- 1Facultad de Medicina Veterinaria y Zootecnia, Universidad Autónoma del Estado de México, Toluca, Mexico
- 2Centro de Investigaciones Agropecuarias, Facultad de Ciencias Agrarias, Universidad Estatal Península de Santa Elena, Santa Elena, Ecuador
- 3Facultad de Estudios Superiores Cuautitlan, Universidad Nacional Autonoma de Mexico (UNAM), Cuautitlan, Mexico
- 4Department of Farm Animals and Public Health, Institute of Animal Nutrition and Functional Plant Compounds, University of Veterinary Medicine Vienna, Vienna, Austria
- 5Facultad de Medicina Veterinaria y Zootecnia No. 1, Universidad Autónoma de Guerrero, Guerrero, Mexico
- 6Facultad de Ciencias Agropecuarias, Universidad Técnica de Ambato, Sector el Tambo-La Universidad, vía a Quero, Cevallos, Ambato, Ecuador
- 7Department of Animal Science, Faculty of Agriculture, University of Uyo, Uyo, Nigeria
- 8School of Animal Technology and Innovation, Institute of Agricultural Technology, Suranaree University of Technology, Nakhon Ratchasima, Thailand
Introduction: Mitigation of ruminant greenhouse gas (GHG) emissions is crucial for more appropriate livestock production. Thus, there is a need of further research evaluating feed supplementation strategies to mitigate enteric GHG emissions and other gases produced within the rumen.
Methods: This study was conducted as a completely randomized experimental design to determine the effectiveness of liquid extracts from A. indica (AZI), C. angustidens (CNA), or their combination (Mix. 1:1) at dosages of 0, 36, 72, and 108 mg of liquid extract/g DM substrate incubated in reducing GHG production in vitro, particularly methane (CH4), from the diet of steers during anaerobic incubation in rumen fluid. Total gas production, CH4, CO, H2S, and fermentative characteristics were all measured in vitro.
Results: Treatment AZI at a dose of 108 mg of liquid extract/g DM substrate produced the highest (P < 0.05) gas volume at 6 h, whereas CNA at a dose of 72 mg of liquid extract/ g DM substrate produced the least (P < 0.05) at 6 and 24 h, and Mix. at a dose of 72 mg of liquid extract/g DM substrate produced the least (P < 0.05) at 48 h. In addition, CH4 levels at 6 and 24 h of incubation (36 mg/g DM substrate) were highest (P < 0.05) for CNA, and lowest (P < 0.05) for AZI, whereas this variable was lowest (P < 0.05) at 72 mg of liquid extract for CNA at 24 and 48 h. At 6 and 24 h, CO volume was highest (P < 0.05) for AZI at 108 mg of liquid extract and lowest (P < 0.05) for Mix. at 72 mg of liquid extract. Treatment Mix. had a high (P < 0.05) concentration of short chain fatty acids at 72 mg of liquid extract/g DM of substrate.
Discussion: In general, herbaceous perennial plants, such as AZI and CNA, could be considered suitable for mitigating enteric GHG emissions from animals. Specifically, the treatment Mix. achieved a greater sustainable reduction of 67.6% in CH4 and 47.5% in H2S production when compared to either AZI. This reduction in CH4 might suggest the potential of the combination of both plant extracts for mitigating the production of GHG from ruminants.
Introduction
Enteric greenhouse gas (GHG) emissions have been a crucial ecological concern for humanity during the last two decades (1). From these emissions, ruminant livestock contributes the most, particularly beef and dairy cattle, about 7–18% of global GHG emissions, represented by methane (CH4), carbon dioxide (CO2), carbon monoxide (CO), hydrogen sulfide (H2S), and nitrogen oxide (2). In the United States, for example, livestock produced 3.10% of total GHG emissions in 2009, ranking second in terms of CH4 emissions and third in terms of non-CO2 emissions (3). These gases have a warming potential between 25 and 298 CO2 equivalent kg/kg (4). On-farm emissions account for a sizable portion of the carbon footprint associated with the dairy or beef supply chains (5, 6). Thus, ruminants and their associated gaseous emissions (enteric or manure) including CH4, CO2, and non-CO2 emissions should be the primary focus of any successful GHG mitigation effort. Additionally, in the rumen, H2S and CO originate from ruminal fermentation, and are utilized by the archaea in the synthesis of CH4, as detailed in Russel et al. (7). Components containing sulfur and sulfate can use H+ at low concentrations to produce H2S; therefore, interfering with methanogenesis CH4 (8, 9). Reducing sulfite to produce H2S consumes eight electrons and thus can be an alternate electron acceptor (8). On the other hand, CO may also interfere with CH4 formation because of its inhibitory effect on some ruminal bacteria (10). Therefore, evaluating the production of these gasses is important as well.
There are numerous strategies tested over the last few decades to reduce the GHG emissions from livestock production systems, including the use of natural products and their potential applications as feed additives. In this context, a variety of secondary metabolites are produced by plants as a means of protecting themselves from parasite attacks (11). These compounds include tannins, saponins, essential oils, cinnamic acids, flavonoids, and other phenolics, all of which have the potential to be used as anti-methanogenic chemicals (12). Notably, it has been demonstrated that supplementing with medicinal plant either as an extract or fermented has a beneficial effect on reducing methane production by altering the composition and structure of the ruminal microbial community (13–16). Indeed, by altering metabolic pathways, the addition of favorable additives to animal feed results in a subsequent reduction in the substrate availability for methanogenesis (17).
The use of fodder trees has been reported to improve ruminal degradation of nutrients, volatile fatty acids (18), microbial protein synthesis in the rumen (19), and reduce methanogens possibly due to the presence the secondary metabolites that may influence microbial activity (20, 21). In particular, trees such as Azadirachta indica (AZI), and Cnidoscolus angustidens (CNA) (22) may represent a good option to mitigate methanogenesis. One study use the in vitro fermentation technique to evaluate AZI and CNA in horse feces (18). It is worth noting, however, that studies conducted in vitro (23–25) should be confirmed by in vivo methods. Despite the promising effects of AZI and CNA for the mitigation of CH4 emission, to the authors’ knowledge, there is limited research evaluating their effects in the rumen (26), which represents a research gap that needs to be addressed in the scientific literature. In this regard, the combination of two or more plant extracts might provide unique biological characteristics that differ from the individual extracts because of the potential synergistic effect (27, 28). For example, certain phytogenic compound, such as the essential oils, possess a mixture of phytochemicals and their synergistic effects may lead to the synthesis of new compounds with different bioactivity (29). In particular, AZI is rich in essential oils, including 9.5% palmitic acid, 5.0% stearic acid, 32.0% oleic acid, 52.0% linoleic acid 0.51% linolenic acid (30), and other compounds such as azadirachtin, nimbolinin, nimbin, and quercetin (31). In the present experiment, our hypothesis was that the incubations with the extracts of AZI and CNA will effectively reduce the production of GHG under in vitro conditions. We also hypothesized that the combination of both treatments may result in a synergistic effect for the mitigation of GHG production.
Therefore, the objective of this study was to evaluate effects of aqueous leaf extracts of AZI, CNA or their combination on rumen GHG production in vitro.
Materials and methods
Collection of plants and preparation of extracts
The protocol of this in vitro study did not require the approval of the Animal Care and Use Committee because the utilized ruminal fluid was collected from slaughtered animals that were not part of the experiment.
Bioactive compounds are commonly present in the plants, but their concentration may vary according to plant maturity. For this reason, the vegetative material was collected throughout 7 months for both plants, when their phenological stage changes to flowering. In CNA the root was used, whereas in AZI the leaves were used. Roots of the CNA were taken manually from five plants in Puente de Ixtla, Morelos, Mexico, in the month of August 2021, later they were washed, peeled and frozen (approximately between –16°C and 24°C) for later use. Leaves samples of the AZI, were taken from five trees in the municipality of Tecpan de Galeana, Guerrero, Mexico, in February 2022, after which this vegetative material was dried at 40°C (BINDER Model FD115 Incubator, Germany) and ground (Willey Mill Model 5KH39ON5525, Mexico with a 1 mm sieve) in the bromatology laboratory of the Faculty of Veterinary Medicine and Animal Science of the Autonomous University of the State of Mexico. Finally, it was stored at room temperature (i.e., 25–30°C) for later use.
To prepare the extract of each plant species, we followed a protocol similar to reports from other researchers (18). Briefly, collected samples of individual species were ground into 1 mm length using a blender and subsequently extracted using 1 g of sample for every 8 mL of distilled water. Plant samples were soaked and incubated in water at 27–29°C for 72 h in closed 5 L glass containers. After incubation, the contents were filtered through 4–5 layers of gauze and the extract was collected. For practicality and because of the timeframe associated with the preparation of the leaf extracts, these were prepared weekly (at a stock volume of 8 L each). This mixture was stored at 2°C –4°C prior to use for the in vitro incubation, in order to avoid any further fermentation. Sometimes the extract was used immediately after preparation for the in vitro incubation, but no differences in fermentation performance was found between fresh and stored extract.
Chemical analysis
Dietary substrate samples were analyzed in triplicate for DM, ash, organic matter (OM), nitrogen (N) and ether extract according to AOAC methods (32, 33). The analyses of neutral detergent fiber (NDFom) and acid detergent fiber (ADF) were performed in accordance with AOAC standards (32) using an ANKOM200 Fiber Analyzer Unit (ANKOM Technology Corp., Macedon, NY). The NDF was assayed without the use of alpha amylase, but with sodium sulphite in the NDF. Both NDF and ADF are expressed without residual ash as reported by others (34, 35).
Phenolic content of extracts
Total phenolic content of the extracts were evaluated by a colorimetric method using Folin–Ciocalteu reagent, this method is based on a redox reaction mechanism where the phenolic compounds react with the folin-cicalteu reagent and give rise to a blue coloration, which is quantifiable in spectrophotometry at 765 nm based on a straight pattern of gallic acid. In brief, 1 mL of leaf extract was dissolved in 2 mL of methanol, and 500 μL aliquots of extract were combined with 2.5 mL Folin–Ciocalteu reagent (ten-fold diluted) and 2.5 mL (75 g/L) sodium carbonate. The tubes were vortexed for 10 s and allowed to stand at 25°C for 2 h. After 2 h of incubation at 25°C, absorbance at 765 nm was measured against a reagent blank. Total phenolic content was expressed as mg of gallic acid equivalent (GAE)/g. Total flavonoid content of the extracts was evaluated by using a modified AlCl3 calorimetric method (36). In a 10 mL volumetric flask, 1 mL of leaf extract was dissolved in 2 mL of methanol. A volume of each 200 μL leaf extract was filled to a prepared glass vial. Then, 75 μL of 5% NaNO3 was added to a prepared glass vial and abruptly sealed. The sealed glass vials were then stored at temperatures of 30–35°C for 5 min. Following that, each vial was added with 1.25 mL AlCl3 and 0.5 mL NaOH. The sealed glass vials were then sonicated and incubated for 5 min at a temperature of 30–35°C. Following incubation, the absorbance of all working and standard solutions was measured at 510 nm against a methanol blank. Estimation of flavonoid content of extracts was performed using the quercetin standard calibration curve, and the flavonoid content of the extracts was represented as μg of quercetin equivalent per one gram of dry extract.
Rumen incubation
In the morning of each in vitro incubation day (06:00 h), rumen contents (2 kg/animal that included semisolid and liquid phases) were collected from four crossbreed steers (≈400 ± 20 kg body weight) for each incubation run. The ruminal contents of the four steers were collected immediately after slaughtering the animals in the city of Toluca, State of Mexico, and used as source of inocula for the in vitro incubations. Collection of the ruminal fluid is conducted as representative samples from different locations of the rumen (cranial, ventral, dorsal and caudal regions). After that, the collected ruminal contents were transferred immediately at the morning to the laboratory and mixed to get a pool rumen content sample, and then mixed with the Goering and Van Soest buffer solution (37) in a 1:4 vol/vol ratio (10 mL mixed rumen fluid and 40 mL of the buffer solution). Following that, the incubation media was subsequently mixed and strained through four layers of cheesecloth into a flask with an O2-free headspace and used to inoculate three replicates of incubation in 120-ml serum bottles containing 0.5 g substrate. The diet (substrate) was formulated according to NRC requirements for finishing cattle (the ingredients and chemical composition are shown in Table 1).
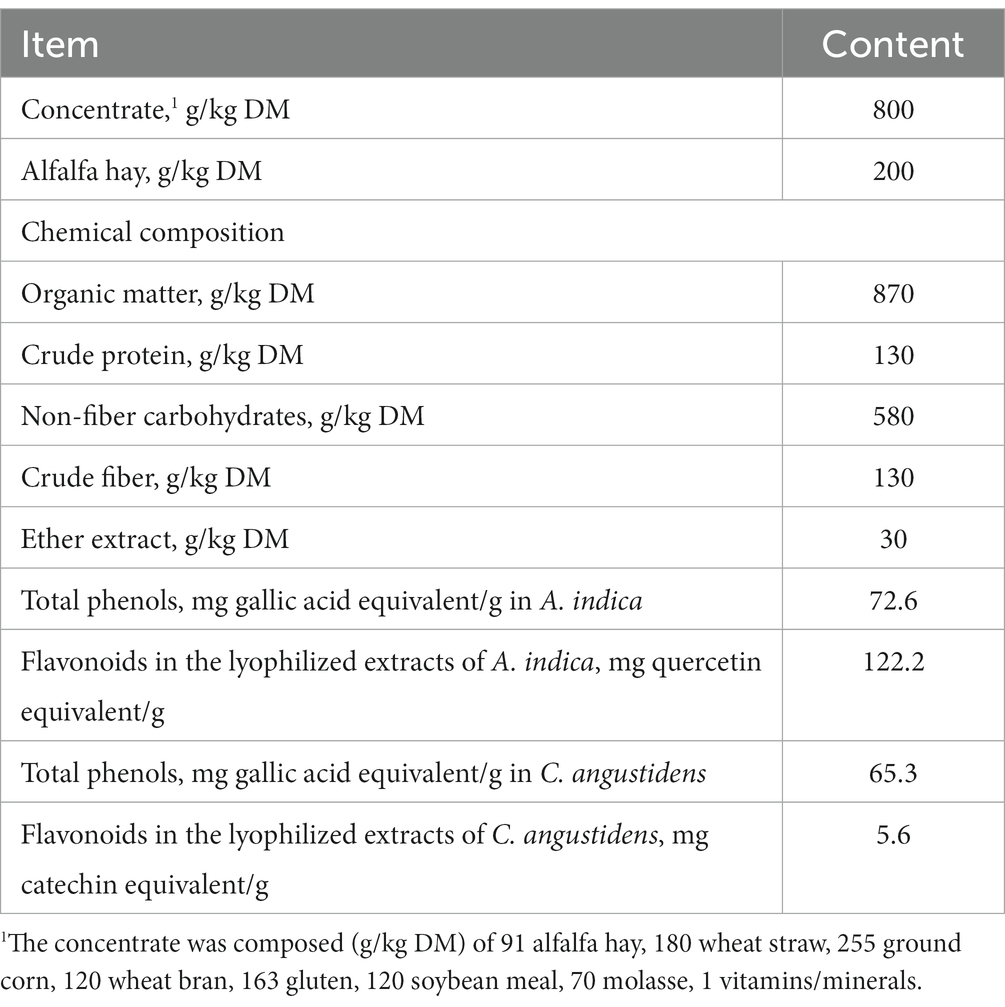
Table 1. Ingredient and chemical composition of the dietary substrate used for the in vitro fermentation.
The study was conducted as a completely randomized experimental design replicated 3 times. The following treatments were added to the substrate in four doses of extract: 0, 36, 72 and 108 mg of liquid extract per gram of DM of substrate incubated of AZI, CNA or the mixture of the aqueous extracts (Mix. 1:1 vol/vol). Three vials were also included as blanks (the bottles containing rumen fluid and the in vitro buffer solution, but with no dietary substrate). There were 3 replicates in each group, and the dosages used were based on previous knowledge on the utilization on these extracts (18). However, dosages were adjusted for the incubation with ruminal fluid and utilizing cattle dietary substrates.
All assays (substrate, extracts doses, mixture of rumen liquor with Goering and Van Soest buffer solution) were mixed and prepared immediately before in vitro incubation. Three incubation runs were performed in 9 days (3 days for each run). A total of 135 bottles (3 bottles of each triplicate sample within each of the 3 plant species extracts) (2 species and 1 mixture of both), in 4 doses of extract for each plant species, in 3 runs on different weeks, with 9 bottles as blanks per each run (i.e., 3 rumen fluid only, 3 buffer only and 3 mixed rumen fluid with buffer only) were incubated for 48 h. Once all bottles were filled, the prepared bottles were flushed with CO2 and quickly closed with rubber stoppers, shaken and placed in an incubator at a temperature of 39°C. Total gas production (psi) was recorded at 2, 4, 6, 10, 14, 21, 26, 28, 33, 37, and 48 h utilizing pressure transducer (Extech Instruments, Waltham, United States) following the technique of Theodorou et al. (38). These measurement timepoints allowed evaluation of the asymptotic gas production, the rate of gas production as well as the lag time before gas production begins. The productions of CH4, CO, and H2S were also measured at the same hours of incubation using a diffusion-based gas detector (MONITOR de Dräger Safety X-am 2,500, Lübeck, Germany) using a sample of 5 mL. After each value was recorded, using a syringe needle, the gas was dispersed to avoid gas accumulation (Figure 1). The device used consists of a portable gas detection instrument for monitoring the concentration of a variety of gases (i.e., CH4, CO, and H2S). With this method, after calibration has been performed, the sample of gas (5 mL) is collected and inserted into the instrument. Then the output results are visualized in a matrix display; data may also be downloaded in a computer for better visualization of the results. This approach for the measurement of gasses has been previously tested and its use has been reported elsewhere (39, 40).
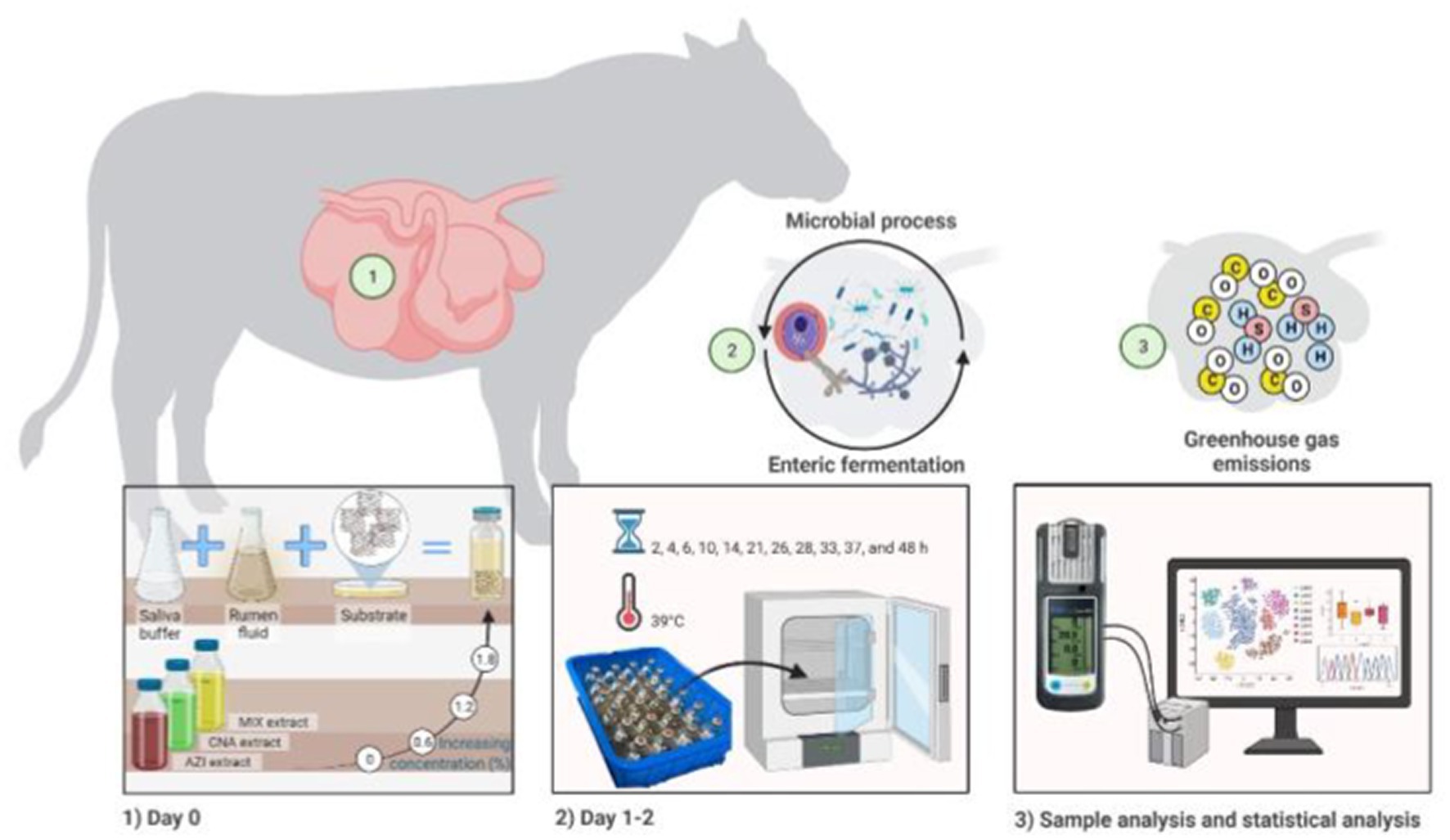
Figure 1. In vitro fermentation illustrating the synergistic role of treatment Mix consisting of Azadirachta indica and Cnidoscolus angustidens (1:1, AZI: CNA) at 72 mg extract/g DM of substrate as a feed additive contributes for reducing GHG as well as the other measured gases.
Dry matter degradation
Measurement of DM degradation was performed following the approach used by Rodriguez et al. (34). Briefly, after 48 h of incubation, bottles were uncapped and the pH was measured using a digital pH meter (Conductronic pH15, Puebla, Mexico), and the residual of each bottle was filtered through Whatman filter paper, and the bottles were rinsed thoroughly in order to collect the feed residues, similar to the approach used by Zhang et al. (41). After fermentation, residues were dried at 45°C for 72 h to estimate dry matter degradability [DMD; (37)]. The weight loss after drying was used to calculate undegradable DM. At 48 h of incubation, the DM degradability of the substrate (i.e., apparent degradation) was determined as the difference between the substrate’s DM content and its undegradable DM (34).
Calculation and statistical analysis
To estimate the kinetic parameters of gas production (GP), CH4, CO, and H2S, the results of GP, CH4, CO, and H2S (mL/g DM) were fitted using the NLIN option of SAS 9.4 to the France, Dijkstra model (42). The model is shown in the following equation:
where A is the volume of GP, CH4, CO and H2S at time t; b the asymptotic GP, CH4, CO, and H2S (mL/g DM); c is the rate of GP, CH4, CO, and H2S (/h), and lag (h) is the discrete lag time is the time prior to production of gas (GP), CH4, CO, and H2S.
Metabolizable energy (ME, MJ/kg DM) was estimated as stated by Menke et al. (25) equation as:
In vitro organic matter digestibility (OMD, g/kg OM) according to Menke and Steingass (25). The aquation was: OMD (%) = 16.49 + 0.9042 × GP + 0.0492 × CP + 0.0387 × ash.
Short chain fatty acids concentration (SCFA) was calculated according to Getachew, Makkar equation (43). The calculations are illustrated in the following equation:
where GP is the 24 h net gas production (mL/200 mg DM).
Experimental design was completely randomized with repeated measures over time. Before performing the statistical analyses, data from each of the three runs within the same sample of each of the three individual samples (AZI, CNA, and Mix.) were averaged, and the mean values of each individual sample were used as the experimental unit. Results of rumen fermentation parameters, CH4 conversion efficiency were analyzed as a factorial experiment using the PROC GLM option of SAS 9.4 equation as:
where Yij is every observation of the i plant species (Pi) with j extract dose (Ej); μ is the general mean; (P × E)ij is the interaction between plant species and extract dose; ɛijk represents the experimental error, normally distributed with the average 0 and the constant variance. Linear and quadratic polynomial contrasts were used to examine responses of greenhouse gas production and gas production of the fermented diet fermentation with the increasing addition levels (doses) of the plant species extracts. Statistical significance was declared at p < 0.05, and tendency was discussed if p > 0.05 and ≤ 0.10.
Results
Production of total Gas, CH4, CO, and H2S
The mixture of the leaf extracts (Mix.) resulted in the lowest (p < 0.05) gas produced for plant species for all the gases determined, while the dose extract at 72 mg of either AZI, CNA or Mix. produced the least (p < 0.05) gas over the 48-h incubation period (Figures 2–5A,B).
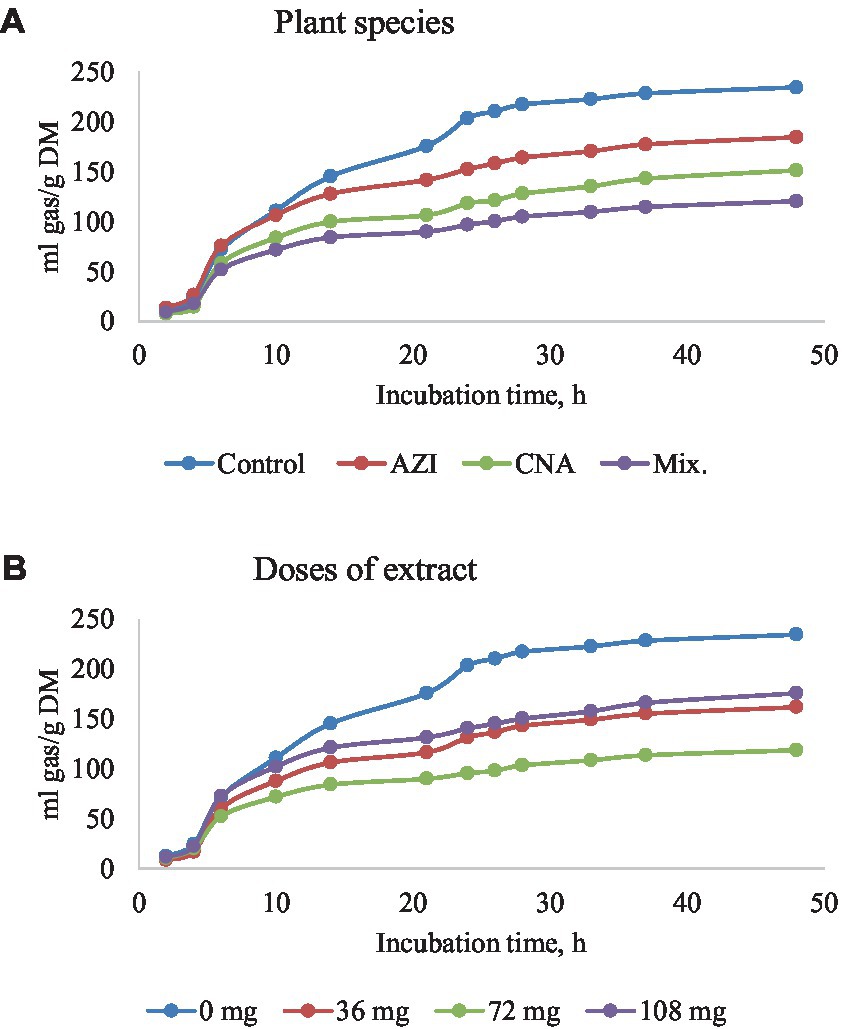
Figure 2. Rumen total gas production [mL/g dry matter (DM)] at different hours of incubation as affected by the dietary inclusion with the aqueous extract of A. indica (AZI), C. angustidens (CNA) or their combination (Mix. 1:1, vol/vol) – (A) at 0, 36, 72 and 108 mg of liquid extract/g DM (B) incubated with rumen contents slaughtered steers.
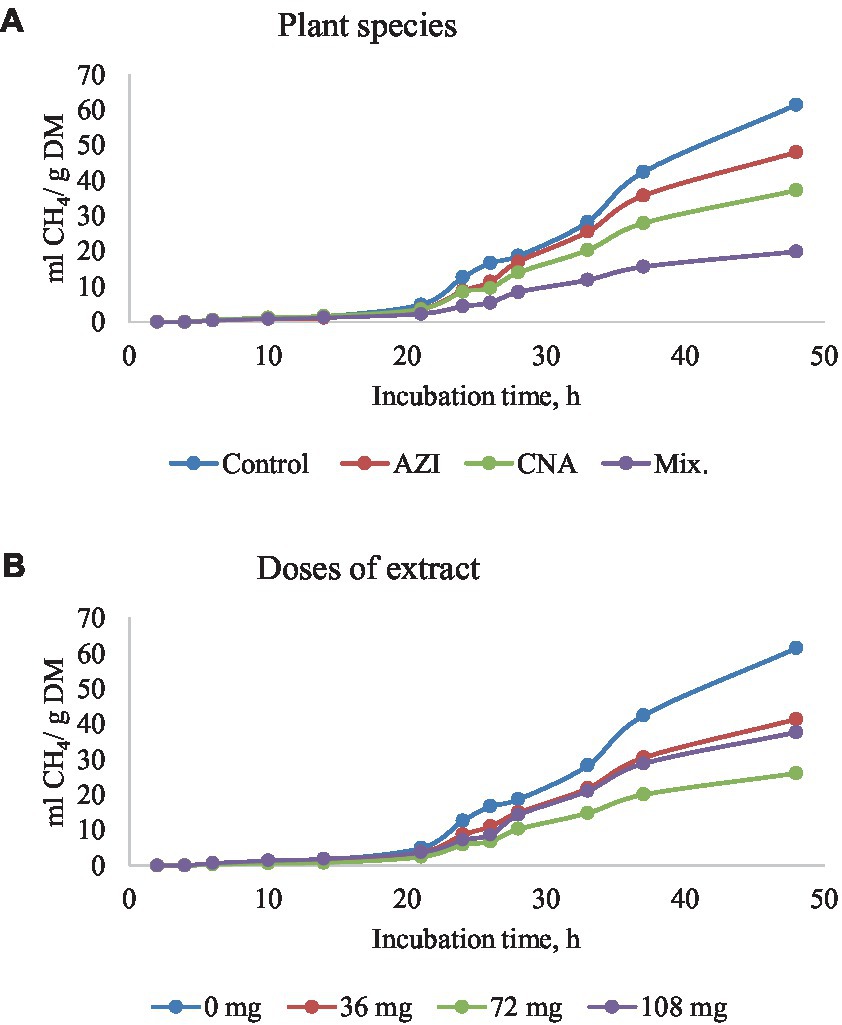
Figure 3. Rumen methane (CH4) production [mL/g dry matter (DM)] at different hours of incubation as affected by the dietary inclusion with the aqueous extract of A. indica (AZI), C. angustidens (CNA) or their combination (Mix. 1:1, vol/vol) – (A) at 0, 36, 72 and 108 mg of liquid extract/g DM (B) incubated with rumen contents slaughtered steers.
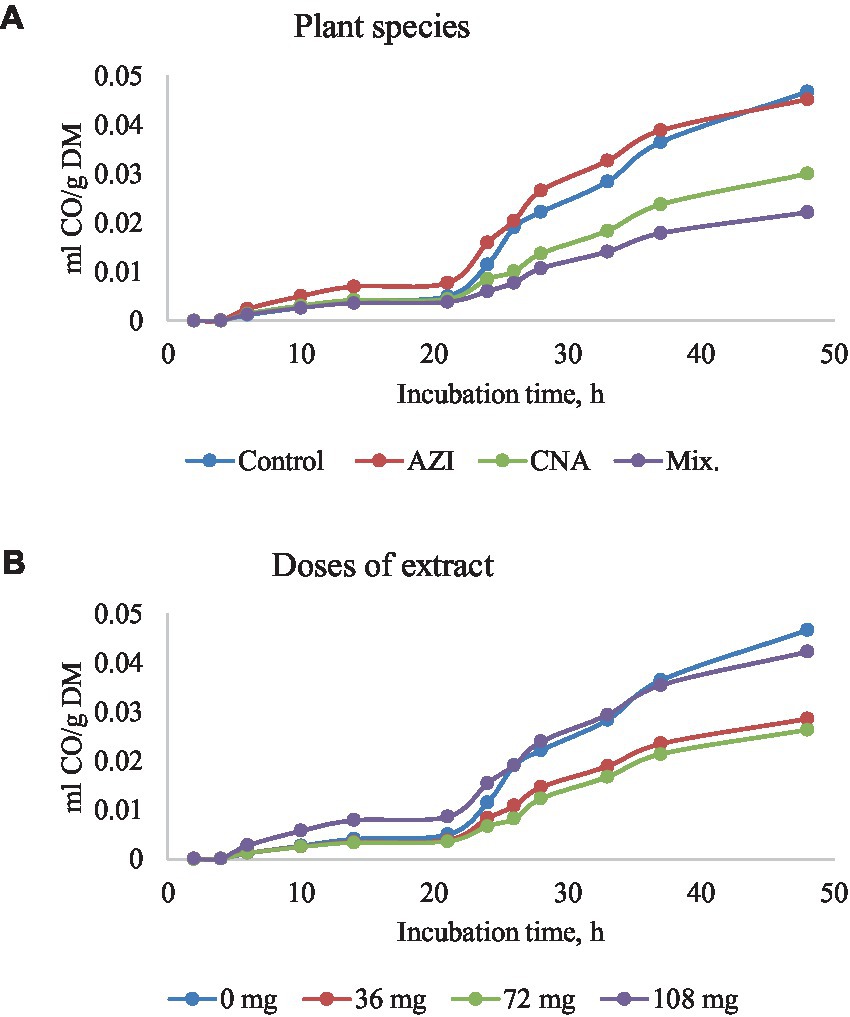
Figure 4. Rumen carbon monoxide (CO) production [mL/g dry matter (DM)] at different hours of incubation as affected by the dietary inclusion with the aqueous extract of A. indica (AZI), C. angustidens (CNA) or their combination (Mix. 1:1, vol/vol) – (A) at 0, 36, 72 and 108 mg of liquid extract/g DM (B) incubated with rumen contents slaughtered steers.
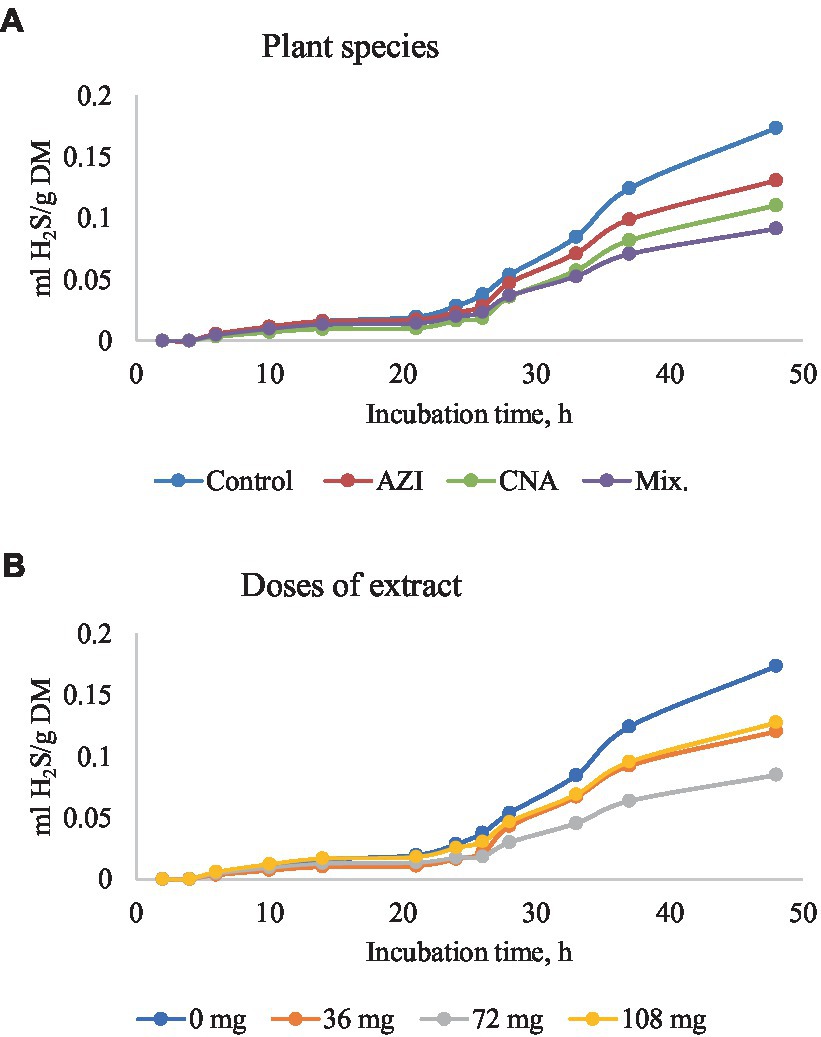
Figure 5. Rumen hydrogen sulfide (H2S) production [mL/g dry matter (DM)] at different hours of incubation as affected by the dietary inclusion with the aqueous extract of A. indica (AZI), C. angustidens (CNA) or their combination (Mix. 1:1, vol/vol) – (A) at 0, 36, 72 and 108 mg of liquid extract/g DM (B) incubated with rumen contents slaughtered steers.
The production of gas at time points 6, 24 and 48 h are shown for practicality, and because these are key timepoints that allow illustration of the fermentation kinetics throughout the evaluated fermentation timeframe. There was a significant difference on the parameters measured. An increase in period of in vitro fermentation (hour of incubation) led to an increase (p < 0.05) in gas produced. Increases in dosage and incubation elicited an increased (p < 0.05) volume of gas for AZI while CNA and Mix recorded high volumes at a low dose (36 mg) after 48; these results are shown in Table 2. We also found a quadratic effect (p < 0.05) of CNA on the asymptotic gas production and lag with the lowest values for these variables observed for the dose 72 mg/g fermentation substrate. Likewise, the asymptotic gas production was quadratically affected by Mix., with the lowest (p < 0.05) value observed for dosage 72 mg/g of fermentation substrate.
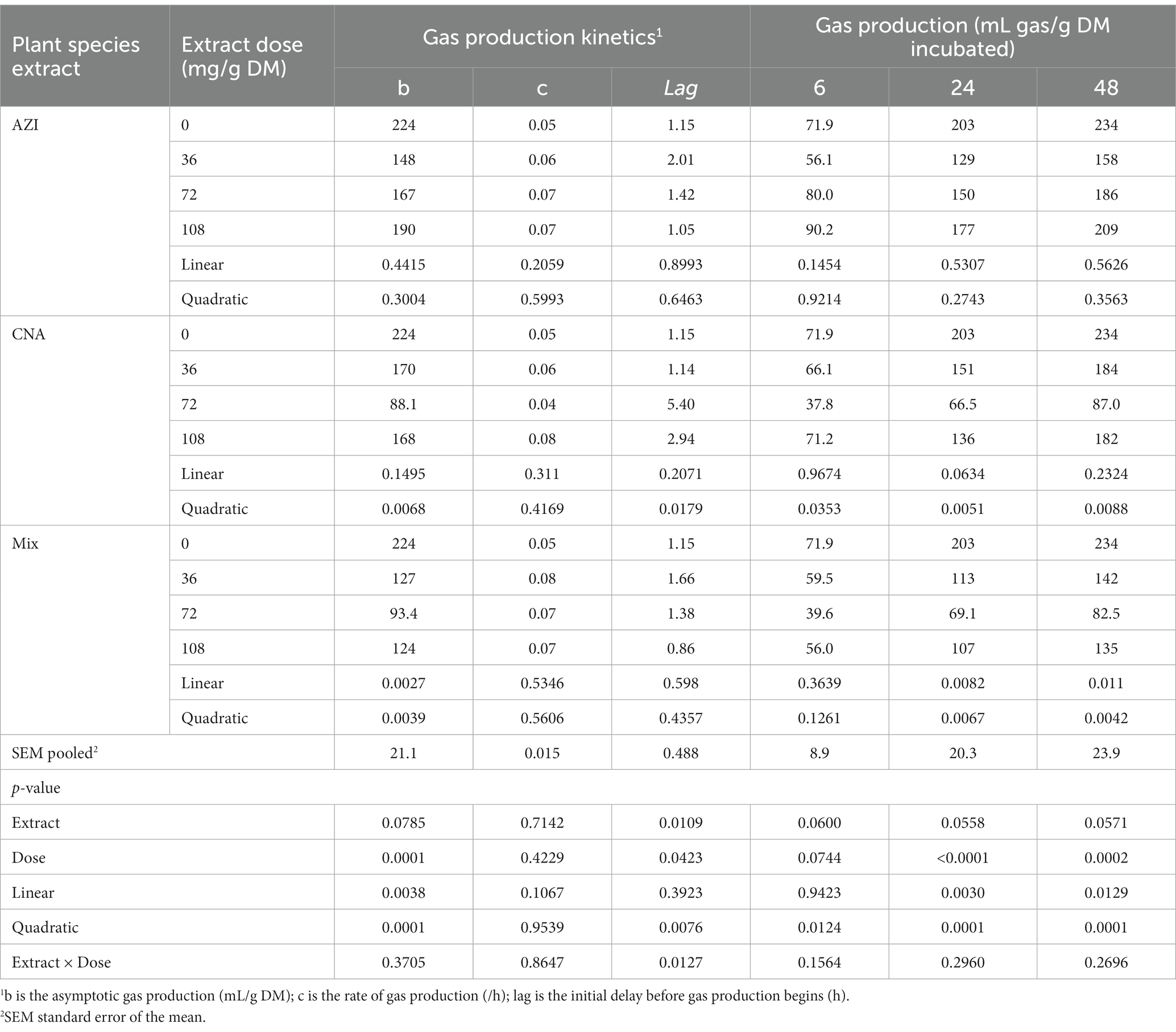
Table 2. In vitro rumen gas production kinetics and total production at 6, 24, and 48 h of the incubated diet with the leaf aqueous extract of A. indica (AZI), C. angustidens (CNA) or their combination (Mix. 1:1, vol/vol) at 0, 36, 72 and 108 mg liquid extract/g DM incubated with rumen fluid of slaughtered steers.
Similar to total gas, increased dosage resulted in increased (p < 0.05) CH4 gas for AZI while the opposite was observed in Mix. The highest (p < 0.05) CH4 was recorded for CNA at 36 mg dose. There was no significant difference for CH4 proportions when AZI increased with an increase in dosage. After 48 h, CO volume was high (p < 0.05) for AZI at 108 mg dose and least (p < 0.05) was seen for Mix. at 72 mg dose after 48 h. After 6 h, there was no H2S gas produced for all the plant species. High (p < 0.05) volume was observed for AZI after 48 h at a 108 mg dose (Tables 3–5).
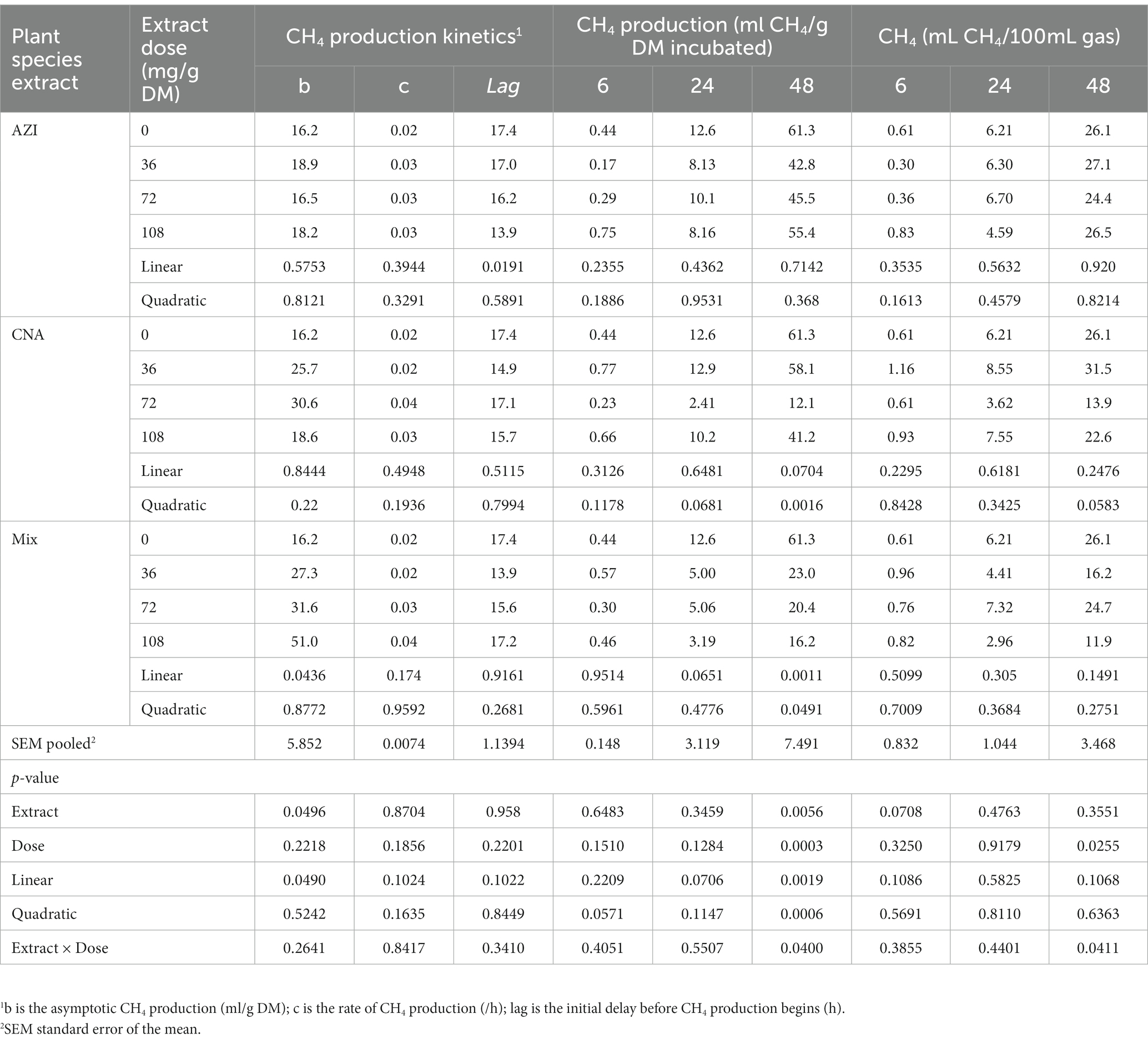
Table 3. In vitro rumen methane (CH4) production at 6, 24, and 48 h of the incubated diet with the leaf aqueous extract of A. indica (AZI), C. angustidens (CNA) or their combination (Mix. 1:1, vol/vol) at 0, 36, 72 and 108 mg of liquid extract/ g DM incubated with rumen fluid of slaughtered steers.
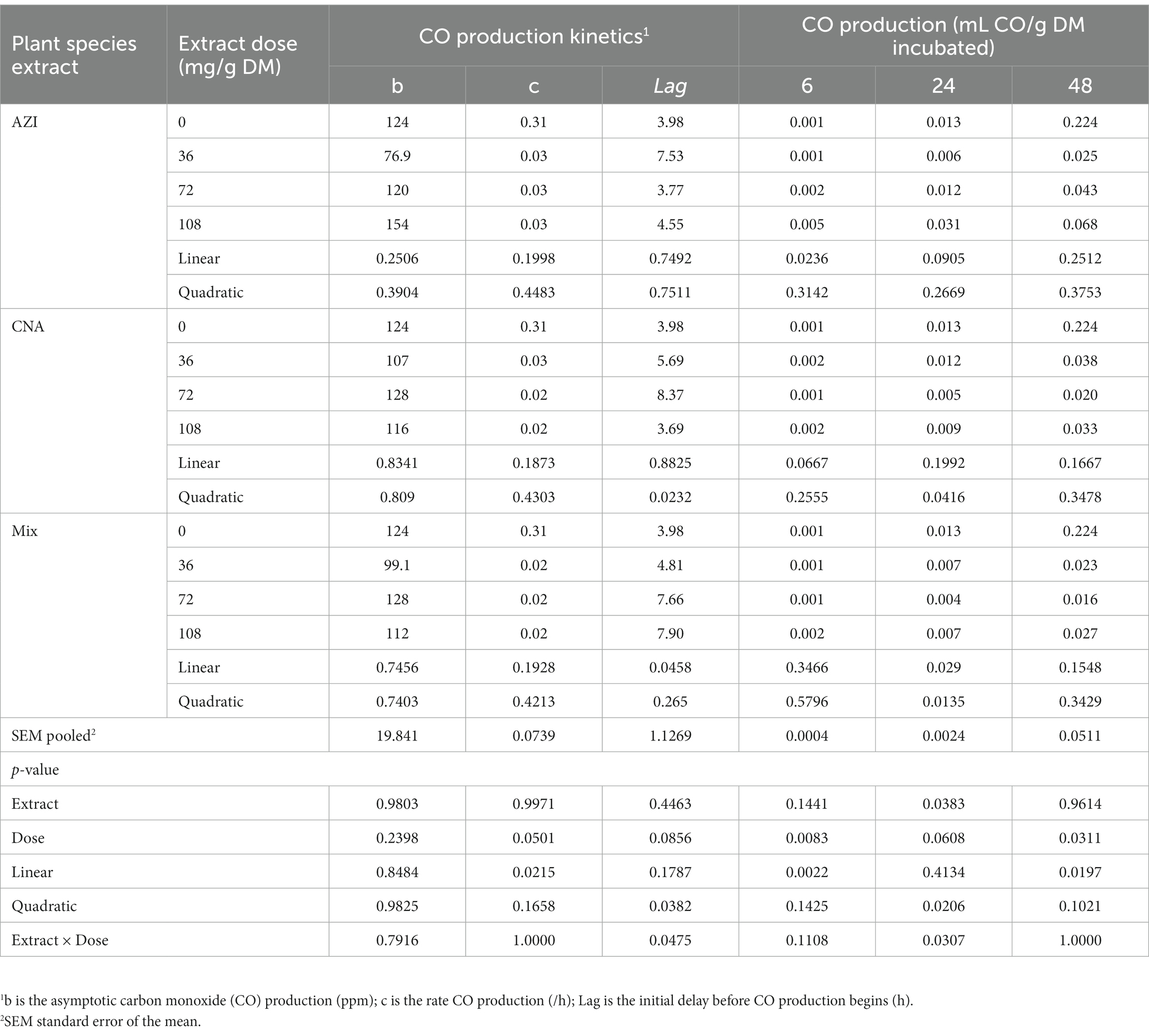
Table 4. In vitro rumen carbon monoxide (CO) production at 6, 24, and 48 h of the incubated diet with the leaf aqueous extract of A. indica (AZI), C. angustidens (CNA) or their combination (Mix. 1:1, vol/vol) at 0, 36, 72 and 108 mg of liquid extract/ g DM incubated with rumen fluid of slaughtered steers.
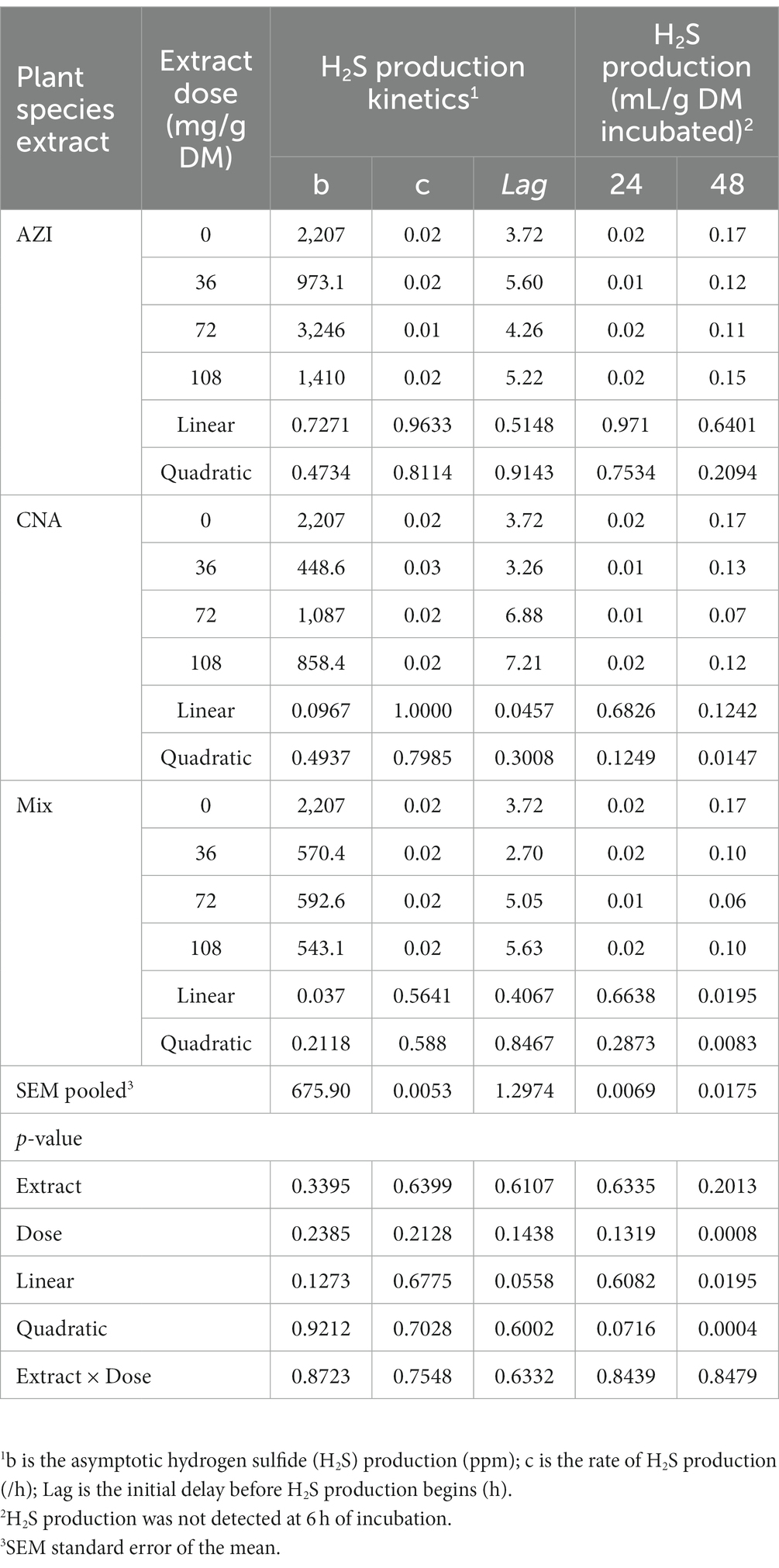
Table 5. In vitro rumen hydrogen sulfide (H2S) production at 24, and 48 h of the incubated diet with the leaf aqueous extract of A. indica (AZI), C. angustidens (CNA) or their combination (Mix. 1:1, vol/vol) at 0, 36, 72 and 108 mg of liquid extract/g DM incubated with rumen fluid of slaughtered steers.
Fermentation characteristics and CH4 conversion efficiency
The ruminal pH tended to decrease (p = 0.09) for AZI and decreased (p < 0.01) for CNA plant species as dose levels were increased. A similar trend was observed for DMD and ME in the presence of CNA. There was a significant difference (p < 0.05) at dose level for DMD and ME (Table 6). Additionally, OMD content was 67.8%. However, CH4 conversion efficiency to SCFA, ME or OM were not affected by AZI, CNA or Mix any supplemented dose levels (p ≥ 0.12).
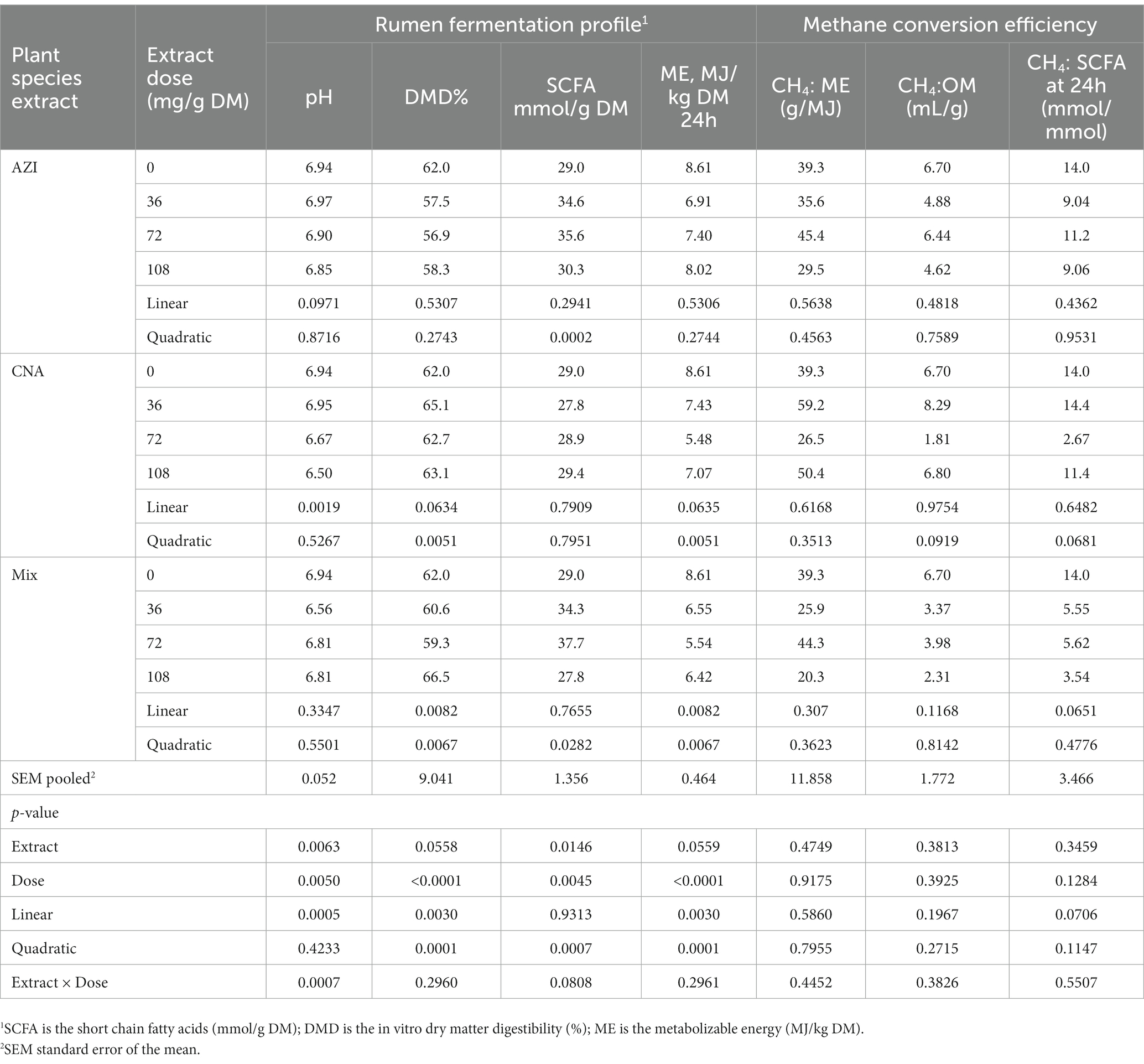
Table 6. In vitro rumen fermentation profile and methane conversion efficiency to short chain fatty acids (CH4: SCFA at 24 h, mmol/mmol), metabolizable energy [CH4: ME (g/MJ)], and organic matter [CH4: OM (ml/g)] of the dietary inclusion with different doses of A. indica (AZI), C. angustidens (CNA) or their combination (Mix. 1:1, vol/vol) at 0, 36, 72 and 108 mg of liquid extract/g DM incubated with rumen fluid of slaughtered steer.
Discussion
To the authors’ knowledge, this is the first study evaluating the efficacy of leaf extracts from AZI and CNA, or their combination (Mix. 1:1) in a formulated ruminant diet as a viable alternative for reducing GHG production under in vitro conditions. Previous investigations have included these fodder trees in ruminant diets to evaluate whether the presence of tannins and/or saponin improves animal performance (20, 44), except for enteric CH4 and other gasses produced in the rumen that may influence the production of GHG as well as other gases produced within the rumen such as CO and H2S. However, the effects observed in these studies cannot be attributed solely to condensed tannins and/or saponin due to the synergistic and antagonistic effects of complex matrices such as flavonoids and other phenolics from AZI (45) and CNA (46). Several previous studies have examined synergistic compounds in animal nutrition and physiology (44–46). However, none of these compounds were derived from AZI and CNA as in the present experiment.
In addition to the total phenols and flavonoids that were measured in the plants extract, previous researchers have reported the presence of essential oils including palmitic acid, oleic acid and linoleic acid in CNA (30) as well as azadirachtin, nimbolinin, nimbin, nimbidin, nimbidol, sodium nimbinate, gedunin, salannin, and quercetin in AZI (31). Some of these compounds have been shown to have potent antibacterial properties (31), which may have influenced the rumen bacterial community profile and activity when used in the present study. Therefore, it is possible that the chemical compounds of the extracts found in this study in combination with those previously reported by other investigators acted synergistically enhancing their effect on the ruminal microbial fermentation dynamics, which results in an increased effect for reducing the synthesis the GHG as shown by the results from this experiment.
Total gas production
Given the current global problem associated with GHG emissions, there is a growing interest in finding effective strategies to mitigate the emissions of enteric GHG from ruminants. Despite extensive research in this topic, mitigation strategies are still needed. According to our hypothesis, results from this study showed promising effects of two plant species for the mitigation of total gas production as well as GHG when included as aqueous extracts in ruminant diets in vitro.
Gas produced during in vitro fermentation reflects the extent of feed fermentation and digestion by the microbial community. Our findings indicated that insoluble feed substrate (b, asymptotic fraction) and total gas production showed a quadratic response, with these variables being reduced in the two evaluated fodder trees only when the dosages of extracts were 72 mg of liquid extract/g DM substrate. These quadratic responses in asymptotic GP with increasing levels of treatment Mix., which included the mixture of the two evaluated fodder trees suggest a potential antagonistic effect of the dose 72 mg/g DM substrate. However, greater dosages lead to a possibly synergistic effect when the plant extracts were combined. It is reasonable to assume that changes in metabolic processes in the rumen are dose-dependent. Thus, leaf extracts used in the present experiment might have an effect on the cumulative gas production after 48 h of in vitro incubation. Previously, the increase in gas volumes observed in extracts rich in condensed tannins, phenolics, and flavonoids could be attributed to improved rumen fermentation and nutrient digestibility (26). The latter study utilized an extract from Cnidoscolus aconitifolius, which was included in the ration in pelleted form and contained 2.3% of total tannins and 7.3% of total flavonoids; authors concluded that the use of these inclusion levels represents a promising approach to enhance rumen fermentation and to mitigate CH4 production. However, in the present study, AZI contained twofold the number of flavonoids (expressed as quercetin) that Totakul and coworkers used (26). This indicates that the increase in gas production can be attributed to the quercetin’s stimulatory effect on the ruminal microbes, resulting in increased activity (2). Furthermore, it has been asserted that diets containing tannins with low astringency could not negatively affect feed intake and animal growth performance, resulting in an increase in gas production as seen in AZI tree species, as reported by Waghorn (19). Previous research has demonstrated that synergistic compounds expressed as gallic acid (tannins) increase gas production as well as the dosage of tannins or flavonoids (47, 48). Thereby stimulating the growth of ruminal cellulolytic bacteria capable of fermenting organic substrates. In addition, a decrease in gas produced might be caused by the inhibitory effects of synergistic compounds such as the presence of AZI or CNA on methanogens during methanogenesis, as will be discussed further below. Therefore, results suggest that in the Mix. treatment, the combination of extracts may well have enhanced the potency of phytochemicals or synergistic compounds that each plant species contributed to the mixture, which may have resulted in the generation of new compounds that mitigated the activity of methanogens, thereby reducing the amount of total gas produced (2).
The significant increase in the concentration of individual gases after 20 h of incubation may be related to ruminal microbial dynamics. At the start of the in vitro fermentation, there is a period of time where the microbial community needs to adapt to the fermentation conditions. During this initial stage, feed degradation is minimal. However, as fermentation time increases, the microbial community completely adapts to the environment, there is increased degradation of feed as well as generation of soluble nutrients, which are taken up and fermented by the microbial community. Thus, this results in a significant increase in the concentration of individual gases, as observed in this study because fermentation and gas production are highly dependent on the availability of nutrients from substrates. However, it should be noted that the digestibility of the starch in substrates was not measured in this study. Reports have shown that starch availability for microbial fermentation may be affected not only by the type of corn processing (49), but also by several factors within a specific type of grain processing (50).
CH4 production
The initial delay before CH4 production (Lag) decreased with increasing dose levels and CH4 gas production for AZI, whereas the opposite happened for Mix. treatment. As demonstrated in the AZI plant species extract, the delay would have been reduced as a result of the extract’s high dose providing a favorable environment for methanogens to thrive, resulting in high CH4 production. On the other hand, it is reasonable to assume that a synergistic effect among phytogenic compounds in Mix. treatment, which resulted in the inhibition of methanogens from the start of the in vitro fermentation, leading to an increase of the lag phase. On average, Mix. plant species achieved a greater sustainable reduction in CH4 production (approximately 67.58%). However, the proportion of CH4 was not affected by dietary substrate, and this may be due to a uniform decrease in the production of other gases, so that the proportion of CH4 was maintained. When protozoans are reduced, methanogens are suppressed as well because they have a symbiotic relationship in the rumen, as explained by Park and Yu (51). The quadratic effect of CNA on either total gas production or CH4 production suggests that if using CNA alone, the dosage should be 72 mg/g DM (not greater or lower) in order to achieve the most beneficial effect in the mitigation of GHG production. Synergistic chemicals have been shown to reduce CH4 production more effectively (47, 48). The synergistic chemicals have been shown to have an antibacterial impact in rumen methanogenesis by interfering with gram-positive bacteria’s outer membrane (23, 52) which may have occurred with the Mix. treatment used in the present experiment. Specifically, bacteria lose control of ion gradients, electron mobilization, phosphorylation cascades, protein translocation, and other enzymatic processes as a result of this motion (53). According to Seradj, supplementation with the phytonutrient Bioflavex, which is based on flavonoids, reduced CH4 emissions (54). Though these bioactive compounds have direct effects on methanogens by suppressing CH4 production, as seen in Mix. plant species, this improves animal productivity (21, 55). However, because of the nature of this in vitro study, the effects of the evaluated treatments on animal production performance could not be determined, and this is worth considering in future experiments.
Carbon monoxide production and hydrogen sulfide production
To the author’s knowledge, the current in vitro results are the first to report on the incubation of AZI, CNA, or Mix. form, and the data indicate that incubating AZI, CNA, or Mix. form resulted in a longer delay before CO production (Lag), leading to reduced gas volumes, particularly at 7 h and above. This may also be another effect of a potential synergistic effect of the phytochemical compounds originating from the plants extract combined in Mix., which may have results in mitigation of ruminal methanogens. Thus, findings from the present study showing a reduction in CO production is consistent with the reports of Parra-Garcia et al. (56). Therefore, it can be concluded that CH4 and CO are positively related (57).
Extract of Mix. had a mitigation extent of approximately 47.51% on average, compared to CNA and AZI (36.59 and 24.71%, respectively). Indeed, the reduction in H2S gas was 62.07% at 72 mg Mix. Gerber et al. stated that the benefits of tannins consumption corresponded to reductions in N2O emissions and may have had an effect on H2S production (2). The H2 required for the formation of CH4 and H2S could have been used by other microbes or disposed in a sink (2, 58). Therefore, our results demonstrate a synergistic effect of the combination of phytochemicals in Mix. not only on GHG production, but also in associated molecules like H2S. However, given the wide range of compounds present in each plant species used in this study, further research is warranted to elucidate which specific combination of phytochemical results in the mitigation of methanogens and their byproducts during fermentation.
Fermentation characteristics and CH4 conversion efficiency
The ruminal pH ranged between 6.50 and 6.97, which is normal for rumen ecology and the efficiency of rumen fermentation (26, 47). A greater production of short chain fatty acids (SCFAs) is most likely explained by rumen digestibility when the rumen fluids were incubated with Mix. The present results could be corroborating to previous observations that higher level of dietary CP and supplementation of CNA pellets resulted in increased gas production and in vitro digestibility (26, 59). In agreement with this report, a greater DMD and GP had been observed to linearly increase of availability CP in diet (60). This is in agreement with that of AZI in this study where its gas volumes increased with increase in DMD and could be attributed to the concentrate and AZI extracts, which might have enhanced the nutrient digestibility in the rumen (61). Additionally, synergistic compounds such as tannins, phenolic acids, and flavonoids were shown to have a greater stimulatory effect on DMD and OMD, implying an increase in SCFAs and a more acidic environment (47, 48). Findings of increased DMD and ME in AZI plant species appeared to be due to increased bacterial activity (62). The low digestibility observed for the dietary substrates could be due to a slow activity of the microbiome on the substrates during the initial stage of the in vitro incubation timeframe. The measurement of DMD at a longer incubation time point when microbial activity has increased may result in greater digestibility. Values of ME obtained in this study were greater than those reported by Njidda for eight browse leaves (63). These values ranged between 3.28 and 3.84 MJ/Kg. Though gas volumes were reduced, no effect on SCFAs was observed for all plant species. According to Salem et al., phytochemicals could have an effect on rumen modification by stimulating ruminal fermentation and increasing protein metabolism and digestibility of plant cell wall constituents (64). Regrettably, neither the microbial community profile nor the SCFA fraction including protein utilization were quantified in the current in vitro study, and thus the current results do not provide additional information to speculate on the fate of rumen fermentation. Therefore, additional research is necessary to shed further light on this subject. However, this study serves as a first approach to evaluate the effects of the plant extracts on GHG, which will be the basis for future experiments where we will include the evaluation of the microbial community (protozoa, bacteria and potentially the archaeal community) as well as the profile of volatile fatty acids.
The discrepancies between the results from different research settings may be due to a multitude of factors, including the composition of the non-tannic-supplemented diet utilized in the study, fermentation substrates, flavonoid bioavailability, and animal physiological states. The current observation of increased mitigation of sustainable rumen greenhouse gas emissions (primarily enteric CH4) under in vitro conditions appeared to validate our hypothesis. Overall, it suggested that the presence of flavonoids either in the form of AZI, CNA, or Mix. modulates the activity of flavonoids and other phytochemicals, in the in vitro fermentation of a ruminant ration, which lowers ruminant greenhouse gas production and promotes a more appropriate ecosystem while having no negative effect on fermentative properties. Further studies should test similar conditions in on-farm conditions.
Conclusion
The treatment Mix. which includes a combination of the leaf extracts of the plant treatments AZI and CNA achieved the greater sustainable in vitro reduction, 67.6% in CH4 production when compared to either AZI or CNA alone. A concentration of 72 mg/g DM incubated of the mix was the best dosage for lowering in vitro ruminant GHG production while having no negative effect on total gas production. Thus, suggesting a potential synergistic effect among the phytochemical compounds from each plant species. It is important to note, however, that in vitro studies cannot always be directly extrapolated to in vivo conditions. Therefore, results should be confirmed with studies. In addition, future studies should consider evaluation of the ruminal microbial community and the complete volatile fatty acid profile.
Data availability statement
The raw data supporting the conclusions of this article will be made available by the authors, without undue reservation.
Author contributions
ME: conceptualization, methodology, formal analysis, investigation, resources, data curation, writing—review and editing, visualization, and project administration. NA-L and TA: conceptualization, methodology, writing—review and editing, supervision, project administration, and funding acquisition. UI: conceptualization, methodology, data curation, and writing—review and editing. RP: conceptualization, methodology, formal analysis, investigation, resources, data curation, writing—original draft preparation, writing—review and editing, visualization, supervision, project administration, and funding acquisition. EC-L: conceptualization writing—original draft preparation, and writing—review and editing. AS and MB-R: conceptualization, methodology, resources, data curation, writing—review and editing, supervision, project administration, and funding acquisition. All authors contributed to the article and approved the submitted version.
Funding
The authors appreciate the financial support from Universidad Autónoma del Estado de México (Project UAEM 4304/2017/CI).
Acknowledgments
Graphical abstract was created with BioRender.com (license number QN23UAOIXF).
Conflict of interest
The authors declare that the research was conducted in the absence of any commercial or financial relationships that could be construed as a potential conflict of interest.
Publisher’s note
All claims expressed in this article are solely those of the authors and do not necessarily represent those of their affiliated organizations, or those of the publisher, the editors and the reviewers. Any product that may be evaluated in this article, or claim that may be made by its manufacturer, is not guaranteed or endorsed by the publisher.
References
1. Ae, V, Az, S, Khusro, A, Ab, P, Gb, R, and Mm, E. Sustainable mitigation of fecal greenhouse gases emission from equine using safflower and fish oils in combination with live yeast culture as additives towards a cleaner ecosystem. J Clean Prod. (2020) 256:120460. doi: 10.1016/J.Jclepro.2020.120460
2. Pj, G, An, H, Henderson, B, Makkar, H, Oh, J, Lee, C, et al. Technical options for the mitigation of direct methane and nitrous oxide emissions from livestock: a review. Animal. (2013) 7 Suppl 2:220–34. doi: 10.1017/S1751731113000876
3. Usepa. Global anthropogenic non-Co2 greenhouse gas emissions: 1990–2020 (2006). U.S. Environmental Protection Agency. Available at: https://www.epa.gov/global-mitigation-non-co2-greenhouse-gases/global-non-co2-ghg-emissions-1990-2030.
4. Solomon, S, Qin, D, Manning, M, Alley, RB, Berntsen, T, Bindoff, NL, et al. Technical summary In: S Solomon, D Qin, M Manning, Z Chen, M Marquis, and KB Averyt, et al., editors. Climate change 2007: the physical science basis. Contribution of working group I to the fourth assessment report of the intergovernmental panel on climate change. Cambridge, United Kingdom And New York, Ny, USA: Cambridge University Press (2007).
5. Ka, B, Henry Janzen, H, Sm, L, Ta, M, and Sm, M. Life cycle assessment of greenhouse gas emissions from beef production in Western Canada: a case study. Agric Syst. (2010) 103:371–9. doi: 10.1016/J.Agsy.2010.03.008
6. Thoma, G, Popp, J, Nutter, D, Shonnard, D, Ulrich, R, Matlock, M, et al. Greenhouse gas emissions from Milk production and consumption in the United States: a cradle-to-grave life cycle assessment circa 2008. Int Dairy J. (2013) 31:S3–S14. doi: 10.1016/J.Idairyj.2012.08.013
7. Russell, JB. Rumen microbiology and its role in ruminant nutrition, Cornell University, College of Agriculture and Life Sciences, Ithaca NY, (2002).
8. Sm, VZ, Wj, G, Ja, A, Jr, N, Dijkstra, J, Ra, L, et al. Nitrate and sulfate: effective alternative hydrogen sinks for mitigation of Ruminal methane production in sheep. J Dairy Sci. (2010) 93:5856–66. doi: 10.3168/Jds.2010-3281
9. Em, U. Metabolic hydrogen flows in rumen fermentation: principles and possibilities of interventions. Front Microbiol. (2020) 11:589. doi: 10.3389/Fmicb.2020.00589
10. Jb, R, and Jl, J. Effect of carbon monoxide on fermentation of Fiber, starch, and amino acids by mixed rumen microorganisms in vitro. Appl Environ Microbiol. (1984) 48:211–7. doi: 10.1128/Aem.48.1.211-217.1984
11. Purba, R, Paengkoum, S, and Paengkoum, P. Development of a simple high-performance liquid chromatography-based method to quantify synergistic compounds and their composition in dried leaf extracts of Piper Sarmentosum Roxb. Separations. (2021) 8:152. doi: 10.3390/Separations8090152
12. Jq, F, Mc, H, Bm, R, Jm, T, and Kebreab, E. Enteric methane mitigation interventions. Transl. Anim Sci. (2022) 6:1–16. doi: 10.1093/Tas/Txac041
13. Sinz, S, Marquardt, S, Cr, S, Braun, U, Liesegang, A, and Kreuzer, M. Phenolic plant extracts are additive in their effects against in vitro Ruminal methane and Ammonia formation. Asian Australas J Anim Sci. (2019) 32:966–76. doi: 10.5713/Ajas.18.0665
14. Suong, N, Paengkoum, S, Schonewille, J, Ra, P, and Paengkoum, P. Growth performance, blood biochemical indices, rumen bacterial community, and carcass characteristics in goats fed anthocyanin-rich black cane silage. Front Vet Sci. (2022) 9:880838. doi: 10.3389/Fvets.2022.880838
15. Suong, N, Paengkoum, S, Az, S, Paengkoum, P, and Ra, P. Silage fermentation quality, anthocyanin stability, and in vitro rumen fermentation characteristic of ferrous sulfate Heptahydrate-treated black cane (Saccharum Sinensis R.). Front Vet Sci. (2022) 9:896270. doi: 10.3389/Fvets.2022.896270
16. Ra, P, Suong, N, Paengkoum, S, Jt, S, and Paengkoum, P. Dietary inclusion of anthocyanin-rich black cane silage treated with ferrous sulfate Heptahydrate reduces oxidative stress and promotes tender meat production in goats. Front Vet Sci. (2022) 9:969321. doi: 10.3389/Fvets.2022.969321
17. Mn, H. Dietary manipulation: a sustainable way to mitigate methane emissions from ruminants. J Anim Sci Technol. (2018) 60:15. doi: 10.1186/S40781-018-0175-7
18. Alvarado, T, Mm, E, Nj, E, Alcala-Canto, Y, Ae, V, Eb, P, et al. Influence of Azadirachta Indica and Cnidoscolus Angustidens dietary extracts on equine fecal greenhouse gas emissions. J Equine Vet Sci. (2022) 116:104049. doi: 10.1016/J.Jevs.2022.104049
19. Waghorn, G. Beneficial and detrimental effects of dietary condensed tannins for sustainable sheep and goat production—Progress and challenges. Anim Feed Sci Technol. (2008) 147:116–39. doi: 10.1016/J.Anifeedsci.2007.09.013
20. Viennasay, B, and Wanapat, M. Strategic supplementation of Flemingia silage to enhance rumen fermentation efficiency, microbial protein synthesis and methane mitigation in beef cattle. BMC Vet Res. (2020) 16:480. doi: 10.1186/S12917-020-02703-X
21. Nowak, B, Moniuszko-Szajwaj, B, Skorupka, M, Puchalska, J, Kozłowska, M, Bocianowski, J, et al. Effect of Paulownia leaves extract levels on in vitro Ruminal fermentation, microbial population, methane production, and fatty acid biohydrogenation. Molecules (2022) 27:4288. doi: 10.3390/molecules27134288
22. Flachowsky, G, and Lebzien, P. Effects of phytogenic substances on rumen fermentation and methane emissions: a proposal for a research process. Anim Feed Sci Technol. (2012) 176:70–7. doi: 10.1016/J.Anifeedsci.2012.07.009
23. Suong, N, Paengkoum, S, Purba, R, and Paengkoum, P. Optimizing anthocyanin-rich black cane (Saccharum Sinensis Robx.) silage for ruminants using molasses and Iron Sulphate: a sustainable alternative. Fermentation. (2022) 8:248. doi: 10.3390/Fermentation8060248
24. Fievez, V, Oj, B, and Demeyer, D. Estimation of direct and indirect gas production in syringes: a tool to estimate short chain fatty acid production that requires minimal laboratory facilities. Anim Feed Sci Technol. (2005) 123:197–210. doi: 10.1016/J.Anifeedsci.2005.05.001
25. Menke, K, Raab, L, Salewski, A, Steingass, H, Fritz, D, and Schneider, W. The estimation of the digestibility and Metabolizable energy content of ruminant Feedingstuffs from the gas production when they are incubated with rumen liquor in vitro. J Agric Sci. (1979) 93:217–22. doi: 10.1017/S0021859600086305
26. Totakul, P, Matra, M, Sommai, S, and Wanapat, M. Cnidoscolus Aconitifolius leaf pellet can manipulate rumen fermentation characteristics and nutrient degradability. Anim Biosci. (2021) 34:1607–15. doi: 10.5713/Ab.20.0833
27. Fs, J-P, Salem, A, Mejia-Hernández, P, González-Ronquillo, M, Albarrán-Portillo, B, Rojo-Rubio, R, et al. Influence of individual and mixed extracts of two tree species on in vitro gas production kinetics of a high concentrate diet fed to growing lambs. Livest Sci. (2011) 136:192–200. doi: 10.1016/J.Livsci.2010.09.008
28. Ae, K, Uy, A, Ak, P, and Editorial, VZ. The use of phytogenic feed additives to enhance productivity and health in ruminants. Front Vet Sci. (2021) 8:685262. doi: 10.3389/Fvets.2021.685262
29. Hassan, F-U, Ma, A, Hm, E, Ms-U, R, Ms, K, Shahid, S, et al. Phytogenic additives can modulate rumen microbiome to mediate fermentation kinetics and Methanogenesis through exploiting diet-microbe interaction. Front Vet Sci. (2020) 7:575801. doi: 10.3389/Fvets.2020.575801
30. Jl, LL-DL, Troyo-Diéguez, E, Ortega-Nieblas, M, and López-Gutiérrez, F. ‘Caribe’ (Cnidoscolus Angustidens Torr.), a promising oilseed geophyte from north-West Mexico. J Arid Environ. (1999) 41:299–308. doi: 10.1006/Jare.1998.0473
31. Ma, A. Therapeutics role of Azadirachta Indica (Neem) and their active constituents in diseases prevention and treatment. Evid Based Complement Alternat Med. (2016) 2016:7382506. doi: 10.1155/2016/7382506
32. AOAC. Total dietary Fiber in foods - enzymatic-gravimetric method. Official method 985.29 (45.4.07). Aoac official methods of analysis. Association of official analytical chemists international. Gaithersburg, Md, USA: AOAC (2005).
33. Paengkoum, S, Tatsapong, P, Taethaisong, N, Sorasak, T, Ra, P, and Paengkoum, P. Empirical evaluation and prediction of protein requirements for maintenance and growth of 18-24 months old Thai swamp buffaloes. Animals. (2021) 11:1405. doi: 10.3390/Ani11051405
34. Mp, R, Mariezcurrena, M, Ma, M, Bc, L, Mm, E, Am, K, et al. Influence of live cells or cells extract of Saccharomyces Cerevisiae on in vitro gas production of a Total mixed ration. Ital J Anim Sci. (2015) 14:3713. doi: 10.4081/Ijas.2015.3713
35. Vorlaphim, T, Paengkoum, P, Ra, P, Yuangklang, C, Paengkoum, S, and Jt, S. Treatment of Rice stubble with Pleurotus Ostreatus and urea improves the growth performance in slow-growing goats. Animals. (2021) 11:1053. doi: 10.3390/Ani11041053
36. Al-Owaisi, M, Al-Hadiwi, N, and Sa, K. Gc-Ms analysis, determination of Total Phenolics, flavonoid content and free radical scavenging activities of various crude extracts of Moringa Peregrina (Forssk.) Fiori leaves. Asian Pac J Trop Biomed. (2014) 4:964–70. doi: 10.12980/Apjtb.4.201414b295
37. Hk, Goering, and Pj, Van Soest. Feeds, analysis, and forage plants (1970). Available at: https://naldc.nal.usda.gov/download/CAT87209099/PD
38. Mk, T, Ba, W, Ms, D, Ab, M, and France, J. A simple gas production method using a pressure transducer to determine the fermentation kinetics of ruminant feeds. Anim Feed Sci Technol. (1994) 48:185–97. doi: 10.1016/0377-8401(94)90171-6
39. Martinez-Fernandez, G, Duval, S, Kindermann, M, Hj, S, Se, D, and Cs, M. 3-Nop Vs. halogenated compound: methane production, Ruminal fermentation and microbial community response in forage fed cattle. Front Microbiol. (2018) 9:1582. doi: 10.3389/Fmicb.2018.01582
40. Vahidpour, F, Alghazali, Y, Akca, S, Hommes, G, and Mj, S. An enzyme-based Interdigitated electrode-type biosensor for detecting low concentrations of H2o2 vapor/aerosol. Chemosensors. (2022) 10:202. doi: 10.3390/Chemosensors10060202
41. Xm, Z, Wang, M, Yu, Q, Zy, M, Ka, B, Wang, R, et al. Liquid hot water treatment of Rice straw enhances anaerobic degradation and inhibits methane production during in vitro Ruminal fermentation. J Dairy Sci. (2020) 103:4252–61. doi: 10.3168/Jds.2019-16904
42. France, J, Dijkstra, J, Ms, D, Lopez, S, and Bannink, A. Estimating the extent of degradation of ruminant feeds from a description of their gas production profiles observed in vitro: derivation of models and other mathematical considerations. Br J Nutr. (2000) 83:143–50. doi: 10.1017/S0007114500000180
43. Getachew, G, Hp, M, and Becker, K. Tropical browses: contents of phenolic compounds, in vitro gas production and stoichiometric relationship between short chain fatty acid and in vitro gas production. J Agric Sci. (2002) 139:341–52. doi: 10.1017/S0021859602002393
44. Ban, C, Paengkoum, S, Yang, S, Tian, X, Thongpea, S, Ra, P, et al. Feeding meat goats Mangosteen (Garcinia Mangostana L.) Peel rich in condensed tannins, flavonoids, and Cinnamic acid improves growth performance and plasma antioxidant activity under tropical conditions. J Appl Anim Res. (2022) 50:307–15. doi: 10.1080/09712119.2022.2068557
45. Saleem, S, Muhammad, G, Ma, H, and Sn, B. A comprehensive review of phytochemical profile, bioactives for pharmaceuticals, and pharmacological attributes of Azadirachta Indica. Phytother Res. (2018) 32:1241–72. doi: 10.1002/Ptr.6076
46. De, O-JR, Ca, F, De, OA, Cs, A, Lf, O, Picot, L, et al. Phytochemical and pharmacological aspects of Cnidoscolus Pohl species: a systematic review. Phytomedicine. (2018) 50:137–47. doi: 10.1016/J.Phymed.2017.08.017
47. Ra, P, Paengkoum, S, Yuangklang, C, and Paengkoum, P. Flavonoids and their aromatic derivatives in Piper Betle powder promote in vitro methane mitigation in a variety of diets. Ciênc Agrotecnologia. (2020) 44:12420. doi: 10.1590/1413-7054202044012420
48. Ra, P, Yuangklang, C, and Paengkoum, P. Enhanced conjugated linoleic acid and biogas production after Ruminal fermentation with Piper Betle L. Supplementation. Cienc Rural. (2020) 50:91001. doi: 10.1590/0103-8478cr20191001
49. Yu, P, Jt, H, Fa, S, Jm, S, and Cb, T. Effects of ground, steam-flaked, and steam-rolled corn grains on performance of lactating cows. J Dairy Sci. (1998) 81:777–83. doi: 10.3168/Jds.S0022-0302(98)75634-6
50. Ef, S, Me, H, Thomson, D, Ci, V, Sj, B, and Reinhardt, C. A survey of starch availability of steam-flaked corn in commercial feedlots evaluating roll size and flake density. Prof Anim Sci. (2016) 32:550–60. doi: 10.15232/Pas.2015-01496
51. Park, T, and Yu, Z. Do Ruminal ciliates select their preys and prokaryotic Symbionts? Front Microbiol. (2018) 9:1710. doi: 10.3389/Fmicb.2018.01710
52. Patra Ak, YZ, Effects Of Adaptation Of In Vitro Rumen Culture To Garlic Oil. Nitrate, and Saponin and their combinations on Methanogenesis, fermentation, and abundances and diversity of microbial populations. Front Microbiol. (2015) 6:1434. doi: 10.3389/Fmicb.2015.01434
53. Ultee, A, Bennik, M, and Moezelaar, R. The phenolic hydroxyl group of Carvacrol is essential for action against the food-borne pathogen Bacillus Cereus. Appl Environ Microbiol. (2002) 68:1561–8. doi: 10.1128/Aem.68.4.1561-1568.2002
54. Seradj, Ahmad RezaStudy the effect of inclusion of feed flavonoid substances on animal performance and Ruminal fermentation in calves. Universitat De Lleida (2015). Available at: Http://Hdl.Handle.Net/10803/288291
55. Huang, H, Lechniak, D, Szumacher-Strabel, M, Ak, P, Kozłowska, M, Kolodziejski, P, et al. The effect of ensiled Paulownia leaves in a high-forage diet on Ruminal fermentation, methane production, fatty acid composition, and Milk production performance of dairy cows. J Anim Sci Biotechnol. (2022) 13:104. doi: 10.1186/S40104-022-00745-9
56. Parra-Garcia, A, Mm, E, Greiner, R, Barbabosa-Pliego, A, Lm, C-D, and Az, S. Effects of Moringa Oleifera leaf extract on Ruminal methane and carbon dioxide production and fermentation kinetics in a steer model. Environ Sci Pollut Res Int. (2019) 26:15333–44. doi: 10.1007/S11356-019-04963-Z
57. Zheng, C, Ma, J, Liu, T, Wei, B, and Yang, H. Effects of Mannan oligosaccharides on gas emission, protein and energy utilization, and fasting metabolism in sheep. Animals. (2019) 9:741. doi: 10.3390/Ani9100741
58. Ra, P, Suong, N, Paengkoum, S, Paengkoum, P, and Liang, J. Iron sulfate and molasses treated anthocyanin-rich black cane silage improves growth performance, rumen fermentation, antioxidant status, and meat tenderness in goats. Anim Biosci. (2023) 36:218–28. doi: 10.5713/Ab.22.0252
59. Norrapoke, T, Wanapat, M, Cherdthong, A, Kang, S, Phesatcha, K, and Pongjongmit, T. Improvement of nutritive value of cassava pulp and in vitro fermentation and microbial population by urea and molasses supplementation. J Appl Anim Res. (2018) 46:242–7. doi: 10.1080/09712119.2017.1288630
60. Anantasook, N, and Wanapat, M. Influence of rain tree pod meal supplementation on Rice straw based diets using in vitro gas fermentation technique. Asian-Australas J Anim Sci. (2012) 25:325–34. doi: 10.5713/Ajas.2011.11131
61. Promkot, C, and Wanapat, M. Effect of level of crude protein and use of cottonseed meal in diets containing cassava chips and Rice straw for lactating dairy cows. Asian Australas J Anim Sci. (2005) 18:502–11. doi: 10.5713/Ajas.2005.502
62. Naseri, V, Hozhabri, F, and Kafilzadeh, F. Assessment of in vitro digestibility and fermentation parameters of alfalfa Hay-based diet following direct incorporation of fenugreek seed (Trigonella Foenum) and Asparagus root (Asparagus Officinalis). J Anim Physiol Anim Nutr (Berl). (2013) 97:773–84. doi: 10.1111/J.1439-0396.2012.01318.X
63. Aa, N. Determining dry matter degradability of some semi-arid browse species of north-eastern Nigeria using the in vitro technique. Nig J Bas App Sci. (2011) 18:18. doi: 10.4314/Njbas.V18i2.64306
Keywords: in vitro gas production, greenhouse gases, plant polyphenols, fermentative characteristics, cattle
Citation: Elghandour MMMY, Acosta-Lozano N, Alvarado TD, Castillo-Lopez E, Cipriano-Salazar M, Barros-Rodríguez M, Inyang UA, Purba RAP and Salem AZM (2023) Influence of Azadirachta indica and Cnidoscolus angustidens aqueous extract on cattle ruminal gas production and degradability in vitro. Front. Vet. Sci. 10:1090729. doi: 10.3389/fvets.2023.1090729
Edited by:
Yosra Ahmed Soltan, Alexandria University, EgyptReviewed by:
Sameh A. Abdelnour, Zagazig University, EgyptAnusorn Cherdthong, Khon Kaen University, Thailand
Amr Salah Morsy, City of Scientific Research and Technological Applications, Egypt
Copyright © 2023 Elghandour, Acosta-Lozano, Alvarado, Castillo-Lopez, Cipriano-Salazar, Barros-Rodríguez, Inyang, Purba and Salem. This is an open-access article distributed under the terms of the Creative Commons Attribution License (CC BY). The use, distribution or reproduction in other forums is permitted, provided the original author(s) and the copyright owner(s) are credited and that the original publication in this journal is cited, in accordance with accepted academic practice. No use, distribution or reproduction is permitted which does not comply with these terms.
*Correspondence: Abdelfattah Z. M. Salem, c2FsZW1AdWFlbWV4Lm14; Marcos Barros-Rodríguez, bWFfYmFycm9zckB5YWhvby5lcw==