- 1Infection Medicine, Deanery of Biomedical Sciences, College of Medicine and Veterinary Medicine, University of Edinburgh, Edinburgh, United Kingdom
- 2School of Medicine, Kabale University, Kabale, Uganda
- 3Zhejiang University-University of Edinburgh Institute, Zhejiang University School of Medicine, Zhejiang University, Haining, China
Background: African animal trypanocide resistance (AATr) continues to undermine global efforts to eliminate the transmission of African trypanosomiasis in endemic communities. The continued lack of new trypanocides has precipitated drug misuse and overuse, thus contributing to the development of the AATr phenotype. In this study, we investigated the threat associated with AATr by using the major globally available chemotherapeutical agents.
Methods: A total of seven electronic databases were screened for an article on trypanocide resistance in AATr by using keywords on preclinical and clinical trials with the number of animals with treatment relapse, days taken to relapse, and resistant gene markers using the PRISMA checklist. Data were cleaned using the SR deduplicator and covidence and analyzed using Cochrane RevMan®. Dichotomous outputs were presented using risk ratio (RR), while continuous data were presented using the standardized mean difference (SMD) at a 95% confidence interval.
Results: A total of eight publications in which diminazene aceturate (DA), isometamidium chloride (ISM), and homidium chloride/bromide (HB) were identified as the major trypanocides were used. In all preclinical studies, the development of resistance was in the order of HB > ISM > DA. DA vs. ISM (SMD = 0.15, 95% CI: −0.54, 0.83; I2 = 46%, P = 0.05), DA vs. HB (SMD = 0.96, 95% CI: 0.47, 1.45; I2 = 0%, P = 0.86), and HB vs. ISM (SMD = −0.41, 95% CI: −0.96, 0.14; I2 = 5%, P = 0.38) showed multiple cross-resistance. Clinical studies also showed evidence of multi-drug resistance on DA and ISM (RR = 1.01, 95% CI: 0.71–1.43; I2 = 46%, P = 0.16). To address resistance, most preclinical studies increased the dosage and the treatment time, and this failed to improve the patient's prognosis. Major markers of resistance explored include TbAT1, P1/P2 transporters, folate transporters, such as F-I, F-II, F-III, and polyamine biosynthesis inhibitors. In addition, immunosuppressed hosts favor the development of AATr.
Conclusion: AATr is a threat that requires a shift in the current disease control strategies in most developing nations due to inter-species transmission. Multi-drug cross-resistance against the only accessible trypanocides is a major public health risk, justifying the need to revise the policy in developing countries to promote control of African trypanosomiasis.
1. Introduction
African animal trypanosomiasis (AAT) is caused by infection with parasitic protozoa of the genus Trypanosoma (1). Major Trypanosoma species belongs to T. brucei (T. b.) brucei, T. evansi, T. congolense, and T. vivax [see Ref. (2) on AAT]. The major challenge associated with the elimination of AAT is the development of drug resistance, namely, African animal trypanocide resistance (AATr) (3–5). Previously, resistance was associated with mutations at particular loci in the pathogen's genome. For example, mutations in the TbAT1 and purine transporters (P1/P2) were associated with an increased resistance phenotype in T. brucei isolates (6). Unfortunately, scant attention has been given to the development of novel therapies to address AATr with most funding being pledged for the control of human African trypanosomiasis (HAT). The World Health Organization (WHO) has a target of 2030 to eliminate the transmission of HAT [in particular, gHAT (7)]; however, livestock will continue to be sources of reinfection in endemic communities since these will continue to act as reservoirs of infection (8–10). In developing countries, especially in Africa, the continuous lack of capacity to develop novel therapies against AAT has led to a high reliance on foreign aid and little effort to promote local institutional research to explore novel therapeutical options and build capacity to invest and develop infrastructure in trypanosome chemotherapeutics (11, 12).
Major trypanocides used in Africa include diminazene aceturate (DA), isometamidium chloride (ISM), and homidium bromide/chloride (HB) (13). The established curative properties for DA are 3.5 mg/kg, ISM at 0.5 mg/kg, and HB at 0.02 mg/kg, all administered intramuscularly in cattle (14). The intramuscular route is preferred since most farmers in African farming communities do not have the crushes to effectively restrain the animals before drug administration, leading to the common practice of injecting animals while in motion within a traditional African village enclosure made of a fence (15). Commonly used trypanocides for prophylactic purposes are ISM/HB due to their slow release, leading to their common usage with DA to widen their spectrum of action. This is important since ISM is ineffective against T. evansi (16). DA absorption is mainly through the P2/TbAT1 transporters, while ISM has also been associated with mitochondrial F1F0-ATP synthase activity (17, 18). Other less commonly used trypanocides in AAT include aminoquinaldine (quinapyramine sulfate), which interrupts mitochondrial activity in the parasites while melarsomine dihydrochloride arsenic is hydrophilic and highly trypanocidal (17, 19). Loss of P2/TbAT1 receptors in the pathogen is a cornerstone of AATr. Mutations in the expression of mitochondrial proteins, namely, F1F0-ATP synthase, result in reduced ISM absorption by Trypanosoma species. Furthermore, increased expression of efflux pumps (20) is responsible for increased resistance although studies on this continue to be scarce. The objective of the current study was to quantify evidence on the development of AATr with an emphasis on preclinical and clinical studies.
2. Materials and methods
In this study, emphasis was placed on experimental and qualitative studies conducted on African animal trypanocide resistance. Electronic databases, such as EMBASE, PubMed, Web of Science, CABI Abstracts, and CABI Global Health, were searched for publications while gray literature from an advanced Google Search using the WHO, African Union, Food and Agriculture Organization (FAO), Organization for Animal Health [OIE, currently renamed World Organization for Animal Health (WOAH)], Drugs for Neglected Disease International (DNDI), Kenya Medical Research Institute (KEMRI), Coordinating Center for Trypanosomes in Uganda (COCTU), United States Center for Disease Control (CDC), and United States AID (USAID) websites were added. The following search terms used included: “African trypanosomiasis/ or T. brucei/ or trypanosomiasis/ or tsetse fly-borne diseases/ OR bovine trypanosomiasis or Trypanosoma or T. brucei).mp. OR tsetse fly.mp. OR glossina.mp.” AND “T. brucei brucei.mp. OR exp T. vivax/OR exp T. congolense/ OR T. evansi.mp” AND “trypanocides/ or diminazene/ or homidium bromide/ or isometamidium chloride/ or melarsomine/ or quinapyramine/AND trypano* resistance” (see Supplementary material 1).
A total of 54 publications were acquired from the search, that is, 11 publications from Ovid, 2 from NCBI, 37 from Web of Science, and 4 from Google Search by searching several databases up to 4 January 2022 (Table 1).
Publications acquired through this search (Table 1) were subsequently reviewed for relevance based on keywords, title, and abstract (by KIK and ETM). The selected publications were thoroughly screened by the SR deduplicator to remove duplications and scored for relevance by independent scholars (ETM and SCW). After resolving publication conflicts, the selected publications were searched for the full text and subsequent analysis using the PRISMA checklist (Figure 1). Furthermore, three more publications were identified by reviewing references in review articles and one from Google (n = 4), which were included among the papers eliminated during the data cleaning process (Figure 1) to generate a total of 54 research publications included in this systematic review. From the database registries, only 20 out of 50 publications were included after removing duplicates, ethnopharmacology articles, and review articles. From Google Search, only one out of four articles was added, while from the citation reviews within the review articles, three articles were included, leading to a total of 24 articles included for data extraction.
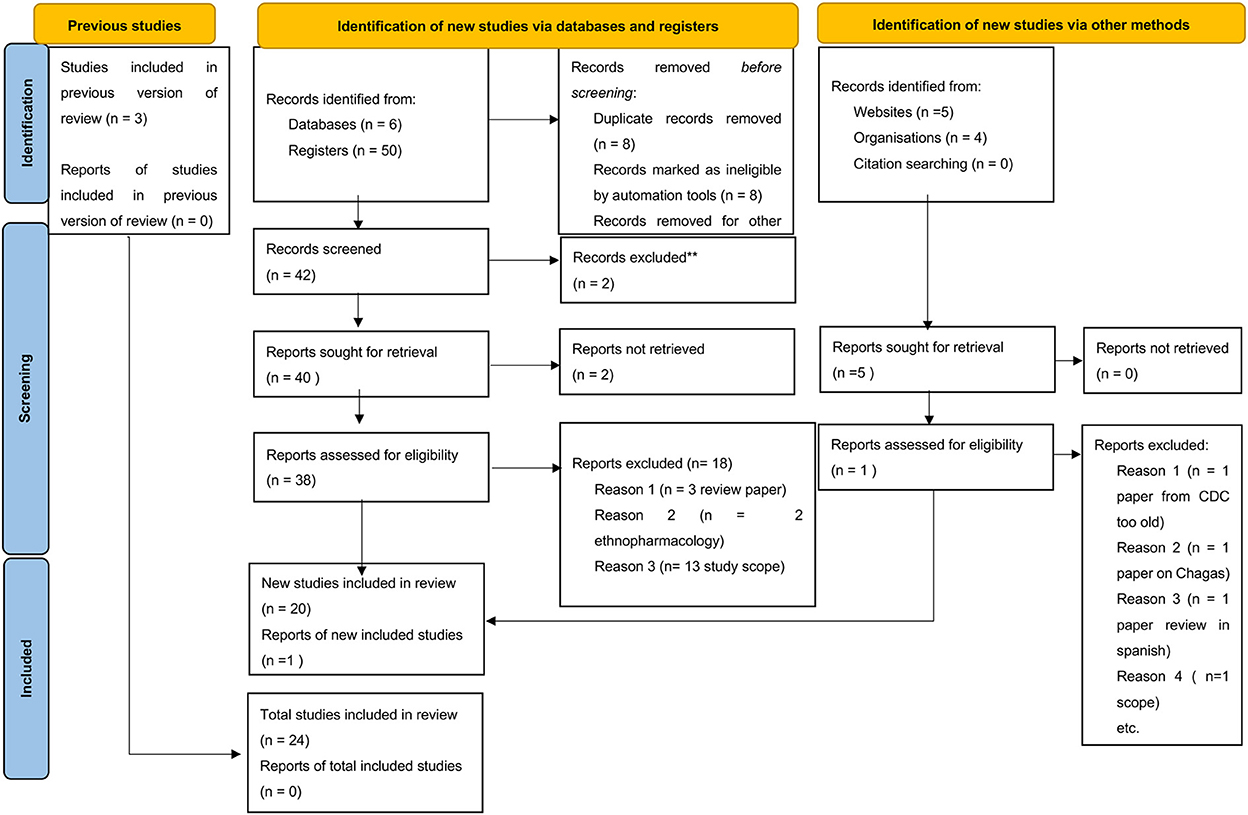
Figure 1. PRISMA checklist showing searched databases, registries, google search directories, and publications included in the study.
2.1. Statistical analysis
Data on the detection of AATr were presented as proportions, fixed, and random-effects models, and Begg's test and Egger's test were conducted to assess publication bias at a 95% confidence interval. Dichotomous data were analyzed using inverse variance at a 95% confidence interval, and the risk ratio was calculated using the fixed model, while continuous data were analyzed using standard mean difference, and random effect sizes were computed using RevMan® provided by Cochrane.
3. Results
3.1. Description of study articles by geographical distribution
A total of eight quantitative studies and 16 qualitative studies were identified in this study (Table 2). Only 4 out of 24 studies were cross-sectional studies that reported a prevalence of AATr ranging from 3.7% in Uganda (21) to 20.8% in Cameroon (41).
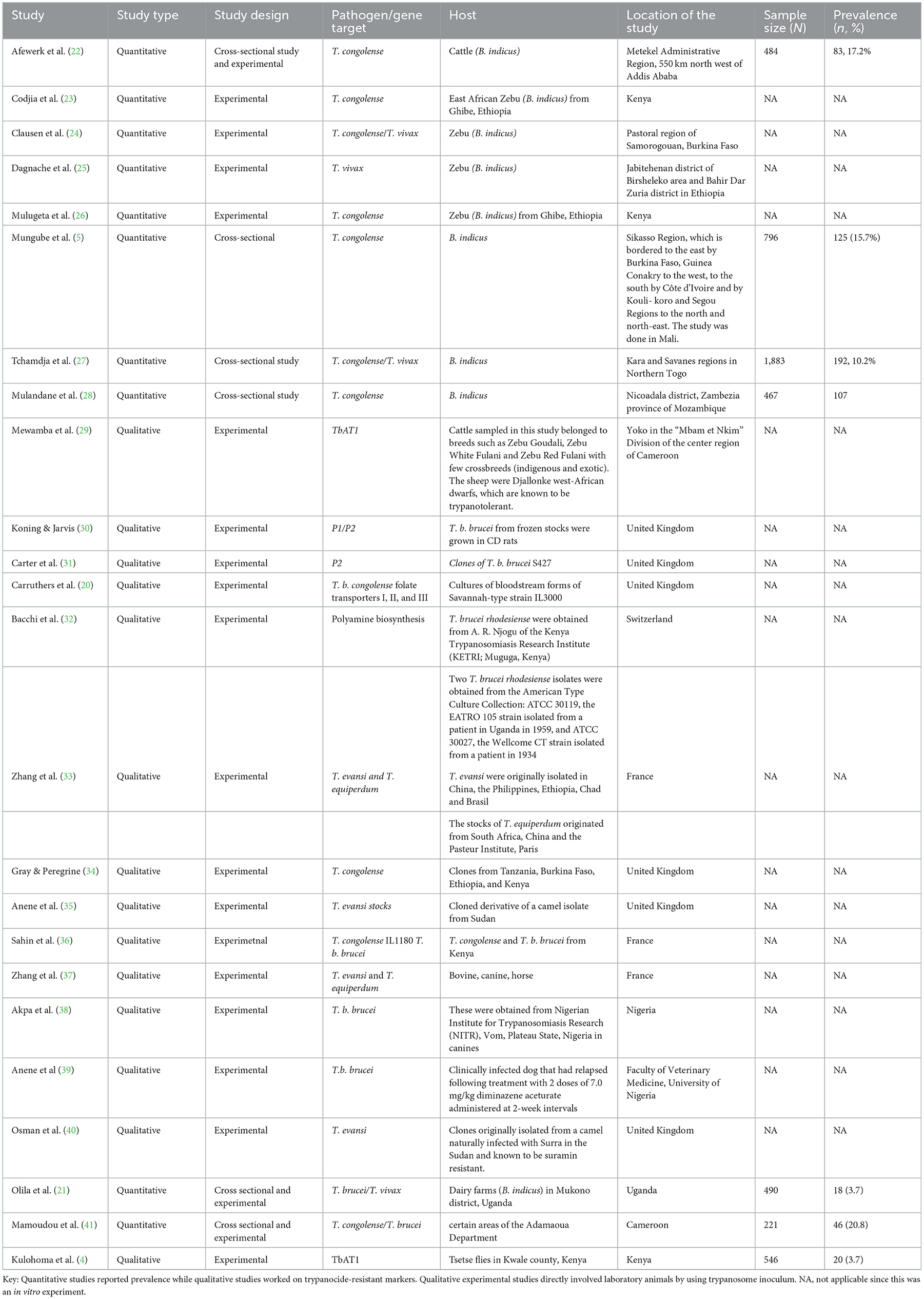
Table 2. Description of study publication on trypanosome pathogen, primary host, and location of study and prevalence.
Most experimental studies have been conducted in Europe, that is, France and the United Kingdom, while in Africa, only Nigeria and Kenya have strongly developed biomedical laboratories, which have attempted to conduct these studies (Figure 2A). Cross-sectional surveys are seldom conducted in African countries with no country reporting more than two studies over the last two decades (Figure 2B). This raises major challenges for trypanosomiasis control and eradication initiatives since the high prevalence of AAT on the African continent is mainly spread along the sub-Saharan belt, a region with continuous neglected clinical trials on AATr to date.
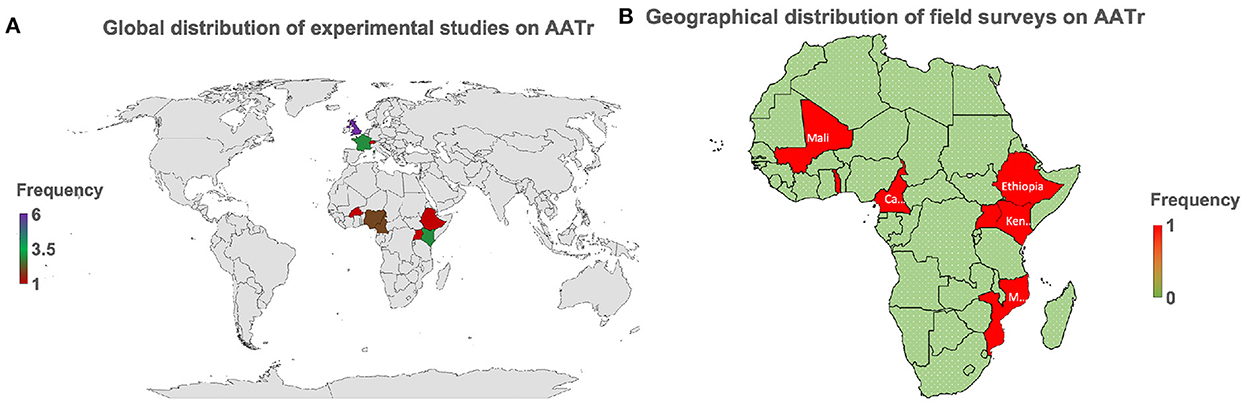
Figure 2. AATr, African animal trypanocide resistance. (A) This provides the global perspective showing that emphasis on AATr is in Africa and Europe. (B) Field surveys are only taking place in Africa globally demonstrating why AATr continues to be a neglected tropical disease. Biomedical experiments have generally been spearheaded by collaborative institutions in Europe while Africa continues to lag in this field due to the low number of active experimental studies on the African continent on AATr (A). This has subsequently translated to a continued lack of commitment by local African institutions to routinely conduct surveillance activities to monitor the development of drug resistance in their countries (B). It is important to note that West and East African countries are more prepared on AATr than their African neighbors in Central and Southern Africa, which all lie within the endemic regions of the continent.
3.2. Community evidence on African animal trypanocide resistance
Here we report an AAT total random effect of 12.4% (95% CI: 7.6–18.2) using the randomized model (Table 3). The test for heterogenicity in these studies was Q (df); 95%CI, P-value at 182.21(6), I2 = 96.71%, 95% CI: 94.97–97.85; P < 0.0001. To assess publication bias, Begg's test and Egger's test were used. Begg's test (correlations of proportions and meta-analysis weight) and Kendall's τ = 0.4286, P = 0.1765, while Egger's test (difference over standard error against one over standard error, that is, the regression analysis of study difference) was 3.6, 95% CI: −14.6 to 21.8, P = 0.6324, demonstrating a lack of publication bias in the studies assessed.
3.3. Preclinical studies on African animal trypanocide resistance
A total of seven quantitative studies were identified; however, only five studies were included in the analysis [eliminated (21) due to the long observation period and (41) who worked on only ISM]. The development of AATr has been investigated using DA at 3.5–7 mg/kg and ISM at 0.5–1.0 mg/kg (Figure 3). An experimental study by Afewerk et al. (22) conducted in Ethiopia showed relapse after 90 days with ISM. In this study (Figure 3, section 1.2.1), the sample size was small (n = 15), thus accounting for the moderate effect size in the study (I2 = 58%). Drug sensitivity showed that ISM relapse was higher than DA, although these findings were coincidental (RR = 0.42, 95% CI: −0.79, 1.63). In addition, the double resistant phenotype was genetically transferable to clones (A, B, and C), demonstrating genetic stability in the resistant genotype.
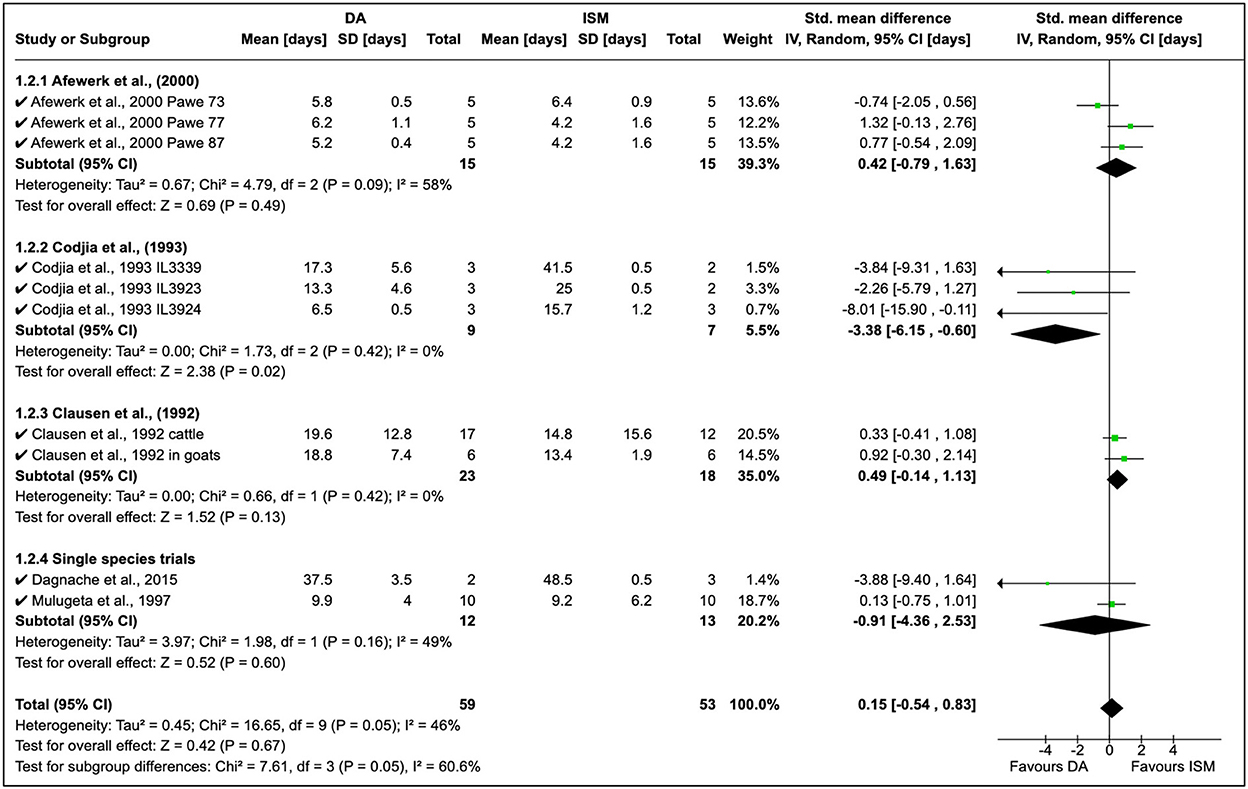
Figure 3. Mean relapse time in preclinical trials with diminazene aceturate and isometamidium chloride.
Cordjia et al. (23) demonstrated a high DA relapse although the reliability of this study was poor (I2 = 0%). In this study, three strains of T. congolense were used in goats; however, the high homogeneity (τ2 = 0%) showed these arose from the same population (Figure 3, Section 1.2.2). This was true since the field isolates were all from male and female East African cattle isolated in 1989 in Ethiopia. Clausen et al. (24) in Burkina Faso reported more ISM relapse than DA although these observations were also incidental due to the low variability in the study although authors worked on both cattle and goats (see Figure 3 where T. congolense and T. vivax isolates are used).
Cordjia et al. (23) also combined DA and HB and showed that relapse was highest with HB (SMD = 1.47, 95% CI: 0.05, 2.88), and similar findings (Figure 4) were reported by Clausen et al. (24) and Mulugeta et al. (26). The overall effect of these studies was identical (τ2 = 0.00) although these were conducted in different animal species (Figure 4).
Homidium resistance in livestock was found to be higher than isometamidium (Table 5). Cordjia et al. (23) demonstrated that HB was a weaker drug than ISM against AATr phenotypes (see Section 1.4.1 in Figure 5) and this was in agreement with Clausen et al. (24). In addition, Mulugeta et al. (26) reproduced the same phenotypic characteristics showing that HB is a weaker (resistance higher) therapeutical option than ISM in Ethiopia using Boran (Bos indicus) calves infected with T. congolense strains. In addition, the same study showed that absorption of ISM was six times lower than the drug-sensitive phenotype, demonstrating the importance of drug-resistance mechanisms.
3.4. Clinical studies on African animal trypanocide resistance
A total of three surveys were identified in the study, and treatment relapse was the common approach used. Mungube et al. (5) showed that there was multiple resistance to DA and ISM in Mali (see Figure 6). Tchamdja (27) showed that DA resistance was most abundant in Togo, while Mulandane (28) reported a higher ISM relapse risk than DA in Mozambique. There was no common consensus on the shift between DA and ISM (RR = 1.01, 95% CI: 0.81, 2.62). In these clinical studies, there was a moderate level of homogeneity (P = 0.16).
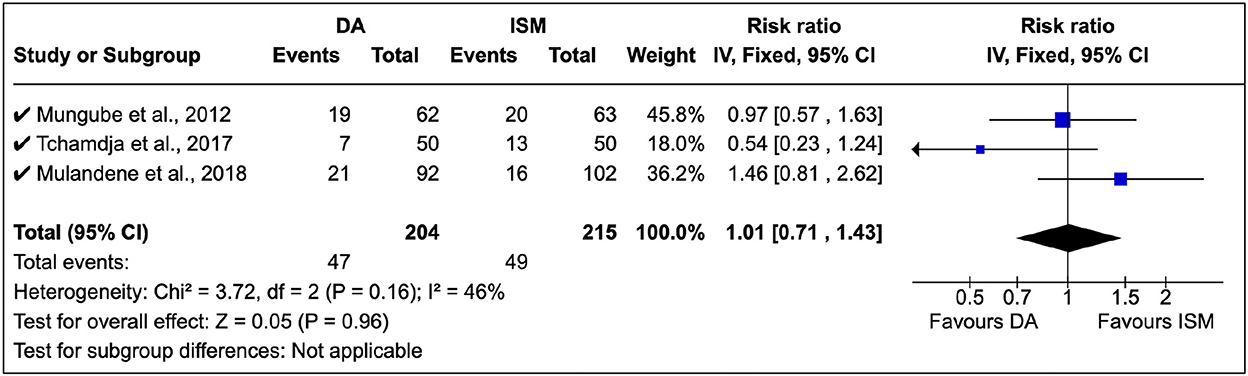
Figure 6. Clinical studies on African trypanocide resistance showing the number of animals that relapsed after 14 days.
In these studies, DA was administered at 3.5 mg/kg while ISM was at 0.5 mg/kg in line with the manufacturer's instructions.
3.5. Resistance markers screened in African animal trypanocide resistance
Mewamba et al. (29) found that the TbAT1 gene is responsible for DA resistance, while de Koning and Jarvis (30) reported that P1/P2 was associated with DA resistance. In addition, methylglyoxal bis (guanylhydrazone) (MGBG) mitochondrial transport (32) was associated with diminazene resistance (Table 4). Resistance has also been associated with immune suppression of leukocytes in association with elevated serum liver enzymes (38).
3.6. Rationale for increasing the dosage to combat trypanocide resistance
To address AATr, an increase in the treatment period has been explored; that is, Mungumbe (5) explored about maintaining the recommended dosage of 0.5 mg/kg and followed up the cattle for a month; however, this approach did not eliminate the development of resistance against DA. In addition, increasing the DA dosage by 3–4 times [see Ref. (24) on goats and Ref. (22) on rats] did not improve the therapeutical outcome in these patients (Table 5).
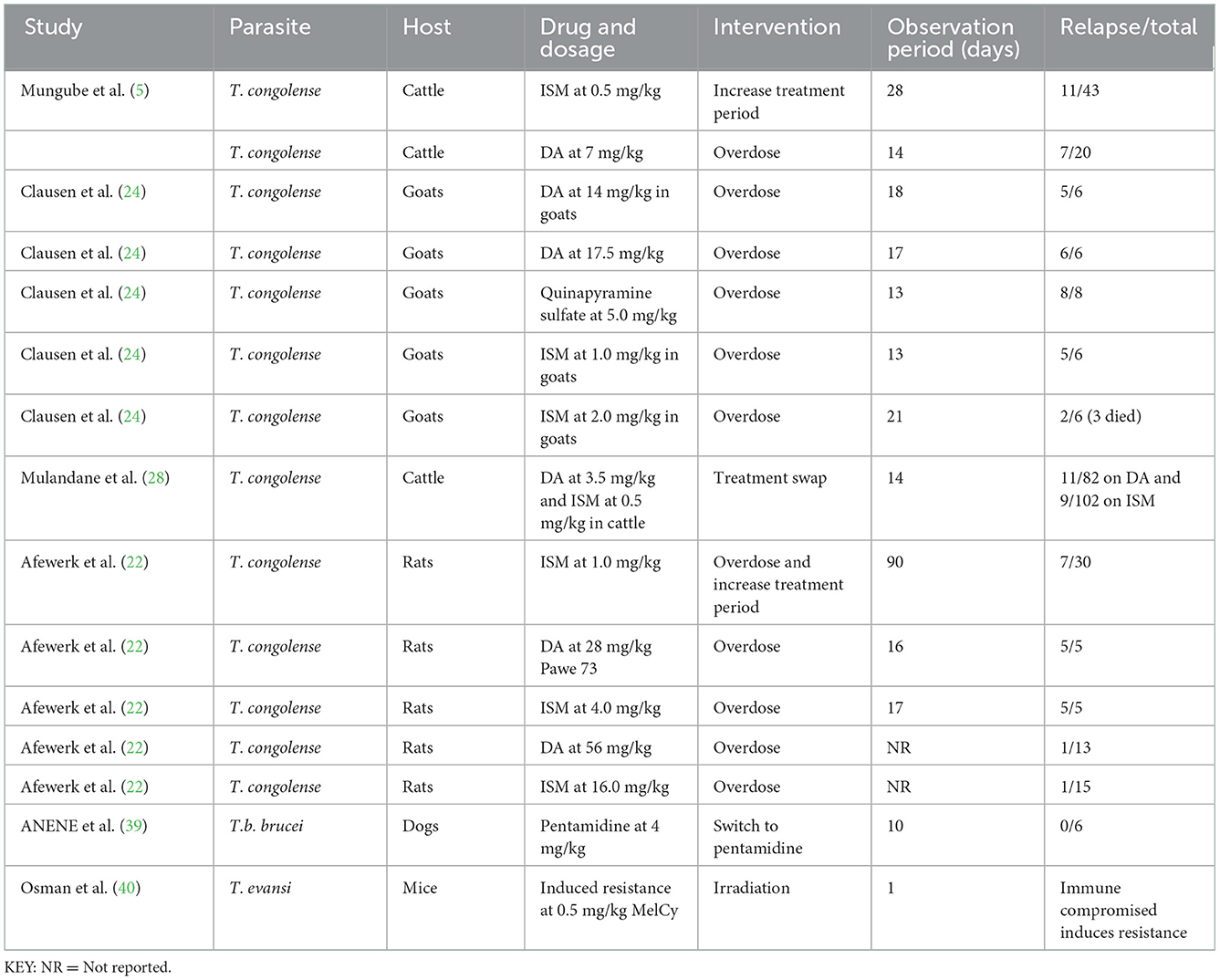
Table 5. Interventions to address trypanocide resistance in preclinical studies with dosages administered using different experimental models.
Several surveys explored the effect of increasing the dosage on treatment outcomes (Table 5). Treatment swaps (DA/ISM for ISM/DA) have also been attempted by Mulandane et al. (28) for an extended 2 weeks and these did not eliminate resistance against DA and ISM. In Nigeria, Anene et al. (39) assessed the quality of DA and found 2 out of 4 samples to contain < 95 and 90% of the stated constituency (Table 5), and cross-reactive resistance with pentamidines was reported in dogs. Furthermore, the continuous passage of T. evansi clones produced a resistant phenotype to melCyl (40), and this provided evidence that immunosuppressed hosts provide a conducive atmosphere for the development of drug resistance (Table 5).
4. Discussion
This study provides evidence on the low global interest in AATr clinical and preclinical trials following sporadic development of drug resistance in most livestock species, and this was in agreement with previous findings (42). In the African continent, there is a scarcity of information on AATr with only a couple of studies arising from West and East Africa, despite their interconnected livelihoods (especially livestock trade and free movement of animals), which act as conduits for infection and reinfection in neighboring countries. Laboratory studies have mainly been supported by the United Kingdom and France (98%) demonstrating their strategic importance for the strengthening of global health collaborations for the attainment of the WHO 2030 target since most of these surveys were conducted before the development of the sustainable developmental goals (SDGs). Developing countries, especially in Africa, continue to lag in biomedical research and routine monitoring of disease [little effort is taken on HAT (43) demonstrating a lack of commitment to AAT], demonstrating critical infrastructure challenges, justifying the need for collaborative research on the African continent (12). In particular, only one biomedical (preclinical) study was fully conducted in an African country (Nigeria) (38), demonstrating critical infrastructure weaknesses in the continent.
AATr has mainly been investigated using DA and ISM with a few studies working on HB. Here, we showed that trypanocide resistance profiles were in the order of HB > DA > ISM; however, the chronic lack of sufficient studies from the African continent implies that it is difficult to apply findings from this study across the entire African continent. Preclinical studies (22–24), which employed dosages at clinically acceptable dosages, demonstrated cross-reactive resistance to DA-ISM-HB although reasons for the development of these resistant phenotypes were not fully explored. This trend raises major threats to developing countries that have not received any capacity to develop alternative therapeutical options for the management of AATr. Furthermore, findings by Afewerk et al. (22) and Clausen et al. (24) showed that ISM relapse was a realistic threat, while Cordjia et al. (23) presented the only study that coincidentally reported DA relapse being higher than ISM. For curative purposes, DA is often preferred compared with ISM, which has both curative and prophylactic purposes and affordability, thus making it an easier drug to access and abuse. It is apparent that ISM is most preferred for the management of AATr, demonstrating its high contribution to drug resistance in this study.
Cross-resistance was also reported in these studies between DA and HB (23, 24, 26). Relapses due to HB were found to occur more often than those due to DA despite the fact these studies were conducted in varying animal species. Evidence from our study shows that the resistant phenotype may not be lost through passages in different animal species. Cross-species infections naturally occur in the wild [see Ref. (9) on wildlife species and Ref. (10) on small ruminants at the wildlife–human interface], so it can be implied that other livestock species do act as maintenance species responsible for the re-emergence of HAT. For example, most African countries have trypanosome control programs aimed at cattle while ignoring small ruminants, dogs, and other animals, which live in the same environment. These findings justify a revision in the current disease control strategies in several developing countries to eliminate and minimize maintenance hosts for reinfection.
The major AATr-responsible genes that have been investigated include TbAT1, P1/P2 transporters, and folate transporters I, II, and III (20, 29–31), as well as host liver enzymes (38) and inhibitors of polyamine biosynthesis (32). Findings in this study are important since P2/TbAT1 resistant genes continue to be essential for DA, ISM, and HB, which was in agreement with our previous study (17). Mutations in the aminopurine transporter AT1 have been associated with reduced trypanocide absorption, while loss of P2 favors the expression of more resistant phenotypes (6). Increased circulation of resistant genes in a community exerts selection pressure, which leads to the development of AATr (Figure 7).
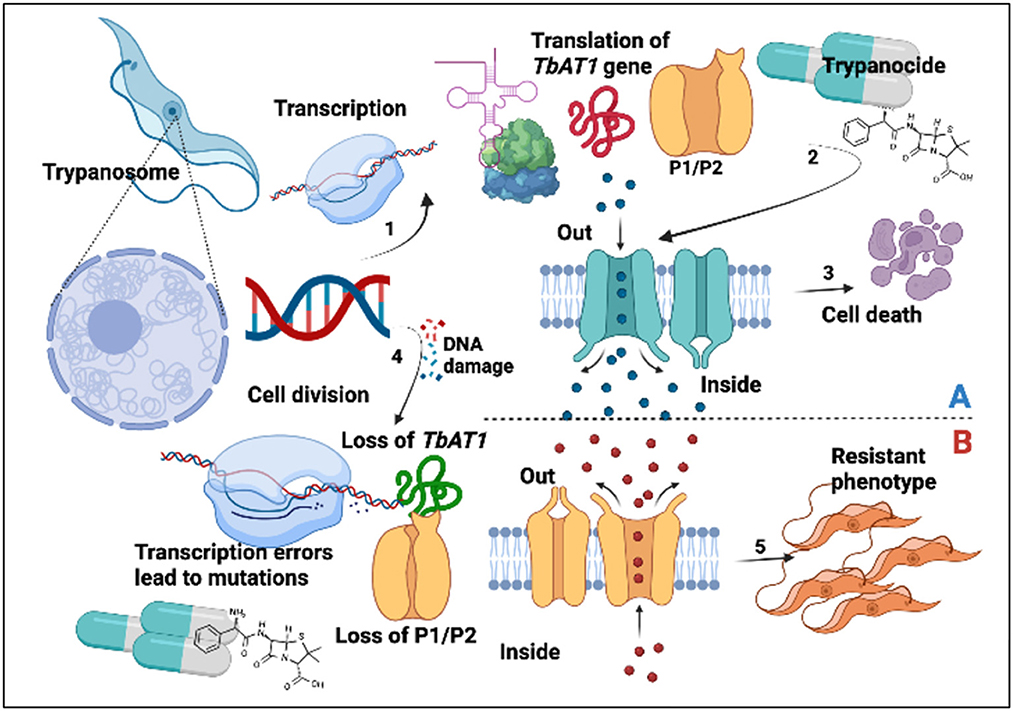
Figure 7. Trypanocide activity is favored by a healthy phenotype (A). (1) Gene expression leading to production of TbAT1/P2. Trypanocides (2) move into the cell to exert their effects leading to cell death (3). Resistant phenotype (B). This arises following DNA damage (4), transcription errors, and subsequent loss of P1/P2 transporters. This subsequently disrupts trypanocide absorption into the parasite, leading to the establishment of a resistant phenotype (5).
The use of high-dosage regimens in several experimental studies [see Ref. (24) on goats and Ref. (22) on rats] failed to improve the clinical outcomes in cases of DA and ISM resistance. This provides a basis to discourage clinicians from doubling and increasing dosages once resistance has been reported against a trypanocide. Furthermore, interchanging medications and increasing the treatment period failed to provide any relief (5), providing a rationale for the promotion of evidence-based therapy while addressing drug resistance. AATr is also propagated by counterfeit trypanocides in circulation (39) and an immune-suppressed status of the host (40) demonstrating the importance of strong drug regulatory policies against fake medicines and farmer education to improve pharmacovigilance in affected communities.
5. Conclusion
African animal trypanocide resistance is a valid threat to the attainment of the WHO 2030 target. This situation has been created due to the limited number of therapeutical options available and limited epidemiological and biomedical studies to monitor drug efficacy in endemic communities. The development of AATr has heavily been associated with homidium bromide and isometamidium chloride, since they are routinely used for prophylaxis purposes; however, drug resistance of all available major trypanocides is cross-reactive. Interventions involving an increase in the dosage and treatment duration have not been able to improve the prognosis of affected animals. Further research to create localized genetic databases and libraries could pave the way toward further knowledge and research in developing countries for the effective monitoring of the AATr challenge and help to advise policy in resource-limited countries.
Data availability statement
The datasets presented in this study can be found in online repositories. The names of the repository/repositories and accession number(s) can be found in the article/Supplementary material.
Author contributions
KK and SW conceptualized the study. KK, SW, and EM conducted the study design. KK collected the data and conducted data analysis. All authors reviewed, approved the publication of the manuscript, and remain in agreement on all aspects of the work.
Funding
This research was supported by the National Institute for Health Research (NIHR) Global Health Research programme (16/136/33) using UK aid from the UK Government. The views expressed in this publication are those of the author(s) and not necessarily those of the NIHR or the Department of Health and Social Care. This work was also supported by the Zhejiang University Education Foundation Emergency Research Fund, the Global Challenges Research Fund, and the University of Edinburgh (SW and KK) and the commonwealth scholarship commission (Grant ID Number: UGCS-2021-447) in the UK (KK).
Acknowledgments
Authors are so grateful for the technical support offered by Ms. Marshall Dozier from the University Library at the University of Edinburgh, United Kingdom.
Conflict of interest
The authors declare that the research was conducted in the absence of any commercial or financial relationships that could be construed as a potential conflict of interest.
Publisher's note
All claims expressed in this article are solely those of the authors and do not necessarily represent those of their affiliated organizations, or those of the publisher, the editors and the reviewers. Any product that may be evaluated in this article, or claim that may be made by its manufacturer, is not guaranteed or endorsed by the publisher.
Supplementary material
The Supplementary Material for this article can be found online at: https://www.frontiersin.org/articles/10.3389/fvets.2022.950248/full#supplementary-material
References
1. Nok AJ. African trypanosomiasis. In: Vaccines for Biodefense and Emerging and Neglected Diseases. Amsterdam: Elsevier (2009), 1255–73. doi: 10.1016/B978-0-12-369408-9.00062-7
2. Radwanska M, Vereecke N, Deleeuw V, Pinto J, Magez S. Salivarian trypanosomosis: a review of parasites involved, their global distribution and their interaction with the innate and adaptive mammalian host immune system. Front Immunol. (2018) 9:2253. doi: 10.3389/fimmu.2018.02253
3. Chitanga S, Marcotty T, Namangala B, Van den Bossche P, Van Den Abbeele J, Delespaux V. High prevalence of drug resistance in animal trypanosomes without a history of drug exposure. PLoS Negl Trop Dis. (2011) 5:e1454. doi: 10.1371/journal.pntd.0001454
4. Kulohoma BW, Wamwenje SAO, Wangwe II, Masila N, Mirieri CK, Wambua L. Prevalence of trypanosomes associated with drug resistance in Shimba Hills, Kwale County, Kenya. BMC Res Notes. (2020) 13:234. doi: 10.1186/s13104-020-05077-3
5. Mungube EO, Vitouley HS, Allegye-Cudjoe E, Diall O, Boucoum Z, Diarra B, et al. Detection of multiple drug-resistant T. congolense populations in village cattle of south-east Mali. Parasit Vect. (2012) 5:155. doi: 10.1186/1756-3305-5-155
6. Geiser F, Luscher A, de Koning HP, Seebeck T, Maser P. Molecular pharmacology of adenosine transport in T. brucei: P1/P2 revisited. Mol Pharmacol. (2005) 68:589–95. doi: 10.1124/mol.104.010298
7. Franco JR, Cecchi G, Paone M, Diarra A, Grout L, Kadima Ebeja A, et al. The elimination of human African trypanosomiasis: Achievements in relation to WHO road map targets for 2020. PLoS Negl Trop Dis. (2022) 16:e0010047. doi: 10.1371/journal.pntd.0010047
8. Fong IW. Animals and mechanisms of disease transmission. In: Emerging Zoonoses. (2017) 15–38. doi: 10.1007/978-3-319-50890-0_2
9. Kasozi KI, Zirintunda G, Ssempijja F, Buyinza B, Alzahrani KJ, Matama K, et al. Epidemiology of trypanosomiasis in wildlife-implications for humans at the wildlife interface in Africa. Front Vet Sci. (2021) 8:621699. doi: 10.3389/fvets.2021.621699
10. Kasozi KI, Namayanja M, Gaithuma AK, Mahero M, Matovu E, Yamagishi J, et al. Prevalence of hemoprotozoan parasites in small ruminants along a human-livestock-wildlife interface in western Uganda. Vet Parasitol Reg Stud Rep. (2019) 17:100309. doi: 10.1016/j.vprsr.2019.100309
11. Azevedo MJ. The state of health system(s) in Africa: Challenges and opportunities. In: Historical Perspectives on the State of Health and Health Systems in Africa, Volume II. Cham: Springer International Publishing (2017). p. 1–73. doi: 10.1007/978-3-319-32564-4_1
12. Kiriiri GK, Njogu PM, Mwangi AN. Exploring different approaches to improve the success of drug discovery and development projects: a review. Fut J Pharm Sci. (2020) 6:27. doi: 10.1186/s43094-020-00047-9
13. Ngumbi AF, Silayo RS. A cross-sectional study on the use and misuse of trypanocides in selected pastoral and agropastoral areas of eastern and northeastern Tanzania. Parasit Vect. (2017) 10:607. doi: 10.1186/s13071-017-2544-3
14. Giordani F, Morrison LJ, Rowan TG, De Koning HP, Barrett MP. The animal trypanosomiases and their chemotherapy: a review. Parasitology. (2016) 143:1862–89. doi: 10.1017/S0031182016001268
15. Manyi-Loh C, Mamphweli S, Meyer E, Okoh A. Antibiotic use in agriculture and its consequential resistance in environmental sources: potential public health implications. Molecules. (2018) 23:795. doi: 10.3390/molecules23040795
16. Mekonnen G, Mohammed EF, Kidane W, Nesibu A, Yohannes H, Van Reet N, et al. Isometamidium chloride and homidium chloride fail to cure mice infected with Ethiopian T. evansi type A and B. PLoS Negl Trop Dis. (2018) 12:e0006790. doi: 10.1371/journal.pntd.0006790
17. Kasozi KI, MacLeod ET, Ntulume I, Welburn SC. An update on African trypanocide pharmaceutics and resistance. Front Vet Sci. (2022) 9:828111. doi: 10.3389/fvets.2022.828111
18. Dean AC, Gould S, Dewar MK, Schnaufer CE. Single point mutations in ATP synthase compensate for mitochondrial genome loss in trypanosomes. Proc Natl Acad Sci U S A. (2013) 110:14741–6. doi: 10.1073/pnas.1305404110
19. Munday HP, Eze JC, Baker AA, Glover N, Clucas L, Aguinaga Andrés C, et al. T. brucei aquaglyceroporin 2 is a high-affinity transporter for pentamidine and melaminophenyl arsenic drugs and the main genetic determinant of resistance to these drugs. J Antimicrob Chemother. (2014) 69:651–63. doi: 10.1093/jac/dkt442
20. Carruthers LV, Munday JC, Ebiloma GU, Steketee P, Jayaraman S, Campagnaro GD, et al. Diminazene resistance in T. congolense is not caused by reduced transport capacity but associated with reduced mitochondrial membrane potential. Mol Microbiol. (2021) 116:564–88. doi: 10.1111/mmi.14733
21. Olila D, McDermott JJ, Eisler MC, Mitema ES, Patzelt RJ, Clausen PH, et al. Drug sensitivity of trypanosome populations from cattle in a peri-urban dairy production system in Uganda. Acta Trop. (2002) 84:19–30. doi: 10.1016/S0001-706X(02)00137-7
22. Afewerk Y, Clausen PH, Abebe G, Tilahun G, Mehlitz D. Multiple-drug resistant T. congolense populations in village cattle of Metekel district, north-west Ethiopia. Acta Trop. (2000) 76:231–8. doi: 10.1016/S0001-706X(00)00108-X
23. Codjia V, Mulatu W, Majiwa PA, Leak SG, Rowlands GJ, Authié E, et al. Epidemiology of bovine trypanosomiasis in the Ghibe valley, southwest Ethiopia. 3 Occurrence of populations of T. congolense resistant to diminazene, isometamidium, and homidium. Acta Trop. (1993) 53:151–63. doi: 10.1016/0001-706X(93)90026-8
24. Clausen P-H, Sidibe I, Kabore I, Bauer B. Development of multiple drug resistance of T. congolense in Zebu cattle under high natural tsetse fly challenge in the pastoral zone of Samorogouan, Burkina Faso. Acta Trop. (1992) 51:229–36. doi: 10.1016/0001-706X(92)90041-U
25. Dagnachew S, Terefe G, Abebe G, Sirak A, Bollo E, Barry D, et al. Comparative clinico-pathological observations in young Zebu (B. indicus) cattle experimentally infected with T. vivax isolates from tsetse infested and non-tsetse areas of Northwest Ethiopia. BMC Vet Res. (2015) 11:307. doi: 10.1186/s12917-015-0625-0
26. Mulugeta W, Wilkes J, Mulatu W, Majiwa PAO, Masake R, Peregrine AS. Long-term occurrence of T. congolense resistant to diminazene, isometamidium and homidium in cattle at Ghibe, Ethiopia. Acta Trop. (1997) 64:205–17. doi: 10.1016/S0001-706X(96)00645-6
27. Tchamdja E, Kulo AE, Vitouley HS, Batawui K, Bankolé AA, Adomefa K, et al. Cattle breeding, trypanosomosis prevalence and drug resistance in Northern Togo. Vet Parasitol. (2017) 236:86–92. doi: 10.1016/j.vetpar.2017.02.008
28. Mulandane FC, Fafetine J, Van Den Abbeele J, Clausen PH, Hoppenheit A, Cecchi G, et al. Resistance to trypanocidal drugs in cattle populations of Zambezia Province, Mozambique. Parasitol Res. (2018) 117:429–36. doi: 10.1007/s00436-017-5718-1
29. Mewamba EM, Farikou O, Kamga RM, Magang ME, Tume C, Tiofack AA, et al. Molecular identification of diminazene aceturate-resistant strains of T. congolense in naturally infected domestic animals of Yoko in the centre region of Cameroon. Vet Parasitol Reg Stud Rep. (2020) 20:100405. doi: 10.1016/j.vprsr.2020.100405
30. de Koning HP, Jarvis SM. Adenosine transporters in bloodstream forms of T. brucei brucei: substrate recognition motifs and affinity for trypanocidal drugs. Mol Pharmacol. (1999) 56:1162–70.
31. Carter NS, Berger BJ, Fairlamb AH. Uptake of diamidine drugs by the P2 nucleoside transporter in melarsen-sensitive and -resistant T. brucei brucei. J Biol Chem. (1995) 270:28153–7. doi: 10.1074/jbc.270.47.28153
32. Bacchi CJ, Brun R, Croft SL, Alicea K, Buhler Y. In vivo trypanocidal activities of new S-adenosylmethionine decarboxylase inhibitors. Antimicrob Agents Chemother. (1996) 40:1448–53.
33. Zhang ZQ, Giroud C, Baltz T. In vivo and in vitro sensitivity of T. evansi and T. equiperdum to diminazene, suramin, MelCy, quinapyramine and isometamidium. Acta Trop. (1991) 50:101–10. doi: 10.1016/0001-706X(91)90002-2
34. Gray MA, Peregrine AS. An in vitro assay for drug sensitivity of T. congolense using in vitro-derived metacyclic trypanosomes. Acta Trop. (1993) 54:291–300. doi: 10.1016/0001-706X(93)90101-G
35. Anene BM, Ross CA, Anika SM, Chukwu CC. Trypanocidal resistance in T. evansi in vitro: effects of verapamil, cyproheptidine, desipramine and chlorpromazine alone and in combination with trypanocides. Vet Parasitol. (1996) 62:43–50. doi: 10.1016/0304-4017(95)00856-X
36. Sahin A, Asencio C, Izotte J, Pillay D, Coustou V, Karembe H, et al. The susceptibility of T. congolense and T. brucei to isometamidium chloride and its synthetic impurities. Vet Parasitol. (2014) 203:270–5. doi: 10.1016/j.vetpar.2014.04.002
37. Zhang ZQ, Giroud C, Baltz T. Trypanosoma evansi: in vivo and in vitro determination of trypanocide resistance profiles. Exp Parasitol. (1993) 77:387–94. doi: 10.1006/expr.1993.1098
38. Akpa PO, Ezeokonkwo RC, Eze CA, Anene BM. Comparative efficacy assessment of pentamidine isethionate and diminazene aceturate in the chemotherapy of T. brucei brucei infection in dogs. Vet Parasitol. (2008) 151:139–49. doi: 10.1016/j.vetpar.2007.10.024
39. Anene BM, Ezeokonkwo RC, Mmesirionye TI, Tettey JN, Brock JM, Barrett MP, et al. A diminazene-resistant strain of T. brucei brucei isolated from a dog is cross-resistant to pentamidine in experimentally infected albino rats. Parasitology. (2006) 132:127–33. doi: 10.1017/S0031182005008760
40. Osman AS, Jennings FW, Holmes PH. The rapid development of drug-resistance by T. evansi in immunosuppressed mice. Acta Trop. (1992) 50:249–57. doi: 10.1016/0001-706X(92)90081-8
41. Mamoudou A, Delespaux V, Chepnda V, Hachimou Z, Andrikaye JP, Zoli A, et al. Assessment of the occurrence of trypanocidal drug resistance in trypanosomes of naturally infected cattle in the Adamaoua region of Cameroon using the standard mouse test and molecular tools. Acta Trop. (2008) 106:115–8. doi: 10.1016/j.actatropica.2008.02.003
42. Matovu E, Seebeck T, Enyaru JCK, Kaminsky R. Drug resistance in T. brucei spp, the causative agents of sleeping sickness in man and nagana in cattle. Microb Infect. (2001) 3:763–70. doi: 10.1016/S1286-4579(01)01432-0
Keywords: African animal trypanosomiasis, Trypanosoma brucei brucei, trypanocide resistance, drug resistance, T. evansi, T. congolense, T. vivax, bovine trypanosomiasis
Citation: Kasozi KI, MacLeod ET and Welburn SC (2023) African animal trypanocide resistance: A systematic review and meta-analysis. Front. Vet. Sci. 9:950248. doi: 10.3389/fvets.2022.950248
Received: 22 May 2022; Accepted: 28 November 2022;
Published: 04 January 2023.
Edited by:
María-Aránzazu Martínez, Complutense University of Madrid, SpainReviewed by:
Ayhan Filazi, Ankara University, TurkeySimon Peter Musinguzi, Kyambogo University, Uganda
Dusit Laohasinnarong, Mahidol University, Thailand
Copyright © 2023 Kasozi, MacLeod and Welburn. This is an open-access article distributed under the terms of the Creative Commons Attribution License (CC BY). The use, distribution or reproduction in other forums is permitted, provided the original author(s) and the copyright owner(s) are credited and that the original publication in this journal is cited, in accordance with accepted academic practice. No use, distribution or reproduction is permitted which does not comply with these terms.
*Correspondence: Keneth Iceland Kasozi, keneth.kasozi@ed.ac.uk;
kicelandy@kab.ac.ug; Susan Christina Welburn,
sue.welburn@ed.ac.uk