Corrigendum: Dihydromyricetin improves growth performance, immunity, and intestinal functions in weaned pigs challenged by enterotoxigenic Escherichia coli
- 1Institute of Animal Nutrition, Sichuan Agricultural University, Chengdu, China
- 2Key Laboratory for Animal Disease-Resistance Nutrition of China Ministry of Education, Chengdu, China
- 3College of Veterinary Medicine, Sichuan Agricultural University, Chengdu, China
Enteric infection is a major cause of enteric disorder in neonatal pigs during the weaning transition. Dihydromyricetin (DMY) is a natural flavanonol compound extracted from Ampelopsis grossedentata with numerous biological activities such as antioxidative and immunomodulatory functions. The objective of this study was to investigate the effects of dietary dihydromyricetin supplementation on growth performance, immunity, and intestinal functions in weaned pigs challenged by enterotoxigenic Escherichia coli (ETEC). In total, 24 weaned DLY (Duroc × Landrace × Yorkshire) pigs were allotted to 3 treatments. Pigs fed with basal diet or basal diet containing 300 mg/kg DMY were orally infused with sterilized culture or ETEC (2.5 × 1011 colony-forming units). Dietary DMY supplementation significantly elevated the final weight and average daily gain (ADG) but reduced diarrhea incidence in the weaned pigs of the EDMY group compared to the pigs of the ECON group (p < 0.05). Compared to the ECON group, DMY also improved the digestibility of dry matter (DM), ether extract (EE), gross energy (GE), and ash of the EDMY group (p < 0.05). Moreover, DMY not only significantly decreased the ratio of albumin/globulin but also elevated serum concentrations of immunoglobulins (e.g., IgA and IgG) in the weaned pigs of the EDMY group compared to the pigs of the ECON group (p < 0.05). Interestingly, the villus height, the ratio of villus height to crypt depth (V:C), and the activities of mucosal alkaline phosphatase, sucrase, and maltase in the duodenum and jejunum of the EDMY group were higher than those in the ECON group (p < 0.05). Importantly, DMY significantly elevated the expression levels of jejunal zonula occludens-1 (ZO-1), claudin-1, cationic amino acid transporter-1 (CAT-1), and fatty acid transport protein-1 (FATP-1) in the weaned pigs of the EDMY group compared to the pigs of the ECON group (p < 0.05). Additionally, compared to the ECON group, DMY increased the concentrations of microbial SCFA metabolites (e.g., acetic acid and propanoic acid), but reduced the abundance of Escherichia coli in the cecum of the EDMY group (p < 0.05). Dietary DMY supplementation can attenuate the ETEC–induced growth retardation and intestinal injury, which was attributed to the amelioration of intestinal nutrient digestion and transport functions as well as the improved microbiota.
1 Introduction
The weaned pigs lack effective resistance to diseases and stress due to the deprivation of maternal passive immunity and incomplete gastrointestinal (GI) tract development (1). Previous studies indicated that pathogenic bacteria can induce diarrhea, growth retardation, and even considerable mortality in piglets, especially in the first week after weaning, which causes huge economic losses in pig farming (2, 3). Enterotoxigenic Escherichia coli (ETEC), as the most common and typical infectious pathogen of postweaning diarrhea (PWD) for piglets, is characterized by various adhesions [e.g., F4 (K88+), F5 (K99+), F18, and F41 fimbriae], which could promote malignant colonization on the intestinal mucosa via specific fimbriae receptors (4). During ETEC-induced diarrhea, the intestine is subjected to enormous stress, accompanied by remarkable changes in microbiota homeostasis, epithelial brush border enzymatic activities, intestinal permeability, and intestinal morphology, such as villous atrophy and crypt hyperplasia (5, 6). In past decades, antibiotics have been widely used as therapeutic drugs for ETEC infection to prevent diarrhea and intestinal injury in pig production. Nevertheless, the abuse of antibiotics can lead to bacterial resistance and drug residues in animal products, eventually threatening human safety (7). Therefore, alternatives to antibiotics that are safe for humans, animals, and the environment are urgently needed. Currently, some plant-derived compounds are reported to improve intestinal function, barrier, and microbiota dysbiosis, deeming they are a good choice for antibiotic substitutes (8–10).
Dihydromyricetin (DMY, 2R,3R-3,5,7,3′,4′,5′-hexahydroxy-2,3-dihydroflavonol), as a natural flavonoid compound mainly extracted from Ampelopsis grossedentata (A. grossedentata), has received considerable attention for its several biological properties such as anti-inflammatory (11), immune-enhancing (12), and intestinal microbiota-regulating biological activities (13). Recently, DMY has also been found to inhibit the replication of some viruses such as the African swine fever virus in vitro (14). These advantages suggest a promising protective role of DMY in preventing intestinal injury in animal production. For growing-finishing pigs, dietary supplementation of DMY can effectively ameliorate the intestinal barrier by increasing the immune compounds such as immunoglobulin M (IgM) and IgA contents (12). In addition to this, a previous study has shown that DMY significantly depressed the inflammatory response and restored the intestinal redox status, thus attenuating ileum injury in chickens that suffer E. coli lipopolysaccharide infection (15). Intriguingly, it has been reported that the water extract of A. grossedentata can regulate gut microbiota, promoting the growth of beneficial bacteria (e.g., Lactobacillus) and inhibiting harmful microbes (e.g., norank_f_Muribaculaceae) (16). However, to the best of our knowledge, little is known regarding the action of DMY on growth performance and intestinal epithelial functions in weaned pigs infected with ETEC. Hence, this study aimed to assess the effectiveness of dietary DMY supplementation on intestinal inflammation and epithelium injury in weaned pigs induced by ETEC, and the potential mechanisms of action were also investigated.
2 Materials and methods
2.1 Animal experimental design
All animal procedures were approved by the Committee on Animal Care Advisory of Sichuan Agricultural University (authorization number SICAU-2022-014). All experiment procedures were carried out in accordance with the guidelines for the Care and Use of Laboratory Animals. In total, 24 healthy barrows weaned on their 21st day and with an average body weight of 7.84 ± 0.08 kg were individually housed in a metabolism cage (0.7 m × 1.5 m × 1.5 m) and allowed to acclimatize to the study condition for 3 days. All pigs were randomly allocated to three groups (with eight replicates in each group and one pig in each replicate). Pigs in the CON and ECON groups were fed with a basal diet, and those in the EDMY group were fed the basal diet added with 300 mg kg−1 DMY (Guangzhou Nuacid Co., Ltd., Guangzhou, China) for 21 days. On day 19 (i.e., after the adaptation period), pigs in the ECON and EDMY groups were orally administered 100 mL of LB culture containing approximately 2.5 × 109 cfu mL−1 E. coli K88 (hereafter referred to as ETEC; serotype O149: K91: K88ac), while pigs in the CON group were administered equivalent amount of sterile LB medium. The basal diet (Table 1) was formulated to meet the swine nutrient requirements recommended by the National Research Council (NRC, 2012). Fresh feed and water were provided ad libitum to pigs throughout the experimental period. The ambient temperature of 26°C ± 1.5°C and relative humidities of 65% ± 5% were maintained. The ETEC F4+ (serotype O149:K91, K88ac) used in the challenge study was purchased from China Veterinary Culture Collection Center.
2.2 Sample collection
From days 19 to 21 of the experiment, the fresh fecal samples were collected immediately after excretion from pigs in each group. After collection, the daily excreta of each pig were weighed, and 10 mL of a 10% H2SO4 solution was added to each 100 g of wet fecal sample, and subsequently stored in a sealed plastic bag at −20°C. At the end of the experiment, all fecal samples of each pig were thawed at room temperature, mixed thoroughly, and then dried at 70°C for 48 h. After being crushed on a 1-mm screen, these samples were stored at −20°C for chemical analyses including dry matter (DM), crude protein (CP), ether extract (EE), ash, and gross energy (GE).
At 8:00 a.m. on day 21, blood samples were collected from the jugular vein of overnight-fasted piglets into non-heparinized vacuum tubes. The serum samples were prepared by centrifuging blood samples at 3,500 × g at 4°C for 20 min and then stored in a −20°C refrigerator until analysis. Subsequently, the pigs were euthanized with an intravenous injection of sodium pentobarbital (200 mg/kg BW) and followed by an exsanguination protocol. After euthanization, the duodenum, jejunum, and ileum samples of each pig were separated from the exposed abdomen and washed with ice-cold PBS. The mid segments of the duodenum, jejunum, and ileum, approximately 3 cm in length, were harvested and fixed in 4% paraformaldehyde solution for intestinal morphology. Other duodenum, jejunum, and ileum (approximately 4 cm in length) tissue samples were collected, snap-frozen, and stored at −80°C for real-time polymerase chain reaction (RT-PCR) analyses. The remaining segments of the duodenum, jejunum, and ileum were opened longitudinally, washed with ice-cold PBS, and gently scraped with a sterile glass microscope slide at 4°C to obtain mucosa samples. The mucosa samples were immediately snap-frozen in liquid nitrogen and stored at −80°C until further analysis of related enzyme activities. In addition, digesta of the colon and cecum was immediately placed in liquid nitrogen and stored at −80°C for analysis of microbial DNA and short-chain fatty acid (SCFA) concentration analyses.
2.3 Measurement of growth performance
Each pig’s body weight (BW) was monitored on days 1, 19, and 21 after 12-h fasting in the morning at 8:00, and feed intake and waste feed were collected and weighed daily. The average daily gain (ADG), average daily feed intake (ADFI), and the feed: gain ratio (F/G) were then calculated.
2.4 Determination of diarrhea rate
During the challenge period, the feces of the pigs were observed daily, and the diarrhea rate was calculated according to the formula, diarrhea rate (%) = (number of pigs with diarrhea within a treatment × total observational days) / (number of pigs × total observational days) × 100%. The incidence of diarrhea was defined in accordance with the following standards: fecal score of 0 (normal); fecal score of 1 (normal feces); fecal score of 2 (moist feces), fecal score of 3 (mild diarrhea), fecal score of 4 (severe diarrhea), and fecal score of 5 (watery diarrhea) in all the experiments. The occurrence of diarrhea was defined as maintaining fecal scores of 2 or 3 for two consecutive days.
2.5 Determination of the organ index
Organ index (%) was calculated as the organ fresh weight (kg)/piglet weight (kg) × 100% before slaughter.
2.6 Apparent total tract digestibility
The apparent total tract digestibility (ATTD) was determined according to the endogenous indicator acid-insoluble ash (AIA) standard method (GB/T 23742–2009). The procedures utilized for the determination of nutrient composition were conducted with the international standard methods described by the AOAC International (17), including dry matter (930.15; AOAC), crude protein (930.15; AOAC), crude fat (920.39; AOAC), and crude ash (942.05; AOAC). For calculating the ATTD of the nutrients, we used the following formula: ((100-A1 × F2/A2 × F1) × 100). A1: digesta nutrient; A2: digesta AIA; F1: diet AIA; F2: digesta AIA.
2.7 Serum parameter measurements
The concentrations of serum total protein (TP), albumin (ALB), alkaline phosphatase (AKP), glutamic oxaloacetic transaminase (GOT), glutamic pyruvic transaminase (GPT), total cholesterol (TC), glucose (GLU), triglyceride (TG), and urea (UREA) were gaged using an automatic biochemical analyzer (Olympus, Shanghai, China). Immunoglobulin A (IgA), including IgA, immunoglobulin G (IgG), and immunoglobulin M (IgM), were determined using a multi-mode microplate reader (SpectraMax M2, Molecular Devices, Sunnyvale, CA, United States) following the procedures outlined by the corresponding commercially available swine Enzyme-Linked Immunosorbent Assay (ELISA) kits (Jiangsu Meimian Industrial Co., Ltd., Yancheng, China). The minimum detection limits were 1 μg/mL, 12 μg/mL, and 1.2 μg/mL, respectively, and the intra-assay coefficients of variation (CV) were 6.3, 7.3, and 6.7%, and inter-assay CV were 10.2, 9.7, and 12.3%, respectively. The standards provided in the kits were used to generate standard curves for quantification and each test was run in duplicate.
2.8 Intestinal morphology measurement
For the intestinal morphology study, approximately 3 cm segments isolated from mid-duodenum, mid-jejunum, and mid-ileum were immobilized with 4% paraformaldehyde solution for 24 h and then embedded in paraffin. After cutting the paraffin-coated intestinal samples into sections of approximately 3 μm, they were transferred to a 70% ethanol solution for dehydration. Afterward, the samples were wax-embedded on slides and stained with hematoxylin and eosin. Each section was put on a slide for intestinal morphology observation by using an Olympus CK40 inverted phase-contrast microscope equipped with the AxioVision software. The villus height (VH) was measured from the tip to the villi–crypt junction, and the crypt depth (CD) was measured from the villi base to the lowest point of the CD; 20 well-orientated and intact villi and crypts from each segment were counted to evaluate intestinal morphology.
2.9 Intestinal enzyme activities
The concentrations of sucrase, lactase, maltase, and alkaline phosphatase (AKP) in intestinal mucosa were evaluated in accordance with the instructions of the respective kits (Nanjing Jiancheng Bioengineering Institute). Briefly, approximately 100 mg of each thawed intestinal mucosa sample extracted from the duodenum, jejunum, and ileum was homogenized with a precooled 0.9% saline and then centrifuged at 3,000 × g, 4°C for 15 min. The supernatant was assayed for protein content in accordance with the Bradford method (18), following the sucrase, lactase, maltase, and AKP activities were measured in triplicate on a spectrophotometer. The results were normalized to protein concentration and expressed as U/mg protein.
2.10 Gene expression analysis
For RNA extraction, approximately 0.1 g of each frozen sample isolated from duodenum, jejunum, and ileum was homogenized with 1 mL of RNAiso Plus reagent (TaKaRa, Dalian, China) to extract the total RNA according to the manufacturer’s instructions. After analyzing integrity using 1.0% agarose gel, RNA purity and concentration were quickly determined on a spectrophotometer (NanoDrop-ND2000, Thermo Fisher Scientific, Inc., Waltham), of which OD260/OD280 ratio ranged from 1.8 to 2.0 were deemed appropriate. Subsequently, 1.0 μg of total RNA was reverse-transcribed into complementary DNA (cDNA) for RT-PCR using the PrimeScript™ RT reagent kit with gDNA Eraser (Takara Biotechnology Co., Ltd., Dalian, China).
As shown in Table 2, the primers were designed with Primer 5.0 software to amplify target gene fragments by performing q-PCR. The q-PCR was performed with the SYBR® Green PCR I PCR reagents (Takara Bio Inc., Dalian, China) on a CFX96 Real-Time PCR Detection System (Bio-Rad Laboratories, Hercules, CA, United States). All cDNA samples were detected in triplicate. Each q-PCR consisted of 1 μL of cDNA, 5 μL of SYBR Green JumpStart Taq ReadyMix (1×), 0.2 μL of ROX Reference Dye II (50×), 0.4 μL of each primer, and 3 μL of diethyl pyrocarbonate-treated water in a total volume of 10 μL. The protocol used in q-PCR was as follows: 95°C for 30 s, followed by 40 cycles: at 95°C for 5 s and 60°C for 34 s. The generated gene-specific amplification products were confirmed by melting curve analysis after each real-time quantitative PCR assay. The housekeeping gene glyceraldehyde-3-phosphate dehydrogenase (GAPDH) was used to standardize the mRNA expression level of target genes, which was calculated based on the 2–ΔΔCt method (19).
2.11 Intestinal microbiological analysis
The digesta samples (approximately 200 mg) isolated from the caecum and colon in individual pigs were used to extract total microbial DNA using the Stool DNA Kit (Omega Bio-Tek, Doraville, CA, United States) according to the manufacturer’s instructions. Based on the sequences downloaded from the National Center for Biotechnology Information (GenBank), the primers and probes listed in Table 3 were designed to amplify the reactions of total bacteria, E. coli, Lactobacillus, Bifidobacterium, and Bacillus on a CFX96 Real-Time PCR system (Bio-Rad Laboratories, Inc., Hercules, CA).
More precisely, total bacteria were detected by the reaction, which runs in a volume of 25 μL with 12.5 μL SYBR Premix Ex Taq (2×), 1 μL of each primer (100 nmol/L), 1 μL 50 × ROX Reference Dye*3, 7.5 μL of RNase-Free ddH2O, and 2 μL template DNA. The SuperReal PreMix (Probe) kit (Tiangen Biotech Co., Ltd., Beijing, China) was used for Lactobacillus, E. coli, Bacillus, and Bifidobacterium detection. Each reaction was run in a volume of 25 μL with 12.5 μL Super Real PreMix (2×), 1 μL of each primer (100 nmol/L), 1 μL probe (100 nmol/L), 1 μL 50 × ROX Reference Dye*3, 6.5 μL of RNase-Free ddH2O, and 2 μL DNA. All reaction cycling protocols consisted of enzyme activation and denaturation at 95°C for 15 min; 40 cycles of 95°C for 3 s and 60°C for 30 s, followed by dissociation at 60–95°C with 0.5°C increments every 1 s. The cycle threshold (Ct) values and baseline settings were determined by automatic analysis settings, and the copy numbers of the target group for each reaction were calculated from the standard curves, which were generated by constructing standard plasmids by a 10-fold serial dilution of plasmid DNA (1 × 101 to 1 × 109 copies/μL).
2.12 Analysis of SCFAs
The SCFAs in digesta were determined using gas–liquid chromatography according to the previously method described (20). Briefly, approximately 0.5 g of thawed digesta was diluted with 2 mL of sterile Milli-Q water. After being vortexed, the entire sample was centrifuged at 12,000 × g for 10 min to obtain the suspension liquid. Then, a sample of 25% metaphosphoric acid solution was combined in a 9:1 ratio and centrifuged at 12,000 × g again for 10 min after being placed at 4°C for 30 min. Finally, a 0.45-mm filter membrane was used to filter the supernatant after it was aspirated with a syringe. The gas chromatographic system (VARIAN CP-3800, America) equipped with a polyethylene glycol-packed column with an inner diameter of 0.32 mm and 30 m length, and 0.25 μm film thickness, was used to separate and quantify the SCFAs (e.g., acetate, propionate, and butyrate). After each injection sample (1 μL) entered the column, the column temperature rose from the initial temperature of 70°C to 150°C within 3 min, while the temperature of the injector and detector reached 250°C.
2.13 Statistical analysis
All data were subjected to a one-way analysis of variance for a completely randomized design using the general linear model procedure of SPSS 24.0 (SPSS, Inc.), with each pig representing one experimental unit. Statistical differences among treatments were separated using Tukey’s multiple-range test. The results are expressed as with their standard errors. Statistical significance was set at p < 0.05 and 0.05 < p < 0.10, indicating a trend.
3 Results
3.1 Effect of DMY on growth performance, organ index, and nutrient digestibility in weaned pigs upon ETEC challenge
Before the ETEC challenge, DMY treatment had no effect (p > 0.05) on initial weight, final weight, and F/G (Table 4). During days 19–21, ETEC significantly reduced (p < 0.05) the final weight and ADG in the weaned pigs of the ECON group compared to the pigs of the CON group. In particular, DMY supplementation significantly increased (p < 0.05) the final weight and ADG but decreased (p < 0.05) the diarrhea incidence and kidney index in the weaned pigs of the EDMY group compared to the pigs of the ECON group (Table 5). Compared to the CON and ECON groups, the EDMY group showed a significant increase in the apparent digestibility of DM, EE, GE, and ash (Table 6).
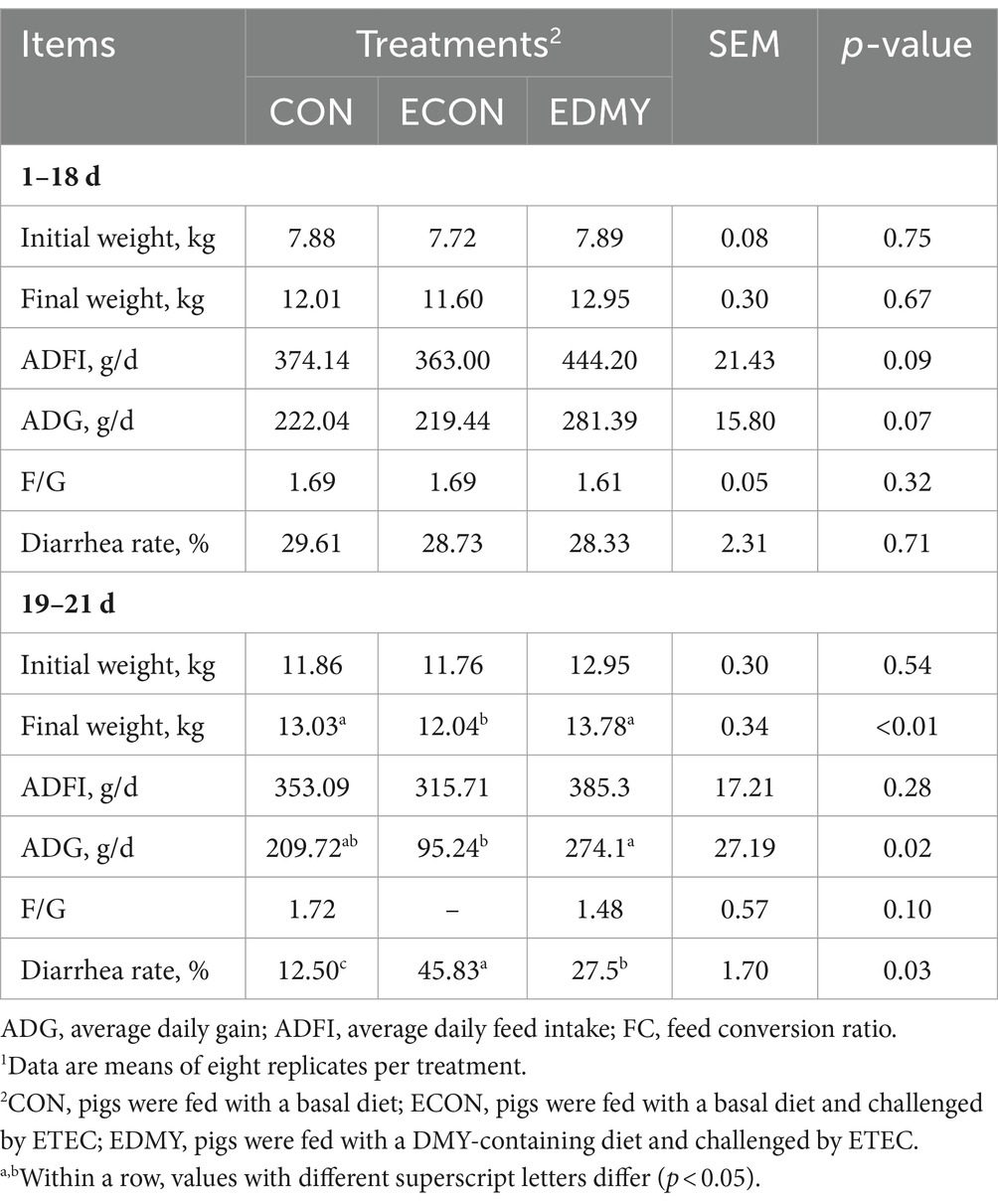
Table 4. Effect of dietary DMY supplementation on the growth performance of weaned pigs upon ETEC challenge.1
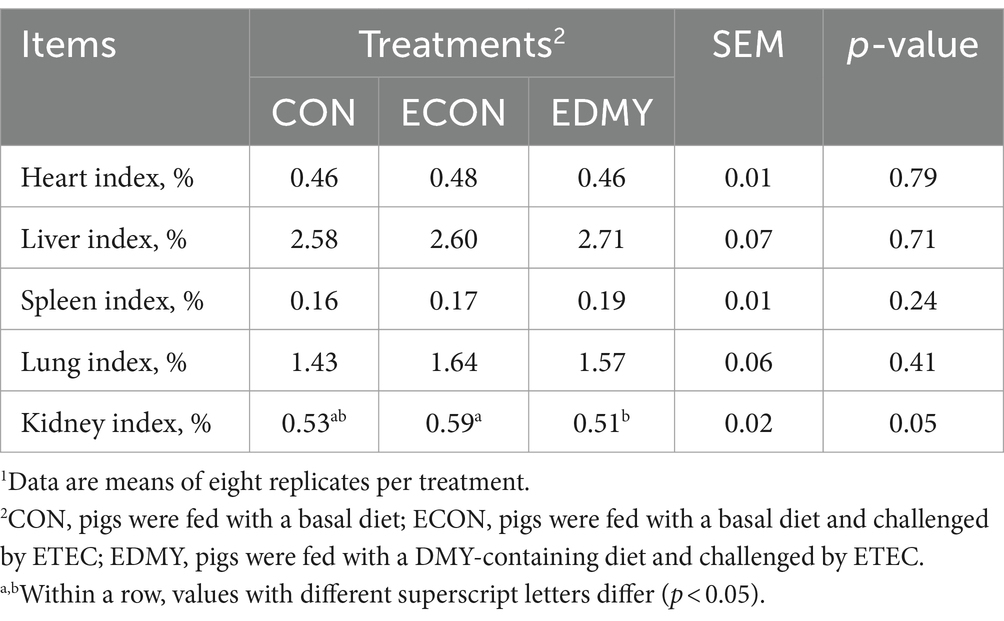
Table 5. Effects of dietary DMY supplementation on organ index of weaned piglets upon ETEC challenge.1
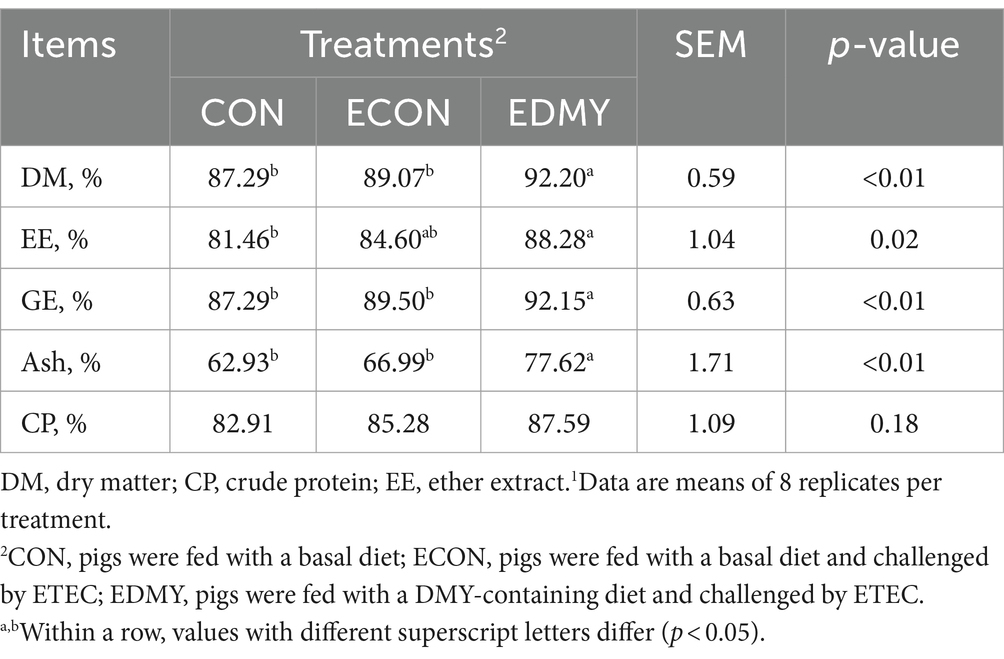
Table 6. Effect of dietary DMY supplementation on nutrient digestibility of weaned pigs upon ETEC challenge1.
3.2 Effect of DMY on serum biochemical indices and immunoglobulins in weaned pigs upon ETEC challenge
As shown in Figure 1, the ETEC challenge significantly decreased (p < 0.05) concentrations of immunoglobulins such as IgA and IgG in the weaned pigs of the ECON group compared to the pigs of the CON group. However, DMY supplementation not only decreased (p < 0.05) albumin/globulin ratio but also elevated levels of serum levels of IgA, IgG, and IgM in the weaned pigs of the EDMY group compared to the pigs of the ECON group (Figure 2).
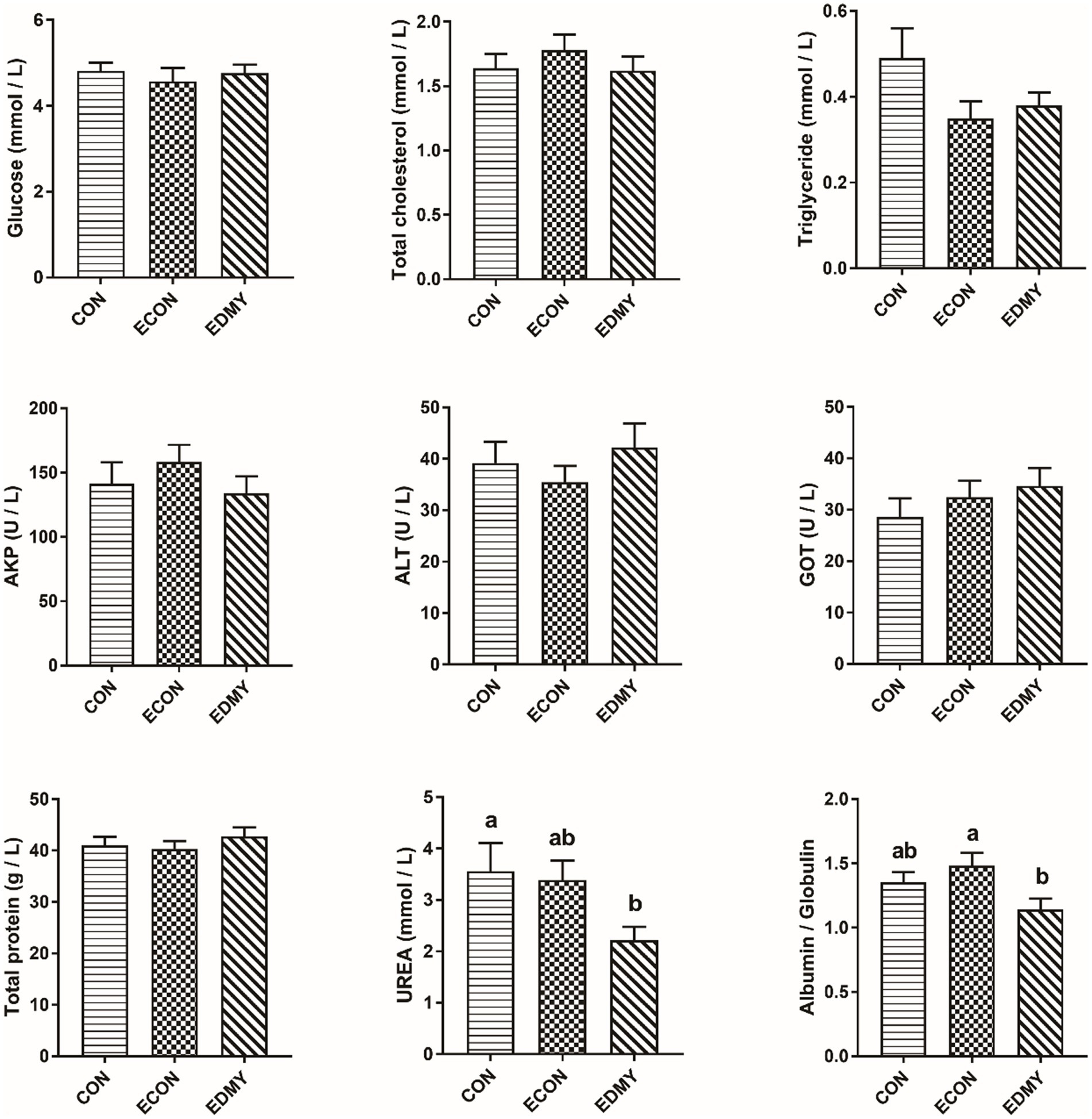
Figure 1. Effect of dietary DMY supplementation on plasma biochemical indices concentrations of weaned pigs upon ETEC challenge. Values are means ± SEM, (n = 8). CON, pigs were fed with a basal diet; ECON, pigs were fed with a basal diet and challenged by ETEC; EDMY, pigs were fed with a DMY containing diet and challenged by ETEC. AKP, alkaline phosphatase; ALT, alanine aminotransferase; GOT, glutamic oxalacetic transaminase.
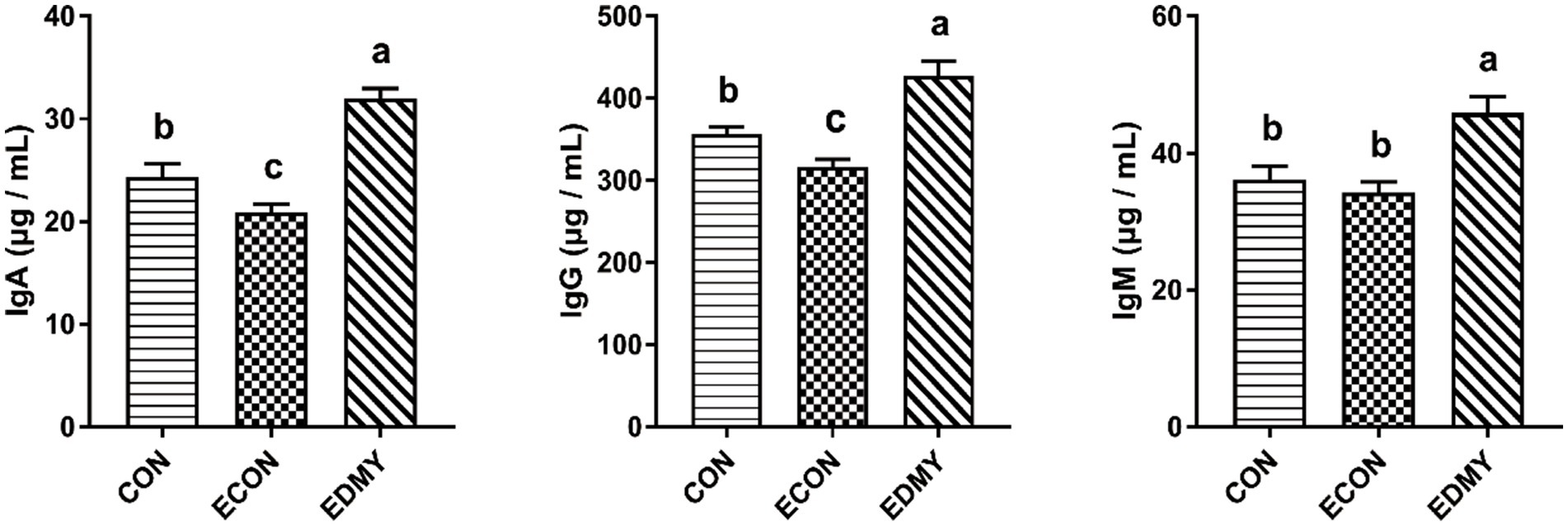
Figure 2. Effect of dietary DMY supplementation on plasma Immunoglobulin concentrations of weaned pigs upon ETEC challenge. Values are means ± SEM, (n = 8). CON, pigs were fed with a basal diet; ECON, pigs were fed with a basal diet and challenged by ETEC; EDMY, pigs were fed with a DMY containing diet and challenged by ETEC. IgA, immunoglobulin A; IgG, immunoglobulin G; IgM, immunoglobulin M.
3.3 Effect of DMY supplementation on intestinal morphology and mucosal enzyme activity in weaned pigs upon ETEC challenge
The ETEC challenge significantly decreased (p < 0.05) the villus height of the jejunum and ileum of the weaned pigs of the ECON group compared to those in pigs of the CON group. However, DMY treatment significantly increased (p < 0.05) the villus height of the jejunum and ileum of the weaned pigs of the EDMY group compared to those in pigs of the ECON group. For crypt depth, DMY treatment significantly decreased (p < 0.05) the crypt depth in the duodenum of the weaned pigs of the EDMY group compared to that in pigs of the ECON group. Additionally, although the ETEC challenge significantly decreased (p < 0.05) ratio of V: C in the duodenum and jejunum of the weaned pigs of the ECON group compared to those in pigs of the CON group, DMY treatment eliminated this effect (Figure 3).
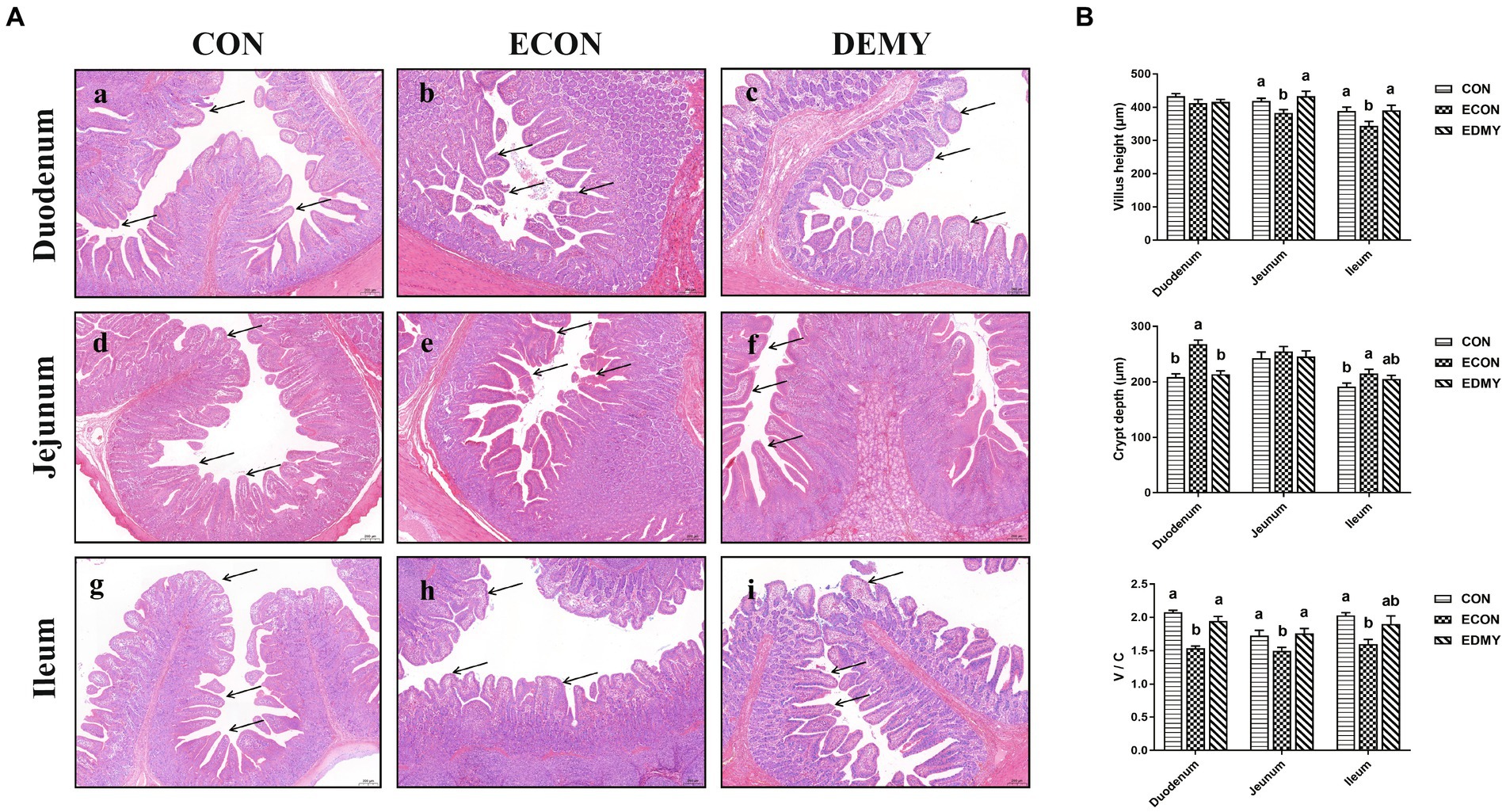
Figure 3. Effect of dietary DMY supplementation on small intestinal morphology of weaned pigs upon ETEC challenge (H&E; × 40). (A) Representative photomicrographs of villi in the duodenum (a−c), jejunum (d−f) and ileum (g−i) from pigs in the CON, ECON, and EDMY groups, respectively. (B) Villus height, crypt depth, and villus height to crypt depth ratio of the duodenum, jejunum, and ileum of piglets in the CON, ECON, and EDMY groups, respectively. CON, pigs were fed with a basal diet; ECON, pigs were fed with a basal diet and challenged by ETEC; EDMY, pigs were fed with a DMY containing diet and challenged by ETEC.
In Table 7, DMY treatment increased the duodenal mucosa AKP, sucrase, and maltase activities, jejunal mucosa lactase and sucrase activities, and ileal mucosa sucrase activity in the weaned pigs of the EDMY group compared to the pigs of the ECON group (p < 0.05). Moreover, the mucosa activities of sucrase and maltase in the duodenum were higher (p < 0.05) in the EDMY group than those in the ECON group.
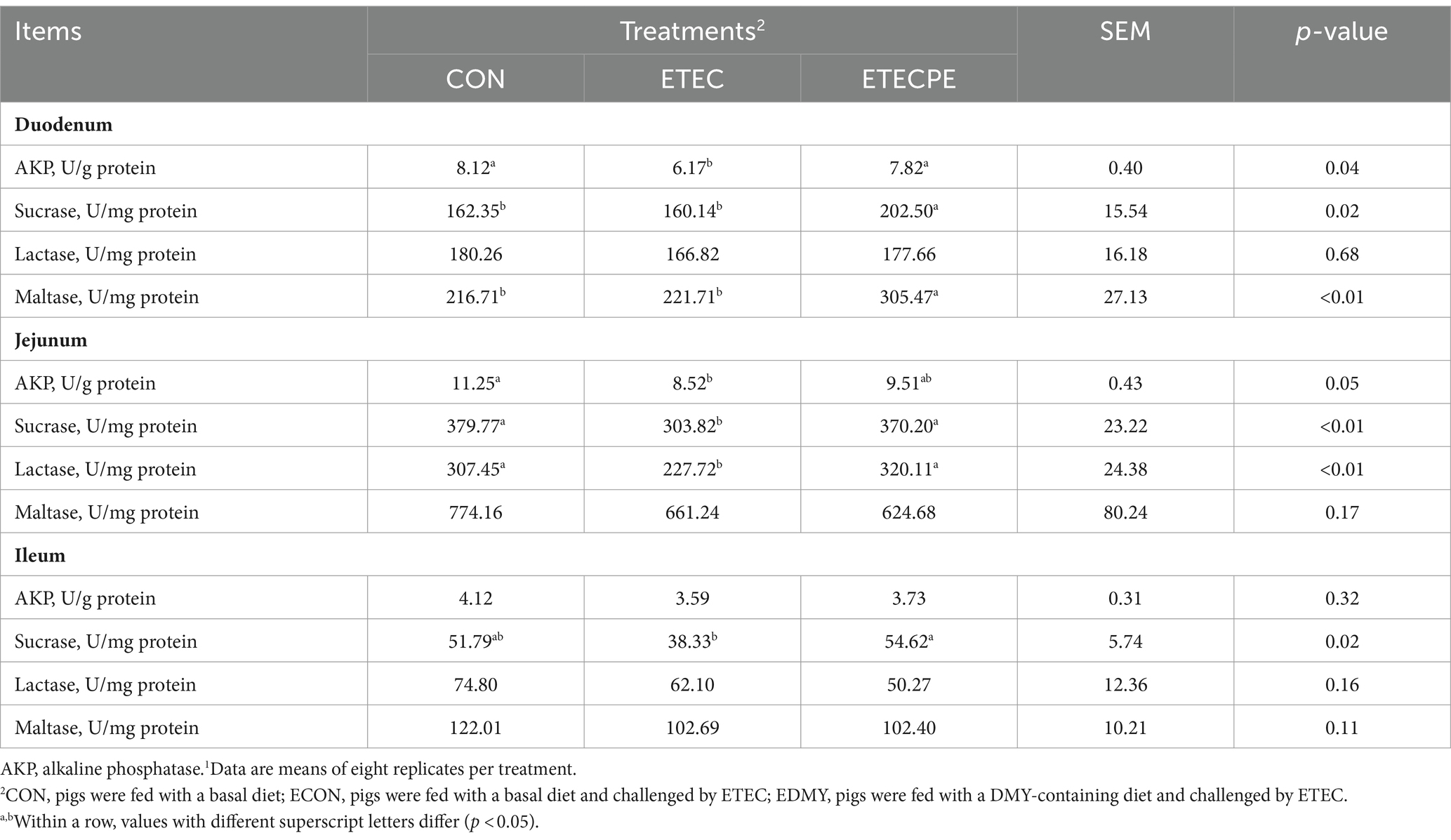
Table 7. Effect of dietary DMY supplementation on mucosal enzyme activity of small intestine in weaned piglets upon ETEC challenge.1
3.4 Effect of DMY supplementation on expressions of critical genes involved in intestinal epithelium functions
As shown in Figure 4, the ETEC challenge significantly decreased (p < 0.05) the expression levels of GLUT2, CAT-1, and FATP-1 in the jejunum of the weaned pigs of the ECON group compared to those in pigs of the CON group. However, DMY supplementation not only elevated the mRNA expression levels of ZO-1 and Claudin-1 in the jejunum but also elevated the mRNA expression levels of CAT-1 and FATP-1 in the duodenum of the EDMY group compared to those of the ECON group (p < 0.05).
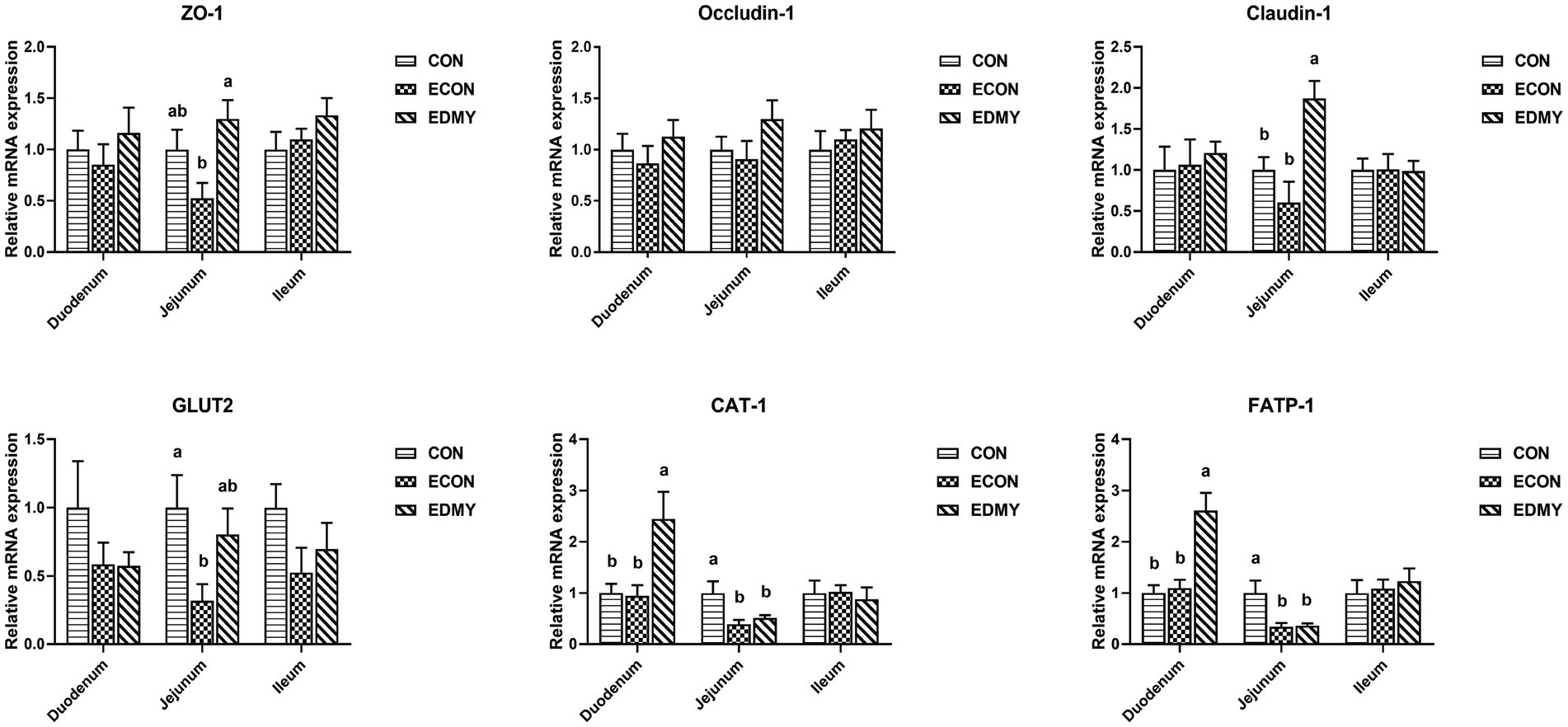
Figure 4. Relative expression levels of critical genes involved in the intestinal barrier functions. Values are means ± SEM, (n = 8). Within a panel, bars labeled with different superscript letters significantly different at P < 0.05. CON, pigs were fed with a basal diet; ECON, pigs were fed with a basal diet and challenged by ETEC; EDMY, pigs were fed with a DMY containing diet and challenged by ETEC. ZO-1, zonula occludens-1; GLUT2, glucose transporter-2; CAT1, cationic amino acid transporter-1; FATP1, Fatty acid transport protein-1.
3.5 Effect of DMY supplementation on intestinal microbial populations and metabolites in weaned pigs upon ETEC challenge
In Table 8, the ETEC challenge significantly increased (p < 0.05) the abundance of Escherichia coli in the cecum and colon as well as total bacteria in the colon of weaned piglets in the ECON group compared to those in the CON group. DMY supplementation significantly decreased (p < 0.05) the abundance of Escherichia coli in the colon of weaned piglets in the EDMY group compared to that in the ECON group. As shown in Figure 5, DMY supplementation significantly elevated (p < 0.05) the cecal and colonic concentrations of acetic acid and propanoic acid in weaned piglets of the EDMY group compared to those of the ECON group.
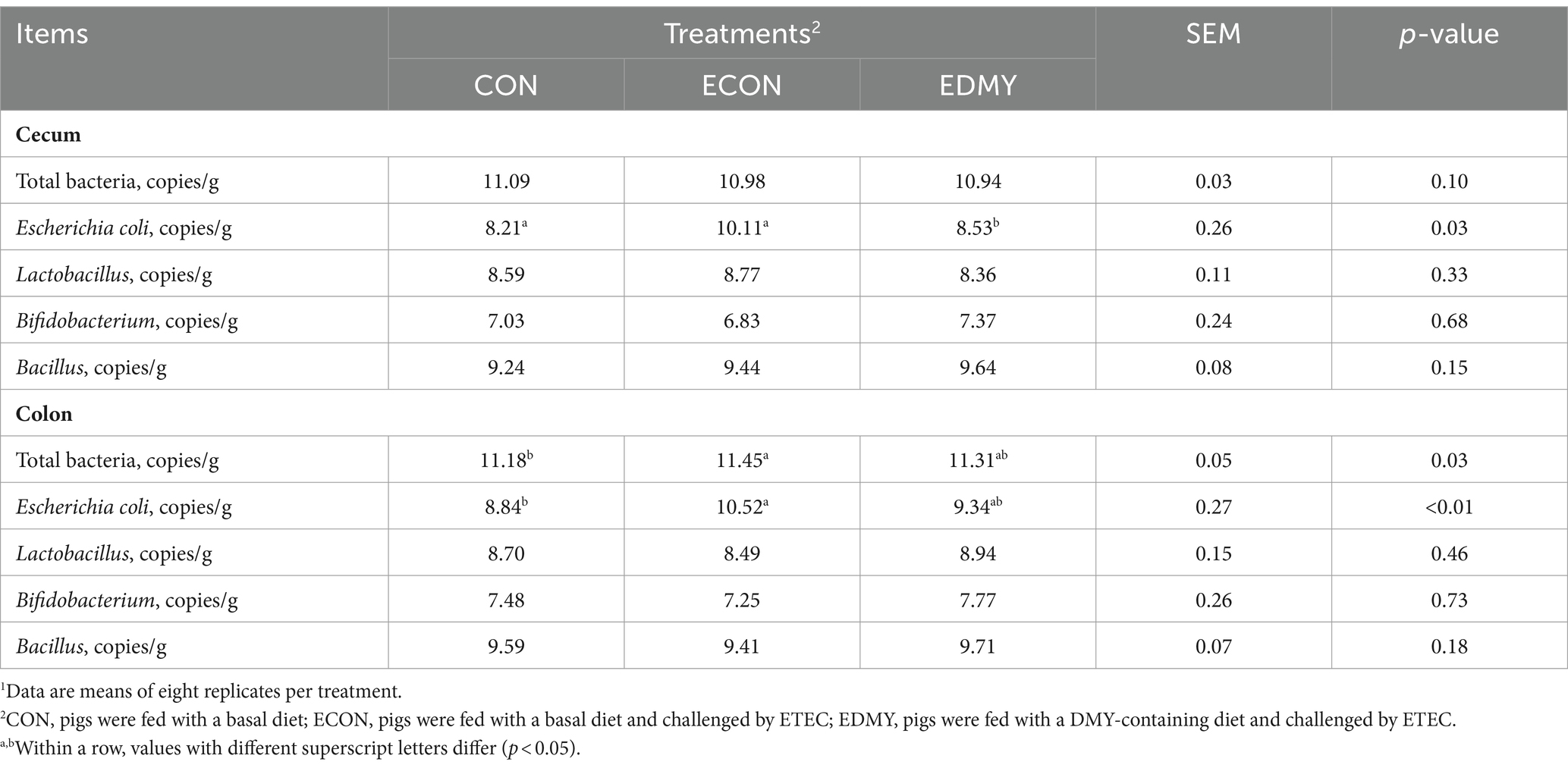
Table 8. Effect of dietary DMY supplementation on intestinal bacteria in digesta of cecum and colon in weaned piglets upon ETEC challenge.1
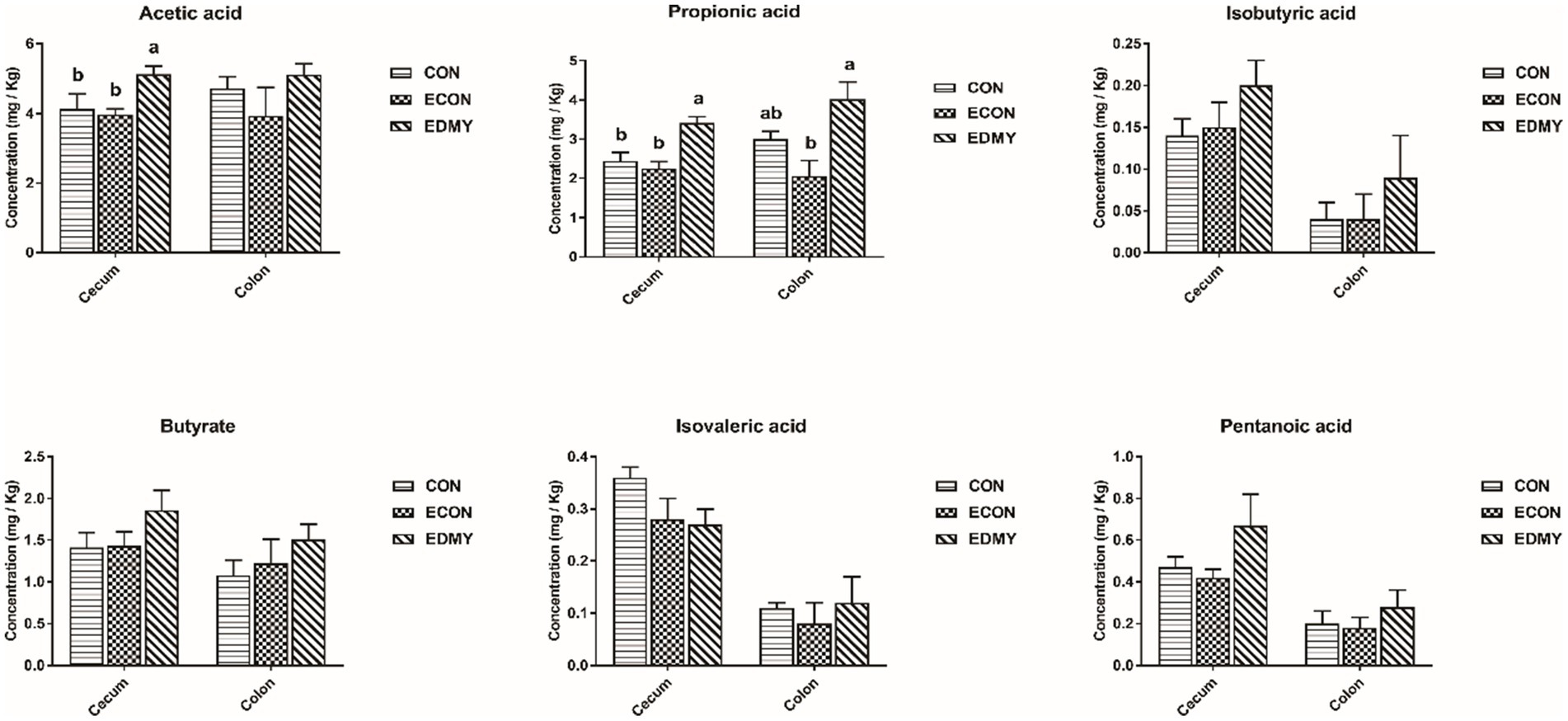
Figure 5. Effect of dietary DMY supplementation on concentrations of VFAs in digesta of cecum and colon in weaned pigs upon ETEC challenge. Values are means ± SEM, (n = 8). CON, pigs were fed with a basal diet; ECON, pigs were fed with a basal diet and challenged by ETEC; EDMY, pigs were fed with a DMY containing diet and challenged by ETEC.
4 Discussion
It has been shown that ETEC can cause intestine disturbance and an increase in diarrhea rate and F/G of piglets, thereby establishing a feasible model of intestinal infection (5, 6, 21). DMY has been extensively studied as it possesses multiple health-promoting biological and pharmacological properties, of which antioxidative ability (12), anti-inflammatory (11), immune-enhancing (12), and intestinal microbiota regulating biological activities (13) may be pertinent to improving growth performance of animals, especially during the weaning period. A previous study indicated that dietary supplementation of DMY at 500 mg/kg decreased F/G in growing-finishing pigs (12). In contrast, the current results suggested that oral administration of DMY alone did not show beneficial effects on F/G and diarrhea rate of piglets during days 1–18 (pre-challenge). Given that numerous plant-derived compounds are more effective when livestock animals suffer from pathogenic pressure (22–24). Accordingly, the positive effect of DMY was obvious under the condition of the ETEC challenge. In this study, we found that dietary DMY not only reversed the negative alternations in diarrhea rate caused by ETEC and therefore improved the ADG and F/G, but also increased the digestibility of DM, Ash, EE, and GE in the ETEC-challenged pigs, suggesting a beneficial effect of DMY supplementation on the intestinal health of weaned pigs.
The metabolic and health status of animal bodies or organs could be reflected by the blood biochemical parameters. In the male Sprague–Dawley rat study, the result showed that feeding 5 μg/kg body weight DMY decreased the level of blood urea nitrogen, which is a marker of kidney injury (25). Consistent with the previous report, this study found that dietary DMY had decreased serum UREA level and kidney index in the ETEC-challenged pigs, suggesting DMY alleviated the symptoms associated with the infection of weaned pigs challenged with ETEC. The albumin, globulin, and A/G ratio can be used as an indicator to indicate the situation of protein synthesis and nutritional status in vivo, and it is worth noting that a large number of immunoglobulins constitute an important source of globulin (26, 27). In this study, dietary DMY downregulated the plasma A/G ratio and globulin, indicating that DMY may influence the immune status of piglets. Generally, the levels of Immunoglobulins such as IgG, IgM, and IgA are used as indicators of immunological status, which play a critical role in clearing particular bacteria or viruses (28). In the present study, DMY significantly elevated the serum IgA, IgG, and IgM concentrations in the ETEC-challenged pigs, which is consistent with a previous study (12), indicating an enhanced immunity upon DMY supplementation.
The small intestine is of critical importance for nutrient digestion and absorption, and its villous height and V/C ratio have been considered as integrated morphological structure indicators to evaluate intestinal health, such as intestinal barrier integrity, nutrient digestion, and absorption capacity (29). Recently, several studies have confirmed that ETEC strains produce enterotoxins that act on the enterocytes, leading to the secretion of fluids and electrolytes, which ultimately results in the impaired intestinal barrier and function (4–6). Along the same lines, we found that the ETEC challenge decreased the duodenal and ileal villus height, and significantly reduced the ratio of V/C in the duodenum and jejunum, indicating injury of the intestinal epithelium. However, dietary DMY supplementation attenuated the intestinal injury by increasing the villus height and the ratio of V/C. Changes in the intestinal morphology are usually accompanied by the mucosal enzyme activities expressed in the brush border, which can reflect the function of intestinal epithelium (30). In the present study, dietary DMY supplementation not only elevated duodenal the activities of sucrase, maltase, and AKP but also elevated the jejunal activity of AKP, sucrase, and lactase in the ETEC-challenged pigs. As important endogenous enzymes, sucrase, maltase, and lactase are considered an excellent marker enzyme involved in carbohydrate digestion (31). However, alkaline phosphatase (AKP) has received wide attention due to its important protective properties in the gut, including absorption of lipids, detoxification of bacterial lipopolysaccharide, and possible modulation of the gut microbiota (32). All the evidence suggested that dietary DMY has a protective effect on intestinal health.
To gain insights into the mechanisms underlying the DMY-regulated intestinal health, we further investigated the expression levels of several critical genes involved in intestinal epithelium functions. The occludin, ZO-1, and Claudin-1 were members of transmembrane barrier proteins, cytoplasmic scaffold proteins, and adhesion molecules proteins of tight junctions (TJs), respectively, which play a key role in maintaining intestinal permeability (33). However, Escherichia coli lipopolysaccharide-induced injury in the intestinal barrier by reducing the expression of TJs (34). In the present study, dietary DMY supplementation not only significantly elevated the expression levels of ZO-1 and Claudin-1 but also elevated the expression levels of nutrient transporters (CAT-1, and FATP-1) in the intestinal epithelium of ETEC-challenged pigs. CAT-1 is a small molecule protein that is responsible for the transportation of cationic amino acids across cell membranes (35). However, the FATP-1 is closely associated with the transportation of fatty acids (36). The elevated expressions of these nutrient transporters may contribute to the improved barrier integrity and functions of intestinal epithelium in piglet exposure to the ETEC challenge.
It has been reported that DMY inhibits the growth of Staphylococcus aureus by disrupting membrane integrity and decreasing activities of a few energy metabolism enzymes, total ATPase (37). Similarly, we unexpectedly found that dietary DMY selectively inhibits the growth of potential pathogenic bacterial species (Escherichia coli) in this study. Recently, increasing evidence suggested that a dysregulated or perturbed state of the gut microbiota increases the susceptibility of piglets to enteric pathogens (38). Although we did not find that DMY affected the abundance of Lactobacillus, Bifidobacterium, and Bacillus in this study, it is necessary to conduct more extensive microbiome research in the future to explore whether DMY can improve ETEC-induced microbiota disturbance. Meanwhile, SCFAs (e.g., acetic acid, propionic acid, and butyric acid), as metabolites of the intestinal microbiota have been increasingly focused on due to their effective antimicrobial properties (39–41). Moreover, SCFAs not only act as a substrate for energy production but also regulate cell proliferation, apoptosis, and immunity, thereby promoting the functional maturation of intestinal epithelial cells (42). In the present study, dietary DMY was able to elevate SCFA levels in digesta of ETEC-challenged pigs. These results were consistent with the trend of intestinal barrier integrity and nutrient digestion and absorption capacity in the present study.
5 Conclusion
Hence, this study confirmed the protective effect of DMY as a dietary supplement in alleviating ETEC-induced intestinal injury in weaning pigs. As metabolites of intestinal microbiota regulated by DMY, SCFAs protect the intestinal barrier and nutrient digestion and absorption capacity, which might be the potential mechanism of dietary DMY supplements to protect intestinal injury induced by ETEC exposure. In addition, this study will also provide a new perspective on the application of dietary DMY in swine production.
Data availability statement
The raw data supporting the conclusions of this article will be made available by the authors, without undue reservation.
Ethics statement
The animal studies were approved by the Committee on Animal Care Advisory of Sichuan Agricultural University (authorization number SICAU-2022-014). All experiment procedures were carried out in accordance with the guidelines for the Care and Use of Laboratory Animals. The studies were conducted in accordance with the local legislation and institutional requirements. Written informed consent was obtained from the owners for the participation of their animals in this study.
Author contributions
KX: Formal analysis, Project administration, Software, Writing – original draft. JQ: Formal analysis, Project administration, Software, Writing – original draft. LD: Formal analysis, Writing – original draft. BY: Investigation, Methodology, Writing – review & editing. YuL: Investigation, Methodology, Writing – review & editing. ZH: Methodology, Writing – review & editing. XM: Methodology, Writing – review & editing. JY: Methodology, Writing – review & editing. PZ: Methodology, Writing – review & editing. HY: Methodology, Writing – review & editing. YaL: Methodology, Writing – review & editing. HL: Methodology, Writing – review & editing. JH: Conceptualization, Funding acquisition, Investigation, Resources, Writing – review & editing.
Funding
This study was supported by The National Key R&D Program of China (2023YFD1301200), and The Innovation Team of Sichuan Province (SCCXTD-2024-8).
Conflict of interest
The authors declare that the research was conducted in the absence of any commercial or financial relationships that could be construed as a potential conflict of interest.
The author(s) declared that they were an editorial board member of Frontiers, at the time of submission. This had no impact on the peer review process and the final decision.
Publisher’s note
All claims expressed in this article are solely those of the authors and do not necessarily represent those of their affiliated organizations, or those of the publisher, the editors and the reviewers. Any product that may be evaluated in this article, or claim that may be made by its manufacturer, is not guaranteed or endorsed by the publisher.
Abbreviations
ADFI, Average daily feed intake; ADG, Average daily gain; AIA, Acid-insoluble ash; AKP, Alkaline phosphatase; ALT, Alanine aminotransferase; DMY, Dihydromyricetin; CAT1, Cationic amino acid transporter-1; CON, Pigs were fed with a basal diet; CP, Crude protein; Ct, Cycle threshold; DM, Dry matter; ECON, Pigs were fed with a basal diet and challenged by ETEC; EE, Ether extract; EDMY, Pigs were fed with basal diet containing 300 mg/kg DMY product and challenged by ETEC; ETEC, Enterotoxigenic Escherichia coli; FATP-1, Fatty acid transport proteins 1; GE, Gross energy; Glu, Glucose; GLUT-2, Glucose transporter-2; GOT, Glutamic oxaloacetic transaminase; IgA, Immunoglobulins A; IgG, Immunoglobulins G; IgM, Immunoglobulins M; LB, Luria-Bertani; PBS, Phosphate-buffered saline; PWD, Post-weaning diarrhea; SGLT-1, Sodium/glucose cotransporter-1; TJ, Tight junctions; V: C, Villus height: crypt depth; VFA, Volatile fatty acids; ZO-1, Zonula occludens-1
References
1. Wijtten, PJ, van der Meulen, J, and Verstegen, MW. Intestinal barrier function and absorption in pigs after weaning: a review. Br J Nutr. (2011) 105:967–81. doi: 10.1017/S0007114510005660
2. Nagy, B, and Fekete, PZ. Enterotoxigenic Escherichia coli (ETEC) in farm animals. Vet Res. (1999) 30:259–84.
3. Ter Huurne, AA, and Gaastra, W. Swine dysentery: more unknown than known. Vet Microbiol. (1995) 46:347–60. doi: 10.1016/0378-1135(95)00049-G
4. von Astrid, M, and Ann-Mari, S. Colonization factors of human and animal-specific enterotoxigenic Escherichia coli (ETEC). Trends Microbiol. (2023) 32:448–64. doi: 10.1016/j.tim.2023.11.001
5. Thomas, S, Josefien, VL, Claude, D, Charlène, R, Adeline, S, Sandrine, C, et al. Role of mucus-bacteria interactions in Enterotoxigenic Escherichia coli (ETEC) H10407 virulence and interplay with human microbiome. NPJ Biofilms Microbiomes. (2022) 8:86. doi: 10.1038/s41522-022-00344-6
6. Zha, A, Tu, R, Qi, M, Wang, J, Tan, B, Liao, P, et al. Mannan oligosaccharides selenium ameliorates intestinal mucosal barrier, and regulate intestinal microbiota to prevent Enterotoxigenic Escherichia coli-induced diarrhea in weaned piglets. Ecotoxicol Environ Saf. (2023) 264:115448. doi: 10.1016/j.ecoenv.2023.115448
7. Sudan, S, Zhan, X, and Li, J. A novel probiotic Bacillus subtilis strain confers Cytoprotection to host pig intestinal epithelial cells during Enterotoxic infection. Microbiol Spectr. (2022) 10:e0125721. doi: 10.1128/spectrum.01257-21
8. Piegholdt, S, Pallauf, K, Esatbeyoglu, T, Speck, N, Reiss, K, Ruddigkeit, L, et al. Biochanin a and prunetin improve epithelial barrier function in intestinal CaCo-2 cells via downregulation of ERK, NF-κB, and tyrosine phosphorylation. Free Radic Biol Med. (2014) 70:255–64. doi: 10.1016/j.freeradbiomed.2014.02.025
9. Zheng, C, Zhong, Y, Zhang, W, Wang, Z, Xiao, H, Zhang, W, et al. Chlorogenic acid ameliorates post-infectious irritable bowel syndrome by regulating extracellular vesicles of gut microbes. Adv Sci. (2023) 10:e2302798. doi: 10.1002/advs.202302798
10. Zhang, J, Liang, F, Chen, Z, Chen, Y, Yuan, J, Xiong, Q, et al. Vitexin protects against dextran sodium sulfate-induced colitis in mice and its potential mechanisms. J Agric Food Chem. (2022) 70:12041–54. doi: 10.1021/acs.jafc.2c05177
11. Hou, XL, Tong, Q, Wang, WQ, Shi, CY, Xiong, W, Chen, J, et al. Suppression of inflammatory responses by Dihydromyricetin, a flavonoid from Ampelopsis grossedentata, via inhibiting the activation of NF-κB and MAPK signaling pathways. J Nat Prod. (2015) 78:1689–96. doi: 10.1021/acs.jnatprod.5b00275
12. Wei, C, Chen, X, Chen, D, He, J, Zheng, P, Chen, H, et al. Effects of dietary dihydromyricetin supplementation on intestinal barrier and humoral immunity in growing-finishing pigs. Anim Biotechnol. (2022) 33:1398–406. doi: 10.1080/10495398.2022.2099881
13. Fan, L, Zhao, X, Tong, Q, Zhou, X, Chen, J, Xiong, W, et al. Interactions of Dihydromyricetin, a flavonoid from vine tea (Ampelopsis grossedentata) with gut microbiota. J Food Sci. (2018) 83:1444–53. doi: 10.1111/1750-3841.14128
14. Chen, Y, Song, Z, Chang, H, Guo, Y, Wei, Z, Sun, Y, et al. Dihydromyricetin inhibits African swine fever virus replication by downregulating toll-like receptor 4-dependent pyroptosis in vitro. Vet Res. (2023) 54:58. doi: 10.1186/s13567-023-01184-8
15. Chang, Y, Yuan, L, Liu, J, Muhammad, I, and Liu, F. Dihydromyricetin attenuates Escherichia coli lipopolysaccharide-induced ileum injury in chickens by inhibiting nlrp3 inflammasome and TLR4/NF-κB signalling pathway. Vet Res. (2020) 51:72. doi: 10.1186/s13567-020-00796-8
16. Wang, Z, Jiang, Q, Li, P, Shi, P, Liu, C, Wang, W, et al. The water extract of Ampelopsis grossedentata alleviates oxidative stress and intestinal inflammation. Antioxidants (Basel). (2023) 12:547. doi: 10.3390/antiox12030547
17. AOAC (Association of Official Analytical Collaboration). Official methods of analyses. 18th ed. Arlington, VA: AOAC (2007).
18. Bradford, MM. A rapid and sensitive method for the quantitation of microgram quantities of protein utilizing the principle of protein-dye binding. Anal Biochem. (1976) 72:248–54. doi: 10.1016/0003-2697(76)90527-3
19. Yan, H, Cao, S, Li, Y, Zhang, H, and Liu, J. Reduced meal frequency alleviates high-fat diet-induced lipid accumulation and inflammation in adipose tissue of pigs under the circumstance of fixed feed allowance. Eur J Nutr. (2020) 59:595–608. doi: 10.1007/s00394-019-01928-3
20. Cummings, JH, Pomare, EW, Branch, WJ, Naylor, CP, and Macfarlane, GT. Short chain fatty acids in human large intestine, portal, hepatic and venous blood. Gut. (1987) 28:1221–7. doi: 10.1136/gut.28.10.1221
21. Xiao, D, Wang, Y, Liu, G, He, J, Qiu, W, Hu, X, et al. Effects of chitosan on intestinal inflammation in weaned pigs challenged by enterotoxigenic Escherichia coli. PLoS One. (2014) 9:e104192. doi: 10.1371/journal.pone.0104192
22. Luo, X, Wu, S, Jia, H, Si, X, Song, Z, Zhai, Z, et al. Resveratrol alleviates enterotoxigenic Escherichia coli K88-induced damage by regulating SIRT-1 signaling in intestinal porcine epithelial cells. Food Funct. (2022) 13:7346–60. doi: 10.1039/D1FO03854K
23. Smith, BN, Oelschlager, ML, Abdul Rasheed, MS, and Dilger, RN. Dietary soy isoflavones reduce pathogen-related mortality in growing pigs under porcine reproductive and respiratory syndrome viral challenge. J Anim Sci. (2020) 98:30–1. doi: 10.1093/jas/skaa054.054
24. Zhang, X, Zhao, Q, Ci, X, Chen, S, Xie, Z, Li, H, et al. Evaluation of the efficacy of chlorogenic acid in reducing small intestine injury, oxidative stress, and inflammation in chickens challenged with Clostridium perfringens type a. Poult Sci. (2020) 99:6606–18. doi: 10.1016/j.psj.2020.09.082
25. Wang, J, Jiao, P, Zhou, Y, and Liu, Q. Protective effect of Dihydromyricetin against lipopolysaccharide-induced acute kidney injury in a rat model. Med Sci Monit. (2016) 22:454–9. doi: 10.12659/MSM.897076
26. Fahey, JL, and Sell, S. The immunoglobulins of mice. The metabolic (catabolic) properties of five immunoglobulin classes. J Exp Med. (1965) 122:41–58. doi: 10.1084/jem.122.1.41
27. Park, JH, and Kim, IH. The effects of betaine supplementation in diets containing different levels of crude protein and methionine on the growth performance, blood components, total tract nutrient digestibility, excreta noxious gas emission, and meat quality of the broiler chickens. Poult Sci. (2019) 98:6808–15. doi: 10.3382/ps/pez412
28. Chen, K, Magri, G, Grasset, EK, and Cerutti, A. Rethinking mucosal antibody responses: IgM, IgG and IgD join IgA. Nat Rev Immunol. (2020) 20:427–41. doi: 10.1038/s41577-019-0261-1
29. Van Beers-Schreurs, HM, Nabuurs, MJ, Vellenga, L, Kalsbeek-van der Valk, HJ, Wensing, T, and Breukink, HJ. Weaning and the weanling diet influence the villous height and crypt depth in the small intestine of pigs and alter the concentrations of short-chain fatty acids in the large intestine and blood. J Nutr. (1998) 128:947–53. doi: 10.1093/jn/128.6.947
30. Støy, AC, Heegaard, P, Thymann, T, Bjerre, M, Skovgaard, K, Boye, M, et al. Bovine colostrum improves intestinal function following formula-induced gut inflammation in preterm pigs. Clin Nutr. (2014) 33:322–9. doi: 10.1016/j.clnu.2013.05.013
31. Williamson, G. Possible effects of dietary polyphenols on sugar absorption and digestion. Mol Nutr Food Res. (2013) 57:48–57. doi: 10.1002/mnfr.201200511
32. Lallès, JP. Intestinal alkaline phosphatase: novel functions and protective effects. Nutr Rev. (2014) 72:82–94. doi: 10.1111/nure.12082
33. Kuo, WT, Zuo, L, Odenwald, MA, Madha, S, Singh, G, Gurniak, CB, et al. The tight junction protein ZO-1 is dispensable for barrier function but critical for effective mucosal repair. Gastroenterology. (2021) 161:1924–39. doi: 10.1053/j.gastro.2021.08.047
34. Becker, SL, Li, Q, Burrough, ER, Kenne, D, Sahin, O, Gould, SA, et al. Effects of an F18 enterotoxigenic Escherichia coli challenge on growth performance, immunological status, and gastrointestinal structure of weaned pigs and the potential protective effect of direct-fed microbial blends. J Anim Sci. (2020) 98:1–10. doi: 10.1093/jas/skaa113
35. Devés, R, and Boyd, CA. Transporters for cationic amino acids in animal cells: discovery, structure, and function. Physiol Rev. (1998) 78:487–545. doi: 10.1152/physrev.1998.78.2.487
36. Li, F, Duan, Y, Li, Y, Tang, Y, Geng, M, Oladele, OA, et al. Effects of dietary n-6: n-3 PUFA ratio on fatty acid composition, free amino acid profile and gene expression of transporters in finishing pigs. Br J Nutr. (2015) 113:739–48. doi: 10.1017/S0007114514004346
37. Liang, H, He, K, Li, T, Cui, S, Tang, M, Kang, S, et al. Mechanism and antibacterial activity of vine tea extract and dihydromyricetin against Staphylococcus aureus. Sci Rep. (2020) 10:214–6. doi: 10.1038/s41598-020-78379-y
38. He, K, Yan, W, Sun, C, Liu, J, Bai, R, Wang, T, et al. Alterations in the diversity and composition of gut microbiota in weaned piglets infected with Balantioides coli. Vet Parasitol. (2020) 288:109298. doi: 10.1016/j.vetpar.2020.109298
39. Cieplak, T, Soffer, N, Sulakvelidze, A, and Nielsen, DS. A bacteriophage cocktail targeting Escherichia coli reduces E. coli in simulated gut conditions, while preserving a non-targeted representative commensal normal microbiota. Gut Microbes. (2018) 9:391–9. doi: 10.1080/19490976.2018.1447291
40. Stecher, B. The roles of inflammation, nutrient availability and the commensal microbiota in enteric pathogen infection. Microbiol Spectr. (2015) 3:3. doi: 10.1128/microbiolspec.MBP-0008-2014
41. Van Der Wielen, PW, Biesterveld, S, Notermans, S, Hofstra, H, Urlings, BA, and van Knapen, F. Role of volatile fatty acids in development of the cecal microflora in broiler chickens during growth. Appl Environ Microbiol. (2000) 66:2536–40. doi: 10.1128/AEM.66.6.2536-2540.2000
Keywords: Escherichia coli, immunity, DMY, intestinal epithelium, microbiota, weaned pigs
Citation: Xie K, Qi J, Deng L, Yu B, Luo Y, Huang Z, Mao X, Yu J, Zheng P, Yan H, Li Y, Li H and He J (2024) Dihydromyricetin improves growth performance, immunity, and intestinal functions in weaned pigs challenged by enterotoxigenic Escherichia coli. Front. Vet. Sci. 11:1421871. doi: 10.3389/fvets.2024.1421871
Edited by:
Elena De Felice, University of Camerino, ItalyReviewed by:
Hongbin Pan, Yunnan Agricultural University, ChinaTsungcheng Tsai, University of Arkansas, United States
Copyright © 2024 Xie, Qi, Deng, Yu, Luo, Huang, Mao, Yu, Zheng, Yan, Li, Li and He. This is an open-access article distributed under the terms of the Creative Commons Attribution License (CC BY). The use, distribution or reproduction in other forums is permitted, provided the original author(s) and the copyright owner(s) are credited and that the original publication in this journal is cited, in accordance with accepted academic practice. No use, distribution or reproduction is permitted which does not comply with these terms.
*Correspondence: Jun He, aGVqdW44MDY3QDE2My5jb20=
†These authors have contributed equally to this work