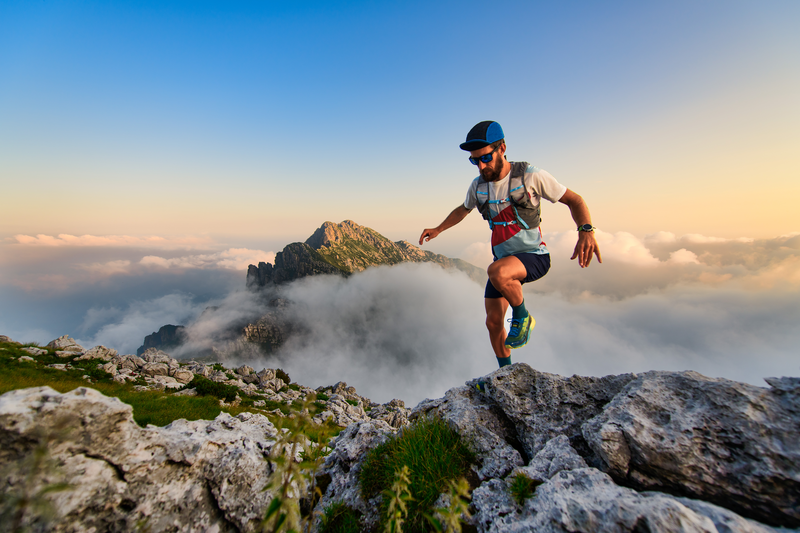
95% of researchers rate our articles as excellent or good
Learn more about the work of our research integrity team to safeguard the quality of each article we publish.
Find out more
ORIGINAL RESEARCH article
Front. Vet. Sci. , 28 May 2024
Sec. Veterinary Infectious Diseases
Volume 11 - 2024 | https://doi.org/10.3389/fvets.2024.1382288
Pullorum disease (PD) is a bacterial infection caused by Salmonella pullorum (S. pullorum) that affects poultry. It is highly infectious and often fatal. Antibiotics are currently the mainstay of prophylactic and therapeutic treatments for PD, but their use can lead to the development of resistance in pathogenic bacteria and disruption of the host's intestinal flora. We added neomycin sulfate and different doses of tannic acid (TA) to the drinking water of chicks at 3 days of age and infected them with PD by intraperitoneal injection of S. pullorum at 9 days of age. We analyzed intestinal histopathological changes and the expression of immune-related genes and proteins by using the plate smear method, histological staining, real-time fluorescence quantitative PCR, ELISA kits, and 16S rRNA Analysis of intestinal flora. The results demonstrate that S. pullorum induces alterations in the immune status and impairs the functionality of the liver and intestinal barrier. We found that tannic acid significantly ameliorated S. pullorum-induced liver and intestinal damage, protected the intestinal physical and chemical barriers, restored the intestinal immune barrier function, and regulated the intestinal flora. Our results showed that TA has good anti-diarrhoeal, growth-promoting, immune-regulating, intestinal barrier-protecting and intestinal flora-balancing effects, and the best effect was achieved at an additive dose of 0.2%.
Pullorum disease (PD) is a septicaemia that affects chickens, turkeys, and other poultry, caused by Salmonella pullorum infection. Clinical manifestations in chicks include anorexia, diarrhea, debilitation, reduced egg production, and increased mortality rate (1, 2). PD outbreaks can cause significant financial losses for the poultry farming industry in developing nations (3). Research has shown that S. pullorum invasion initially causes severe damage to chick intestinal function and microbiota and subsequently spreads to host tissues and organs, weaken immunity, causing septicaemia and compromising the immune responses (4–6). Tetracycline and kanamycin are commonly used drugs in the clinical treatment and prevention of PD. Nevertheless, prolonged use of these antibiotics can lead to the development of resistance in S. pullorum and disrupt the host's intestinal flora, resulting in serious residual problems (7–9). Therefore, it is essential to find a safe and effective alternative to antibiotics.
Tannins are naturally occurring polyphenolic compounds primarily known for their haemostatic, anti-infective, and anti-diarrhoeal properties (10, 11). Tannic acid (TA), the most basic form of hydrolyzed tannins, plays a crucial role in traditional Chinese medicines like gallnut. Recent research has revealed that tannic acid can significantly improve animal performance, regulate immunological function, and enhance resistance against disease (12–14). In addition, TA has been approved by regulatory authorities, such as the US Food and Drug Administration (FDA) (11) and the European Union (15), as a food additive and flavoring agent due to its potential antimicrobial properties. The results presented above demonstrate TA's effective antimicrobial activity and biosafety. Furthermore, TA has been shown to effectively reduce damage to the intestinal mucosa caused by oxidative stress and inflammatory responses (16, 17), improve gut microbial composition, and enhance intestinal barrier function (18–20). Therefore, TA may have a preventive effect against PD and could alleviate the intestinal damage caused by S. pullorum.
Brus et al. (21) demonstrated that concentrations of TA solutions ranging from 0.05 to 0.1% can promote the growth of small intestinal epithelial cells in chickens. However, no studies have investigated the use of tannic acid as a supplement in poultry drinking water for the treatment of PD. Moreover, the exact influence of TA on the function of the intestinal mucosal barrier and the composition of the intestinal flora remains incompletely comprehended. Thus, this study seeks to examine the consequences of tannic acid on the gut's morphological structure and barrier function in broilers infected with S. pullorum, and to investigate potential mechanisms associated with microbiota involvement.
TA, extracted from gallnut, was purchased from Wufeng Chicheng Biotech Co., Ltd (content ≥ 95%, 20220309), quality requirements outlined in the FDA's “Food Chemical Specification” 5th edition and the U.S. Food Chemical Codex standards. Furthermore, it complied with the U.S. food FCC-IV standards.
The S. pullorum strain (CVCC1792) utilized in this study was acquired from the National Center for Veterinary Culture Collection (Beijing, China). The strain was activated in TSA medium and incubated at 37°C for 24 h. Following this, individual colonies were cultured in TSB medium and incubated at 37°C with a shaking speed of 180 rpm for 24 h. The cultured bacteria were then centrifuged (5,000 g/min, 5 min), washed three times with phosphate-buffered solution (PBS), and resuspended to achieve a final concentration of 1 × 109 CFU/ml.
A total of 240 1-day-old B380 broilers were purchased from Chengdu Tianxinli Poultry Co (Chengdu, China). The groups included: (1) the negative control group (NC), which did not receive TA treatment or S. pullorum infection; (2) the S. pullorum challenged group (SP), which received S. pullorum infection and 0% gallnut TA; (3) the positive control group (PC), which received S. pullorum infection and 0.04% Neomycin sulfate soluble powder in drinking water; (4) the TA low dose group (TAL), which received S. pullorum infection and 0.1% gallnut TA in drinking water; (5) the TA middle dose group (TAM), which received S. pullorum infection and 0.2% gallnut TA in drinking water; (6) the TA high dose group (TAH), which received S. pullorum infection and 0.3% gallnut TA in drinking water. In order to prevent cross-infection, various groups were segregated and maintained at a distance from one another's enclosures. At 3 days old, the administration was started, and lasted for a duration of 12 days.
At the age of 9 days, all groups, except for the NC group, were intraperitoneal injected with 0.5 ml of S. pullorum (1 × 109 CFU/ml). The NC group received an equivalent volume of PBS. The diets and drinking water were kept consistent throughout the experiment. Clinical signs were monitored daily, and changes in weight and mortality rates were recorded for each group of chicks.
The mortality rate of chicks following the challenge was recorded to determine the survival rate. At the age of 10 and 14 days, 20 chicks were chosen at random from each group for weighing. Additionally, six chicks were randomly selected to have blood samples collected from their jugular vein, which were subsequently centrifuged to obtain serum. Following the collection of blood, the chicks were administered anesthesia andeuthanized. Subsequently, the organ lesions were dissected and examined. Immune organs were weighed, and the organ-to-body weight ratio was calculated. Liver samples were collected for bacterial load determination, with a portion being immersed in 4% paraformaldehyde. Two sections each of mid-jejunum and mid-ileum (1 cm each) were collected; one section was fixed in 4% paraformaldehyde, while the other was frozen at −80°C. Cecal contents were collected and stored in liquid nitrogen for subsequent 16S rRNA sequencing.
On days 1 and 5 post-infection, a small amount of liver was weighed and homogenized in 1 ml of PBS. Serial dilutions of the homogenate were then spread on XLD agar plates to determine the quantity of S. pullorum.
The liver and 1 cm of the jejunum and ileum were collected, rinsed with PBS at 4°C, and fixed in 4% paraformaldehyde for 24 h at room temperature. Liver, jejunum, and ileum samples were then embedded in paraffin, sectioned at a thickness of 5 μm, and stained with hematoxylin-eosin (HE) for morphological analysis. Goblet cells in the jejunum were then counted using periodic acid-Schiff stain (PAS).
The concentrations of interleukin-4 (IL-4), interferon-γ (IFN-γ), immunoglobulin G (IgG), lipopolysaccharide (LPS), and Diamine oxidase (DAO) in serum, as well as sIgA in the jejunum, were determined using ELISA kits following the manufacturer's instructions (MlBio, Shanghai, China). The total protein concentration was assessed using the BCA assay kit (Beyotime, Shanghai, China).
Jejunum and tissue samples were collected on days 10 and 14. The samples were snap-frozen in liquid nitrogen and then transferred to −80°C for storage. Total RNA was extracted using TRIzol reagent (Biomed, Beijing, China) following the manufacturer's instructions. RNA (5 μg/μl) was reverse transcribed into cDNA using the M-MLV 4 First-Strand cDNA Synthesis Kit (Biomed, Beijing, China) and further applied to qRT-PCR in a step One plus System (Biosystems, Foster City, CA, USA) with double-stranded DNA. qRT-PCR was performed using Hieff UNICONR Universal Blue qPCR SYBR Green Master Mix (Yeasen Biotechnology Co., Ltd, Shanghai, China). The predenaturation procedure was 95°C for 30 s, followed by 40 cycles of 95°C for 10 s, and annealing/extension at the optimal annealing temperature for 30 s. All procedures were performed according to the manufacturer's instructions. The 2−ΔΔCt method was used to analyze the relative levels of gene expression. Gene expression levels were normalized to the internal standard, β-actin. The primer sequences (Tsingke Biotechnology Co., Ltd, China) used for gene expression analysis in this study are listed in Table 1.
The fresh digesta isolated from the cecum was quickly frozen in liquid nitrogen and then rapidly sent to Novogene Technology Co. (Beijing, China), Ltd. for intestinal microbiota analysis under dry ice conditions. The DNA was extracted using the CTAB/SDS (cetyltrimethylammonium bromide/sodium dodecyl sulfate) bromide method, and the concentration was detected by agarose gel electrophoresis, and then diluted to 1 ng/μl with sterile water. The V3–V4 region of 16S rRNA was amplified by PCR using specific primers: 341F(CCTAYGGGRBGCASCAG) and 806R(GGACTACNNGGGTATCTAAT). The process was followed by mixing and purification of PCR products, and library construction using the TruSeq® DNA PCR-Free Sample Preparation Kit. The constructed libraries were quantified using Qubit and Q-PCR, and then sequenced on the NovaSeq 6000 platform.
Data represent the mean ± standard deviation (SD) or mean ± standard error of the mean (SEM). One-way ANOVA with LSD post-hoc test was performed by using SPSS 27.0 software. Significance was determined at P < 0.05.
The survival rate and body weight of broiler chickens infected with S. pullorum improved with the addition of tannic acid to their drinking water, as shown in Figure 1A. The SP group had a survival rate of 70%, while the PC, TAL, TAM, and TAH groups had survival rates of 92.5%, 82.5%, 90%, and 95%, respectively. On day 5 post-infection, the body weight of chicks in the SP group decreased by 18% to 110.71 g ± 13.18, compared to the NC group (135.00 g ± 11.25, P < 0.0001). However, the chicks' body weight was normalized by treatment with TA, and the most significant increase was observed in the TAM group (Figure 1B).
Figure 1. Effects of tannic acid supplementation on survival and body weight of broiler chickens. (A) Survival of broiler chickens (n = 40) supplemented with tannic acid. (B) Body weight of broiler chickens (n = 20) supplemented with tannic acid. NC, negative control. SP, Salmonella pullorum challenge; PC, positive control; TAL, low-dose tannic acid; TAM, medium-dose tannic acid; TAH, high-dose tannic acid. Data are mean ± SD. Results are mean ± SD. ****P < 0.0001 (one-way ANOVA); ns, not significant (compared with NC group).
Figure 2 shows the organ index, liver bacterial load, and liver pathology findings. Chickens infected with S. pullorum had a significantly higher liver/body weight ratio on the first and fifth day of infection. In addition, there was a significant increase in the spleen/body weight ratio on the fifth day of infection. In contrast, the thymus/body weight ratios and bursa/body weight ratios were significantly reduced (P < 0.05; Figure 2A). On the first day of infection, supplementation of tannic acid resulted in a decrease in the bacterial load in the liver compared to the SP group, and returned the ratio of organ/body weight to normal levels (Figures 2A–C). The group treated with SP showed significant liver sinusoidal dilatation and extensive necrosis on the first day of the experiment. In contrast, the group treated with TA exhibited only mild hepatic inflammation and congestion. On day 5, the TAM and TAH groups showed almost normal liver morphology, except for the localized inflammation observed in the TAL group (Figures 2D, E). Nevertheless, the SP group still showed persistent liver damage and congestion.
Figure 2. Organ-specific gravity, liver bacterial load, and pathological changes in broilers. Liver/body weight ratio and immune organ/body weight ratio on days 1 and 5 post-infection (A) (n = 6). Bacterial loads (log10 CFU per gram of Salmonella pullorum) in the liver on day 1 (B) and day 5 (C) post infection (n = 6). Pathology of the liver of chicks in different treatment groups on day 1 (D) and day 5 (E) post infection (scale bar = 100 μm). Data are expressed as mean ± SD. Results are presented as mean § SEM. Different letters indicate significant differences between groups (P < 0.05). **P < 0.01 (One-way ANOVA). → : areas of liver necrosis; → : congested areas of liver.
Figure 3A shows the levels of serum cytokines IFN-γ, IL-4 and immunoglobulin IgG. The results indicate that S. pullorum infection significantly increased serum levels of IL-4, IFN-γ, and IgG on day 5 compared to the NC group (Figures 3A–C). Treatment with antibiotics and tannic acid reduced serum levels of IL-4 and IFN-γ in infected chicks, with the lowest levels observed in the TAH group (P < 0.05). Serum IgG levels remained high in all groups with TA compared to the NC group (P < 0.05) and were significantly higher than in the SP group (P < 0.05).
Figure 3. Levels of serum immunity indexes in broiler chickens at the 5th day of infection. (A) Serum levels of IL-4. (B) Serum levels of IFN-γ; (C) Serum levels of IgG. All data are expressed as the mean ± SD. The results are presented as the mean ± SD. Different letters indicate significant differences between the groups (P < 0.05).
Histopathological analysis showed that infection with S. pullorum resulted in significant damage to the jejunal and ileal villi in the SP group. The presence of broken villi, thickened crypts, and a decrease in the number of villi in the SP group of chicks on days 1 and 5, when compared to the NC group (Figures 4A, B, D, E). The chicks in the TAL, TAM, and TAH groups had a higher presence of intact intestinal structures and villi when compared to the SP group. The results of further analysis indicate that the heights of both jejunal and ileal villi were significantly lower in the SP group than in the NC group on days 1 and 5 (P < 0.05). Supplementation with TA effectively restored the height of these villi to normal levels (Figures 4C, F).
Figure 4. Pathologic sections of the jejunum and ileum of broiler chickens. (A, B) Representative images of H.E staining shows pathology of the jejunum and ileum in different treatment groups on day 1 post infection. (C) Statistics of villus height and crypt depth contents on day 1 post infection. (D, E) Representative images of H.E staining shows pathology of the jejunum and ileum in different treatment groups on day 5 post infection (scale bar =100 μ m). (F) Statistics of villus height and crypt depth contents on day 5 post infection. All data are expressed as the mean ± SD. The results are presented as the mean § SEM. Different letters indicate significant differences between the groups (P < 0.05).
The PAS staining revealed that the goblet cells of the chick jejunum were stained red (Figures 5A, B). The SP group had fewer jejunal goblet cells than the NC group on both days 1 and 5 after S. pullorum infection (P < 0.05). On day 1, all TA groups had normal goblet cell numbers (P < 0.05). On day 5, all TA groups had more goblet cells than the SP group (P < 0.05), and there was no significant difference between the TAM group and the NC group (Figures 5C, D).
Figure 5. PAS staining of the jejunum. (A, C) PAS staining of the jejunum on days 1 and 5 post-infection (scale bar =100 μ m). (B, D) Statistics of goblet cells numbers of the jejunum in different groups on day 1 and day 5 post infection. All data are expressed as the mean ± SD. The results are presented as the mean ± SD. Different letters indicate significant differences between the groups (P < 0.05).
Figure 6 illustrates the impact of TA on intestinal tight junction proteins, mucin and permeability in broilers challenged with S. pullorum. On the day 1 of infection, the mRNA transcript levels of tight junction proteins (Claudin-1 and Occludin) and mucin (MUC-2) were significantly reduced in the jejunum and ileum of chicks in the SP group compared to the NC group. The expression of both tight junction proteins and mucins was markedly diminished on the fifth day of infection (P < 0.05). On the first and fifth days of infection, TA supplementation increased the mRNA transcript levels of tight junction proteins and mucins compared to the SP group. On the fifth day, the TAM group showed significantly higher levels of jejunal Occludin, ZO-1, and MUC2, as well as ileal ZO-1, compared to the NC group (P < 0.05). The TAL group showed lower levels of Claudin-1 and Occludin compared to the NC group. Figures 6A, B show that the PC and TAH groups had lower levels of Claudin-1 and higher levels of ileal ZO-1 and MUC2 compared to the NC group. Alterations in serum lipopolysaccharide (LPS) and diamine oxidase (DAO) levels were observed on the fifth day after infection. The SP group exhibited considerably elevated levels of LPS and DAO in comparison to the NC group (P < 0.05). TA can effectively reduce the blood levels of LPS and DAO in chicks, with the most significant effect observed in the TAM group. In the TAM group, serum levels of LPS and DAO returned to normal (Figures 6C, D). During the initial infection stage, IL-10 mRNA transcript levels significantly increased in the jejunum, while IFN-γ markedly decreased in both the jejunum and ileum of chicks in the SP group. The mRNA transcript levels of the cytokines IL-4, IL-18 and IFN-γ were found to be significantly higher in the TAM group than in the SP group (Figure 7A). On the fifth day of infection, the SP group showed significantly lower levels of IL-4, IL-10, IL-18, and IFN-γ in the jejunal and ileal regions compared to the NC group (P < 0.05). The cytokine levels in the PC, TAM, and TAH groups increased to varying degrees, except for the TAL group. The group subjected to TAM demonstrated the most significant promotion impact, as illustrated in Figure 7B. Secretory IgA (sIgA) is crucial for establishing and maintaining the immune barrier. On the fifth day after infection, we observed a 28.29% decrease in the level of sIgA in the jejunal tissue of the SP group compared to the NC group (P < 0.05). After the addition of tannic acid, the levels of jejunal sIgA in broilers were significantly higher than in the SP group. Only the TAH group showed a significant decrease compared to the NC group (Figure 7C).
Figure 6. Relative expression levels from qRT-PCR and serum ELISA. (A) Expression of mRNA for Claudin-1, Occluding, ZO-1 and MUC-2 in the jejunum and ileum of chicks on day 1 post-infection (n = 4). (B) Expression of mRNA for Claudin-1, Occludin, ZO-1 and MUC-2 in the jejunum and ileum of chicks on day 1 post-infection (n = 4). (C) LPS levels in serum on the day 5 of infection. (D) DAO levels in serum on the day 5 of infection. All data are expressed as the mean ± SD. The results are presented as the mean § SEM. Different letters indicate significant differences between the groups (P < 0.05).
Figure 7. Relative expression levels from qRT-PCR and jejunum ELISA. (A) Expression of mRNA for IL-4, IL-10, IL-18 and IFN-γ in the jejunum and ileum of chicks on day 1 post-infection (n = 4). (B) Expression of mRNA for IL-4, IL-10, IL-18 and IFN-γ in the jejunum and ileum of chicks on day 5 post-infection (n = 4). (C) Levels of sIgA in jejunum on day 5 of infection (n = 5). All data are expressed as the mean ± SD. The results are presented as the mean ± SD. Different letters indicate significant differences between the groups (P < 0.05).
The aim of this study was to investigate the impact of S. pullorum infection and TA treatment on the cecal microbiota of chicks. To achieve this, we sequenced the 16S rRNA gene in samples from four groups: NC, SP, PC, and TAM. Figure 8A shows the rarefaction curves of the samples, indicating adequate sequencing depth and variations in species richness between the groups. The results of the Venn diagram show that there were 689, 537, 707, and 234 unique Operational Taxonomic Units (OTUs) in each respective group. The number of observed OTUs in each group were 2,879, 2,572, 2,456, and 1,654, respectively (Figure 8B). The microbiota's richness and diversity were measured using the Chao1 and Shannon indices. After infection with S. pullorum, all groups showed a decrease in Chao1 indices compared to the NC. But only the TAM treatment group showed a statistically significant difference (P < 0.05; Figure 8C). The Shannon index did not exhibit any significant differences among the groups (Figure 8D). The UPGMA clustering analysis revealed that PC formed a distinct cluster, whereas NC, SP, and TAM were grouped together on a single branch. It is worth noting that NC and TAM exhibited a closer relationship, as depicted in Figure 8E. The cecal microbiota of chicks from four groups (NC, SP, PC, and TAM) were analyzed at the phylum, genus, and species levels. The main phyla observed in all groups were Firmicutes, Bacteroidetes, Actinobacteria, and Proteobacteria (Figure 8F). The Actinobacteria, though, were only abundant in NC (10.99%) and decreased significantly in SP (2.09%, P < 0.05), PC (0.54%, P < 0.05), and TAM (1.87%, P < 0.05). Additionally, SP had a significantly higher abundance of Campylobacter (11.57%, P < 0.05) than NC (0.46%). The genus Bacteroides was dominant in all groups (Figure 8G). Bifidobacterium was significantly lower in SP (1.84%, P < 0.05), PC (0.19%, P < 0.05), and TAM (1.40%, P < 0.05) compared to NC (10.40%). In contrast, Campylobacter was significantly higher in SP (11.56%, P < 0.05) than in NC (0.46%). Escherichia-Shigella was more abundant in SP (12.50%) and PC (12.85%) than in TAM (3.00%, P < 0.05). The abundance of Ruminococcus torques group was higher in the PC (6.06%) and TAM (8.23%) compared to the NC (2.28%), but the difference was not statistically significant. At the species level (Figure 8H), Bacteroides fragilis (20.43%) and Bacteroides uniformis (14.17%) were the most abundant species in NC, while Bacteroides uniformis (22.14%) prevailed in SP, and Bacteroides fragilis (35.43%) prevailed in TAM. At the genus level, there was a significant increase in Campylobacter_jejuni (11.56%, P < 0.05) in SP compared to NC (0.49%). Conversely, TAM had significantly lower levels of both Campylobacter_jejuni (0.21%, P < 0.05) and Escherichia coli (3.0%, P < 0.05) compared to NC. Linear discriminant analysis (LDA) was used to identify cecal microbial taxa that displays significant differences among the four groups: NC, SP, PC, and TAM. Figure 8I shows the taxa with LDA scores >4, indicating significant differences. All groups exhibited a decrease in Bifidobacterium abundance at the genus level following S. pullorum infection compared to NC. Additionally, the SP group showed a higher abundance of Campylobacter. The abundance of Erysipelatoclostridium, Butyricicoccus, and Lactobacillus was higher in PC, while Bacteroides was more abundant in the TAM group. To visualize the differences in taxa abundance, a heatmap was generated using the top 20 genera by abundance. The SP group had 11 genera that differed from the NC group, the PC group had 18, and the TAM group had 8 (Figure 8J).
Figure 8. Microbiota of broiler cecum on the 5th day of infection. (A) Rarefaction curves of OTUs. (B) Venn diagram. (C, D) Alpha diversity comparison (Chao1 index and Shannon index). (E) UPGMA clustering tree based on weighted Unifrac distances at the phylum level. Relative abundance of species in the top 10 of the intestinal flora at the phylum (F), genus (G) and species level (H). (I) The histogram of the distribution of LDA values (LDA scores >4). (J) Heatmap depicting the relative abundance of 35 dominant microbiota genera at the genus level. All data are expressed as the mean ± SD. The results are presented as the mean ± SD. “*” stands for the comparison with NC; *P < 0.05 by one-way ANOVA.
The gut mucosa serves as the primary defensive barrier against pathogenic microorganisms (22, 23), playing a crucial role in preventing bacterial infections. Infections caused by S. pullorum to significantly disturb the structure and function of gut morphology, leading to considerable mortality rates in poultry (24–26). This issue has caused significant economic losses in the poultry farming industry in developing countries. The study aims to evaluate the protective effects of TA on the integrity and functionality of the intestinal barrier after S. pullorum infection in broiler chickens, given the well-documented antimicrobial properties of TA and their potential to modulate the composition of the intestinal microbiota. The immune response of broiler chickens relies on the thymus, spleen, and bursa (27, 28) and the relative weights of these organs can indicate immune function in chickens (29). The study found that infection with S. pullorum resulted in a decrease in the weight of the thymus and bursa. In contrast, treatment with TA, preserved the normal development of these organs and also resulted in an increase in spleen weight. These findings are consistent with the results reported by Chen et al. (26), suggesting that TA can enhance the immune function and disease resistance of chicks. Previous studies have also reported a protective effect of TA on the liver (30). The study found that the administration of TA significantly reduced the colonization of S. pullorum and mitigated liver damage (31).
The integrity and height of the intestinal villus reflect gut integrity and are fundamental to the gut's barrier function (5, 32). Previous studies have reported that the invasion of S. pullorum into chick intestines disrupts the intestinal barrier function (26, 33). Our study confirms these findings, as the intestinal villi of chicks in the SP group were fragmented and shortened. By contrast, the three TA-supplemented groups showed a marked improvement in the morphological structure of the intestine, with the TAM group showing the most improvement. Serum concentrations of lipopolysaccharide (LPS) and diamine oxidase (DAO) are important indicators of intestinal permeability. As previously found, TA significantly reduced serum LPS and DAO levels, indicating its potential to reduce intestinal permeability in chicks. These results suggest that TA helps to preserve the structural integrity of the intestines and mitigate damage caused by S. pullorum. The intestinal epithelium is composed of different cell types with different functions: goblet cells, for example, secrete mucus and form a barrier to pathogenic microorganisms; these cells are connected by tight junctions (34–36). The invasion of S. pullorum disrupts the mucus layer and facilitates the spread of harmful substances (37–39). The protective effect of TA on the intestinal barrier function of broiler chickens infected with S. pullorum was evaluated in this study based on the antimicrobial properties of TA and the regulation of tight junction proteins (13, 20). The gene expression of intestinal claudin-1, occludin, ZO-1, and MUC2, as well as the number of goblet cells, were found to be significantly reduced as a result of S. pullorum infection. TA supplementation improved the goblet cell count and increased the expression of tight junction proteins and MUC2, with the most significant effect observed in the TAM group. S. pullorum is a facultative intracellular parasitic bacterium that primarily induces Th2-type immune responses, which may contribute to its persistence and infection (40–42). Secretory immunoglobulin A (sIgA) is a crucial marker of intestinal immunity (43, 44). Supplementation with TA enhanced the mRNA expression of Th1 and Th2 cytokines and increased jejunal sIgA secretion. These findings suggest that TA improves the intestinal mucosal barrier function and antimicrobial capacity. The composition of intestinal flora is closely linked to the function of the intestinal barrier and plays a crucial role in the host's defense against pathogenic microorganisms and energy provision (45, 46). Previous studies have indicated that S. pullorum invasion disrupts gut flora, although the exact effects remain to be elucidated (33, 47, 48). Our study confirms these findings, demonstrating a significant decrease in gut microbial diversity in chicks infected with S. pullorum. Notably, the addition of TA supplementation did not significantly increase microbial diversity, but it did align more closely with the NC group in terms of evolutionary levels. This may be due to the selective bacteriostatic effect of TA. To further analyze the dominant flora at different levels, changes at the phylum level were investigated. The study found a significant increase in the relative abundance of Proteobacteria in the SP group of chicks, which is often associated with many diseases (49, 50). Furthermore, the pathogen Campylobacter, a which is known to harm the gut mucosa and increase gut permeability, was identified as particularly harmful to humans (51, 52). The study conducted on SP group broilers revealed a significant increase in the relative abundance of both Campylobacter and Escherichia–Shigella, which is consistent with the findings of Huang et al. (47). These results suggest that S. pullorum disrupts the gut flora and promotes the growth of harmful bacteria. Supplementation with TA, resulted in a significant decrease in the relative abundance of Campylobacter and a significant increase in Bacteroides fragilis. Bacteroides fragilis has potential as a probiotic, as it enhances macrophage phagocytosis and promotes a Th1-type immune response (53). This may further inhibit S. pullorum enterica colonization in chickens with dysentery. The results of the heat map indicate that Lactobacillus (54), Ligilactobacillus (55), and Limosilactobacillus (56), which are closely related to gut homeostasis, were enriched in the TAM group. This suggests that TA can effectively prevent the colonization of harmful bacteria in the intestine, promote the growth of beneficial microorganisms, enhance immune function, and maintain the structural and functional integrity of the intestine.
In conclusion, our study provides evidence that TA supplementation can effectively mitigate S. pullorum colonization, reduce chick mortality and improve body weight. These effects are mainly mediated by upregulation of genes associated with gut barrier function and modulation of the gut microbiota. Our findings provide a novel approach for the clinical management of PD.
The original contributions presented in the study are publicly available. This data can be found at the National Center for Biotechnology Information (NCBI) using accession number PRJNA1108228.
The animal study was approved by the Ethics Committee of Sichuan Agricultural University. The study was conducted in accordance with the local legislation and institutional requirements.
JZ: Conceptualization, Data curation, Formal analysis, Investigation, Methodology, Resources, Validation, Visualization, Writing – original draft, Writing – review & editing. HL: Conceptualization, Data curation, Formal analysis, Investigation, Methodology, Resources, Validation, Visualization, Writing – original draft, Writing – review & editing. PX: Conceptualization, Data curation, Formal analysis, Investigation, Methodology, Resources, Validation, Visualization, Writing – original draft, Writing – review & editing. JX: Formal analysis, Investigation, Methodology, Visualization, Writing – original draft, Writing – review & editing. JF: Data curation, Formal analysis, Validation, Visualization, Writing – original draft, Writing – review & editing. XZhon: Data curation, Formal analysis, Validation, Visualization, Writing – original draft, Writing – review & editing. XZhou: Validation, Visualization, Writing – original draft, Writing – review & editing. XS: Formal analysis, Investigation, Supervision, Writing – original draft, Writing – review & editing. XZha: Validation, Visualization, Writing – original draft, Writing – review & editing. YZ: Validation, Visualization, Writing – original draft, Writing – review & editing. LL: Validation, Visualization, Writing – original draft, Writing – review & editing. RJ: Validation, Visualization, Writing – original draft, Writing – review & editing. YF: Validation, Visualization, Writing – original draft, Writing – review & editing. ZL: Validation, Visualization, Writing – original draft, Writing – review & editing. ZY: Conceptualization, Formal analysis, Funding acquisition, Investigation, Methodology, Project administration, Resources, Supervision, Writing – original draft, Writing – review & editing.
The author(s) declare financial support was received for the research, authorship, and/or publication of this article. This research was supported by the Program Sichuan Veterinary Medicine and Drug Innovation Group of China Agricultural Research System (SCCXTD-2020-18), the Science and Technology Project of Sichuan Province (2021NZZJ0021), Application and Industrialization Plan of Scientific and Technological Achievements of Guizhou Province (202221481317030275), and the Natural Science Foundation of Sichuan Province (24GJHZ0440).
We are grateful to all the staff of the Natural Medicines Research Center of Sichuan Agricultural University for their assistance in this study.
HL was employed by Qilu Animal Health Products Co., Ltd. ZL was employed by Chengdu QianKun Veterinary Pharmaceutical Co., Ltd.
The remaining authors declare that the research was conducted in the absence of any commercial or financial relationships that could be construed as a potential conflict of interest.
All claims expressed in this article are solely those of the authors and do not necessarily represent those of their affiliated organizations, or those of the publisher, the editors and the reviewers. Any product that may be evaluated in this article, or claim that may be made by its manufacturer, is not guaranteed or endorsed by the publisher.
1. Shivaprasad HL. Fowl typhoid and pullorum disease. Rev. Sci. Tech. (2000) 19:405–24. doi: 10.20506/rst.19.2.1222
2. Thøfner I, Christensen J. Bacterial Diseases in Poultry. Amsterdam: Elsevier (2021), p. 199–227. doi: 10.1016/B978-0-12-818030-3.00005-2
3. Xu L, Liu Z, Li Y, Yin C, Hu Y, Xie X, et al. A rapid method to identify Salmonella enterica serovar Gallinarum biovar Pullorum using a specific target gene ipaJ. Avian Pathol. (2018) 47:238–44. doi: 10.1080/03079457.2017.1412084
4. Wu QJ, Jiao C, Liu ZH, Li SW, Zhu DD, Ma WF, et al. Effect of glutamine on the intestinal function and health of broilers challenged with Salmonella pullorum. Indian J Anim Res. (2019) 53:1210–6. doi: 10.18805/ijar.B-1133
5. Wang Y, Yan X, Han D, Liu Y, Song W, Tong T, et al. Lactobacillus casei DBN023 protects against jejunal mucosal injury in chicks infected with Salmonella pullorum CMCC-533. Res Vet Sci. (2019) 127:33–41. doi: 10.1016/j.rvsc.2019.09.010
6. Chen C, Li J, Zhang H, Xie Y, Xiong L, Liu H, et al. Effects of a probiotic on the growth performance, intestinal flora, and immune function of chicks infected with Salmonella pullorum. Poult Sci. (2020) 99:5316–23. doi: 10.1016/j.psj.2020.07.017
7. Sørum H, Sunde M. Resistance to antibiotics in the normal flora of animals. Vet Res. (2001) 32:227–41. doi: 10.1051/vetres:2001121
8. Penha Filho RAC, Ferreira JC, Kanashiro AMI, Darini ALDC, Berchieri Junior A. Antimicrobial susceptibility of Salmonella gallinarum and Salmonella pullorum isolated from ill poultry in Brazil. Ciência Rural. (2016) 46:513–18. doi: 10.1590/0103-8478cr20150398
9. Gaudin V. Advances in biosensor development for the screening of antibiotic residues in food products of animal origin–a comprehensive review. Biosens Bioelectron. (2017) 90:363–77. doi: 10.1016/j.bios.2016.12.005
10. Khanbabaee K, Van Ree T. Tannins: classification and definition. Nat Prod Rep. (2001) 18:641–49. doi: 10.1039/b101061l
11. Jing W, Xiaolan C, Yu C, Feng Q, Haifeng Y. Pharmacological effects and mechanisms of tannic acid. Biomed Pharmacother. (2022) 154:113561. doi: 10.1016/j.biopha.2022.113561
12. Mazmanian SK, Liu CH, Tzianabos AO, Kasper DL. An immunomodulatory molecule of symbiotic bacteria directs maturation of the host immune system. Cell. (2005) 122:107–18. doi: 10.1016/j.cell.2005.05.007
13. Choi J, Marshall B, Ko H, Shi H, Singh AK, Thippareddi H, et al. Antimicrobial and immunomodulatory effects of tannic acid supplementation in broilers infected with Salmonella Typhimurium. Poult Sci. (2022) 101:102111. doi: 10.1016/j.psj.2022.102111
14. Liu S, Wang K, Lin S, Zhang Z, Cheng M, Hu S, et al. Comparison of the effects between tannins extracted from different natural plants on growth performance, antioxidant capacity, immunity, and intestinal flora of broiler chickens. Antioxidants. (2023) 12:441. doi: 10.3390/antiox12020441
15. EFSA POAA. Scientific opinion on the safety and efficacy of tannic acid when used as feed flavouring for all animal species. EFSA J. (2014) 12:3828. doi: 10.2903/j.efsa.2014.3828
16. Tosi G, Massi P, Antongiovanni M, Buccioni A, Minieri S, Marenchino L, et al. Efficacy test of a hydrolysable tannin extract against necrotic enteritis in challenged broiler chickens. Ital J Anim Sci. (2013) 12:e62. doi: 10.4081/ijas.2013.e62
17. Zhang ZF Xi Y, Wang ST, Zheng LY, Qi Y, Guo SS, et al. Effects of Chinese gallnut tannic acid on growth performance, blood parameters, antioxidative status, intestinal histomorphology, and cecal microbial shedding in broilers challenged with aflatoxin B1. J Anim Sci. (2022) 100:c99. doi: 10.1093/jas/skac099
18. Starčević K, Krstulović L, Brozić D, Maurić M, Stojević Z, Mikulec Z, et al. Production performance, meat composition and oxidative susceptibility in broiler chicken fed with different phenolic compounds. J Sci Food Agric. (2015) 95:1172–78. doi: 10.1002/jsfa.6805
19. Niu J, Wang Q, Jing C, Liu Y, Liu H, Jiao N, et al. Dietary Galla chinensis tannic acid supplementation in the diets improves growth performance, immune function and liver health status of broiler chicken. Front Vet Sci. (2022) 9:1024430. doi: 10.3389/fvets.2022.1024430
20. Xu H, Fu J, Luo Y, Li P, Song B, Lv Z, et al. Effects of tannic acid on the immunity and intestinal health of broiler chickens with necrotic enteritis infection. J Anim Sci Biotechnol. (2023) 14:1–20. doi: 10.1186/s40104-023-00867-8
21. Brus M, Gradišnik L, Trapečar M, Škorjanc D, FrangeŽ R. Beneficial effects of water-soluble chestnut (Castanea sativa Mill) tannin extract on chicken small intestinal epithelial cell culture. Poult Sci. (2018) 97:1271–82. doi: 10.3382/ps/pex424
22. Kim M, Ashida H, Ogawa M, Yoshikawa Y, Mimuro H, Sasakawa C. Bacterial interactions with the host epithelium. Cell Host Microbe. (2010) 8:20–35. doi: 10.1016/j.chom.2010.06.006
23. Martens EC, Neumann M, Desai MS. Interactions of commensal and pathogenic microorganisms with the intestinal mucosal barrier. Nat Rev Microbiol. (2018) 16:457–70. doi: 10.1038/s41579-018-0036-x
24. Chalghoumi R, Marcq C, Thewis A, Portetelle D, Beckers Y. Effects of feed supplementation with specific hen egg yolk antibody (immunoglobin Y) on Salmonella species cecal colonization and growth performances of challenged broiler chickens. Poult Sci. (2009) 88:2081–92. doi: 10.3382/ps.2009-00173
25. Wu QJ, Zheng XC, Wang T, Zhang TY. Effect of dietary oridonin supplementation on growth performance, gut health, and immune response of broilers infected with Salmonella pullorum. Ir Vet J. (2018) 71:1–06. doi: 10.1186/s13620-018-0128-y
26. Chen F, Zhang H, Du E, Fan Q, Zhao N, Jin F, et al. Supplemental magnolol or honokiol attenuates adverse effects in broilers infected with Salmonella pullorum by modulating mucosal gene expression and the gut microbiota. J Anim Sci Biotechnol. (2021) 12:1–15. doi: 10.1186/s40104-021-00611-0
27. Song B, Tang D, Yan S, Fan H, Li G, Shahid MS, et al. Effects of age on immune function in broiler chickens. J Anim Sci Biotechnol. (2021) 12:1–12. doi: 10.1186/s40104-021-00559-1
28. Hu W, Zhang W, Shah SWA, Ishfaq M, Li J. Mycoplasma gallisepticum infection triggered histopathological changes, oxidative stress and apoptosis in chicken thymus and spleen. Dev Comp Immunol. (2021) 114:103832. doi: 10.1016/j.dci.2020.103832
29. Rivas AL, Fabricant J. Indications of immunodepression in chickens infected with various strains of Marek's disease virus. Avian Dis. (1988) 32:1–8. doi: 10.2307/1590941
30. Yuan P, Xu H, Ma Y, Niu J, Liu Y, Huang L, et al. Effects of dietary Galla chinensis tannin supplementation on immune function and liver health in broiler chickens challenged with lipopolysaccharide. Front Vet Sci. (2023) 10:1126911. doi: 10.3389/fvets.2023.1126911
31. Wang J, Yao L, Su J, Fan R, Zheng J, Han Y. Effects of Lactobacillus plantarum and its fermentation products on growth performance, immune function, intestinal pH, and cecal microorganisms of Lingnan yellow chicken. Poult Sci. (2023) 102:102610. doi: 10.1016/j.psj.2023.102610
32. Elnesr SS, Alagawany M, Elwan HA, Fathi MA, Farag MR. Effect of sodium butyrate on intestinal health of poultry–a review. Ann Anim Sci. (2020) 20:29–41. doi: 10.2478/aoas-2019-0077
33. Deng Z, Han D, Wang Y, Wang Q, Yan X, Wang S, et al. Lactobacillus casei protects intestinal mucosa from damage in chicks caused by Salmonella pullorum via regulating immunity and the Wnt signaling pathway and maintaining the abundance of gut microbiota. Poult Sci. (2021) 100:101283. doi: 10.1016/j.psj.2021.101283
34. Ulluwishewa D, Anderson RC, McNabb WC, Moughan PJ, Wells JM, Roy NC. Regulation of tight junction permeability by intestinal bacteria and dietary components. J Nutr. (2011) 141:769–76. doi: 10.3945/jn.110.135657
35. Gerbe F, Legraverend C, Jay P. The intestinal epithelium tuft cells: specification and function. Cell Mol Life Sci. (2012) 69:2907–17. doi: 10.1007/s00018-012-0984-7
36. Capaldo CT, Powell DN, Kalman D. Layered defense: how mucus and tight junctions seal the intestinal barrier. J Mol Med. (2017) 95:927–34. doi: 10.1007/s00109-017-1557-x
37. Feng L, Chen S, Zhang L, Qu W, Chen Z. Bisphenol A increases intestinal permeability through disrupting intestinal barrier function in mice. Environ Pollut. (2019) 254:112960. doi: 10.1016/j.envpol.2019.112960
38. Zhu L, Lu X, Liu L, Voglmeir J, Zhong X, Yu Q. Akkermansia muciniphila protects intestinal mucosa from damage caused by S. pullorum by initiating proliferation of intestinal epithelium. Vet Res. (2020) 51:1–09. doi: 10.1186/s13567-020-00755-3
39. Chen X, Ishfaq M, Wang J. Effects of Lactobacillus salivarius supplementation on the growth performance, liver function, meat quality, immune responses and Salmonella pullorum infection resistance of broilers challenged with Aflatoxin B1. Poult Sci. (2022) 101:101651. doi: 10.1016/j.psj.2021.101651
40. Pinaud L, Sansonetti PJ, Phalipon A. Host cell targeting by enteropathogenic bacteria T3SS effectors. Trends Microbiol. (2018) 26:266–83. doi: 10.1016/j.tim.2018.01.010
41. Xian H, Yuan Y, Yin C, Wang Z, Ji R, Chu C, et al. The SPI-19 encoded T6SS is required for Salmonella pullorum survival within avian macrophages and initial colonization in chicken dependent on inhibition of host immune response. Vet Microbiol. (2020) 250:108867. doi: 10.1016/j.vetmic.2020.108867
42. Liu Z, Wang L, Gao P, Yu Y, Zhang Y, Fotin A, et al. Salmonella pullorum effector SteE regulates Th1/Th2 cytokine expression by triggering the STAT3/SOCS3 pathway that suppresses NF-κB activation. Vet Microbiol. (2023) 248:109817. doi: 10.1016/j.vetmic.2023.109817
43. Liu SQ, Wang LY, Liu GH, Tang DZ, Fan XX, Zhao JP, et al. Leucine alters immunoglobulin a secretion and inflammatory cytokine expression induced by lipopolysaccharide via the nuclear factor-κB pathway in intestine of chicken embryos. Animal. (2018) 12:1903–11. doi: 10.1017/S1751731117003342
44. Wang Y, Li J, Xie Y, Zhang H, Jin J, Xiong L, et al. Effects of a probiotic-fermented herbal blend on the growth performance, intestinal flora and immune function of chicks infected with Salmonella pullorum. Poult Sci. (2021) 100:101196. doi: 10.1016/j.psj.2021.101196
45. Buffie CG, Pamer EG. Microbiota-mediated colonization resistance against intestinal pathogens. Nat Rev Immunol. (2013) 13:790–801. doi: 10.1038/nri3535
46. Ramakrishna BS. Role of the gut microbiota in human nutrition and metabolism. J Gastroenterol Hepatol. (2013) 28:9–17. doi: 10.1111/jgh.12294
47. Huang J, Liang L, Cui K, Li P, Hao G, Sun S. Salmonella phage CKT1 significantly relieves the body weight loss of chicks by normalizing the abnormal intestinal microbiome caused by hypervirulent Salmonella pullorum. Poult Sci. (2022) 101:101668. doi: 10.1016/j.psj.2021.101668
48. Xu Y, Wang Q, Dong M, Song H, Hang B, Sun Y, et al. Evaluation of the efficacy of the antimicrobial peptide HJH-3 in chickens infected with Salmonella pullorum. Front Microbiol. (2023) 14:1102789. doi: 10.3389/fmicb.2023.1102789
49. Mukhopadhya I, Hansen R, El-Omar EM, Hold GL. IBD—what role do proteobacteria play? Nat Rev Gastroenterol Hepatol. (2012) 9:219–30. doi: 10.1038/nrgastro.2012.14
50. Rizzatti G, Lopetuso LR, Gibiino G, Binda C, Gasbarrini A. Proteobacteria: a common factor in human diseases. Biomed Res Int. (2017) 2017:9351507. doi: 10.1155/2017/9351507
51. Van Deun K, Pasmans F, Ducatelle R, Flahou B, Vissenberg K, Martel A, et al. Colonization strategy of Campylobacter jejuni results in persistent infection of the chicken gut. Vet Microbiol. (2008) 130:285–97. doi: 10.1016/j.vetmic.2007.11.027
52. Awad WA, Hess C, Hess M. Re-thinking the chicken–Campylobacter jejuni interaction: a review. Avian Pathol. (2018) 47:352–63. doi: 10.1080/03079457.2018.1475724
53. Deng H, Li Z, Tan Y, Guo Z, Liu Y, Wang Y, et al. A novel strain of Bacteroides fragilis enhances phagocytosis and polarises M1 macrophages. Sci Rep. (2016) 6:29401. doi: 10.1038/srep29401
54. Mu Q, Tavella VJ, Luo XM. Role of Lactobacillus reuteri in human health and diseases. Front Microbiol. (2018) 9:757. doi: 10.3389/fmicb.2018.00757
55. Fei Y, Zhang S, Han S, Qiu B, Lu Y, Huang W, et al. The role of dihydroresveratrol in enhancing the synergistic effect of Ligilactobacillus salivarius Li01 and resveratrol in ameliorating colitis in mice. Research. (2022) 2022:9863845. doi: 10.34133/2022/9863845
56. Paulino Do Nascimento LC, Lacerda DC, Ferreira DJS, de Souza EL, de Brito Alves JL. Limosilactobacillus fermentum, current evidence on the antioxidant properties and opportunities to be exploited as a probiotic microorganism. Probiotics Antimicrob Proteins. (2022) 14:960–79. doi: 10.1007/s12602-022-09943-3
Keywords: Salmonella pullorum, pullorum disease, tannic acid, gut barrier, intestinal flora
Citation: Zou J, Luan H, Xi P, Xue J, Fan J, Zhong X, Zhou X, Song X, Zhao X, Zou Y, Li L, Jia R, Fu Y, Liu Z and Yin Z (2024) Gallnut tannic acid alleviates gut damage induced by Salmonella pullorum in broilers by enhancing barrier function and modulating microbiota. Front. Vet. Sci. 11:1382288. doi: 10.3389/fvets.2024.1382288
Received: 05 February 2024; Accepted: 30 April 2024;
Published: 28 May 2024.
Edited by:
Ilias Giannenas, Aristotle University of Thessaloniki, GreeceReviewed by:
Alireza Seidavi, Islamic Azad University, Rasht Branch, IranCopyright © 2024 Zou, Luan, Xi, Xue, Fan, Zhong, Zhou, Song, Zhao, Zou, Li, Jia, Fu, Liu and Yin. This is an open-access article distributed under the terms of the Creative Commons Attribution License (CC BY). The use, distribution or reproduction in other forums is permitted, provided the original author(s) and the copyright owner(s) are credited and that the original publication in this journal is cited, in accordance with accepted academic practice. No use, distribution or reproduction is permitted which does not comply with these terms.
*Correspondence: Zhongqiong Yin, eWluemhvbmdxQDE2My5jb20=
†These authors have contributed equally to this work
Disclaimer: All claims expressed in this article are solely those of the authors and do not necessarily represent those of their affiliated organizations, or those of the publisher, the editors and the reviewers. Any product that may be evaluated in this article or claim that may be made by its manufacturer is not guaranteed or endorsed by the publisher.
Research integrity at Frontiers
Learn more about the work of our research integrity team to safeguard the quality of each article we publish.