-up period of 1 min at a speed of 1.5 m/s and 0% inclination, followed by 2 min at a speed of 3.5 m/s and 2 min at VLT, both with 5% inclination. To intensify the external load, aiming to induce hyperlactatemia and metabolic acidosis, the AIEB was completed in the gallop, with 2 min at 110% of the VLT and 3 min at 130% of the VLT, with an inclination of 5%. Once the AIEB was finished, the recovery phase commenced to assess the suggested strategies for recovery.
Before the experiment began, the horses were randomly assigned into three experimental groups, in a crossover design, each specifically designed to evaluate a different type of recovery after AIEB. All horses participated in all three experimental groups, carried out in three blocks. The randomization process involved numbering the animals from 1 to 8, followed by a drawing to create the experimental blocks (1, 2, and 3) using an Excel spreadsheet with the formula =RANDBETWEEN(1;8). All strategies for recovery were meticulously planned and executed, each lasting 10 min. The treadmill group (TG) performed the active recovery period on the treadmill at a comfortable speed for each horse (between 1.2 and 1.6 m/s). The horses in the sham group (SG) remained on the turned-off vibrating platform, providing a control group for the experiment. The whole-body vibration group (WBVG) performed one WBV session during recovery. The horses in the SG and WBVG groups began their recovery roughly 5 min after the end of AIEB. For the TG group, the horses waited 5 min on the treadmill before the active recovery period began, further ensuring the scientific rigor of the experiment.
The horses in the WBVG group underwent a WBV session after AIEB, using the Theraplate® (Original Equine Unit Model K21, Theraplate, Weatherford, TX, USA). This VP, equipped with a unique “proprietary technology” called vortex wave stimulation, utilizes centrifugal force and internal oscillating movement to provide zero-impact therapy. The horses were maintained in a quadrupedal position on the VP. The WBV recovery protocol, developed according to the manufacturer's guidelines from TheraPlate (TPR, LLC), was implemented for horse recovery following AIEB. This protocol lasted 10 min and was designed to gradually decrease the frequency levels. It included 2 min at each load corresponding to 100%, 80%, 60%, 40%, and 20% of the equipment's maximum motor capacity, which correspond to 76 Hz [peak displacement (Dpeak) = 0.09 mm; peak acceleration (Apeak) = 10.36 m/s2], 66 Hz (Dpeak = 0.12 mm; Apeak = 9.96 m/s2), 55 Hz (Dpeak = 0.16 mm; Apeak = 9.46 m/s2), 46 Hz (Dpeak = 0.22 mm; Apeak = 9.12 m/s2), and 32 Hz (Dpeak = 0.51 mm; Apeak = 10.35 m/s2), respectively. The frequency and peak acceleration were measured using an accelerometer (App Accelerometer meter). The peak displacement was calculated using the formula:
HR was measured using a Polar Equine Heart Rate Monitor for Trotters (Polar Electro, Kempele, Finland). The peak HR for each stage was extracted from the Polar Flow® app (https://flow.polar.com/). The data were collected before (baseline) and after (A) AIEB, before (BR) and after (AR) recovery, and 10 min at the end of the recovery period (10 min).
A digital predictive thermometer (Clean View RM-TD0403A, Relaxmedic, Vargem Grande Paulista, Brazil) was used to measure RT. Temperature readings were taken at three time points: baseline, A, and AR.
Blood samples were collected through jugular vein catheterization in tubes containing sodium fluoride and EDTA to determine the plasma concentration of lactate and glucose. The samples were centrifuged, and the plasma was separated and immediately analyzed. The analyses were carried out using the electroenzymatic method with an automatic bioanalyzer (YSI 2300 Stat Plus®, Ohio-USA). Samples were obtained at the following time points: baseline, A, BR, AR, 10 min, and 1 h after the end of the recovery period.
Blood was collected through jugular vein catheterization to analyze blood gases and electrolytes, avoiding contact with environmental oxygen and carbon dioxide, in 3.0 mL blood gas syringes. Samples were collected at three-time points: baseline, A, and AR. Analyses were performed immediately after obtaining the samples using a portable analyzer (i-STAT Analyzer, Abbott Laboratories, Libertyville Township, IL, USA), cartridge EC8+, which contains tests for electrolytes, chemistries, blood gases, hematocrit, and hemoglobin. The variables measured were pH, venous partial pressure of carbon dioxide (PVCO2), total carbon dioxide (tCO2), base excess/deficit (BEecf), anion gap (AnGap), bicarbonate (), sodium ion (Na+), potassium ion (K+), chloride ion (Cl−), total hemoglobin (Hb), hematocrit (Hct) and urea nitrogen (Ure). pH and PVCO2 values were adjusted for rectal temperature (36, 37). The strong ion difference (SID) is calculated as the difference between the total concentration of strong cations and the total concentration of strong anions, using the formula:
The horses were subjected to muscle biopsies at the following time points: baseline (around 18 h before AIEB), AR, 3 and 6 h after recovery, as described previously (38). The horses were restrained in a quadrupedal position on a horse stock before biopsy sampling. The needle insertion site in the gluteus medius (gluteal) was in the middle third between the coxal tuberosity and the tailhead. Collections were alternated between the right and left sides of the gluteus medius muscles. The sample collection site was shaved and cleaned with chlorhexidine before being rinsed with 70% ethanol. A local anesthetic block was then applied with subcutaneous infiltration of 3 mL of 2% lidocaine hydrochloride without vasoconstrictor. After 5 min, an incision was made in the skin, subcutaneous tissue and gluteal fascia at the needle insertion site using a sterile disposable #24 scalpel blade. Next, the sterile 6.0-mm Bergström-type needle was introduced into the previously made incision to a depth of 60 mm at a 90° angle to obtain the muscle fragments. The muscle samples were immediately snap-frozen in liquid nitrogen and later stored in a −80°C freezer until the analysis.
The analyses were performed based on a previous study in mice (39).
The samples (25 mg) were homogenized, through maceration, in 300 μL of RIPA buffer with the following components: 1% Tris HCl (50 mM), NaCl (150 mM), EDTA (1 mM), IGEPAL® CA-630 (1%), Deoxycholate (0.5%), SDS (0.1%), 1% protease inhibitor (Protease and Phosphatase Inhibitor Cocktail, cat# P8340, Sigma Aldrich®) and 1% phosphatase inhibitor (Phosphatase II Inhibitor Cocktail Set, cat# US1524625-1SET, Calbiochem®, San Diego, CA, USA), ensuring the highest quality of protein preservation. Then, the samples were placed in a sonicator (Q55 Sonicator, Qsonica®, Newtown, CT, USA) twice for 5 s (60%). Subsequently, the lysates were centrifuged at 12,000 rpm for 10 min at 4°C (Centrifuge 5424 R, Eppendorf AG, Hamburg, Germany). Soon after, the supernatant was separated (total protein extract), and the pellet was discarded. The Bradford colorimetric method was used to quantify total proteins. The absorbance was read at a wavelength of 595 nm using a spectrophotometer.
The samples (40 μg of total protein) were mixed with LDS buffer (lithium dodecyl sulfate and 1% mercaptoethanol) and taken to a dry bath at 94°C for 10 min. Then, the samples were placed on ice for 5 min. Next, 9 μL of each sample was pipetted into the gel wells (Mini Protean TGX Precast Protein Gels, 10% 15-well, Bio-Rad®), and then 7.5 μL of weight molecular marker was pipetted in the first well of the gel. Electrophoresis was performed using specific equipment (PowerPac 300 Electrophoresis Power Supply—Bio Rad, São Paulo, SP, Brazil) at a constant 130 V for ~60 min. An iBlot™ 2 Gel Dry Transfer Device (20 V for 7 min cat# IB21001, Waltham, MA, USA) was used to transfer proteins (PVDF) to the membrane (Invitrogen™, iBlot™ 2 Transfer Stacks cat# IB # IB24002, Waltham, MA, USA).
Membranes were subjected to fluorescence staining (RevertTM total protein stain, cat # 926-11010) and scanned using the 700 nm channel of the LI-COR Odyssey Fc imaging system. Afterwards, blocking was performed in 1% milk (skimmed milk powder, cat# 9999, Cell Signaling Technology®, Danvers, MA, USA) in PBS (10 mL of PBS, 0.1 g of NFDM milk) for 1 h on an orbital shaker. Then, the membranes were immediately incubated with 5% milk in PBS-Tween for 1 h at room temperature (for MCT4 antibody, cat #BS-2698R, Bioss, Woburn, MA, USA) or overnight at 4°C (for MCT1 antibody, cat #20139-I-AP, Proteintech, Rosemont, IL, USA). Antibody binding was detected by preabsorbed goat anti-rabbit IgG H&L (cat. #ab216773) (IRDye 800CW) at a dilution of 1:20,000 for 1 h at room temperature in the dark. Fluorescence was detected at 800 nm using the same imaging system (Odyssey Fc, LI-COR Biosciences, Lincoln, NE, USA). For accurate results, protein expression was normalized by dividing the antibody signal by the band normalization factor.
The experiment was designed with a 3 × 4 factorial design, with 3 treatments (TG, WBVG, SG) and 4 timepoints (baseline, AR, 3 h, 6 h). It included three paired replications and a split-plot scheme. We used a paired randomized crossover design, allowing horses to experience three recovery strategies, each followed by a vital 7-day washout period. Muscle biopsy assessments were used for the proposed model. Statistical analysis was conducted using RStudio software for Windows (version 2023.06.0+421 “Mountain Hydrangea”), which uses the format “function {package}” to present functions and packages. Normality of residuals was assessed using the Shapiro-Wilk test (shapiro_test {rstatix}) and QQ graphs (qqplot {stats}). The Levene test (leveneTest {car}), considered to be more robust against potential deviations from normality, was applied to evaluate the homoscedasticity of variances. A two way ANOVA for repeated measures (anova_test {rstatix}) and paired t-tests with Bonferroni correction (pairwise_t_test {rstatix} with argument “p.adjust.method = bonferroni”) were used for statistical analysis. To evaluate potential improvements in aerobic fitness throughout the experiment, we assessed aerobic fitness between blocks (periods) using one way ANOVA for repeated measures (anova_test {rstatix}), followed by post-hoc paired t-tests. We applied the Bonferroni correction (pairwise_t_test {rstatix} with argument “p.adjust.method = bonferroni”) to reduce the risk of false-positive results (type I error) in our heart rate and plasma lactate measurements. The use of Bonferroni correction is warranted due to the large number of comparisons made after each ANOVA. This method ensures that the significance level remains consistent across all comparisons (40). The area under the curve (AUC) (auc {MESS}) for plasma lactate, MCT1 and MCT4 was determined using all samples obtained by the trapezoidal method for numerical integration (41). The presence of linear relationships between the variables was evaluated by calculating the Pearson correlation coefficient (r) (cor {stats}). Based on the value obtained, the following degrees of correlation were defined as r = 0, absence of correlation; r < ±0.29, weak correlation; ±0.3 < r < ±0.49, moderate correlation; ±0.5 < r < ±0.79, strong correlation; r > ±0.8, very strong correlation. We used a significant level of 5% for all analysis.
Aiming to verify a possible increase in aerobic fitness throughout the experiment, the variables heart rate and plasma lactate were checked, right after the end of AIEB, and there was no difference between blocks (P = 0.114, for HR and P = 0.36, for lactate), indicating that there was no gain in physical conditioning throughout the experimental trial course (Figure 1; Supplementary Table S3). It is worth noting that all horses used in the current study were previously conditioned. No horses were interrupted during the test attributable to fatigue, as indicated by their ability to maintain speed on the treadmill and preserve motor coordination.
Figure 1. Graphical representation of median, interquartile range and means ± standard deviation (pointrange) of (A) plasma lactate and (B) heart rate of horses (n = 8) after an acute intense exercise bout in each experimental block. For more details see Supplementary Table S3.
The HR average values are shown in Supplementary Table S4. As expected, AIEB induced positive chronotropic responses in all experimental groups. After implementing recovery strategies, the HR was higher (P = 0.001) in the TG compared with the other groups (Figure 2).
Figure 2. Graphical representation of means ± standard deviation of heart rate of horses (n = 8) submitted to an acute intense exercise bout (AIEB) and a 10-min recovery period on the treadmill (TG), on a whole-body vibration session (WBVG), or the vibrating platform off (SG). #Indicates that HR was higher in TG concerning SG and WBVG at a significance level of P < 0.05. A, after AIEB; BR, before recovery; AR, after recovery; 10 min, 10 min after the end of the recovery period. For more details see Supplementary Table S4.
The RT of the animals in all experimental groups increased after AIEB (P = 0.037; Supplementary Table S5). There was no difference between groups at any time point (P = 0.876; Figure 3; Supplementary Table S5).
Figure 3. Graphical representation of median, interquartile range and means ± standard deviation (pointrange) of the rectal temperature of horses (n = 8) submitted to an acute intense exercise bout (AIEB) and a 10-min recovery period on the treadmill (TG), on a whole-body vibration session (WBVG), or the vibrating platform off (SG). A, after AIEB; BR, before recovery; AR, after recovery. For more details see Supplementary Table S5.
Although there was a trend toward an increase in lactatemia (P = 0.08) for SG, there was clearly physiological hyperlactatemia in all groups immediately after AIEB, which decreased over time, regardless of recovery strategy (Figure 4A; Supplementary Table S6). It should be emphasized that there is no difference between groups at any time for glycemia (P = 0.488) and lactatemia (P = 0.352; Figure 4; Supplementary Table S6). Plasma glucose peaked five (BR) to 15 min (AR) after AIEB, returning to baseline values 1h after all recovery strategies (Figure 4B; Supplementary Table S6).
Figure 4. Graphical representation of means ± standard deviation of (A) plasma lactate and (B) plasma glucose of horses (n = 8) submitted to an acute intense exercise bout (AIEB) and a 10-min recovery period on the treadmill (TG), on a whole-body vibration session (WBVG), or the vibrating platform off (SG). A, after AIEB; BR, before recovery; AR, after recovery; 10 min, 10 min after the end of the recovery period. For more details see Supplementary Table S6.
Except for PvCO2 and Ure, all blood gas variables showed significant changes after AIEB for all experimental groups, indicating metabolic acidosis (Table 1). Between the groups, a higher Ure was identified in the WBVG in relation to the TG at baseline (P = 0.01). It is worth noting that the average values of Ure remained within the reference range for the equine species (42).
Table 1. Means ± standard deviation of blood gases and electrolytes of horses submitted to an acute intense exercise bout (AIEB) and a 10-minute recovery period on the treadmill (TG), on a whole-body vibration session (WBVG), or the vibrating platform off (SG).
There was no difference between groups (P = 0.705) or overtime (P = 0.485) for MCT1 (Figure 5A; Supplementary Table S7). No difference between groups at any time (P = 0.298) was detected for MCT4. There was a decrease in MCT4 protein content 3 and 6 h after recovery for all experimental groups (P = 0.007; Figure 5B; Supplementary Table S7).
Figure 5. Graphical representation of median, interquartile range and means ± standard deviation (pointrange) of the (A) MCT1 protein content and (B) MCT4 protein content in the gluteal muscle of horses (n = 8) submitted to an acute intense exercise bout (AIEB) and a recovery period on the treadmill (TG), on a whole-body vibration session (WBVG), or on the vibrating platform off (SG). Representative images of the membranes with protein bands are shown. All membranes were stained for total protein to normalize differences between protein labeling bands. *Indicates difference between moments (P < 0.05). **Indicates difference between moments (P < 0.01). A, after AIEB; BR, before recovery; AR, after recovery; 3 h, 3 h after the end of recovery; 6 h, 6 h after the end of recovery.
None of the AUCs showed any differences between groups for any variable (Figure 6; Supplementary Table S8). Likewise, no correlation was significant (Figure 7; Supplementary Table S8).
Figure 6. Graphical representation of area under the curve (AUC) of (A) plasma lactate, (B) MCT1 protein content and (C) MCT4 protein content of horses (n = 8) submitted to an acute intense exercise bout (AIEB) and a 10-min recovery period on the treadmill (TG), on a whole-body vibration session (WBVG), or the vibrating platform off (SG).
Figure 7. Correlogram of plasma lactate, MCT1 protein content and MCT4 protein content of horses (n = 8) submitted to an acute intense exercise bout (AIEB) and a 10-min recovery period on the (A) vibrating platform off (SG), (B) treadmill (TG), or (C) on a whole-body vibration session (WBVG).
The importance of researching WBV's role in aiding horses to regain internal homeostasis should be emphasized. The current study is paving the way to identify rational therapeutic targets for WBV in horses. Contrary to our hypothesis, the results obtained regarding recovery from exercise-induced hyperlactatemia and acidosis using the WBV were not different from those horses walking on the treadmill or those of the horses placed on the VP turned off. Also, this research sheds light on the relationship between WBV, horses, and muscle lactate recovery after intense exercise. It is also important to highlight that the application of WBV was considered safe, and no adverse effects were observed throughout the current study.
The existing literature on WBV in horses has primarily focused on the effects of WBV on clinical and blood parameters after a single session (43–45), neuromuscular activation (26), use as a warming-up exercise (26), its impact on lameness (24, 44), symmetry and muscle area (25, 46), hoof growth (47), stride length (44, 45), bone mineral content (45), postural stability (25), and thoracolumbar pain (25). No studies in horses have delved into the effects of an acute WBV session as a method to stimulate the transfer of lactate between white, glycolytic muscle fibers, which produce lactate, and red, oxidative muscle fibers, which consume lactate, within the working muscle (48) and a decrease in its concentration from the bloodstream after a high-intensity exercise session, which could help improve the body's acid-base balance (49).
The exercise bout was performed above the individual lactate threshold intensity, leading to increased muscular lactate production, inducing hyperlactatemia. One important performance-related marker is the lactate threshold, which correlates strongly with endurance performance and has proven sensitive to different acute exercise prescriptions. It characterizes the boundary between the heavy and severe exercise intensity domains, representing the threshold at which fast-twitch type II muscle fibers are more activated and the glycolytic pathway hyperactivate lactate production (50). This approach may provide a standard design for further studies focusing on the relationship between whole-body vibration and the acceleration of lactatemia and acid-base status recovery in exercising horses.
The use of WBV has increased in the equestrian industry to improve the performance and health of horses. However, little published research supports its use, and it is unclear what vibration magnitude is required for noticeable changes. The studies have shown mixed results, with some studies showing beneficial effects, others showing no effect and others showing adverse effects. The varying results are caused by differences in study protocols and designs and a lack of standardization in the physical aspects of the device, such as frequency, amplitude, and oscillation magnitude (18, 26, 51). In most studies involving horses, crucial details about vibratory intervention are often missing, such as the type and direction of vibration, the actual frequency, and the extent of vibration (displacement). This lack of information makes it difficult to compare data. However, these parameters are essential for evaluating vibratory intervention (51, 52).
During intense exercise, increased sympathetic activity in the nucleus tractus solitaries leads to elevated secretion of adrenal hormones (53). From an integrative perspective, the AIEB promoted transient adjustment in the homeostasis of the physiological variables studied herein, such as hyperlactatemia, mild acidosis, hyperglycemia, positive cardiac chronotropic, and elevated body temperature across the three recovery strategies trialed. Studies in humans have shown lower lactate concentrations in individuals who cooled down with WBV (2, 4, 5), indicating that WBV can lead to more efficient recovery from exercise attributable to continuous stimulation of blood vessels in the muscle. This stimulus could increase the use of lactate as energy for activated cardiac muscle and skeletal muscles and cause faster distribution of lactate to the liver (2). The findings indicated no difference between recovery strategies, demonstrating that the WBV protocol was ineffective in enhancing muscle and plasma lactate clearance.
Another way to accelerate lactate clearance would be via lactate transporters (MCTs). This mechanism would assist in a reduction of the plasma lactate concentration, which has been speculated to help the lactate outflow from type II muscle fibers (54). WBV also did not modify the MCT1 and MCT4 protein gluteus medius content, mainly expressed in oxidative and glycolytic fibers, respectively. It should be noted that this mechanism could help lactate exchanges between white-glycolytic and red-oxidative fibers and extracellular spaces (48, 55). Although muscle contractile activities can stimulate the gene and protein expression of MCTs in horses (56), a few studies have examined the effects of a single aerobic/anaerobic burst on MCTs (38). As traditionally reported, these authors found that both MCT1 and MCT4 increased following a single bout of maximal incremental exercise test. Aside from horses, this finding has already been described in rodents (57, 58) and humans (59). WBV did not alter lactate shutting through monocarboxylate transporters. Unexpectedly, MCT4 content decreased after AIEB in all recovery scenarios. Similarly, a human study reported that high-intensity exercise acutely decreased the amount of MCT4 protein in the sarcoplasm (60). These fortuitous findings may be caused by differences in exercise intensity, duration, testability, or sample preparation methods (61). Furthermore, it has been demonstrated that the expression of MCT is influenced by the breed of the horse (62, 63).
The results obtained showed the interference of exercise on blood gases and electrolytes, being that the AIEB protocol was of high intensity, characterized by the mobilization of the glycolytic pathway with the development of hyperlactatemia and metabolic acidosis, considering the findings of plasma lactate, pH, BEecf, and SID. High-intensity exercise leads to sharp increases in [H+], which leads to decreased pH and reduced and tCO2 (64). Racehorses that underwent 2 min of high-intensity trotting showed decreased SID on jugular venous blood, mainly responsible for the observed plasma acidosis. The reduction in SID resulted from increased lactate, Na+, K+, and Cl− (65, 66). The decrease in SID observed in the present study was mainly induced by the increase in lactate production caused by intense exercise.
Our study found no changes in PvCO2, consistent with a former study on intense treadmill exercise that suggested compensatory hyperventilation (67). In this study, values after exercise were lower than baseline values, like what Miranda et al. (67) observed, compatible with buffering mechanisms (68). The increase in Hct and Hb after AIEB is attributable to the release of the splenic reserve of red blood cells, mediated by the action of catecholamines in response to intense exercise (6), despite the changes observed after exercise. The results showed no differences in recovery strategies for blood gases and electrolytes, indicating that the recovery methods used did not affect the body's compensatory response to moderate metabolic acidosis caused by exercise.
Some studies have indicated that WBV can control glycaemia in humans (69, 70) and rodents (11, 71). The current study did not indicate that WBV could favor such an effect. The only thing that influenced plasma glucose was AIEB, which increased the bioavailability of this energy substrate for skeletal muscles. This finding can be explained by the increased activity of hormones that regulate energy metabolism, such as catecholamines and glucagon. These, when released, promote hepatic glycogenolysis and neoglycogenesis, an essential mechanism for maintaining plasma glucose concentrations during exercise (66).
After the recovery methods, the WBVG and SG showed lower HR compared to the TG, which can be explained by the fact that WBV is considered a form of passive recovery. However, there was no difference between the WBVG and SG, indicating that the VP turned off had the same effect as the equipment turned on. In adult men subjected to maximal exercise, a more pronounced reduction in HR was observed when recovering with an association of light exercise and WBV, compared to light exercise alone (4) and recovery with squats in VP on, compared to passive rest or only squats (5). These findings indicate that WBV led to a quicker heart rate recovery, a result not seen with the protocol used in the current study.
In the present study, it was possible to observe an increase in rectal temperature after AIEB. This variable increased even more after all interventions, around 15 min after the end of AIEB, in all groups. The increase in rectal temperature after exercise is significantly correlated with exercise (37). Corroborating these results, a study that evaluated different types of cooling with water after medium-intensity exercise observed an increase in rectal temperature, which remained elevated for up to 30 min after exercise, and the use of cooling with water as a form of recovery did not influence the decrease in post-exercise rectal temperature (31). Therefore, WBV did not accelerate the cooling down of this cohort of horses.
The use of mechanical vibration as an alternative to active exercise is of increasing interest. The effect of WBV on vital parameters, muscles and bones is investigated and it is widely suggested that WBV may be an alternative to resistance training for stimulation of the musculoskeletal system (43), which could make the technique an important training option, aiming to reduce the time of animals on the track, especially those with previous injuries and which would have a greater chance of recurrence. With the current search for alternatives to improve athletic capacity in the horse industry, it is not uncommon for participants to be mesmerized by the miraculous effects of therapies on the market. Companies that produce vibrating stimulation plates claim results such as increase/maintenance of muscle mass, reduction of injuries, faster healing, improved balance, and increased circulation, among others. The effectiveness of WBV as a training method, however, is quite controversial, and the results presented here did not indicate any effect on the physiological variables analyzed. In a study that investigated the effects of warming up with WBV in horses, the results showed that WBV can be seen as a passive movement of the limbs and trunk, as a type of relaxation of the locomotor system, without any active involvement (26).
More studies are needed in horses, especially regarding different vibration patterns, as the transmission of vibration through the equine body is poorly understood and is a fundamental consideration in interpreting the effects of WBV. A reduction in amplitude observed in the dorsal compared to the extremities of horses' bodies denotes that vibration transmission is greatly attenuated and may not be effective in provoking a physiological response, such as increased lactate removal from muscles, in the upper part of the body (72). To date, the use of WBV in horses is primarily based on results reported in human or rodent literature. However, horses are the largest animals on which WBV has been tested, and this size difference may impact vibration transmission. Furthermore, a possibly significant difference between WBV in men and horses is body posture during vibration training (24, 26). Moreover, most studies on WBV in humans involve performing other exercises on the VP (4, 5, 8), which, to date, is not possible to be carried out in the equine species.
While the study provides valuable insights, it is important to acknowledge that it has some limitations. For instance, there were a small number of horses involved, although this was enough for the statistical analysis. Additionally, using a single WBV session in the protocol may not have been enough to induce significant changes. It is possible that different results could be observed with longer periods of training using WBV. Also, the way vibrational transmission occurs throughout the horse's body is poorly understood; therefore, electromyography would be necessary to estimate how muscle activation occurs using WBV. Furthermore, although the gluteus medius muscle is the largest muscle in the horse and of great importance in its locomotion, showing great propulsive activity in the gaits during the exercise (73–75), it may be beneficial to assess other muscles in the distal thoracic or pelvic limbs, such as the triceps or semitendinosus, as the effects of vibration may diminish when moving dorsally (72). Hence, it is important to study different protocols for sport horse training to better evaluate the effects of WBV as a recovery method after intense exercise.
The research demonstrated that a single session of whole-body vibration (WBV) does not improve recovery from hyperlactatemia or acid-base balance in horses after intense exercise. This insight offers veterinarians, researchers and riding instructors the opportunity to develop better recovery protocols for horses. Future studies should focus on other protocols and the potential benefits of chronic WBV applications for enhancing recovery after exercise in horses.
The raw data supporting the conclusions of this article will be made available by the authors, without undue reservation.
The animal study was approved by Ethics Committee on the Use of Animals. The study was conducted in accordance with the local legislation and institutional requirements.
JC: Conceptualization, Data curation, Formal analysis, Investigation, Methodology, Software, Writing – original draft, Writing – review & editing. NS: Formal analysis, Investigation, Methodology, Software, Writing – review & editing. TL: Formal analysis, Investigation, Methodology, Software, Writing – review & editing. GC: Formal analysis, Investigation, Methodology, Software, Writing – review & editing. CC: Formal analysis, Investigation, Software, Writing – review & editing. EP: Data curation, Software, Writing – review & editing. JO: Data curation, Software, Writing – review & editing. GR: Data curation, Software, Writing – original draft, Writing – review & editing. IS: Data curation, Formal analysis, Writing – review & editing, Software. CG: Resources, Supervision, Writing – review & editing. FM-G: Resources, Supervision, Writing – review & editing. GF: Conceptualization, Formal analysis, Funding acquisition, Investigation, Methodology, Project administration, Resources, Supervision, Writing – original draft, Writing – review & editing.
The author(s) declare financial support was received for the research, authorship, and/or publication of this article. This research was supported by grants from the São Paulo Research Foundation (FAPESP, Process No. 2019/16779-3 and No. 2020/01172-3). Additionally, this study was financed in part by the Brazilian Federal Agency for Support and Evaluation of Graduate Education (CAPES)-Finance Code 001.
We thank the School of Agricultural and Veterinary Sciences, São Paulo State University (FCAV/UNESP), for the academic support. We also thank the Postgraduate Course Program in Veterinary Medicine (FCAV/UNESP), the Postgraduate Course Program in Animal Science (FCAV/UNESP), and the Department of Animal Morphology and Physiology (FCAV/UNESP) for their academic support. We thank the veterinarian Camila Diniz Junqueira for her support in developing the research. We thank the team of the Laboratory of Applied Sport Physiology, School of Applied Sciences, University of Campinas (FCA/UNICAMP).
The authors declare that the research was conducted in the absence of any commercial or financial relationships that could be construed as a potential conflict of interest.
The author(s) declare that no Gen AI was used in the creation of this manuscript.
All claims expressed in this article are solely those of the authors and do not necessarily represent those of their affiliated organizations, or those of the publisher, the editors and the reviewers. Any product that may be evaluated in this article, or claim that may be made by its manufacturer, is not guaranteed or endorsed by the publisher.
The Supplementary Material for this article can be found online at: https://www.frontiersin.org/articles/10.3389/fvets.2025.1538195/full#supplementary-material
VP, vibrating platform; WBV, whole-body vibration; MCT1, monocarboxylate transporter 1; MCT4, monocarboxylate transporter 4; AIEB, acute intense exercise bout; TG, treadmill group; WBVG, whole-body vibration group; SG, sham group; HR, heart rate; RT, rectal temperature; SID, strong ion difference; CEUA, Ethics Committee on the Use of Animals; VLT, velocity corresponding to the lactate threshold; SET, standardized exercise test; VLTV, velocity corresponding to the visual lactate threshold; VLTBI, velocity corresponding to the bi-segmented lactate threshold; A, after acute intense exercise bout; BR, before recovery; AR, after recovery; EDTA, Ethylenediaminetetraacetic Acid; PVCO2, pressure of carbon dioxide; tCO2, total carbon dioxide; BEecf, base excess/deficit; AnGap, anion gap; , bicarbonate; Na+, sodium ion; K+, potassium ion; Cl−, chloride ion; Hb, total hemoglobin; Hct, hematocrit; Ure, urea nitrogen; bpm, beats per minute; AUC, area under the curve.
1. Ritzmann R, Kramer A, Bernhardt S, Gollhofer A. Whole body vibration training—improving balance control and muscle endurance. PLoS ONE. (2014) 9:e89905. doi: 10.1371/journal.pone.0089905
2. Kang SR, Min JY, Yu C, Kwon TK. Effect of whole-body vibration on lactate level recovery and heart rate recovery in rest after intense exercise. Technol Health Care. (2017) 25(Suppl. 1):115–23. doi: 10.3233/THC-171313
3. Chang SF, Lin PC, Yang RS, Yang RJ. The preliminary effect of whole-body vibration intervention on improving the skeletal muscle mass index, physical fitness, and quality of life among older people with sarcopenia. BMC Geriatr. (2018) 18:17. doi: 10.1186/s12877-018-0712-8
4. Oh JH, Kwon TK, Hong CU, Lee YC. Effect of short-term sling exercise with whole body vibration recovery method on heart rate, blood pressure and lactic acid level variability. J Mech Med Biol. (2018) 18:1840016. doi: 10.1142/S021951941840016X
5. Campos DG, Arancibia JO, Urra PS, Cadenas ES, Lozano AS, Rodríguez FR, et al. Effect of high-intensity whole body vibration on blood lactate removal and heart rate after and all-out test in active young men. Retos. (2021) 39:471–6. doi: 10.47197/retos.v0i39.78441
6. Lai Z, Lee S, Chen Y, Wang L. Comparison of whole-body vibration training and quadriceps strength training on physical function and neuromuscular function of individuals with knee osteoarthritis: a randomised clinical trial. J Exerc Sci Fit. (2021) 19:150–7. doi: 10.1016/j.jesf.2021.01.003
7. Tseng SY, Lai CL, Ko CP, Chang YK, Fan HC, Wang CH. The effectiveness of whole-body vibration and heat therapy on the muscle strength, flexibility, and balance abilities of elderly groups. Int J Environ Res Public Health. (2023) 20:1650. doi: 10.3390/ijerph20021650
8. Colson SS, Gioda J, Silva F. Whole body vibration training improves maximal strength of the knee extensors, time-to-exhaustion and attenuates neuromuscular fatigue. Sports. (2023) 11:94. doi: 10.3390/sports11050094
9. Kale M, Tolali AB, Togram T, Basoglu UD. Acute effects of whole-body vibration during dynamic lunge movement on jump and sprint performances. Pedagogy Phys Cult Sports. (2024) 28:309–19. doi: 10.15561/26649837.2024.0408
10. Ewertowska P, Zapadka BZ, Głażewska M, Poniatowski L, Tuptanowski K, Ossowski Z, et al. Influence of whole-body vibration and drop jump on the range of motion in the ankle joint and running parameters—a randomized crossover study. Physiother Res Int. (2024) 29:e2132. doi: 10.1002/pri.2132
11. Huang CC, Tseng TL, Huang WC, Chung YH, Chuang HL, Wu JH. Whole-body vibration training effect on physical performance and obesity in mice. Int J Med Sci. (2014) 11:1218–27. doi: 10.7150/ijms.9975
12. Lin CI, Huang WC, Chen WC, Kan NW, Wei L, Chiu YS, et al. Effect of whole-body vibration training on body composition, exercise performance and biochemical responses in middle-aged mice. Metabolism. (2015) 64:1146–56. doi: 10.1016/j.metabol.2015.05.007
13. McGee-Lawrence ME, Wenger KH, Misra S, Davis CL, Pollock NK, Elsalanty M, et al. Whole-body vibration mimics the metabolic effects of exercise in male leptin receptor-deficient mice. Endocrinology. (2017) 158:1160–71. doi: 10.1210/en.2016-1250
14. Chen YM, Lee HC, Chen MT, Huang CC, Chen WC. Dehydroepiandrosterone supplementation combined with weight-loading whole-body vibration training (WWBV) affects exercise performance and muscle glycogen storage in middle-aged C57BL/6 mice. Int J Med Sci. (2018) 15:564–73. doi: 10.7150/ijms.23352
15. Higaki S, Koga Y, Inai R, Matsuo T. Long-term whole-body vibration stimulus decreases body fat accumulation in rats fed high-fat diet. J Oleo Sci. (2023) 72:839–47. doi: 10.5650/jos.ess23076
16. Musumeci G. The use of vibration as physical exercise and therapy. J Funct Morphol Kinesiol. (2017) 2:17. doi: 10.3390/jfmk2020017
17. Bonanni R, Cariati I, Romagnoli C, D'Arcangelo G, Annino G, Tancredi V. Whole body vibration: a valid alternative strategy to exercise? J Funct Morphol Kinesiol. (2022) 7:99. doi: 10.3390/jfmk7040099
18. Caprioli L, Campoli F, Edriss S, Padua E, Romagnoli C, Bonaiuto V, et al. Effect of whole-body vibration on sports performance: a literature review. In: Montanari R, Richetta M, Febbi M, Staderini EM, editors. Engineering Methodologies for Medicine and Sports. Cham: Springer (2024). p. 642–62.
19. Pollock RD, Woledge RC, Mills KR, Martin FC, Newham DJ. Muscle activity and acceleration during whole body vibration: effect of frequency and amplitude. Clin Biomech. (2010) 25:840–6. doi: 10.1016/j.clinbiomech.2010.05.004
20. Franchignoni F, Vercelli S, Özçakar L. Hematuria in a runner after treatment with whole body vibration: a case report. Scand J Med Sci Sports. (2013) 23:383–5. doi: 10.1111/j.1600-0838.2012.01478.x
21. Liao LR, Ng GYF, Jones AYM, Huang MZ, Pang MYC. Whole-body vibration intensities in chronic stroke: a randomized controlled trial. Med Sci Sports Exerc. (2016) 48:1227–38. doi: 10.1249/MSS.0000000000000909
22. Lam FMH, Liao LR, Kwok TCY, Pang MYC. Effects of adding whole-body vibration to routine day activity program on physical functioning in elderly with mild or moderate dementia: a randomized controlled trial. Int J Geriatr Psychiatry. (2017) 33:21–30. doi: 10.1002/gps.4662
23. McCann MR, Veras MA, Yeung C, Lalli G, Patel P, Leitch KM, et al. Whole-body vibration of mice induces progressive degeneration of intervertebral discs associated with increased expression of Il-1β and multiple matrix degrading enzymes. Osteoarth Cartil. (2017) 25:779–89. doi: 10.1016/j.joca.2017.01.004
24. Halsberghe BT. Long-term and immediate effects of whole body vibration on chronic lameness in the horse: a pilot study. J Equine Vet Sci. (2017) 48:121–8. doi: 10.1016/j.jevs.2015.12.007
25. Ellis KL, Morris C, Harbold AF, Yokeley ME, Franklin LE, Phelps LM, et al. The effect of whole-body vibration therapy on mechanical nociceptive thresholds and postural stability in horses with thoracolumbar pain. J Equine Rehabil. (2024) 2:100015. doi: 10.1016/j.eqre.2024.100015
26. Buchner HHF, Zimmer L, Haase L, Perrier J, Peham C. Effects of whole body vibration on the horse: actual vibration, muscle activity, and warm-up effect. J Equine Vet Sci. (2017) 51:54–60. doi: 10.1016/j.jevs.2016.12.005
27. Marlin DJ, Scott CM, Roberts CA, Casas I, Holah G, Schroter RC. Post exercise changes in compartmental body temperature accompanying intermittent cold water cooling in the hyperthermic horse. Equine Vet J. (1998) 30:28–34. doi: 10.1111/j.2042-3306.1998.tb04085.x
28. Kang OD, Ryu YC, Yun YM, Kang MS. Effects of cooldown methods and durations on equine physiological traits following high-intensity exercise. Livest Sci. (2012) 143:70–6. doi: 10.1016/j.livsci.2011.08.016
29. Takahashi Y, Ohmura H, Mukai K, Shiose T, Takahashi T. A comparison of five cooling methods in hot and humid environments in Thoroughbred horses. J Equine Vet Sci. (2020) 91:103130. doi: 10.1016/j.jevs.2020.103130
30. Farinelli F, Rezende ASC, Fonseca MG, Lana AMQ, Leme FOP, Klein BOM, et al. Influence of stretching exercises, warm-up, or cool-down on the physical performance of Mangalarga Marchador horses. J Equine Vet Sci. (2021) 106:103714. doi: 10.1016/j.jevs.2021.103714
31. Janczarek I, Wiśniewska A, Tkaczyk E, Wnuk-Pawlak E, Kaczmarek B, Liss-Szczepanek M, et al. Effect of different water-cooling treatments on changes in rectal and surface body temperature in leisure horses after medium-intensity effort. Animals. (2022) 12:525. doi: 10.3390/ani12040525
32. Kang H, Zsoldos RR, Skinner JE, Gaughan JB, Mellor VA, Sole-Guitart A. The use of percutaneous thermal sensing microchips to measure body temperature in horses during and after exercise using three different cool-down methods. Animals. (2022) 12:1267. doi: 10.3390/ani12101267
33. Lisboa BRF, Silva JAR, Silva WC, Barbosa AVC, Silva LKX, Lourenço-Júnior JB. Evaluation of thermoregulation of horses (Equus caballus) submitted to two methods of post-exercise cooling, in hot and humid climate conditions, in the Eastern Amazon. Front Vet Sci. (2023) 10:1150763. doi: 10.3389/fvets.2023.1150763
34. Theraplate (2024). Available online at: https://theraplate.com/ (accessed October 16, 2024).
35. Lamprecht ED, Williams CA. Biomarkers of antioxidant status, inflammation, and cartilage metabolism are affected by acute intense exercise but not superoxide dismutase supplementation in horses. Oxid Med Cell Longev. (2012) 2012:920932. doi: 10.1155/2012/920932
36. Taylor LE, Kronfeld DS, Ferrante PL, Wilson JA, Tiegs W. Blood-gas measurements adjusted for temperature at three sites during incremental exercise in the horse. J Appl Physiol. (1998) 85:1030–6. doi: 10.1152/jappl.1998.85.3.1030
37. Lindinger MI, Waller AP. Physicochemical analysis of mixed venous and arterial blood acid-base state in horses at core temperature during and after moderate-intensity exercise. Animals. (2022) 12:1875. doi: 10.3390/ani12151875
38. Kitaoka Y, Endo Y, Mukai K, Aida H, Hiraga A, Takemasa T, et al. Effect of acute exercise on monocarboxylate transporters 1 and 4 in untrained and trained Thoroughbreds. Am J Vet Res. (2013) 74:642–7. doi: 10.2460/ajvr.74.4.642
39. Orsi JB, Araujo LS, Scariot PPM, Polisel EEC, Cardoso LO, Gobatto CA, et al. Critical velocity, maximal lactate steady state, and muscle MCT1 and MCT4 after exhaustive running in mice. Int J Mol Sci. (2023) 24:15753. doi: 10.3390/ijms242115753
40. Armstrong RA. When to use the Bonferroni correction. OPO. (2014) 34:502–8. doi: 10.1111/opo.12131
41. Purves RD. Optimum numerical integration methods for estimation of area-under-the-curve (AUC) and area-under-themoment-curve (AUMC). J Pharmacokinet Biopharm. (1992) 20:211–26. doi: 10.1007/BF01062525
43. Carstanjen B, Balali M, Gajewski Z, Furmanczky K, Bondzio A, Remy B, et al. Short-term whole body vibration exercise in adult health horses. Pol J Vet Sci. (2013) 16:403–5. doi: 10.2478/pjvs-2013-0057
44. Nowlin C, Nielsen B, Mills J, Robison C, Schott H, Peters D. Acute and prolonged effects of vibrating plataform treatment on horses: a pilot study. J Equine Vet Sci. (2018) 62:116–22. doi: 10.1016/j.jevs.2017.12.009
45. Maher K, Spooner H, Hoffman R, Haffner J. The influence of whole-body vibration on heart rate, stride length, and bone mineral content in the mature exercising horse. Comp Exerc Physiol. (2020) 16:403–8. doi: 10.3920/CEP190073
46. Halsberghe BT, Gordon-Ross P, Peterson R. Whole body vibration affects the cross-sectional area and symmetry of the M. multifidus of the thoracolumbar spine in the horse. Equine Vet Educ. (2017) 29:493–9. doi: 10.1111/eve.12630
47. Halsberghe BT. Effect of two months whole body vibration on hoof growth rate in the horse: a pilot study. Res Vet Sci. (2018) 119:37–42. doi: 10.1016/j.rvsc.2018.05.010
48. Brooks GA. Lactate as a fulcrum of metabolism. Redox Biol. (2020) 35:101454. doi: 10.1016/j.redox.2020.101454
49. Xie H, Mao X, Wang Z. Effect of high-intensity interval training and moderate-intensity continuous training on blood lactate clearance after high-intensity test in adult men. Front Physiol. (2024) 15:1451464. doi: 10.3389/fphys.2024.1451464
50. Watanabe T, Kondo S, Kakinoki K, Fukusaki C, Hatta H. Stride-to-stride variability and fluctuations at intensities around lactate threshold in distance runners. Heliyon. (2023) 9:e17437. doi: 10.1016/j.heliyon.2023.e17437
51. Gomes MVF, Santos IFC, Rahal SC, Silva BM. Applicability of whole-body vibration exercises as a new tool in veterinary medicine. Revista Hospital Universitário Pedro Ernesto. (2018) 17:30–4. doi: 10.12957/rhupe.2018.39273
52. Rauch F, Sievanen H, Boonen S, Cardinale M, Degens H, Felsenberg D, et al. Reporting whole-body vibration intervention studies: recommendations of the International Society of Musculoskeletal and Neuronal Interactions. J Musculoskelet Neuronal Interact. (2010) 10:193–8.
53. Sales MM, Sousa CV, Aguiar SS, Knechtle B, Nikolaidis PT, Alves PM, et al. An integrative perspective of the anaerobic threshold. Physiol Behav. (2019) 205:29–32. doi: 10.1016/j.physbeh.2017.12.015
54. Koho NM, Hyyppä S, Pösö AR. Monocarboxylate transporters (MCT) as lactate carriers in equine muscle and red blood cells. Equine Vet J. (2006) 38(Suppl. 36):354–8. doi: 10.1111/j.2042-3306.2006.tb05568.x
55. Kitaoka Y, Hoshino D, Hatta H. Monocarboxylate transporter and lactate metabolism. J Phys Fitness Sports Med. (2012) 1:247–52. doi: 10.7600/jpfsm.1.247
56. Kitaoka Y, Masuda H, Mukai K, Hiraga A, Takemasa T, Hatta H. Effect of training and detraining on monocarboxylate transporter (MCT) 1 and MCT4 in Thoroughbred horses. Exp Physiol. (2011) 96:348–55. doi: 10.1113/expphysiol.2010.055483
57. Coles L, Litt J, Hatta H, Bonen A. Exercise rapidly increases expression of the monocarboxylate transporters MCT1 and MCT4 in rat muscle. J Physiol. (2004) 561:253–61. doi: 10.1113/jphysiol.2004.073478
58. Araujo GG, Gobatto CA, Manchado-Gobatto FB, Teixeira LFM, Reis IGM, Caperuto LC, et al. MCT1 and MCT4 kinetic of mRNA expression in different tissues after aerobic exercise at maximal lactate steady state workload. Physiol Res. (2015) 64:513–22. doi: 10.33549/physiolres.932695
59. Green H, Halestrap A, Mockett C, Otoole S, Grant S, Ouyang J. Increases in muscle MCT are associated with reductions in muscle lactate after a single exercise session in humans. Am J Physiol Endocrinol Metab. (2002) 282:154–60. doi: 10.1152/ajpendo.2002.282.1.E154
60. Bishop D, Edge J, Thomas C, Mercler J. High-intensity exercise acutely decreases the membrane content of MCT1 and MCT4 and buffer capacity in human skeletal muscle. J Appl Physiol. (2007) 102:616–21. doi: 10.1152/japplphysiol.00590.2006
61. Seyedi R, Tayebi SM, Zhang D, Yiming Q. The role of monocarboxylate transporter-1 and−4 in exercise and training: a mini-review article. Sci Sport. (2023) 39:144–52. doi: 10.1016/j.scispo.2022.11.009
62. Regatieri IC, Curi RA, Ferraz GC, Queiroz-Neto A. Candidate genes for performance in horses, including monocarboxylate transporters. Pesq Vet Bras. (2017) 37:66–72. doi: 10.1590/s0100-736x2017000100011
63. Feringer-Júnior WH, Carvalho JRG, Almeida MLM, Lemos EGM, Soares OAB, Ribeiro G, et al. Differential expression of monocarboxylate transporter 1 and ancillary protein CD147 in red blood cells of show jumping horses. J Equine Vet Sci. (2019) 81:102791. doi: 10.1016/j.jevs.2019.102791
64. Vengust M, Stämpfli H, Moraes AN, Teixeiro-Neto F, Viel L, Heigenhauser G. Effects of chronic acetazolamide administration on gas exchange and acid-base control in pulmonary circulation in exercising horses. Equine Vet J. (2010) 42(Suppl. 38):40–50. doi: 10.1111/j.2042-3306.2010.00240.x
65. Waller A, Lindinger MI. Physicochemical analysis of acid-base status during recovery from high-intensity exercise in Standardbred racehorses. Equine Comp Exerc Physiol. (2005) 2:119–27. doi: 10.1079/ECP200549
66. Ferraz GC, Soares OAB, Foz NSB, Pereira MC, Queiroz-Neto A. The workload and plasma ion concentration in a training match session of high-goal (elite) polo ponies. Equine Vet J. (2010) 42(Suppl. 38):191–5. doi: 10.1111/j.2042-3306.2010.00278.x
67. Miranda ACT, Padilha FGF, Ramos MT, Dimache LAG, Godoi FN, Galina-Filho A, et al. Hemogasometry of eventing horses before and after intense exercise on a high speed treadmill. Livest Sci. (2016) 186:85–7. doi: 10.1016/j.livsci.2015.07.020
68. Beaver WL, Wasserman K, Whipp BJ. Bicarbonate buffering of lactic acid generated during exercise. J Appl Physiol. (1986) 60:472–8. doi: 10.1152/jappl.1986.60.2.472
69. Pessoa MF, Souza HCM, Silva APV, Clemente RS, Brandão DC, Andrade AD. Acute whole body vibration decreases the glucose levels in elderly diabetic women. Rehabil Res Pract. (2018) 2018:3820615. doi: 10.1155/2018/3820615
70. Kim M, Zhang H, Kim T, Mori Y, Okura T, Tanaka K, et al. Novel approach for glycemic management incorporating vibration stimulation of skeletal muscle in obesity. Int J Environ Res Public Health. (2023) 20:4708. doi: 10.3390/ijerph20064708
71. An S, Wang D, Ma X, Liu C. Whole body vibration remodels skeletal muscle via autophagy and energy metabolism in diabetic mice. Mol Med Rep. (2022) 25:182. doi: 10.3892/mmr.2022.12698
72. Read E, Huseman CJ, Tarazaga PA, Soroor AO, Zoller J. Quantification of frequency, amplitude, and transmission from equine whole body vibration platform. J Equine Vet Sci. (2023) 124:127. doi: 10.1016/j.jevs.2023.104429
73. Essén-Gustavsson B, McMiken D, Karlström K, Lindholm A, Persson S, Thornton J. Muscular adaptation of horses during intensive training and detraining. EVJ. (1989) 21:27–33. doi: 10.1111/j.2042-3306.1989.tb02085.x
74. López-Rivero JL, Agüera E, Monterde JG, Diz A, Vivo J. Fibre size and composition in the middle gluteal muscle of the Andalusian horse. EVJ. (1990) 22:286–7. doi: 10.1111/j.2042-3306.1990.tb04269.x
Keywords: acid-base balance, cool-down, exercise, heart rate, lactate, MCT1, MCT4, whole-body vibration
Citation: Carvalho JRG, Sales NAA, Littiere TO, Costa GB, Castro CM, Polisel EEC, Orsi JB, Ramos GV, Santos IFC, Gobatto CA, Manchado-Gobatto FB and Ferraz GC (2025) Acute whole-body vibration as a recovery strategy did not alter the content of gluteus medius monocarboxylate-transporters, lactatemia, and acidosis induced by intense exercise in horses. Front. Vet. Sci. 12:1538195. doi: 10.3389/fvets.2025.1538195
Received: 02 December 2024; Accepted: 13 February 2025;
Published: 06 March 2025.
Edited by:
Emanuela Dalla Costa, University of Milan, ItalyReviewed by:
Silvia Michela Mazzola, University of Milan, ItalyCopyright © 2025 Carvalho, Sales, Littiere, Costa, Castro, Polisel, Orsi, Ramos, Santos, Gobatto, Manchado-Gobatto and Ferraz. This is an open-access article distributed under the terms of the Creative Commons Attribution License (CC BY). The use, distribution or reproduction in other forums is permitted, provided the original author(s) and the copyright owner(s) are credited and that the original publication in this journal is cited, in accordance with accepted academic practice. No use, distribution or reproduction is permitted which does not comply with these terms.
*Correspondence: Júlia Ribeiro Garcia Carvalho, anVsaWFyZ2NAaG90bWFpbC5jb20=
Disclaimer: All claims expressed in this article are solely those of the authors and do not necessarily represent those of their affiliated organizations, or those of the publisher, the editors and the reviewers. Any product that may be evaluated in this article or claim that may be made by its manufacturer is not guaranteed or endorsed by the publisher.
Research integrity at Frontiers
Learn more about the work of our research integrity team to safeguard the quality of each article we publish.