- 1Department of Pharmacology, Faculty of Veterinary Medicine, Mansoura University, Mansoura, Egypt
- 2Department of Biomedical Sciences, College of Veterinary Medicine, Iowa State University, Ames, IA, United States
Cadmium (Cd) is an ecological pollutant which causes hazardous effects in animals and humans. The aim of this study was to investigate the role of α-bisabolol (BISA) in antagonizing the Cd-induced hepatotoxicity in ducks. Two-week old ducks were allocated into 8 groups (10 ducks/group): Group I received basal diet and was gavaged with sunflower oil (BISA vehicle, 1.1 mL/kg/day); group II was administered BISA orally (50 mg/kg/day; diluted with sunflower oil); groups III, IV, and V were fed the basal diet mixed with CdCl2 at 37.5, 75, and 150 mg/kg diet, respectively, and were gavaged with sunflower oil; group VI, VII, and VIII were given basal diet containing CdCl2 at the aforementioned consecutive doses plus BISA. All treatments were provided daily for 4 weeks. Exposure to CdCl2 induced mortality in ducks, increased hepatic Cd content and serum levels of hepatopathic biomarkers, and caused oxidative stress and morphological alterations in ducks' liver. Furthermore, exposure to Cd caused upregulation of the mRNA of proinflammatory cytokine tumor necrosis factor-α and apoptotic gene Bax, and that of cyclooxygenase-2 protein in the liver. All effects of Cd were dose-dependent. BISA antagonized all of the aforementioned CdCl2-induced changes. These findings suggested that BISA exert the hepatoprotective effect against Cd toxicity through reducing the hepatic content of Cd as well as antagonizing oxidative insults, inflammation, and apoptosis. Thus, BISA has a great potential to be used as an antidote in the control of Cd poisoning.
Introduction
Cadmium (Cd), a heavy metal, is considered one of the deleterious environmental pollutants. Cd is one of the 10 most hazardous compounds to human and animal health (1). In 1993, the International Agency for Research on Cancer ranked Cd as a Class I carcinogen (2, 3). The sources of Cd exposure stem from its industrial utilization as plastic stabilizers, pigments, alloy, and batteries, as well as being used in mining process (4). Additionally, the soil pollution by Cd results from the extensive usage of fertilizers, pesticides, waste water, and sewage sludge (5). Cd is highly water soluble which leads to its rapid absorption by plants with consequent accumulation in food and feed (6). Former reports have revealed that the bioaccumulation of Cd in chickens can result in serious injuries of several organs, e.g., liver, kidney, heart, lung, brain, testis, and ovary (7). Since Cd has a long half-life of 10–30 years and a slow excretion from the body, it is accumulated in multiple tissues and organs, hence threatening human health via transmission through food chain (8).
Oxidative stress is regarded as the chief mechanism for Cd hepatotoxicity (9). Cd enhances the generation of reactive oxygen species (ROS) as hydroxyl radical (OH−), hydrogen peroxide (H2O2), and superoxide () which interact with multiple cellular molecules, resulting in lipid and protein peroxidation and DNA injury as well as apoptosis (10).
Numerous investigations have indicated that antioxidant substances exert their ameliorative effects against Cd-induced hepatic damage through their potential role in free radicals scavenging (11). α-Bisabolol (BISA), also named levomenol, is a volatile sesquiterpene monocyclic alcohol (12). It is a natural component of the essential oil of multiple medicinal plants of the Asteraceae family (13). BISA has many biological effects, e.g., antimicrobial (14), antioxidant (15), anti-inflammatory (16), anticancer (17), and antimutagenic activities (18). Furthermore, prior investigations highlighted the palliative role of BISA against isoproterenol-induced myocardial infarction in rats (19), neurotoxicity induced by rotenone in Drosophila melanogaster (20), and ischemic reperfusion–induced acute renal injury (21). To the best of our knowledge, the effect of BISA on Cd-induced hepatic damage has not been reported.
The present study was performed to assess the antagonistic effect of BISA on Cd-induced hepatotoxicity. In the present research, we used ducks as an experimental model as they are considered as an important experimental animal in environmental toxicology (22). Moreover, ducks are important in animal production in view of their extensive utilization as traditionally available economic animals. Therefore, this study may confer a practical approach to counteract the global Cd pollution.
Materials and methods
Chemicals
Cadmium chloride (CdCl2) and α-bisabolol were procured from Sigma Aldrich Co. (St. Louis, MO. USA). BISA was used after being diluted with sunflower oil (50 mg BISA/mL sunflower oil). Perchloric acid and nitric acid were bought from Merck Co. (Darmstadt, Germany).
Experimental animals
In this study, a total of 80 one-day-old Muscovy ducks were provided by Faculty of Agriculture, Mansoura University, Egypt; they were housed in disinfected floor pens covered with sawdust litter. The ambient temperature was set at 30–32°C during the first 7 days and gradually declined to 25 ± 1°C at the end of the study. The environmental humidity was set at 50–65%. The ducks underwent a 2-week adaptation period prior to the study. Throughout the experiment, feed and water were freely available to ducks. The feed ingredients and chemical components of the basal diet are shown in Supplementary Table S1. All procedures conducted in the present work were accepted by the Medical Research Ethics Committee of the Faculty of Veterinary Medicine, Mansoura University, Mansoura, Egypt (Approval No. R/117).
Experimental design
A total of 80 two-week old ducks were randomly assigned to 8 groups, with 10 ducks in each group. Group I (control group) was offered basal diet and was gavaged with sunflower oil (BISA vehicle) at a dose of 1.1 mL/kg each day (23). Group II was provided with basal diet and received BISA (50 mg/kg/day), in sunflower oil (50 mg BISA/mL), by gavage. The dose of BISA was selected based on previous reports (24, 25). Groups III, IV, and V were fed basal diet containing CdCl2 at 37.5, 75, and 150 mg/kg diet, respectively, and were gavaged with sunflower oil at 1.1 mL/kg/day. These CdCl2 doses were previously used for toxicological studies in chickens (26, 27). Groups VI, VII, and VIII were fed basal diet plus CdCl2 at the above-mentioned consecutive doses and were administered orally BISA (50 mg/kg/day). This experiment lasted 4 weeks, during which the ducks were monitored for clinical signs of Cd-toxicity and weekly body weight changes and mortality. The experimental period was selected according to a former study (28). At the end of the 4th week, blood samples from 6 ducks in each group were collected from the wing vein. The serum was obtained via centrifugation of the clotted blood samples at 3,000 × g for 10 min, and stored at −80°C until the evaluation of liver function biomarkers. The ducks were then euthanized by exsanguination and the liver was dissected and washed with ice-cold 0.9% NaCl solution. The liver tissue was divided into 3 portions; the first portion was homogenized, then centrifuged at 3,000 × g for 15 min and the harvested supernatant was kept at −80°C for later determination of oxidative stress biomarkers. The second portion was kept at −80°C to be used in the quantitative real time polymerase chain reaction (qRT-PCR). The third portion was fixed in 10% formalin for histopathological and immunohistochemical evaluations.
Biochemical investigation
Serum hepatic indices
The activity of serum alanine aminotransferase (ALT) and total bilirubin were assayed spectrophotometrically using commercial kits purchased from Randox Co. (Crumlin, UK) and BioMed Co. (Cairo, Egypt), respectively. The procedures were performed by following the instructions provided by manufacturers.
Antioxidant and oxidative stress parameters
The malondialdehyde (MDA) level in the hepatic tissue homogenate was evaluated as previously described by Ohkawa et al. (29). The antioxidant enzymatic activities of catalase (CAT) and superoxide dismutase (SOD) were determined using a spectrophotometer following the reports of Aebi (30) and Minami and Yoshikawa (31), respectively. In addition, the content of reduced glutathione (GSH), the nonenzymatic antioxidant marker, was evaluated as described by Paglia and Valentine (32).
Determination of hepatic Cd content
One gram of liver sample was excised and subjected to digestion by a mixture of 2.5 mL perchloric acid (70%) and 5 mL nitric acid (65%). After incubation of this mixture overnight in a water bath at 53°C, the suspension was filtered and 30 mL deionized water was added to the filtrate for dilution. The hepatic Cd concentrations were determined using flame atomic absorption spectrophotometer at a wavelength of 228.8 nm (Buck Scientific 210 VGP, Inc.) following the technique recommended by the Association of Official Analytical Chemists (33).
Transcription of target genes [tumor necrosis factor-α (TNF-α), Bax, and Bcl-2] utilizing qRT-PCR)
The hepatic RNA was isolated utilizing QIAamp RNeasy Mini kit (Qiagen, Heidelberg, Germany). A NanoDrop® (ND-1000) was used for measuring the purity and concentration of the extracted RNA. The first strand of cDNA was synthesized from the isolated RNA employing QuantiTect Reverse Transcription kit (Qiagen, Heidelberg, Germany) according to manufacturer's guidelines. qRT-PCR reactions were performed using SYBR Green QuantiTect PCR kits (Qiagen, Heidelberg, Germany) by the aid of a Rotor-Gene Q cycler RT-PCR equipment (Qiagen, Heidelberg, Germany). β-Actin was used as the reference housekeeping gene. Primer sequences of the investigated duck genes were derived from a previous study (32) and are listed in Table 1. The cycling conditions of qRT-PCR were as follows: 94°C for 5 min; 30 cycles of 94°C for 30 s, 55°C for 30 s and 72°C for 60 s, at last elongation at 72°C for 10 min. The relative expression levels of the target genes were determined via the comparative 2ΔΔCt method (Ct: cycle threshold) as reported by Yuan et al. (35).
Hepatic histopathological screening
Tissue specimens from liver were preserved in 10% formalin. Thereafter, they were processed following the classical histological technique, starting with dehydration using ascending concentrations of alcohol and ended by embedding in paraffin. Multiple tissue sections (4 μm in thickness) were prepared for histological staining. The sections were stained with hematoxylin and eosin (H&E) and then investigated under a light microscope as reported by Bancroft and Layton (36). We conducted semiquantitative scoring of hepatic lesions following the method of Gibson-Corley et al. (37). Lesions in 3 fields chosen randomly from each slide for each duck were determined. A blinded process was utilized for lesion scoring [Score scale: 0 = normal with 0% lesions; 1 = ≤ 25%; 2 = 26–50%; 3 = 51–75%; 4 = 76–100%]. The identification of hepatic lesions in ducks depended on the ratio of hepatocyte degeneration. congestion and fibrosis. The extent of fibrosis was examined by staining the liver sections with Masson's trichrome stain. The sections were photographed and investigated using a light microscope. Quantification of the percent of the area with fibrosis was conducted using Image J software (National Institutes of Health, Bethesda, MD, USA).
Immunohistochemistry
Cyclooxygenase-2 (COX-2) immunohistochemical staining of ducks' hepatic sections was conducted utilizing the avidin-biotin peroxidase method as reported by Lin and Prichardas (38). Photomicrographs of the sections were taken employing a digital camera (Leica EC3, Leica, Leica 148 Germany) attached to a microscope (Leica DM500, Leica, Germany). The Image J software (National Institutes of Health, Bethesda, MD, USA) was used to quantify the intensities of immunostaining. The inverse mean density was evaluated as reported by Vis et al. (39) in 3 fields being selected in a random method from different sections of 6 ducks/group.
Statistical analysis
All values are expressed as mean ± SEM. The Shapiro Wilk test was applied for examining the normality of the data. Statistical analysis was achieved using Statistical Package for Social Sciences (SPSS), version 20 for windows (SPSS Inc., Chicago, IL, USA). Data of histopathological score and immunohistochemical examination were investigated utilizing Kruskal-Wallis followed by Dunn's test to compare all means. All other data were analyzed employing the one-way ANOVA. Mean comparisons were performed utilizing Tukey's post-hoc test. The p < 0.05 was regarded as statistically significant.
Results
Clinical signs observations, body weight changes and mortality rates
Several clinical signs of Cd toxicity were observed in ducks receiving CdCl2 at 75 and 150 mg/kg such as dullness, gasping, restlessness, depression, lethargy, increased water consumption, weakness, and imbalanced gait. These clinical signs began to emerge during the 2nd week of the experiment and aggravated by the end of the 4th week. These signs were more prominent in the group receiving CdCl2 at 150 mg/kg feed. On the other hand, ducks in BISA + Cd 75 and BISA + Cd 150 groups displayed milder clinical signs and reduced water consumption. Furthermore, CdCl2-treated groups exhibited a significant reduction (p < 0.05) in the final body weight (FBW) and body weight gain (BWG), compared to the control group. Meanwhile, a significant elevation (p < 0.05) in FBW and BWG was observed in groups receiving BISA + CdCl2, compared to those receiving CdCl2 only. The mortality rates of CdCl2-treated ducks at doses of 75 was 20% as one duck died in the 1st week of experiment and another duck in the 3rd week. Moreover, the mortality rate of ducks receiving CdCl2 at 150 mg/kg was 30% as one duck died in the 1st week, another one died in the 2nd week, and the third duck died in the 4th week. While, the control group had 0% mortality. Contrarily, the mortality rates in BISA + Cd 75 and BISA + Cd 150 groups were 0 and 10% (as one duck died from BISA + Cd 150 in the 2nd week), respectively, which were lower than those in the groups receiving CdCl2 only at the corresponding dose (Table 2).
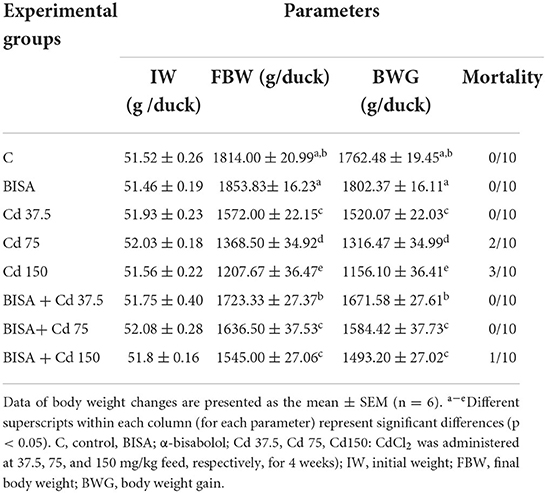
Table 2. Effect of α-bisabolol on body weight changes and mortality in ducks receiving CdCl2 in feed for 4 weeks.
Cd content in hepatic tissues
As illustrated in Figure 1, the hepatic Cd content in CdCl2-exposed ducks at doses of 37.5, 75, and 150 mg/kg diet were significantly higher (p < 0.05) than that in the controls. In contrast, a significant reduction (p < 0.05) in the hepatic Cd content was recorded in all BISA + Cd groups, compared to those treated with the corresponding CdCl2 dose only.
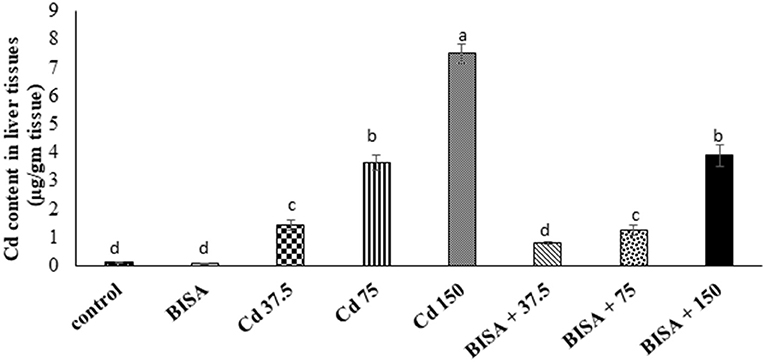
Figure 1. Concentration of Cd in the hepatic tissue of ducks following treatment with oral α-bisabolol (50 mg/kg/day) and/or CdCl2 at 37.5, 75, and 150 mg/kg diet, respectively) for 4 weeks. Data are shown as mean ± SEM (n = 6 ducks). Bars carrying different letters are significantly different from one another (p < 0.05). C, control; BISA, α-bisabolol.
Serum hepatic parameters
BISA alone did not cause significant alterations in the hepatic indices ALT and bilirubin. On the other hand, serum ALT activity and total bilirubin level significantly (p < 0.05) increased in a dose-dependent manner in CdCl2-treated groups (III, IV, and V) by 70, 108, and 193%, respectively (for serum ALT) and by 31, 86, and 186%, respectively (for serum total bilirubin), as compared to the control group. However, co-administration of BISA with CdCl2 at different CdCl2 doses (groups VI, VII, and VIII) showed a significant (p < 0.05) decline in serum ALT activity and total bilirubin level as compared to ducks exposed to CdCl2 alone at the corresponding dose. These hepatic indices returned to normal in BISA + Cd 37.5 and BISA + Cd 75 groups, while they were still higher in BISA + Cd 150 group than the control group, but lower than the CdCl2 alone group (Table 3).
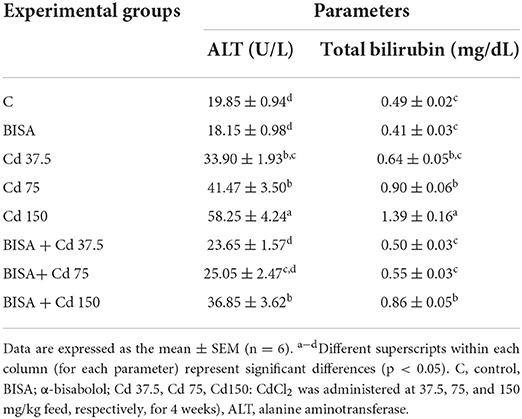
Table 3. Effect of α-bisabolol on serum ALT and bilirubin levels in ducks receiving CdCl2 in feed for 4 weeks.
Histopathological findings
Figure 2 shows the light photomicrographs of hepatic tissues. Figures 2A,B display normal hepatocytes arranged in cords around the central vein with opened sinusoids in the control and BISA groups. In contrast, a dose-dependent histological deformities in the form of hydropic degeneration in hepatic cells, occluded sinusoids, congested central veins, and perivascular fibrosis were detected in CdCl2-exposed groups receiving 37.5, 75, and 150 mg/kg diet; the most extreme lesions were seen in CdCl2 150 mg/kg diet-treated ducks (Figures 2C–E). While, the hepatic sections of groups treated with BISA + CdCl2 revealed much less severe lesions than the groups receiving the corresponding CdCl2 dose alone (Figures 2F–H). The semiquantitative scoring of hepatic lesions is illustrated in Figure 2I.
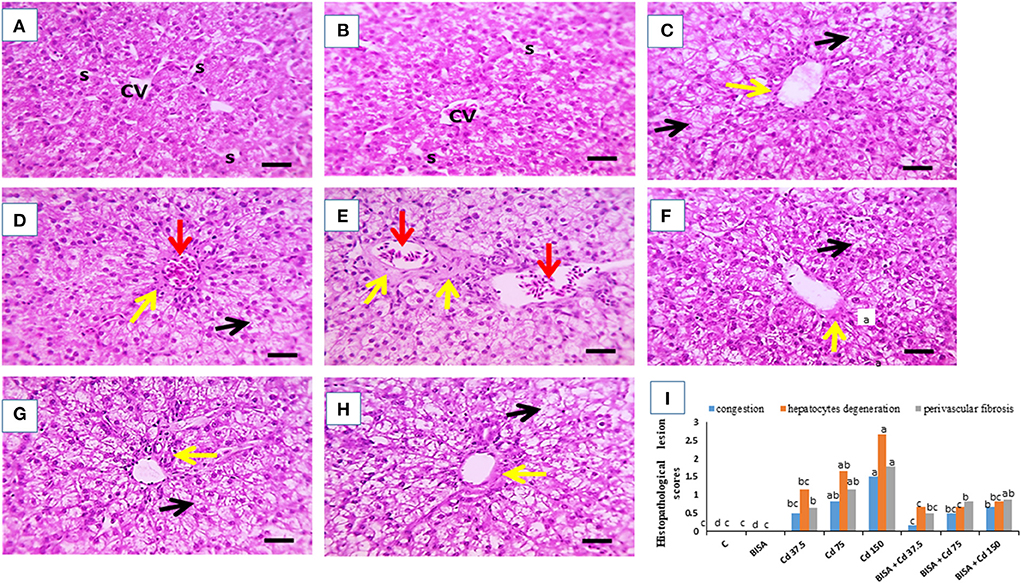
Figure 2. Effect of oral α-bisabolol (BISA; 50 mg/kg/day) on histopathological alterations caused by CdCl2 at different doses in ducks' hepatic tissue. (A) is for the control group (C), and (B) is for the BISA alone group; both groups show normal hepatocytes (H), central vein (CV), and opened sinusoids (S). (C–E) represent groups receiving CdCl2 at 37.5, 75, and 150 mg/kg diet, respectively, and showing hydropic degeneration in hepatocytes (black arrows), occluded sinusoids, congested central veins (red arrows), perivascular fibrosis (yellow arrows). The higher the dose of CdCl2, the more severe the lesions. (F–H) are for groups receiving BISA + CdCl2 at 37.5, 75, and 150 mg/kg diet, respectively, exhibiting less severe lesions than those of the corresponding Cd alone groups. (I) shows the semiquantitative scoring of hepatic lesions. Lesions in 3 fields chosen randomly from each slide for each duck were determined. A blinded process was utilized for lesion scoring [Score scale: 0 = normal with 0% lesions; 1 = ≤ 25%; 2 = 26–50%; 3 = 51–75%; 4 = 76–100%]. The identification of hepatic lesions in ducks depended on the ratio of hepatocyte degeneration. congestion and fibrosis. Bars carrying different letters are significantly different from one another (p < 0.05). Scale bar = 50 μm. The sections were stained with H&E, X400.
The microscopic photograph of Masson's trichrome-stained hepatic sections exhibited normal hepatic cells and central vein with no collagen deposition in the control and BISA groups (Figures 3A,B). While, hepatic sections from ducks receiving CdCl2 at 37.5 mg/kg diet showed blue-stained collagen deposition in portal areas (Figure 3C). Collagen deposition increased in Cd 75- and Cd 150-groups (Figures 3D,E). In contrast, a marked decrease (p < 0.05) in blue-stained collagen deposition was seen in groups receiving CdCl2 + BISA. compared with the groups receiving corresponding CdCl2 dose alone (Figures 3F–H). The percent of area of collagen deposition in hepatic tissues of all experimental groups is shown in Figure 3I.
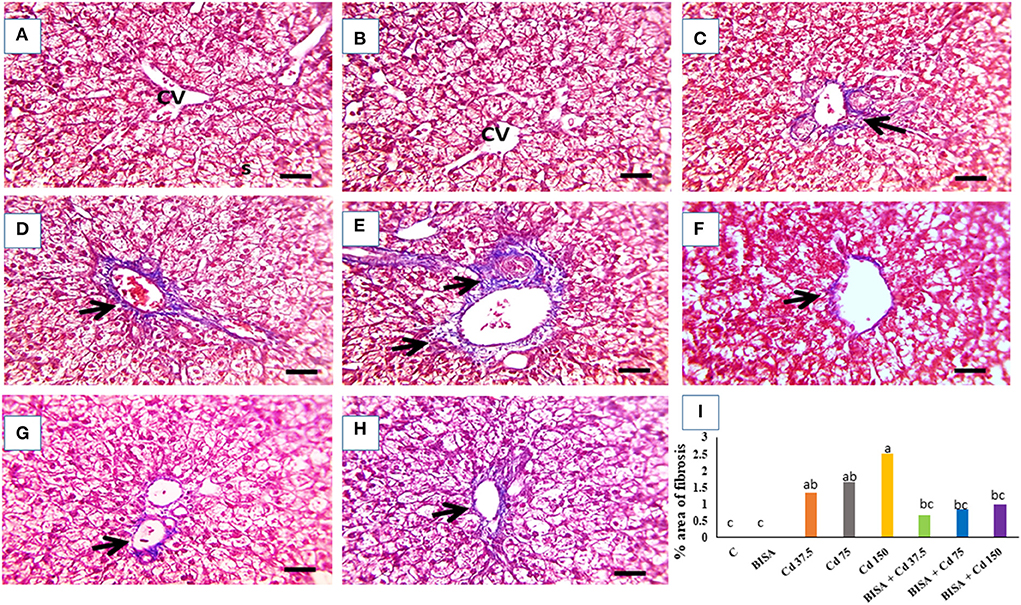
Figure 3. The representative light photomicrographs of ducks' hepatic section (stained with Masson's trichrome, X400). (A) Represents the control group (C) and (B) is for the α-bisabolol (BISA) alone group; both groups show normal hepatocytes and central vein (CV) with no collagen deposition. (C–E) are for groups receiving CdCl2 at 37.5, 75, and 150 mg/kg diet, respectively, revealing blue-stained collagen deposition in portal areas which increase with the increasing dose of CdCl2. (F–H) Represent BISA + Cd 37.5, BISA + Cd 75, and BISA + Cd 150 groups, respectively, and show remarkable reduction in blue color-stained collagen deposition, compared to the corresponding groups receiving CdCl2 only. (I) Exhibits the percent of area of collagen deposition. Data are shown as mean ± SEM (n = 6 ducks). Bars carrying different letters are significantly different from one another (p < 0.05). Quantification of the percent of the area with fibrosis was conducted using Image J software (National Institutes of Health, Bethesda, MD, USA). Scale bar=50 μm.
Oxidative stress and antioxidant markers in hepatic tissues
As shown in Table 4, CdCl2 administration produced a status of oxidative stress in hepatic tissues as depicted by marked elevation of MDA levels (p < 0.05) and reduction in the activities of SOD, CAT and GSH levels in Cd 37.5, Cd 75, and Cd 150 groups, compared to the controls (p < 0.05). In contrast, BISA + Cd 37.5, BISA + Cd 75, and BISA + Cd 150 groups had a significant reduction (p < 0.05) in the hepatic MDA level when compared to the group receiving the corresponding CdCl2 dose alone. Additionally, in ducks receiving both BISA and Cd, the levels of GSH, SOD, and CAT activities were significantly increased (p < 0.05), compared with groups-treated with the corresponding doses of CdCl2 only.
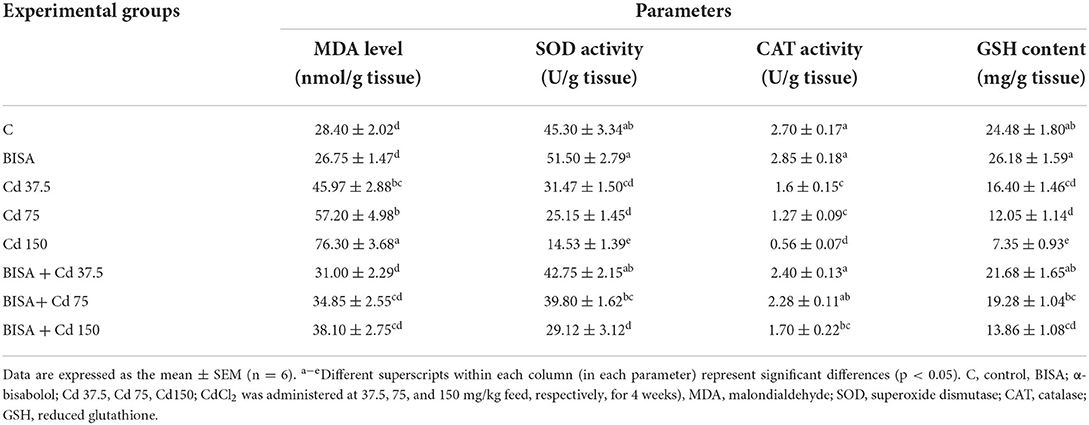
Table 4. Effect of α-bisabolol on hepatic tissue oxidative stress markers in ducks receiving CdCl2 in feed for 4 weeks.
The mRNA expression of TNF-α, Bax, and Bcl-2 genes
The mRNA expression of a proinflammatory cytokine TNF-α and an apoptotic gene Bax were significantly upregulated (p < 0.05) in the hepatic tissue of CdCl2-treated ducks, compared to the controls. Conversely, a marked downregulation (p < 0.05) in the expression of the hepatic anti-apoptotic gene (Bcl-2) was observed in these ducks, compared to the controls. Also, the mRNA levels of TNF-α and Bax dramatically declined (p < 0.05) in ducks receiving BISA + CdCl2, compared to those exposed to the corresponding dose of CdCl2 only. The expression patterns of TNF-α and Bax genes were completely reversed in BISA + Cd 37.5 and BISA + Cd 75 groups, while they remained significantly higher than the controls in BISA + Cd 150 group. Besides, co-administration of BISA with CdCl2 restored the expression levels of Bcl-2 to the level of the controls (Figure 4).
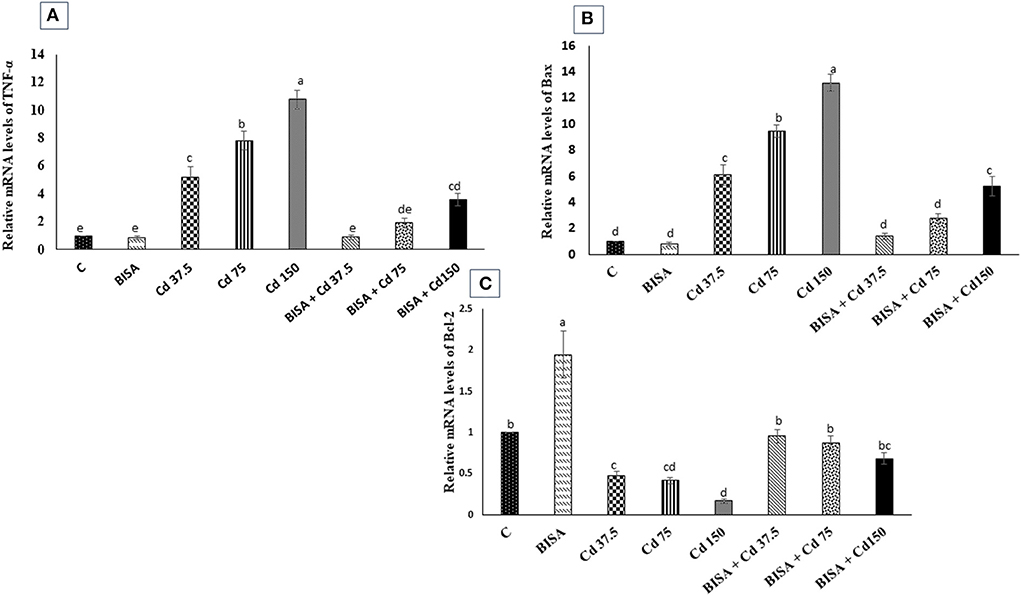
Figure 4. Relative mRNA expression of tumor necrosis factor-α (TNF-α) (A), Bax (B), and Bcl-2 (C) in the hepatic tissue of ducks in response to oral administration of α-bisabolol (BISA, 50 mg/kg/day) and/or CdCl2 at 37.5, 75, or 150 mg/kg diet) for 4 weeks. Data are displayed as mean ± SEM (n = 6 ducks). Bars carrying different letters are significantly different from one another (p < 0.05). C, control.
Immunohistochemical findings of COX-2
The microscopic photographs of immunostained hepatic sections for COX-2 demonstrated very mild positive brown staining of COX-2 in the cytoplasm of hepatocytes in the control and BISA groups (Figures 5A,B). The positive brown staining increased with increasing dose of CdCl2 (Figures 5C–E). In contrast, ducks receiving BISA + CdCl2 displayed remarkable reduction of COX-2 staining, compared to the corresponding CdCl2-alone groups (Figures 5F–H). Figure 5I elucidated the quantitative data of COX-2 staining in hepatic tissues for the experimental groups.
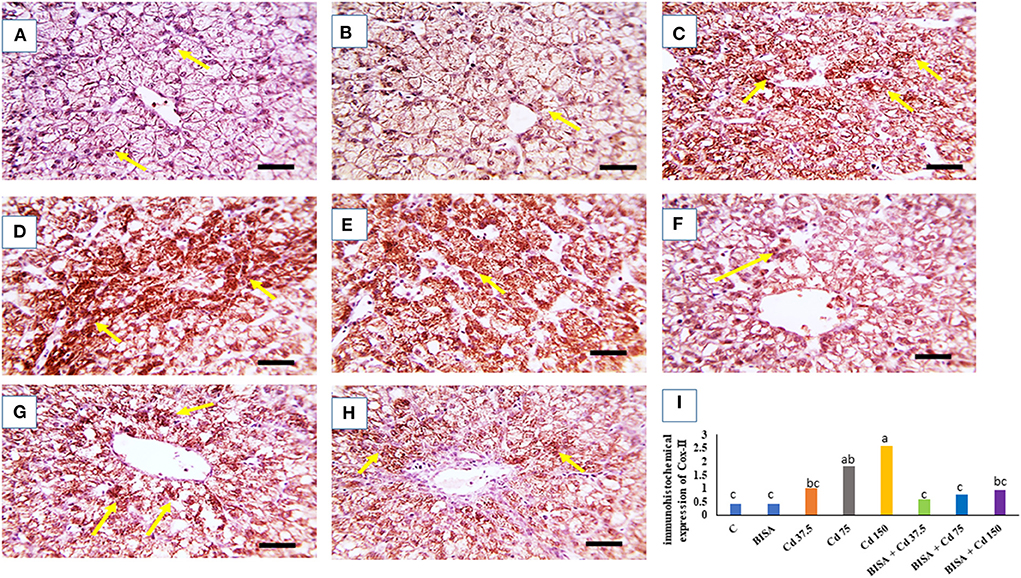
Figure 5. Quantitative data of COX-2 immunohistochemical staining intensity in the duck liver. (A) Expresses the control group (C) and (B) represents the bisabolol (BISA) alone group; both panels show very mild COX-2 brown staining in hepatocytes. (C–E) Are for groups receiving CdCl2 at 37.5, 75, and 150 mg/kg diet, respectively, showing a dose-dependent increase in the brown staining. (F–H) Represent BISA + Cd 37.5, BISA + Cd 75, and BISA+ Cd 15 groups, respectively, and display a marked decline of COX-2-staining, compared to the corresponding CdCl2 alone groups. (I) Shows the quantitative data of COX-2 staining in hepatic tissues for the experimental groups. Quantitative data are expressed as mean ± SEM (n = 6). Bars carrying different letters are significantly different from one another (p < 0.05). The Image J software (National Institutes of Health, Bethesda, MD, USA) was used to quantify the intensities of immunostaining. Scale bar = 50 μm.
Discussion
The current research investigated the toxicological effects of CdCl2 at 3 doses (37.5, 75, and 150 mg/kg feed), on the hepatic tissues and the role of BISA in antagonizing Cd intoxication in ducks. The present study revealed overt adverse clinical signs in ducks after CdCl2 administration at 75 and 150 mg/kg. These observations are in accordance with those of Singh et al. (40) who reported similar clinical signs of toxicity in broiler chickens receiving CdCl2 at 50 mg/L in drinking water. These clinical signs may be due to the immunosuppressant effect of Cd which subsequently increased the bird's susceptibility to stress and disease (40). Furthermore, our results showed a marked reduction in BWG, and an increase in the mortality rate in CdCl2-treated ducks. Similarly, Erdogan et al. (41) reported a decline in BWG in broiler chickens receiving CdSO4 at 25 mg/L drinking water for 6 weeks. Also, Bharavi et al. (28) revealed a drastic drop in BWG in chickens receiving CdCl2 at 100 mg/kg feed for 28 days. Moreover, our results showed that the exposure of ducks to CdCl2 at 37.5 and 150 mg/kg feed for 4 weeks caused marked hepatic injury as witnessed by elevated serum ALT activity and bilirubin level. These findings were consistent with those of Renugadevi and Prabu (42) who reported an elevation in the serum ALT activity and total bilirubin level in rats receiving CdCl2 orally at 5 mg/kg bw/day for 4 weeks.
In the present study, the histopathological investigation of hepatic sections collected from ducks receiving CdCl2 at these doses manifested the Cd-induced hepatic damage by showing hydropic degeneration in hepatic cells, occluded sinusoids, congested central veins, and perivascular fibrosis. These structural changes were consistent with those of Li et al. (43) who reported inflammatory cell infiltration, hepatocyte degeneration, and necrosis in the liver of chickens receiving CdCl2 in the diet at 140 mg/kg for 60 days. Similarly, ballooning degeneration, coagulative necrosis, and cytoplasmic vacuolation were detected in the liver of chickens treated with CdCl2 at 150 mg/kg diet for 60 days (27). Moreover, Koyu et al. (44) reported that CdCl2, even in small amount (15 mg/L of drinking water for 30 days) in rats, caused marked pathological alterations in hepatic tissues. In the present duck study, co-administration of BISA with CdCl2 enhanced BWG, increased survival rate, restored the normal levels of serum hepatic indices and alleviated the histopathological damage induced by CdCl2. Our results were consistent with those of Amaral et al. (45) who reported that BISA ameliorated the hepatic injury induced by acetaminophen in mice. Other sesquiterpenoids, e.g., β-caryophyllene and Rutaecarpine, have a protective effect against the hepatic damage caused by carbon tetrachloride and thioacetamide, respectively (46, 47).
Oxidative stress is the main mechanism underlying the hepatic injury caused by Cd (9). The present study ascertained the capability of Cd to disturb the oxidant-antioxidant balance, evidenced by the increased MDA level, and reduced the anti-oxidant activities of SOD and CAT and the content of GSH in hepatic tissues of ducks. MDA is the main metabolite of lipid peroxidation, which indicates the extent of oxidative damage (48). While, SOD and CAT represent the first line defense antioxidants which play a central role in scavenging free radicals (49). In addition, GSH, a sulfhydryl peptide, is widely distributed in all cellular systems. It participates in the battle against the oxidative insult either by acting as a non-enzymatic antioxidant through direct interaction of its sulfhydryl group with ROS or by acting as a cofactor in the enzymatic detoxification reactions (42). Zhang et al. (50) revealed that Cd has the ability to interact with sulfhydryl (-SH) groups of ROS quenching molecules such as glutathione-SH which ultimately causes indirect elevation of ROS and oxidant-antioxidant imbalance. Our findings were in concordance with those of prior studies (43, 51, 52). In contrast, administration of BISA to CdCl2-poisoned ducks showed marked reduction in MDA level and elevation in the activities of SOD, GSH and CAT. Our findings suggested that BISA decrease the liberation of free radicals and restrain oxidative stress. Corroborating with our findings, several authors have reported the antioxidant activity of BISA (15, 19). Additionally, Vinholes et al. (53) attributed the hepatoprotective effect of sesquiterpenoids, including BISA to their lipophilic and polar characteristics which enhance membrane stability and suppress lipid peroxidation. Furthermore, terpenoids enhancing the activity of SOD, consequently increasing the elimination of free radicals (54).
Cd not only upsets the antioxidant system but also induces inflammatory response (55, 56). Inflammation is regarded as a substantial sequel for oxidative stress (57). As Cd promotes oxidative stress and excessive formation of ROS in the liver, nuclear factor-kappa B (NF-KB) separates from its inhibitory kappa B (IκBα) which enhances the generation of proinflammatory cytokines (56). In this context, Cd-induced hepatic damage is accompanied by the increased production of inflammatory mediators (TNF-α, IL-6, IL-1β) (43). The present work showed a remarkable upregulation of mRNA of TNF-α in hepatic tissues of ducks treated with CdCl2. Moreover, the immunohistochemical screening displayed high COX-2 expression in the liver of CdCl2-intoxicated ducks. COX-2 is an enzyme which is activated by proinflammatory cytokines and serves as a principal contributor to prostanoid synthesis as inflammation proceeds (58). In agreement with our findings, Sanjeev et al. (59) demonstrated a marked increase in the expression of COX-2 in hepatic tissues of rats injected with CdCl2 subcutaneously at 10 mg/kg/day for 30 days. In addition, we found that BISA displayed antiinflammatory effects by lowering the mRNA level of TNF-α and the expression of COX-2 in hepatic tissues of ducks. These findings were parallel to those of Barreto et al. (16) who reported marked antiinflammatory activity of BISA against carrageenan-induced pleurisy through reducing the production of TNF-α and the migration of leukocytes to the peritoneal cavity. Similarly, Kim et al. (60) demonstrated that BISA ameliorated the inflammation caused by lipopolysaccharide in RAW264.7 macrophages via decreasing the expression of COX-2 and inducible nitric oxide synthase.
Apoptosis is another adverse consequence of oxidative stress; it is needed for cellular turnover and homeostasis (61). Previous studies have denoted that Cd could promote apoptosis by provoking the mitochondrial pathway (52). Liu et al. (62) reported that Cd caused leakage in the mitochondrial membrane, inducing translocation of cytochrome C and apoptosis-inducing factors into the cytosol, leading to caspase cascade and eventually apoptosis. Cd-induced oxidative insult plays a prominent role in its ability to cause apoptosis (63). In the present study, CdCl2 intoxication with 3 different dose regimens resulted in significant upregulation of pro-apoptotic gene Bax and downregulation of anti-apoptotic gene Bcl-2 in the liver of ducks. Bax and Bcl-2 are components of Bcl-2 family, responsible for the regulation of the mitochondria-based apoptotic pathway (64). Our findings were consistent with those of prior studies which demonstrated the occurrence of apoptosis in hepatic tissues of chickens intoxicated with Cd (27, 52). In addition, Habeebu et al. (65) reported a dose-dependent apoptosis in the liver of mice exposed to Cd. Furthermore, Cd has been shown to increase the mRNA level of Bax and reduce the mRNA level of Bcl-2 in chicken testis (55).
In the present study, we found that BISA antagonized Cd-induced apoptosis as evidenced by downregulating Bax and upregulating Bcl-2 genes expression in hepatic tissues of ducks. Similarly, Shanmuganathan et al. (66) demonstrated the anti-apoptotic effect of BISA against amyloid-β-induced neurotoxicity in PC12 cells.
In the present study, we found that oral administration of CdCl2 for 4 weeks increased the hepatic content of Cd. CdCl2 supplementation to the diet of birds (ducks, broilers, cocks, and hens) causes an increase of Cd content in the liver (67, 68). In our study, concurrent administration of BISA with CdCl2 dramatically reduced Cd content of the liver in ducks. This effect is probably due to reduction in the active transport of Cd in the gastrointestinal tract (GIT) mucosa or an increase in the excretion of Cd from the GIT or kidneys. Further studies are warranted to investigate the mechanism by which BISA decreases the hepatic Cd content.
Conclusion
This study verified the dose-dependent hepatotoxic effect of CdCl2 at 3 doses (37.5, 75, and 150 mg/kg diet) for 4 weeks in ducks. BISA conferred the protection against hepatoxicity induced by CdCl2 through reducing the hepatic content of Cd as well as antagonizing oxidative insults, inflammation, and apoptosis. BISA even decreased Cd-induced mortality. Future studies are warranted to gain deeper insight into the molecular mechanisms underlying the antagonistic effect of BISA on Cd-induced hepatic damage. Nonetheless, BISA exerts a great potential to be used as an antidote in the control of Cd poisoning. The proposed mechanisms of BISA in antagonizing CdCl2-induced hepatic injury in ducks are presented in Figure 6.
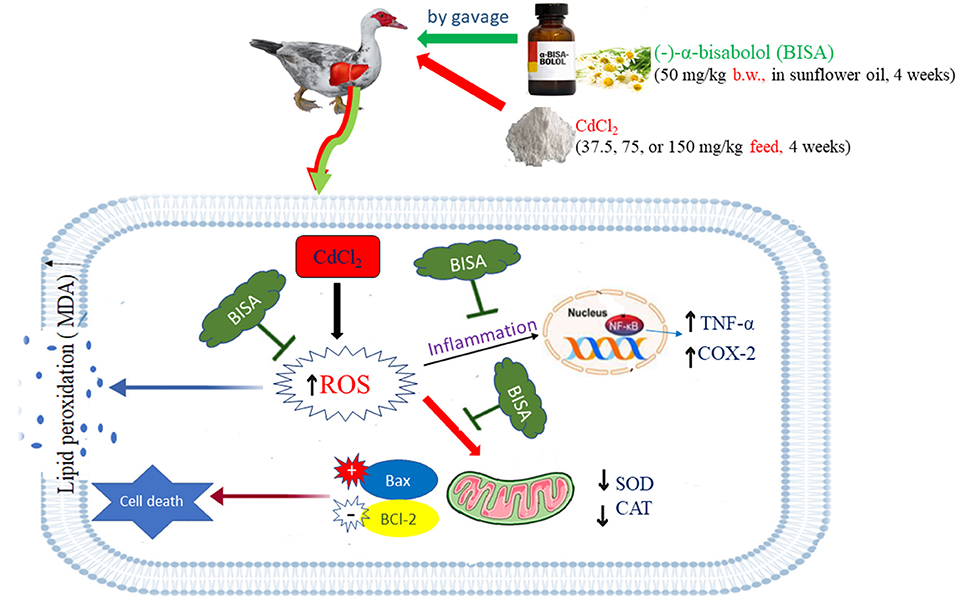
Figure 6. The possible mechanisms of BISA-induced protection against Cd-induced liver toxicity in ducks. The schematic diagram elucidates that BISA exerts its effects via antagonizing the oxidative insult, inflammation, and apoptosis caused by CdCl2 administration.
Data availability statement
The original contributions presented in the study are included in the article/Supplementary materials, further inquiries can be directed to the corresponding author.
Ethics statement
The animal study was reviewed and approved by Medical Research Ethics Committee of the Faculty of Veterinary Medicine, Mansoura University, Mansoura, Egypt.
Author contributions
SE: methodology, data curation, and writing the original draft of the manuscript. WH: conceptualization, data curation review, and editing the manuscript. All authors have read and approved the final manuscript.
Acknowledgments
The authors thank Dr. Ahmed Erfan for his assistance in gene expression analysis and Mr. Ahmed Abbas for his assistance in duck experiment. Also, we appreciate the assistance of the staff members of the Pathology Unit, Faculty of Veterinary Medicine, Mansoura University, Mansoura, Egypt in histopathological and immunohistochemical examination.
Conflict of interest
The authors declare that the research was conducted in the absence of any commercial or financial relationships that could be construed as a potential conflict of interest.
Publisher's note
All claims expressed in this article are solely those of the authors and do not necessarily represent those of their affiliated organizations, or those of the publisher, the editors and the reviewers. Any product that may be evaluated in this article, or claim that may be made by its manufacturer, is not guaranteed or endorsed by the publisher.
Supplementary material
The Supplementary Material for this article can be found online at: https://www.frontiersin.org/articles/10.3389/fvets.2022.1024549/full#supplementary-material
Abbreviations
ALT, Alanine aminotransferase; BISA, α-bisabolol; BWG, Body weight gain; CAT, Catalase; Cd, Cadmium; COX-2, Cyclooxygenase-2; FBW, Final body weight; GSH, Reduced glutathione; IL-1β, Interleukin-1β; IL-6, Interleukin-6; NF-KB, nuclear factor-kappa B; ROS, Reactive oxygen Species; SOD, Superoxide dismutase; TNF-α, Tumor necrosis factor-α.
References
1. Hirano S, Suzuki KT. Exposure, metabolism, and toxicity of rare earths and related compounds. Environ Health Perspect. (1996) 104 (Suppl. 1):85–95. doi: 10.1289/ehp.96104s185
2. Hossein-Khannazer N, Azizi G, Eslami S, Alhassan Mohammed H, Fayyaz F, Hosseinzadeh R, et al. The effects of cadmium exposure in the induction of inflammation. Immunopharmacol Immunotoxicol. (2020) 42:1–8. doi: 10.1080/08923973.2019.1697284
3. Wang Z, Sun Y, Yao W, Ba Q, Wang H. Effects of cadmium exposure on the immune system and immunoregulation. Front Immunol. (2021) 12:695484. doi: 10.3389/fimmu.2021.695484
4. Chen M, Li X, Fan R, Yang J, Jin X, Hamid S, et al. Cadmium induces BNIP3-dependent autophagy in chicken spleen by modulating miR-33-AMPK axis. Chemosphere. (2018) 194:396–402. doi: 10.1016/j.chemosphere.2017.12.026
5. Limei Z, Xiaoyong L, Tongbin C, Xiulan YAN, Hua XIE, Bin WU, et al. Regional assessment of cadmium pollution in agricultural lands and the potential health risk related to intensive mining activities: a case study in Chenzhou City. China J Environ Sci. (2008) 20:696–703. doi: 10.1016/S1001-0742(08)62115-4
6. Chomchan R, Siripongvutikorn S, Maliyam P, Saibandith B, Puttarak P. Protective effect of Selenium-enriched rice grass juice against Cadmium-Induced toxicity and DNA damage in HEK293 kidney cells. Foods. (2018) 7:81–95. doi: 10.3390/foods7060081
7. Cai J, Zhang Y, Yang J, Liu Q, Zhao R, Hamid S, et al. Antagonistic effects of selenium against necroptosis injury via adiponectin-necrotic pathway induced by cadmium in heart of chicken. RSC Adv. (2017) 7:44438–46. doi: 10.1039/C7RA07952D
8. Branca JJ, Fiorillo C, Carrino D, Paternostro F, Taddei N, Gulisano M, et al. Cadmium-induced oxidative stress: focus on the central nervous system. Antioxidants. (2020) 9:492–513. doi: 10.3390/antiox9060492
9. Liu J, Qian SY, Guo Q, Jiang J, Waalkes MP, Mason RP, et al. Cadmium generates reactive oxygen-and carbon-centered radical species in rats: insights from in vivo spin-trapping studies. Free Rad Biol Med. (2008) 45:475–81. doi: 10.1016/j.freeradbiomed.2008.04.041
10. Stohs SJ, Bagchi D, Hassoun E, Bagchi M. Oxidative mechanisms in the toxicity of chromium and cadmium ions. J Environmen Pathol Toxicol Oncol. (2001) 20:77–88. doi: 10.1615/JEnvironPatholToxicolOncol.v20.i2.10
11. Shaikh ZA, Vu TT, Zaman TTK. Oxidative stress as a mechanism of chronic cadmium-induced hepatotoxicity and renal toxicity and protection by antioxidants. Toxicol Appl Pharmacol. (1999) 154:256–63. doi: 10.1006/taap.1998.8586
12. Park J, Han F, Lee IS. Biotransformation of (–)-α-Bisabolol by Absidia coerulea. Molecules. (2022) 27:881. doi: 10.3390/molecules27030881
13. Jakovlev V, Isaac O, Thiemer K, Kunde R. Pharmacological investigations with compounds of chamomile ii. new investigations on the antiphlogistic effects of (-)-alpha-bisabolol and bisabolol oxides (author's transl). Planta Med. (1979) 35:125–40. doi: 10.1055/s-0028-1097194
14. de Sousa Oliveira F, de Freitas TS, da Cruz RP, do Socorro Costa M, Pereira RLS, Quintans-Júnior LJ, et al. Evaluation of the antibacterial and modulatory potential of α-bisabolol, β-cyclodextrin and α-bisabolol/β-cyclodextrin complex, Biomed Pharmacother. (2017) 92:1111–8. doi: 10.1016/j.biopha.2017.06.020
15. Kamatou GP, Viljoen AM. A review of the application and pharmacological properties of α-Bisabolol and α-Bisabolol-rich oils. J Am Oil Chem Soc. (2010) 87:1–7. doi: 10.1007/s11746-009-1483-3
16. Barreto RS, Quintans JS, Amarante RK, Nascimento TS, Amarante RS, Barreto AS, et al. Evidence for the involvement of TNF-α and IL-1β in the antinociceptive and anti-inflammatory activity of Stachys lavandulifolia Vahl. (Lamiaceae) essential oil and (-)-α-bisabolol, its main compound, in mice. J Ethnopharmacol. (2016) 191:9–18. doi: 10.1016/j.jep.2016.06.022
17. Murata Y, Kokuryo T, Yokoyama Y, Yamaguchi J, Miwa T, Shibuya M, et al. The anticancer effects of novel α-bisabolol derivatives against pancreatic cancer. Anticancer Res. (2017) 37:589–98. doi: 10.21873/anticanres.11352
18. Gomes-Carneiro MR, Dias DM, De-Oliveira ACX, Paumgartten FJ. Evaluation of mutagenic and antimutagenic activities of α-bisabolol in the Salmonella/microsome assay. Mutat Res Genet Toxicol Environ Mutagen. (2005) 585:105–12. doi: 10.1016/j.mrgentox.2005.04.007
19. Meeran MFN, Laham F, Al-Taee H, Azimullah S, Ojha S. Protective effects of α-bisabolol on altered hemodynamics, lipid peroxidation, and nonenzymatic antioxidants in isoproterenol-induced myocardial infarction: in vivo and in vitro evidences. J Biochem Mol Toxicol. (2018) 32:e22200. doi: 10.1002/jbt.22200
20. Leite GDO, Ecker A, Seeger RL, Krum BN, Lugokenski TH, Fachinetto R, et al. Protective effect of (–)-α-bisabolol on rotenone-induced toxicity in Drosophila melanogaster. Can J Physiol Pharmacol. (2018) 96:359–65. doi: 10.1139/cjpp-2017-0207
21. Sampaio TL, da Costa MFB, Meneses GC, Arrieta MCV, Chaves Filho AJM, de Morais GB, et al. Nephroprotective effects of (–)-α-bisabolol against ischemic-reperfusion acute kidney injury. Phytomedicine. (2016) 23:1843–52. doi: 10.1016/j.phymed.2016.11.008
22. Lusimbo WS, Leighton FA, Wobeser GA. Histology and ultrastructure of the chorioallantoic membrane of the mallard duck (Anas platyrhynchos). Anat Rec. (2000) 259:25–34. doi: 10.1002/(SICI)1097-0185(20000501)259:1<25::AID-AR3>3.0.CO;2-Y
23. Morais LH, Lima MM, Martynhak BJ, Santiago R, Takahashi TT, Ariza D, et al. Characterization of motor, depressive-like and neurochemical alterations induced by a short-term rotenone administration. Pharmacol Rep. (2012) 64:1081–90. doi: 10.1016/S1734-1140(12)70905-2
24. Javed H, Meeran MF, Azimullah S, Bader Eddin L, Dwivedi VD, Jha NK, et al. α-Bisabolol, a dietary bioactive phytochemical attenuates dopaminergic neurodegeneration through modulation of oxidative stress, neuroinflammation and apoptosis in rotenone-induced rat model of Parkinson's disease. Biomolecules. (2020) 10:1421. doi: 10.3390/biom10101421
25. Fernandes MYD, do Carmo MRS, Fonteles AA, de Sousa Neves JC, da SilvaATA, Pereira JF, et al. (-)-α-bisabolol prevents neuronal damage memory deficits through reduction of proinflammatory markers induced by permanent focal cerebral ischemia in mice. Eur J Pharmacol. (2019) 842:270–80. doi: 10.1016/j.ejphar.2018.09.036
26. Subhan F, Khan A, Wahid F, Shehzad A, Jan AU. Determination of optimal toxic concentration and accumulation of cadmium in broiler chicks. Toxicol Res. (2011) 27:143–7. doi: 10.5487/TR.2011.27.3.143
27. Li JL, Jiang CY, Li S, Xu SW. Cadmium induced hepatotoxicity in chickens (Gallus domesticus) and ameliorative effect by selenium. Ecotoxicol Environ Safety. (2013) 96:103–9. doi: 10.1016/j.ecoenv.2013.07.007
28. Bharavi K, Reddy AG, Rao GS, Reddy AR, Rao SR. Reversal of cadmium-induced oxidative stress in chicken by herbal adaptogens Withania somnifera and Ocimum sanctum, Toxicol Int. (2010) 17:59–63. doi: 10.4103/0971-6580.72671
29. Ohkawa H, Ohishi N, Yagi K. Assay for lipid peroxides in animal tissues by thiobarbituric acid reaction. Anal Biochem. (1979) 95:351–8. doi: 10.1016/0003-2697(79)90738-3
30. Aebi H. Catalase in vitro, Methods Enzymol. (1984) 105:121–6. doi: 10.1016/S0076-6879(84)05016-3
31. Minami M, Yoshikawa H. A simplified assay method of superoxide dismutase activity for clinical use. Clin Chim Acta. (1979) 92:337–42. doi: 10.1016/0009-8981(79)90211-0
32. Paglia DE, Valentine WN. Studies on the quantitative and qualitative characterization of erythrocyte glutathione peroxidase. J Lab Clin Med. (1967) 70:158–69.
33. AOAC. Official Methods of Analysis of the Association of Official Analytical Chemists. 15th ed., Vol. 2. Washington, DC: Association of Official Analytical Chemistry (1990).
34. Liu F, Wang Y, Zhou X, Liu M, Jin S, Shan A, et al. Resveratrol relieved acute liver damage in ducks (Anas platyrhynchos) induced by AFB1 via modulation of apoptosis and Nrf2 signaling pathways. Animals. (2021) 11:3516. doi: 10.3390/ani11123516
35. Yuan JS, Reed A, Chen F, Stewart CN. Statistical analysis of real-time PCR data. BMC Bioinform. (2006) 7:1–12. doi: 10.1186/1471-2105-7-85
36. Bancroft JD, Layton C. Connective and mesenchymal tissues with their stains. In: Bancroft's Theory and Practice of Histological Techniques. Oxford: Churchill Livingstone; Elsevier (2012). p. 187–214.
37. Gibson-Corley KN, Olivier AK, Meyerholz DK. Principles for valid histopathologic scoring in research. Vet Pathol. (2013) 50:1007–15. doi: 10.1177/0300985813485099
38. Lin F, Prichard J. Handbook of Practical Immunohistochemistry: Frequently Asked Questions. New York, NY: Springer (2015).
39. Vis AN, Kranse R, Nigg AL, Van der Kwast TH. Quantitative analysis of the decay of immunoreactivity in stored prostate needle biopsy sections. Am J Clin Pathol. (2000) 113:369–73. doi: 10.1309/CQWY-E3F6-9KDN-YV36
40. Singh R, Srivastava, AK, Gangwar NK, Giri DK, Singh R, et al. Pathology of sub-chronic cadmium and chlorpyrifos toxicity in broilers. Indian J Vet Pathol. (2016) 40:331–6. doi: 10.5958/0973-970X.2016.00076.6
41. Erdogan Z, Erdogan S, Celik S, Unlu A. Effects of ascorbic acid on cadmium-induced oxidative stress and performance of broilers. Biol Trace Elem Res. (2005) 104:19–31. doi: 10.1385/BTER:104:1:019
42. Renugadevi J, Prabu SM. Cadmium-induced hepatotoxicity in rats and the protective effect of naringenin. Exp Toxicol Pathol. (2010) 62:171–81. doi: 10.1016/j.etp.2009.03.010
43. Li T, Yu H, Song Y, Zhang R, Ge M. Protective effects of Ganoderma triterpenoids on cadmium-induced oxidative stress and inflammatory injury in chicken livers. J Trace Elem Med Biol. (2019) 52:118–25. doi: 10.1016/j.jtemb.2018.12.010
44. Koyu A, Gokcimen A, Ozguner F, Bayram DS, Kocak A. Evaluation of the effects of cadmium on rat liver. Mol Cell Biochem. (2006) 284:81–5. doi: 10.1007/s11010-005-9017-2
45. Amaral VD, Cardia GF, Rocha EM, Ribeiro RD, Comar JF, Martins JN, et al. Hepatoprotective effect of (-)-α-bisabolol in an experimental model of paracetamol-induced injury. Res J Pharm Biol Chem Sci. (2021) 12:15–29. doi: 10.33887/rjpbcs/2021.12.3.1
46. Calleja MA, Vieites JM, Montero-Meterdez T, Torres MI, Faus MJ, Gil A, et al. The antioxidant effect of β-caryophyllene protects rat liver from carbon tetrachloride-induced fibrosis by inhibiting hepatic stellate cell activation. Br J Nutr. (2013) 109:394–401. doi: 10.1017/S0007114512001298
47. Ahn H, Lee S, Nam KW, Mar W. Inhibitory effect of rutaecarpine on Thioacetamide (TAA)-induced hepatic fibrosis. Nat Prod Sci. (2014) 20:262–8.
48. Pathak N, Khandelwal S. Oxidative stress and apoptotic changes in murine splenocytes exposed to cadmium. Toxicol. (2006) 220:26–36. doi: 10.1016/j.tox.2005.11.027
49. Pineda J, Herrera A, Antonio MTMT. Comparison between hepatic and renal effects in rats treated with arsenic and/or antioxidants during gestation and lactation. J Trace Elem Med Biol. (2013) 27:236–41. doi: 10.1016/j.jtemb.2012.12.006
50. Zhang L, Li Q, Zheng G, Chen Y, Huang M, Zhang L, et al. Protective effect of Lycium barbarum polysaccharides against cadmium-induced testicular toxicity in male mice. Food Funct. (2017) 8:2322–30. doi: 10.1039/C6FO01583B
51. Wang Y, Chen H, Chang W, Chen R, Xu S, Tao D. Protective effects of selenium yeast against cadmium-induced necroptosis via inhibition of oxidative stress and MAPK pathway in chicken liver. Ecotoxicol Environ Safe. (2020) 206:111329. doi: 10.1016/j.ecoenv.2020.111329
52. Shi J, Chang X, Zou H, Gu J, Yuan Y, Liu X, et al. Protective effects of α-lipoic acid and chlorogenic acid on cadmium-induced liver injury in three-yellow chickens. Animals. (2021) 11:1606. doi: 10.3390/ani11061606
53. Vinholes J, Rudnitskaya A, Gonçalves P, Martel F, Coimbra MA, Rocha SM. Hepatoprotection of sesquiterpenoide: a quantitative dtructure-activity relationship (QSAR) approach. Food Chem. (2014) 145:78–84. doi: 10.1016/j.foodchem.2013.09.039
54. Nunes RCA, Viana RS, MNeto NB. Atividade enzimática da superóxido dismutase em resposta aos fitorreguladores auxina e citocinina em Gerbera sp, Comun Sci. (2015) 6:83–89. doi: 10.1515/9780824856137-012
55. Wang H, Zhang R, Song Y, Li T, Ge M. Protective effect of ganoderma triterpenoids on cadmium-induced testicular toxicity in chickens. Biol Trace Elem Res. (2019) 187:281–90. doi: 10.1007/s12011-018-1364-4
56. Joardar S, Dewanjee S, Bhowmick S, Dua TK, Das S, Saha A, et al. Rosmarinic acid attenuates cadmium-induced nephrotoxicity via inhibition of oxidative stress, apoptosis, inflammation and fibrosis. Int J Mol Sci. (2019) 20:2027. doi: 10.3390/ijms20082027
57. Haddad JJ. Oxygen-sensitive proinflammatory cytokines, apoptosis signaling and redox-responsive transcription factors in development and pathophysiology. Cytokines Cell Mol Ther. (2002) 7:1–14. doi: 10.1080/13684730216401
58. Khan AA, Iadarola M, Yang HY, Dionne RA. Expression of COX-1 and COX-2 in a clinical model of acute inflammation. J Pain. (2007) 8:349–54. doi: 10.1016/j.jpain.2006.10.004
59. Sanjeev S., Bidanchi RM, Murthy MK, Gurusubramanian G, Roy VK. Influence of ferulic acid consumption in ameliorating the cadmium-induced liver and renal oxidative damage in rats. Environ Sci Pollu Res. (2019) 26:20631–53. doi: 10.1007/s11356-019-05420-7
60. Kim S, Jung E, Kim JH, Park Y, Lee J, Park D. Inhibitory effects of (–)-α-bisabolol on LPS-induced inflammatory response in RAW264. 7 macrophages. Food Chemic Toxicol. (2011) 49:2580–5. doi: 10.1016/j.fct.2011.06.076
61. Kannan K, Jain SK. Oxidative stress and apoptosis. Pathophysiology. (2000) 7:153–63. doi: 10.1016/S0928-4680(00)00053-5
62. Liu X, Zhang Y, Wang Y, Yan Y, Wang J, Gu J, et al. Investigation of cadmium-induced apoptosis and the protective effect of N-acetylcysteine in BRL 3A cells. Mol Med Rep. (2016) 14:373–9. doi: 10.3892/mmr.2016.5218
63. Chang KC, Hsu CC, Liu SH, Su CC, Yen CC, Lee MJ, et al. Cadmium induces apoptosis in pancreatic β-cells through a mitochondria-dependent pathway: the role of oxidative stress-mediated c-Jun N-terminal kinase activation. PLoS ONE. (2013) 8:e54374. doi: 10.1371/journal.pone.0054374
64. Tsujimoto Y, Shimizu S. Bcl-2 family: life-or-death switch. FEBS Lett. (2000) 466:6–10. doi: 10.1016/S0014-5793(99)01761-5
65. Habeebu SS, Liu J, Klaassen CD. Cadmium-induced apoptosis in mouse liver. Toxicol. Appl Pharmacol. (1998) 149:203–9. doi: 10.1006/taap.1997.8334
66. Shanmuganathan B, Suryanarayanan V, Sathya S, Narenkumar M, Singh SK, Ruckmani K, et al. Anti-amyloidogenic and anti-apoptotic effect of α-bisabolol against Aβ induced neurotoxicity in PC12 cells. Eur J Med Chem. (2018) 143:1196–207. doi: 10.1016/j.ejmech.2017.10.017
67. Lucia M, Andre JM, Gonzalez P, Baudrimont M, Gontier K, Maury-Brachet R, et al. Impact of cadmium on aquatic bird Cairina moschata. Biometals. (2009) 22:843–53. doi: 10.1007/s10534-009-9232-5
68. Pappas AC, Zoidis E, Georgiou CA, Demiris N, Surai PF, Fegeros K. Influence of organic selenium supplementation on the accumulation of toxic and essential trace elements involved in the antioxidant system of chicken. Food Addit Contam. (2011) 28:446–54. doi: 10.1080/19440049.2010.549152
Keywords: α-bisabolol, cadmium, hepatotoxicity, inflammation, oxidative stress
Citation: Elazab ST and Hsu WH (2022) Antagonism of cadmium-induced liver injury in ducks by α-bisabolol. Front. Vet. Sci. 9:1024549. doi: 10.3389/fvets.2022.1024549
Received: 21 August 2022; Accepted: 19 October 2022;
Published: 07 November 2022.
Edited by:
Zhihua Ren, Sichuan Agricultural University, ChinaReviewed by:
Adil Mehraj Khan, Sher-e-Kashmir University of Agricultural Sciences and Technology, IndiaJianzhao Liao, South China Agricultural University, China
Copyright © 2022 Elazab and Hsu. This is an open-access article distributed under the terms of the Creative Commons Attribution License (CC BY). The use, distribution or reproduction in other forums is permitted, provided the original author(s) and the copyright owner(s) are credited and that the original publication in this journal is cited, in accordance with accepted academic practice. No use, distribution or reproduction is permitted which does not comply with these terms.
*Correspondence: Walter H. Hsu, d2hzdUBpYXN0YXRlLmVkdQ==