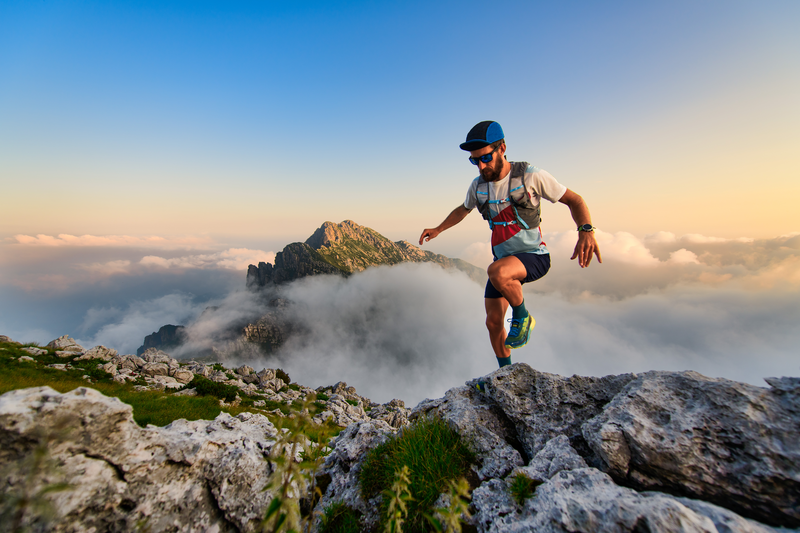
94% of researchers rate our articles as excellent or good
Learn more about the work of our research integrity team to safeguard the quality of each article we publish.
Find out more
ORIGINAL RESEARCH article
Front. Vet. Sci. , 04 January 2024
Sec. Veterinary Infectious Diseases
Volume 10 - 2023 | https://doi.org/10.3389/fvets.2023.1272595
This article is part of the Research Topic Antimicrobials Alternatives for the Prevention and Treatment of Veterinary Infectious Diseases: Volume II View all 9 articles
Staphylococcus coagulans (SC) belongs to a group of coagulase-positive staphylococci occasionally isolated from the skin lesions of dogs with pyoderma. We recently revealed that erythritol, a sugar alcohol, inhibited the growth of SC strain JCM7470. This study investigated the molecular mechanisms involved in this growth inhibition of JCM7470 by erythritol, and determine whether erythritol inhibits the growth of SC isolated from the skin of dogs with pyoderma. Comprehensive analysis of the gene expression of JCM7470 in the presence of erythritol revealed that erythritol upregulated the expression of glcB and ptsG genes, both of which encode phosphotransferase system (PTS) glucoside- and glucose-specific permease C, B, and A domains (EIICBA), respectively, associated with sugar uptake. Moreover, erythritol suppressed in vitro growth of all 27 SC strains isolated from the skin lesions of canine pyoderma, including 13 mecA gene-positive and 14 mecA gene-negative strains. Finally, the growth inhibition of the SC clinical isolates by erythritol was restored by the addition of glucose. In summary, we revealed that erythritol promotes PTS gene expression and suppresses the in vitro growth of SC clinical isolates from dogs with pyoderma. Restoration of the erythritol-induced growth inhibition by glucose suggested that glucose starvation may contribute to the growth inhibition of SC.
Staphylococcus coagulans (SC), formerly classified as S. schleiferi subsp. coagulans, is a coagulase-positive staphylococcus primarily isolated from the skin and ear canals of dogs (1–6). Among staphylococci, SC is the second most prevalent cause of skin lesion in dogs with pyoderma after Staphylococcus pseudintermedius (SP) (7). In addition to infections in dogs, SC has been reported to cause opportunistic infections in humans (8, 9). Similar to findings in other staphylococcal species (10–13), the emergence of methicillin- and multidrug-resistant SC has been reported (14–17), representing a problem for human and veterinary medicine. Against this background, the development of novel strategies for preventing canine pyoderma using bacteriostatic substrates is anticipated.
Erythritol (1,2,3,4-butanetetrol) is a polyol that is widely used in foods as an artificial sweetener (18). Both erythritol and xylitol, another type of polyol, were shown to inhibit the growth of Streptococcus mutans, Streptococcus gordonii, and Porphyromonas gingivalis, major human oral commensal bacteria (18–20). Recently, studies have also reported that erythritol inhibited the growth of the human skin commensal bacteria Corynebacterium minutissimum, Corynebacterium striatum, Staphylococcus epidermidis, and Cutibacterium acnes, as well as the major canine oral commensal bacteria Porphyromonas gulae and Porphyromonas macacae (21–23).
The mechanisms by which xylitol inhibits S. mutans have been well studied (24). S. mutans incorporates xylitol via the phosphoenolpyruvate-dependent phosphotransferase system (PEP-PTS) and phosphorylates it to xylitol 5-phosphate. The xylitol 5-phosphate accumulated in the bacteria directly inhibits enzymatic activity related to glycolysis and competes with the phosphor-heat stable protein (24) to indirectly inhibit sugar uptake. Such mechanisms may result in glucose starvation in S. mutans and the inhibition of bacterial growth.
Very recently, we revealed that erythritol suppressed in vitro growth of the SP and SC strains JCM17571 and JCM7470, respectively (25). Furthermore, erythritol upregulated PEP-PTS-related genes (ptsG, ppdK, and ppdkR) in SP JCM17571 (26). However, the exact molecular mechanism by which erythritol suppresses the growth of SC has not been elucidated. In this study, we aimed to identify the SC gene clusters whose expression was altered by erythritol. Moreover, we investigated whether erythritol suppresses the growth of SC clinical isolates in vitro.
SC strain JCM7470 (identical to ATCC 49545) was provided by the Japan Collection of Microorganisms (JCM) and used as a reference strain (2). A previous study revealed that this strain was susceptible to oxacillin and cefoxitin by disk-diffusion tests (27). A total of 27 SC skin isolates from 27 dogs with pyoderma in different private practices and submitted to Vet Derm Tokyo Co., Ltd., for antibiotic susceptibility testing were also used as clinical isolates. The identification of SC was confirmed as follows: The DNA extracted from the 27 bacterial strains was subjected to multiplex PCR for identification of coagulase-positive staphylococcal strains (28). If the band size of the amplicons was identical to that in S. schleiferi, the strains were further subjected to a coagulase test using rabbit plasma (Eiken Chemical Co. Ltd., Tokyo, Japan) to identify S. schleiferi to the subspecies level (28). The mecA gene in the SC clinical isolates was identified by PCR with primer pairs used to identify this gene in S. aureus, SP, and S. schleiferi isolated from dogs (29). The antimicrobial susceptibility testing was performed by a disk diffusion test using KB Disk™ (Eiken Chemical Co. Ltd.), as described previously (30). The following antimicrobials were used for the susceptibility testing: amoxicillin-clavulanate (AMPC/CVA; 20 or 10 μg/disk), cephalexin (CEX; 30 μg/disk), cefpodoxime (CPDX; 10 μg/disk), enrofloxacin (ERFX; 5 μg/disk), gentamicin (GM; 10 μg/disk), sulfamethoxazole-trimethoprim (ST; 23.75–1.25 μg/disk), clindamycin (CLDM; 2 μg/disk), doxycycline (DOXY; 30 μg/disk), minocycline (MINO; 30 μg/disk), chloramphenicol (CP; 30 μg/disk), and fosfomycin (FOM; 50 μg/disk). Supplementary Table 1 lists the PCR primers used in this study, and Supplementary Table 2 shows the results of the disk diffusion susceptibility tests.
The following experiments were performed in accordance with the methodology used in a previous study (26). A single colony of JCM7470 was inoculated into 3 ml of National Institute of Technology and Evaluation Biological Resource Center (NRBC) #802 medium containing 1% hipolypepton (Fujifilm Wako, Osaka, Japan), 0.2% yeast extract (Nacalai Tesque Inc., Kyoto, Japan), and 0.1% MgSO4·7H2O (Fujifilm Wako, pH 7.0), and incubated with rotation at 210 rpm until the optical density at 600 nm (OD600) reached 3.4. The bacterial suspensions were further diluted 100-fold in NRBC #802 medium with or without 5% (w/w) erythritol (B Food Sciences Co. Ltd., Tokyo, Japan) and incubated at 30°C until OD600 reached 0.8–1.0. These experiments were performed in triplicate. We chose an erythritol concentration of 5% in this study as we had observed that erythritol at higher concentrations significantly inhibited the growth of JCM7470 in a previous study (25).
RNeasy Mini Kit (Qiagen, Venlo, Netherlands) was used to extract total RNA from the bacterial samples. The total RNA samples were submitted to Bioengineering Lab (Sagamihara, Japan). After removal of ribosomal RNA using riboPOOLS (siTOOLs Biotech, Planegg, Germany), a cDNA library for RNA-seq analysis was generated using MGIEasy RNA Directional Library Prep Set (MGI Tech, Shenzhen, China). The cDNA library was used to construct a circular DNA library using the MGIEasy Circularization Kit (MGI Tech). The cDNA library anchored by DNA Nanoball (DNA) was subjected to sequencing analysis using DNBSEC-G400 (MGI Tech). Nucleic Acid SeQuence Analysis Resource (NASQAR; https://nasqar.abudhabi.nyu.edu) was used for creating principal component analysis (PCA) plots of the triplicate samples and a heatmap to visualize the RNA-seq results. A volcano plot was created using ggVolcanoR (https://ggvolcanor.erc.monash.edu). Protein ANNotation with Z-scoRE (PANNZER2; http://ekhidna2.biocenter.helsinki.fi/sanspanz) was used for gene ontology (GO) analysis to predict the genes up- and downregulated in response to erythritol.
Transcriptor First Strand cDNA Synthesis Kit (Roche Diagnostics, Rotkreuz, Switzerland) with random primers was used to synthesize complementary DNA from total RNA extracted from JCM7470 with or without erythritol. Supplementary Table 1 lists the primers used in this study. The primer sets and TB Green® Fast qPCR Mix (Takara Bio, Kusatsu, Japan) were used for RT-qPCR on a Thermal Cycler Dice® Real-Time System III (Takara Bio) with 45 cycles of 95°C for 5 s and 55°C for 60 s. The recA gene, which has been validated as an appropriate reference gene for qPCR in SP (31), was used as a reference to evaluate the relative gene expression levels of the other genes. The RNA-seq performed in this study revealed that the recA gene expression in SC was consistent regardless of the presence of erythritol (log2FC = −0.19, p = 0.111), suggesting the validity of the recA gene as a reference gene for qPCR in SC. This experiment was performed in triplicate, and mean values were compared among the groups.
The SC clinical isolates were pre-cultured in Luria-Bertani (LB) medium (Kanto Chemical Co., Inc., Tokyo, Japan) and diluted fivefold in this medium. Then, 30 μL of the diluted bacterial suspension was mixed with 900 μL of NRBC #802 medium with erythritol at concentrations of 0%, 5%, 10%, and 15% [w/w] in 96-well U-bottomed microplates (Watson Corporation, Tokyo, Japan). The OD600 was measured over time using EpochTM2 (Agilent Technologies, Inc., Santa Clara, CA, USA) from 0 to 6 h.
The effect of glucose on the erythritol-induced growth inhibition of SC clinical isolates was analyzed as follows. The SC isolates were incubated in NRBC #802 medium for 2 h and diluted fivefold in NRBC #802 medium. Then, 30 μL of the diluted bacterial suspension was mixed with 900 μL of NRBC #802 medium containing 0% erythritol and 0% glucose, 0% erythritol and 0.1% glucose, 10% erythritol and 0.1% glucose, 10% erythritol and 1% glucose, or 10% erythritol and 0% glucose, and 200 μL was inoculated into each 96-well plate. The OD600 was measured over time for up to 6 h. The experiments analyzing growth were performed in triplicate, and mean values were compared among the groups.
Empirical Analysis of Digital Gene Expression Data in R (edgeR) exactTest was used to compare gene expression levels analyzed by RNA-seq. GraphPad Prism 9 software (GraphPad Software Inc., San Diego, CA, USA) was used for the following statistical analysis. Welch's t-test was used to compare transcription levels of glcB and ptsG genes between the groups, and the effect of erythritol on mecA gene-positive and -negative SC strains. Dunnett's test was used to compare the turbidity between SC strains incubated in the presence or absence of erythritol and/or glucose. A p-value of less than 0.05 was considered statistically significant.
We first performed RNA-seq analysis to investigate the molecular mechanism behind the growth inhibition of JCM7470 by erythritol. After filtering the raw sequencing reads, we obtained 16,589,804, 16,962,572, and 27,123,064 clean reads of the transcriptome in control samples. By contrast, there were 17,294,425, 18,110,786, and 15,713,381 clean reads in the erythritol-treated samples.
The calculated gene expression levels [|log2 fold change (log2FC)| > 1, p < 0.05] identified a total of 162 differentially expressed genes, including 60 upregulated and 102 downregulated genes, in JCM7470 following erythritol treatment. The PCA plot with 80% of the variance explained by PC1 exhibited a clear split between the control and erythritol-treated samples (Figure 1A). The heatmap is shown in Supplementary Figure 1.
Figure 1. Gene expression analysis. (A) PCA plot shows a clear split between the control and erythritol-treated Staphylococcus coagulans JCM7470, with 80% of the variance explained by PC1. (B) The volcano plot shows differences in expression levels of up- and downregulated genes in JCM7470 treated with erythritol. The genes upregulated >10-fold (log2FC > 3.322, p < 0.05) in response to erythritol are highlighted in red. (C) Comparison of the expression levels of glcB and ptsG genes in JCM7470 treated with erythritol or control medium, as determined by RT-qPCR. **p < 0.01.
Among the 162 genes in JCM7470 differentially expressed in response to erythritol, only four were upregulated more than 10-fold (log2FC > 3.322) in the presence of erythritol. Conversely, no genes were downregulated more than 10-fold in the presence of erythritol.
Supplementary Table 3 shows the predicted functional descriptions and GO biological processes for the top 15 up- and downregulated genes in response to erythritol. The GO analysis revealed that two of the four most upregulated genes were glcB [log2FC = 5.459, p < 0.001, positive predictive value (PPV) = 0.71] and ptsG (log2FC = 3.727, p < 0.001, PPV = 0.71), both of which encode PTS transporter subunit IIBC (Figure 1B). Meanwhile, the other two most upregulated genes encode hypothetical proteins for which the associated biological processes are unknown (Figure 1B). RT-qPCR revealed that transcription levels of glcB and ptsG genes in the erythritol-treated group were significantly higher than those in the control group (glcB, p = 0.0024; ptsG, p = 0.0028, Figure 1C). The log2 fold changes of glcB and ptsG in the erythritol-treated group relative to the levels in the control group were 5.57 ± 0.06 and 4.12 ± 0.06 (mean ± SE), respectively.
We next investigated whether erythritol suppresses the growth of SC isolated from clinical lesions of canine pyoderma. In vitro turbidity assay revealed that the turbidity increased over time up to 6 h in both the control group and the erythritol-supplemented groups, regardless of whether the strains carried the mecA gene (Supplementary Figure 2). The turbidity of 27 SC clinical isolates incubated for 6 h in the presence of 5% (0.728 ± 0.172, p < 0.0001), 10% (0.460 ± 0.130, p < 0.0001), and 15% erythritol (0.283 ± 0.070, p < 0.0001) was significantly lower than the level upon incubation in the absence of erythritol (1.023 ± 0.193). Moreover, erythritol suppressed the growth of the SC clinical isolates in a concentration-dependent manner (p < 0.0001). In contrast, there were no significant differences in the baseline bacterial turbidity in the SC groups between erythritol-supplemented groups and the control group (p > 0.05) (Figure 2A). Furthermore, there were no significant differences in turbidity between the mecA gene-positive strains (n = 13) and mecA gene-negative strains (n = 14) supplemented with 0% (p = 0.295), 5% (p = 0.332), 10% (p = 0.703), and 15% erythritol (p = 0.709) (Figure 2B).
Figure 2. Restoration of erythritol-induced growth inhibition of SC clinical isolates by glucose supplementation. (A) Bacterial turbidity of the SC clinical isolates (n = 27) immediately or 6 h after incubation with different doses of erythritol. (B) Comparison of bacterial turbidity between mecA gene-positive and -negative SC strains 6 h after incubation with different doses of erythritol. (C) Fluctuation of the bacterial turbidity of the SC strains incubated with different doses of erythritol and glucose. ns, not significant; ****p < 0.0001.
Considering the upregulation of PTS-related genes and growth inhibition, we wondered whether carbohydrate starvation in response to erythritol caused the growth inhibition of the SC clinical isolates. Therefore, we investigated whether glucose supplementation of the culture media of the SC clinical isolates would overcome the growth inhibition induced by erythritol. We found that the turbidity of the SC clinical isolates supplemented with 10% erythritol and 0.1% (0.577 ± 0.250, p < 0.0001) or 1% glucose (0.704 ± 0.275, p < 0.0001) was significantly higher than that upon supplementation with 10% erythritol alone (Figure 2C).
The present study revealed that erythritol significantly upregulated the expression of glcB and ptsG encoding PTS transporter subunit IIBC in the SC strain. GO analysis predicted that the transcripts of these two genes function as glycoside- and glucose-specific enzyme II components EIICBA, respectively. The upregulation of ptsG gene expression in response to erythritol in SC was in agreement with the data obtained in our recent study using an SP strain (26). The EIICBA are membrane permeases that play significant roles in the uptake of carbohydrates into the bacterial cytoplasm (32, 33). EIIA, EIIB, and EIIC usually specifically incorporate one substrate or closely related carbohydrates into bacterial cytoplasm (34).
Furthermore, restoration of the erythritol-induced growth suppression by glucose supplementation implies that glucose starvation in response to erythritol may cause the upregulation of PTS-related gene expression and result in the growth inhibition of SC. It was reported that, in S. mutans, xylitol 5-phosphate, a metabolite derived from xylitol, directly inhibits glycolytic enzymes and competes with glucose 6-phosphate, a glucose metabolite incorporated into glycolysis (24). We speculate that erythritol or its metabolites also compete for the glycolytic enzyme in SC, although the exact erythritol uptake and metabolic pathways in the staphylococci have yet to be determined.
Previous studies revealed that the ptsG operon of S. carnosus consists of two adjacent genes, glcA and glcB, which encode IICBAGlc1 and IICBAGlc2, respectively (32, 33, 35). Analysis of the deduced amino acid sequence suggested that the ptsG gene in SC (NCBI WP_ 050331035.1) consists of two components, PTS-II-BC-glcB (glucose-specific IIBC component) and PTS-EIIA-1, while the glcB gene in SC (NCBI WP_ 0503356536.1) consists of two components, PTS-II-BC-glcB and NagE (IIA component). Efforts should be made to ensure consistency in the gene nomenclature between S. carnosus and SC. Nevertheless, the sequence analysis suggested that these two genes encode enzyme II components crucial in carbohydrate uptake into SC.
In the SP strain, erythritol upregulated ppdK and ppdkR, which are other PTS-related genes predicted to encode pyruvate phosphate dikinase (PPDK) and PPDK regulatory protein (PPDKR), respectively (26). The same study also revealed that erythritol downregulated the expression of pur operon genes involved in the synthesis of inosinic acid (IMP) leading to purine biosynthesis in the SP strain. However, such changes were not recognized in the present study using the SC strains. Possible reasons for this discrepancy include differences in the bacterial species or that such changes are a late phenomenon occurring in response to glucose starvation. Indeed, PPDK and PPDKR contribute to the regeneration of PEP necessary to reactivate PTS (36, 37), and phosphoribosyl pyrophosphate, the precursor of IMP, is a metabolite derived from glucose 6-phosphate through the pentose phosphate pathway (38). The expression of genes encoding vraTSR, which are associated with resistance to β-lactams and glycopeptides in S. aureus (39–43), and sgtB, which is involved in proteoglycan biosynthesis in S. aureus (44), was also upregulated in the SP strain. The present study revealed slight increases in vraS, sgtB, and vraR gene expression (|log2FC| < 1.9) (Supplementary Table 3). However, the biological significance of these changes induced by erythritol in SC was not identified in this study because the changes in the susceptibility of SC to antibiotics were not evaluated.
We also revealed that erythritol inhibited the growth of SC isolated from the skin lesions of canines with pyoderma. A previous study revealed that erythritol has a bacteriostatic effect on bacteria associated with canine periodontal disease (45). Based on these findings, we assumed that SC strains whose growth was inhibited in the presence of erythritol may grow on agar plates for colony counting and thus did not analyze the effect of erythritol on colony-forming units. Studies have reported that erythritol was more efficient at inhibiting the growth of human oral commensal streptococci than xylitol (18), while such efficacy of erythritol against SP and SC clinical isolates was similar to that of xylitol (25).
Unlike in humans, the safe dosage range of xylitol in dogs is narrow and there is a risk of xylitol toxicity such as hypoglycemia and acute liver failure, which raises safety concerns (46, 47). In contrast, erythritol was less likely to cause hypoglycemia and appeared to be safe for dogs, as determined by oral toxicity studies (48, 49). Therefore, topical application of erythritol is expected to have the potential to alleviate the clinical severity of canine pyoderma caused by SC or to prevent its recurrence. Future clinical trials with topically applied erythritol for canine pyoderma are expected. It is also anticipated that erythritol can prevent SC infections via contaminated medical equipment in humans and animals.
Our findings suggest that glucose starvation in response to erythritol contributes to growth inhibition in SC. Our findings also suggest the potential of erythritol in preventing SC-associated cutaneous infections in dogs and the contamination of medical equipment.
The RNA-seq data are available at Gene Expression Omnibus (GEO) in The National Center for Biotechnology Information with GEO accession number GSE245057. All other data are available from the corresponding author upon reasonable request.
SO-S: Formal analysis, Investigation, Writing – original draft. TF: Formal analysis, Conceptualization, Methodology, Writing – review & editing. KW: Writing – review & editing, Investigation. RM: Investigation, Writing – review & editing. KI: Investigation, Writing – review & editing, Formal analysis. YT: Writing – review & editing, Methodology. TT: Writing – review & editing, Conceptualization. KN: Conceptualization, Writing – review & editing.
The author(s) declare that no financial support was received for the research, authorship, and/or publication of this article.
The authors are grateful to all members of the Research and Development Center at B Food Science Co., Ltd., for supporting this study. The authors also thank Edanz (https://jp.edanz.com/ac) for editing a draft of this manuscript.
RM is an employee of B Food Science Co., Ltd. TF and TT were employees of the same company at the time when this study was started. KI was employed by Vet Derm Tokyo Ltd. where YT the representative director.
The remaining authors declare that the research was conducted in the absence of any commercial or financial relationships that could be construed as a potential conflict of interest.
All claims expressed in this article are solely those of the authors and do not necessarily represent those of their affiliated organizations, or those of the publisher, the editors and the reviewers. Any product that may be evaluated in this article, or claim that may be made by its manufacturer, is not guaranteed or endorsed by the publisher.
The Supplementary Material for this article can be found online at: https://www.frontiersin.org/articles/10.3389/fvets.2023.1272595/full#supplementary-material
1. Madhaiyan M, Wirth JS, Saravanan VS. Phylogenomic analyses of the Staphylococcaceae family suggest the reclassification of five species within the genus Staphylococcus as heterotypic synonyms, the promotion of five subspecies to novel species, the taxonomic reassignment of five Staphylococcus species to Mammaliicoccus gen. nov, and the formal assignment of Nosocomiicoccus to the family Staphylococcaceae. Int J Syst Evol Microbiol. (2020) 70:5926–36. doi: 10.1099/ijsem.0.004498
2. Igimi S, Takahashi E, Mitsuoka T. Staphylococcus schleiferi subsp. coagulans subsp nov, isolated from the external auditory meatus of dogs with external ear otitis. Int J Syst Bacteriol. (1990) 40:409–11. doi: 10.1099/00207713-40-4-409
3. Bes M, Guerin-Faublee V, Freney J, Etienne J. Isolation of Staphylococcus schleiferi subspecies coagulans from two cases of canine pyoderma. Vet Rec. (2002) 150:487–8. doi: 10.1136/vr.150.15.487
4. Frank LA, Kania SA, Hnilica KA, Wilkes RP, Bemis DA. Isolation of Staphylococcus schleiferi from dogs with pyoderma. J Am Vet Med Assoc. (2003) 222:451–4. doi: 10.2460/javma.2003.222.451
5. May ER, Hnilica KA, Frank LA, Jones RD, Bemis DA. Isolation of Staphylococcus schleiferi from healthy dogs and dogs with otitis, pyoderma, or both. J Am Vet Med Assoc. (2005) 227:928–31. doi: 10.2460/javma.2005.227.928
6. Naing SY, Duim B, Broens EM, Schweitzer V, Zomer A, van der Graaf-van Bloois L, et al. Molecular characterization and clinical relevance of taxonomic reassignment of Staphylococcus schleiferi Subspecies into two separate species, Staphylococcus schleiferi and Staphylococcus coagulans. Microbiol Spectr. (2023):e0467022. doi: 10.1128/spectrum.04670-22
7. Loeffler A, Lloyd DH. What has changed in canine pyoderma? A narrative review. Vet J. (2018) 235:73–82. doi: 10.1016/j.tvjl.2018.04.002
8. Thibodeau E, Boucher H, Denofrio D, Pham DT, Snydman D. First report of a left ventricular assist device infection caused by Staphylococcus schleiferi subspecies coagulans: a coagulase-positive organism. Diagn Microbiol Infect Dis. (2012) 74:68–9. doi: 10.1016/j.diagmicrobio.2012.05.027
9. Kobayashi T, Ikeda M, Ohama Y, Murono K, Ikeuchi K, Kitaura S, et al. First human case of catheter-related blood stream infection caused by Staphylococcus schleiferi subspecies coagulans: a case report and literature review. Ann Clin Microbiol Antimicrob. (2021) 20:68. doi: 10.1186/s12941-021-00474-3
10. Dad RK, Avais M, Khan JA, Anjum AA. Evaluating the effectiveness of multidrug resistant mastitis vaccines in dairy cattle. Pak Vet J. (2022) 42:300–7. doi: 10.29261/pakvetj/2022.038
11. Aljahani AH, Alarjani KM, Hassan ZK, Elkhadragy MF, Ismail EA, Al-Masoud AH, et al. Molecular detection of methicillin heat-resistant Staphylococcus aureus strains in pasteurized camel milk in Saudi Arabia. Biosci Rep. (2020) 40:BSR20193470. doi: 10.1042/BSR20193470
12. Zehra A, Singh R, Kaur S, Gill JPS. Molecular characterization of antibiotic-resistant Staphylococcus aureus from livestock (bovine and swine). Vet World. (2017) 10:598–604. doi: 10.14202/vetworld.2017.598-604
13. Abd-Elfatah EB, Fawzi EM, Elsheikh HA, Shehata AA. Prevalence, virulence genes and antibiogram susceptibility pattern of Staphylococcus aureus and Streptococcus agalactiae isolated from mastitic ewes. Int J Vet Sci. (2023) 12:152–60. doi: 10.47278/journal.ijvs/2022.176
14. Cain CL, Morris DO, O'Shea K, Rankin SC. Genotypic relatedness and phenotypic characterization of Staphylococcus schleiferi subspecies in clinical samples from dogs. Am J Vet Res. (2011) 72:96–102. doi: 10.2460/ajvr.72.1.96
15. Cain CL, Morris DO, Rankin SC. Clinical characterization of Staphylococcus schleiferi infections and identification of risk factors for acquisition of oxacillin-resistant strains in dogs: 225 cases (2003-2009). J Am Vet Med Assoc. (2011) 239:1566–73. doi: 10.2460/javma.239.12.1566
16. Huse HK, Miller SA, Chandrasekaran S, Hindler JA, Lawhon SD, Bemis DA, et al. Evaluation of oxacillin and cefoxitin disk diffusion and mic breakpoints established by the clinical and laboratory standards institute for detection of meca-mediated oxacillin resistance in Staphylococcus schleiferi. J Clin Microbiol. (2018) 56:e01653–17. doi: 10.1128/JCM.01653-17
17. Kawakami T, Shibata S, Murayama N, Nagata M, Nishifuji K, Iwasaki T, et al. Antimicrobial susceptibility and methicillin resistance in Staphylococcus pseudintermedius and Staphylococcus schleiferi subsp. coagulans isolated from dogs with pyoderma in Japan. J Vet Med Sci. (2010) 72:1615–9. doi: 10.1292/jvms.10-0172
18. de Cock P, Makinen K, Honkala E, Saag M, Kennepohl E, Eapen A. Erythritol is more effective than xylitol and sorbitol in managing oral health endpoints. Int J Dent. (2016) 2016:9868421. doi: 10.1155/2016/9868421
19. Hashino E, Kuboniwa M, Alghamdi SA, Yamaguchi M, Yamamoto R, Cho H, et al. Erythritol alters microstructure and metabolomic profiles of biofilm composed of Streptococcus gordonii and Porphyromonas gingivalis. Mol Oral Microbiol. (2013) 28:435–51. doi: 10.1111/omi.12037
20. Park Y-N, Jeong S-S, Zeng J, Kim S-H, Hong S-J, Ohk S-H, et al. Anti-cariogenic effects of erythritol on growth and adhesion of Streptococcus mutans. Food Sci Biotechnol. (2014) 23:1587–91. doi: 10.1007/s10068-014-0215-0
21. Shimizu M, Miyawaki S, Kuroda T, Umeta M, Kawabe M, Watanabe K. Erythritol inhibits the growth of periodontal-disease-associated bacteria isolated from canine oral cavity. Heliyon. (2022) 8:e10224. doi: 10.1016/j.heliyon.2022.e10224
22. Fujii T, Inoue S, Kawai Y, Tochio T, Takahashi K. Suppression of axillary odor and control of axillary bacterial flora by erythritol. J Cosmet Dermatol. (2022) 21:1224–33. doi: 10.1111/jocd.14201
23. Fujii T, Tochio T, Endo A. Ribotype-dependent growth inhibition and promotion by erythritol in Cutibacterium acnes. J Cosmet Dermatol. (2022) 21:5049–57. doi: 10.1111/jocd.14958
24. Miyasawa-Hori H, Aizawa S, Takahashi N. Difference in the xylitol sensitivity of acid production among Streptococcus mutans strains and the biochemical mechanism. Oral Microbiol Immunol. (2006) 21:201–5. doi: 10.1111/j.1399-302X.2006.00273.x
25. Tochio T, Kawano K, Iyori K, Makida R, Kadota Y, Fujii T, et al. Topical erythritol combined with L-ascorbyl-2-phosphate inhibits staphylococcal growth and alleviates staphylococcal overgrowth in skin lesions of canine superficial pyoderma. Pol J Vet Sci. (2023) 26:647–55. doi: 10.24425/pjvs.2023.148284
26. Fujii T, Tochio T, Nishifuji K. Erythritol alters gene transcriptome signatures, cell growth, and biofilm formation in Staphylococcus pseudintermedius. BMC Vet Res. (2023) 19:146. doi: 10.1186/s12917-023-03711-3
27. Schissler JR, Hillier A, Daniels JB, Cole LK, Gebreyes WA. Evaluation of Clinical Laboratory Standards Institute interpretive criteria for methicillin-resistant Staphylococcus pseudintermedius isolated from dogs. J Vet Diagn Invest. (2009) 21:684–8. doi: 10.1177/104063870902100514
28. Sasaki T, Tsubakishita S, Tanaka Y, Sakusabe A, Ohtsuka M, Hirotaki S, et al. Multiplex-PCR method for species identification of coagulase-positive staphylococci. J Clin Microbiol. (2010) 48:765–9. doi: 10.1128/JCM.01232-09
29. Gonzalez-Dominguez MS, Carvajal HD, Calle-Echeverri DA, Chinchilla-Cardenas D. Molecular detection and characterization of the mecA and nuc genes from Staphylococcus species (S. aureus, S pseudintermedius, and S schleiferi) isolated from dogs suffering superficial pyoderma and their antimicrobial resistance profiles. Front Vet Sci. (2020) 7:376. doi: 10.3389/fvets.2020.00376
30. Iyori K, Shishikura T, Shimoike K, Minoshima K, Imanishi I, Toyoda Y. Influence of hospital size on antimicrobial resistance and advantages of restricting antimicrobial use based on cumulative antibiograms in dogs with Staphylococcus pseudintermedius infections in Japan. Vet Dermatol. (2021) 32:668–e178. doi: 10.1111/vde.12975
31. Crawford EC, Singh A, Metcalf D, Gibson TW, Weese SJ. Identification of appropriate reference genes for qPCR studies in Staphylococcus pseudintermedius and preliminary assessment of icaA gene expression in biofilm-embedded bacteria. BMC Res Notes. (2014) 7:451. doi: 10.1186/1756-0500-7-451
32. Christiansen I, Hengstenberg W. Staphylococcal phosphoenolpyruvate-dependent phosphotransferase system–two highly similar glucose permeases in Staphylococcus carnosus with different glucoside specificity: protein engineering in vivo? Microbiology (Reading). (1999) 145:2881–9. doi: 10.1099/00221287-145-10-2881
33. Knezevic I, Bachem S, Sickmann A, Meyer HE, Stulke J, Hengstenberg W. Regulation of the glucose-specific phosphotransferase system (PTS) of Staphylococcus carnosus by the antiterminator protein GlcT. Microbiology (Reading). (2000) 146:2333–42. doi: 10.1099/00221287-146-9-2333
34. Deutscher J, Ake FM, Derkaoui M, Zebre AC, Cao TN, Bouraoui H, et al. The bacterial phosphoenolpyruvate: carbohydrate phosphotransferase system: regulation by protein phosphorylation and phosphorylation-dependent protein-protein interactions. Microbiol Mol Biol Rev. (2014) 78:231–56. doi: 10.1128/MMBR.00001-14
35. Christiansen I, Hengstenberg W. Cloning and sequencing of two genes from Staphylococcus carnosus coding for glucose-specific PTS and their expression in Escherichia coli K-12. Mol Gen Genet. (1996) 250:375–9. doi: 10.1007/BF02174396
36. Cui J, Maloney MI, Olson DG, Lynd LR. Conversion of phosphoenolpyruvate to pyruvate in Thermoanaerobacterium saccharolyticum. Metab Eng Commun. (2020) 10:e00122. doi: 10.1016/j.mec.2020.e00122
37. Chastain CJ, Xu W, Parsley K, Sarath G, Hibberd JM, Chollet R. The pyruvate, orthophosphate dikinase regulatory proteins of Arabidopsis possess a novel, unprecedented Ser/Thr protein kinase primary structure. Plant J. (2008) 53:854–63. doi: 10.1111/j.1365-313X.2007.03366.x
38. Garcia Sanchez R, Hahn-Hagerdal B, Gorwa-Grauslund MF. Cross-reactions between engineered xylose and galactose pathways in recombinant Saccharomyces cerevisiae. Biotechnol Biofuels. (2010) 3:19. doi: 10.1186/1754-6834-3-19
39. Boyle-Vavra S, Yin S, Jo DS, Montgomery CP, Daum RS. VraT/YvqF is required for methicillin resistance and activation of the VraSR regulon in Staphylococcus aureus. Antimicrob Agents Chemother. (2013) 57:83–95. doi: 10.1128/AAC.01651-12
40. Kuroda M, Kuroda H, Oshima T, Takeuchi F, Mori H, Hiramatsu K. Two-component system VraSR positively modulates the regulation of cell-wall biosynthesis pathway in Staphylococcus aureus. Mol Microbiol. (2003) 49:807–21. doi: 10.1046/j.1365-2958.2003.03599.x
41. Gardete S, Wu SW, Gill S, Tomasz A. Role of VraSR in antibiotic resistance and antibiotic-induced stress response in Staphylococcus aureus. Antimicrob Agents Chemother. (2006) 50:3424–34. doi: 10.1128/AAC.00356-06
42. Boyle-Vavra S, Yin S, Daum RS. The VraS/VraR two-component regulatory system required for oxacillin resistance in community-acquired methicillin-resistant Staphylococcus aureus. FEMS Microbiol Lett. (2006) 262:163–71. doi: 10.1111/j.1574-6968.2006.00384.x
43. Mehta S, Cuirolo AX, Plata KB, Riosa S, Silverman JA, Rubio A, et al. VraSR two-component regulatory system contributes to mprF-mediated decreased susceptibility to daptomycin in in vivo-selected clinical strains of methicillin-resistant Staphylococcus aureus. Antimicrob Agents Chemother. (2012) 56:92–102. doi: 10.1128/AAC.00432-10
44. Wu S, Lin K, Liu Y, Zhang H, Lei L. Two-component signaling pathways modulate drug resistance of Staphylococcus aureus (Review). Biomed Rep. (2020) 13:5. doi: 10.3892/br.2020.1312
45. Tochio T, Makida R, Fujii T, Kadota Y, Takahashi M, Watanabe A, et al. The bacteriostatic effect of erythritol on canine periodontal disease-related bacteria. Pol J Vet Sci. (2022) 25:75–82. doi: 10.24425/pjvs.2022.140843
46. Dunayer EK, Gwaltney-Brant SM. Acute hepatic failure and coagulopathy associated with xylitol ingestion in eight dogs. J Am Vet Med Assoc. (2006) 229:1113–7. doi: 10.2460/javma.229.7.1113
47. Schmid RD, Hovda LR. Acute hepatic failure in a dog after xylitol ingestion. J Med Toxicol. (2016) 12:201–5. doi: 10.1007/s13181-015-0531-7
48. Dean I, Jackson F, Greenough RJ. Chronic (1-year) oral toxicity study of erythritol in dogs. Regul Toxicol Pharmacol. (1996) 24:S254–60. doi: 10.1006/rtph.1996.0106
Keywords: erythritol, Staphylococcus coagulans, dog, pyoderma, glucose, phosphotransferase system
Citation: Onishi-Sakamoto S, Fujii T, Watanabe K, Makida R, Iyori K, Toyoda Y, Tochio T and Nishifuji K (2024) Erythritol alters phosphotransferase gene expression and inhibits the in vitro growth of Staphylococcus coagulans isolated from canines with pyoderma. Front. Vet. Sci. 10:1272595. doi: 10.3389/fvets.2023.1272595
Received: 04 August 2023; Accepted: 11 December 2023;
Published: 04 January 2024.
Edited by:
Kun Li, Nanjing Agricultural University, ChinaReviewed by:
Meera Surendran Nair, The Pennsylvania State University (PSU), United StatesCopyright © 2024 Onishi-Sakamoto, Fujii, Watanabe, Makida, Iyori, Toyoda, Tochio and Nishifuji. This is an open-access article distributed under the terms of the Creative Commons Attribution License (CC BY). The use, distribution or reproduction in other forums is permitted, provided the original author(s) and the copyright owner(s) are credited and that the original publication in this journal is cited, in accordance with accepted academic practice. No use, distribution or reproduction is permitted which does not comply with these terms.
*Correspondence: Koji Nishifuji, a29qaW1haWxAY2MudHVhdC5hYy5qcA==
†ORCID: Saki Onishi-Sakamoto orcid.org/0009-0009-9303-283X
Keita Iyori orcid.org/0000-0003-1977-4201
Takumi Tochio orcid.org/0000-0003-3206-4116
Koji Nishifuji orcid.org/0000-0003-4583-5485
Disclaimer: All claims expressed in this article are solely those of the authors and do not necessarily represent those of their affiliated organizations, or those of the publisher, the editors and the reviewers. Any product that may be evaluated in this article or claim that may be made by its manufacturer is not guaranteed or endorsed by the publisher.
Research integrity at Frontiers
Learn more about the work of our research integrity team to safeguard the quality of each article we publish.