- 1Sanofi, Ciudad de México, Mexico
- 2Endowed Health Services Research Center, University of Puerto Rico School of Medicine, San Juan, Puerto Rico
- 3Inversiones en Investigación Médica, Colonia Humuya, Tegucigalpa, Honduras
- 4Unidad de Investigación Clinica, Instituto Nacional de Pediatría, Mexico City, Mexico
- 5Centro de Estudios en Infectología Pediátrica (CEIP), Universidad del Valle and Centro Médico Imbanaco, Valle del Cauca, Colombia
- 6Centro de Investigación Clínica del Pacífico S.A. de C.V., Acapulco, Mexico
- 7Sanofi, Bogotá, Colombia
- 8Sanofi, Beijing, China
- 9Sanofi, Marcy l’Etoile, France
- 10Sanofi, Swiftwater, PA, United States
Objective: The French Polynesia Zika virus (ZIKV) outbreak (2013–2014) broadened the known manifestations of ZIKV disease (ZVD) to include neural and congenital syndromes. A subsequent epidemic in the Americas (2015–2016) caused >685,000 suspected/confirmed cases before seemingly disappearing as fast as it expanded. A study was implemented (2017–2018) to detect ZVD cases in the region (Mexico, Honduras, Colombia and Puerto Rico), with the aim of validating surveillance methodology so as to increase sensitivity in case detection, which would have potential application for future vaccine development endeavors.
Study design and setting: To identify potential cases, we focused on signs/symptoms that were frequently associated with ZVD for confirmation by PCR. Serostatus and seroconversion were evaluated by ZIKV non-structural protein 1 blockade-of-binding enzyme-linked immunosorbent assay (BOB ELISA) and microneutralization assay.
Results: Overall, 2,400 participants aged 15–40 years were enrolled; 959 (40.0%) had signs/symptoms that could be associated with ZVD: axillary temperature ≥37°C (64.3%), myalgia (60.8%) and arthralgia (58.6%). Three suspected cases were virologically confirmed. Zika seroprevalence was high at study initiation (52.6% [BOB ELISA] and 56.0% [microneutralization assay]). In participants who were Zika seronegative, low seroconversion rates were observed after one year follow-up (3.6% [BOB ELISA] and 3.1% [microneutralization assay]).
Conclusion: The ZIKV continued to circulate in the Americas at very low levels following the 2015–2016 outbreak. The epidemiological factors driving Zika’s rapid rise and decline remain poorly understood.
Clinical trial registration: Clinicaltrials.gov: NCT03158233 BARDA (Contract # HHSO100201600039C) WHO Universal Trial Number: U1111-1183-5687.
1 Introduction
Zika virus (ZIKV) is a zoonotic single-stranded RNA arbovirus of the Flavivirus genus; the virus was discovered in Uganda’s Zika Forest in 1947, with the first case in humans reported in 1952 (1). Aedes aegypti and A. albopictus are the two main transmission vectors (2), the same as those that transmit chikungunya (CHIKV), dengue (DENV) and yellow fever (YFV) viruses (3, 4). ZIKV can also be transmitted sexually, perinatally (possibly in utero or via breast feeding) or through the blood-borne route (5, 6). Although most infections are asymptomatic or lead to mild self-limiting illness (7), infection with the virus has been associated with adverse reproductive outcomes (congenital malformations, fetal loss) and other adverse effects including Guillain-Barré Syndrome (GBS) (8, 9).
An unprecedented widespread ZIKV outbreak, initially identified from the suspected association between ZIKV and a surge in serious congenital disorders in Brazil (10), was observed in the Americas during 2015–2016 (11). The World Health Organization (WHO) declared the outbreak a public health emergency of international concern in February 2016, necessitating coordinated international response to intensify surveillance for infections, congenital malformations and neurological complications, and to increase vector control in the region, as well as expedited development of diagnostic tests and vaccines to protect those at risk, especially during pregnancy (12, 13). By November 2016, when the end of the public health emergency of international concern was announced, 48 countries/territories in the Americas had reported >685,000 suspected or confirmed cases (516,487 and 171,553 cases, respectively) (12). Since then, ZIKV transmission is thought to have continued to persist at low levels in the region and a future outbreak remains a potential concern (14, 15).
This study was undertaken to assess the extent of the ZIKV outbreak and its current circulation in Mexico, Honduras, Colombia, and Puerto Rico as representative countries from the Americas region, and which had reported high numbers of cases during 2015–2016. We used specific clinical parameters as identifiers of probable clinical ZIKV disease (ZVD) and PCR for viral confirmation, as well as two novel serology assays (ZIKV non-structural protein 1 blockade-of-binding enzyme-linked immunosorbent assay [BOB ELISA] and microneutralization assay) to assess the presence of Zika-specific antibodies among the study cohort at baseline (to determine seroprevalence) and 1 year after enrolment to assess infection (asymptomatic or symptomatic) during one year follow-up.
2 Methods
2.1 Study design
This was a prospective, multi-center, cohort surveillance study undertaken between 28 April 2017 and 19 December 2018 (WHO Universal Trial number, U1111-1183-5687; ClinicalTrials.gov identifier, NCT03158233) in four countries in Latin America: Mexico (two centers; Acapulco and Temixco), Honduras (Tegucigalpa), Colombia (Cali), and Puerto Rico (Carolina). The conduct of the study was in line with the standards established by the Declaration of Helsinki and compliant with the International Council for Harmonisation guidelines for good clinical practice as well as with all local and/or national regulations and directives. Written informed consent was obtained from all participants or their parents/guardians in case of minors before any procedure associated with the study was performed.
2.2 Participants
Healthy participants aged 15–40 years on the day of inclusion, currently residing within the study site zones in their respective countries and planning to continue to reside in that zone for the duration of the study were recruited. Exclusion criteria included: pregnancy; receipt of dengue or yellow fever vaccine or participation in a clinical trial investigating a vaccine, drug, medical device or a medical procedure in the four weeks preceding enrolment, or during the present study period; or receipt of antibodies (immunoglobulins), blood or blood-derived products in the past three months. Participants with a self-reported history of ZVD (confirmed clinically, serologically, or virologically) were also excluded, but these exclusions were not required to be confirmed by Zika-specific antibody assessment. There were no financial incentives for study participation.
2.3 Procedures and assessments
Two planned study visits took place, at enrolment (Day 0) and 1 year later (Day 365 [ ± 14-day window]). At both these visits, participants provided blood samples for assessment of ZIKV anti-nonstructural protein 1 (NS1) antibodies by blockade-of-binding enzyme-linked immunosorbent assay (BOB ELISA) and for ZIKV neutralizing antibodies by microneutralization (MN) assay (16). Those with Zika NS1 titers <10 or Zika MN titers <100 were defined as Zika seronegative, and those with Zika NS1 titers ≥10 or Zika MN titers ≥100 were defined as seropositive; the specificity and sensitivity of the two immunoassays at these titer cutoffs were reported as 97% and 78%, respectively, for the BOB ELISA, and 100% and 98% for the MN assay for samples obtained in dengue-endemic setting (16). Seroconversion was defined as the change from Zika seronegative at baseline to Zika seropositive post-baseline.
Participants were asked to report any of the following clinical signs/symptoms: rash (pruritic or not), axillary temperature ≥37°C, conjunctivitis (non-purulent/hyperemic), arthralgia/arthritis/myalgia/peri-articular edema, or signs/symptoms of a neurologic/neuroinflammatory disorder (such as acute disseminated encephalomyelitis [including site specific variants], cranial nerve disorders [including paralyses/paresis], GBS—including Miller Fisher syndrome and other variants, immune-mediated peripheral neuropathies or plexopathies, optic neuritis, multiple sclerosis, narcolepsy, transverse myelitis, meningitis, or meningoencephalitis) not explained by other medical conditions. Participants were monitored through regular weekly telephone contact by staff at the study site to ascertain whether they had experienced any of the listed signs or symptoms that could be associated with Zika, and to remind them to inform the staff at the study site within 24 hours of onset of these listed signs/symptoms. Those who reported these symptoms were asked to visit the study site to provide a blood/serum sample within 7 days of onset of signs/symptoms (acute samples), and blood/serum and urine samples 7 to 14 days later (convalescent samples). The samples were assessed for the presence of ZIKV RNA (whole blood and/or urine) by real-time polymerase chain reaction (RT-PCR) by ARUP laboratories (Salt Lake City, UT, USA). Zika RT-PCR was carried out with primers for the NS3 gene sequence conserved among Zika viruses. The limit of detection of the Zika RT-PCR in whole blood and urine was 160 copies/mL. Participants with any sample testing positive for ZIKV were defined as confirmed ZIKV (VCZ) cases. Acute and convalescent serum samples from those with signs/symptoms of a neurologic/neuroinflammatory disorder were additionally tested for anti-ZIKV NS1 antibodies by BOB ELISA and for ZIKV neutralizing antibodies with the MN assay. Additionally, those who became pregnant during the study were asked to provide blood samples for anti-ZIKV NS1 antibody testing when pregnancy became known, and for those with a negative test result, repeated every 3 months until a positive result, end of pregnancy, or end of follow-up occurred (whichever occurred first).
As differential diagnoses, acute serum samples were also tested for DENV (dengue-screen and SimplexaTM RT PCR) and chikungunya by RT-PCR. Dengue RT-PCR assays were conducted at Sanofi’s Global Clinical Immunology laboratories [Swiftwater, PA, USA]) as previously described (17) and the chikungunya RT-PCR assay was developed and validated at ARUP laboratories (Salt Lake City, UT, USA). Dengue screen RT-PCR was carried out using dengue specific primers and probes targeting a conserved dengue region of the 3’-UTR. The Simplexa dengue RT-PCR assay was carried out with primers and probes targeting the conserved dengue NS5 genomic regions for serotype 1 and 3, NS3 for serotype 2 and the capsid region for serotype 4. Chikungunya RT-PCR was carried out with primers targeting the E1 (envelope) gene sequence conserved among CHIKVs. Dengue screen RT-PCR limit of detection was 24 copies/mL and the limit of detection of the CHIK RT-PCR was 1300 copies/mL. The specificity of all PCR assays (including the Zika RT-PCR) was 100% against a panel of related flaviviruses and other common viral and bacterial pathogens.
2.4 Outcomes assessed
The outcomes of interest assessed were: a) identification of signs/symptoms possibly associated with ZVD among participants in order to virologically confirm the occurrence of ZVD (Virologically-Confirmed Zika, VCZ); b) virological confirmation of cases by RT-PCR and c) Zika seroprevalence at baseline and the incidence of infection (asymptomatic and symptomatic) based on Zika seroconversion rates by the end of the study.
2.5 Sample size
The planned sample size of approximately 400 participants per site was based on convenience sampling; a total sample of 2,400 participants was planned to be enrolled across the six study sites. This overall sample size would be able to detect 12 to 120 VCZ cases if the true incidence was 0.5% to 5% and provide a probability of 95% of observing a VCZ event with a true incidence of 0.13% (rule of 3).
2.6 Statistical analyses
All analyses were descriptive. The 95% confidence intervals (CIs) of point estimates were calculated using the normal approximation for quantitative data, and the exact binomial distribution for proportions (Clopper-Pearson method). The full analysis set (FAS) was defined as all participants present at the initial visit. The FAS is the analysis population reported.
3 Results
3.1 Study population
A total of 2,400 participants were enrolled across the four countries: baseline demographic characteristics of the participants are summarized in Table 1. A total of 2,188 (91.2%) participants completed the study: 467/500 (93.4%) in Honduras; 638/700 (91.1%) in Colombia; 741/800 (92.6%) in Mexico; and 342/400 (85.5%) in Puerto Rico. Of these 212/2,400 (8.8%) participants who did not complete the study: 132 (5.5%) withdrew voluntarily, 60 (2.5%) were lost to follow-up and 20 (0.8%) did not comply with the protocol. Reasons for non-compliance included not following inclusion and exclusion criteria at first visit, reporting for visit 2 outside the time window and not attending acute or convalescent visits. Overall, 1 (<0.1%), 275 (11.5%) and 366 (15.3%) had a self-reported (unconfirmed) medical history of YFV, DENV or CHIKV infections, respectively. There were no early terminations due to serious adverse events (SAEs) or other AEs related to the study procedures.
3.2 Outcomes and Assessments
3.2.1 Identification of suspected ZVD and VCZ cases among participants
Of the 2,400 enrolled participants, 959 (40.0%) reported at least one episode of signs/symptoms compatible with ZVD, and 418 (17.4%) had more than one episode. Overall, there were 1,765 (73.5%) suspected episodes reported throughout the study; of these, only three (0.17%) were VCZ as determined by PCR. Acute blood samples were also tested for DENV and CHIKV RNA as a differential diagnosis; DENV was virologically confirmed in 2.3% (41/1,760) of suspected ZVD episodes. The highest numbers of confirmed DENV cases were observed in Honduras (23/742) followed by Mexico (17/624). There were no confirmed cases of CHIKV infection reported in the study.
3.2.2 Description of the reported clinical signs/symptoms
The most frequently reported clinical signs/symptoms were axillary temperature ≥37°C (64.3%; 1,135/1,765), myalgia (60.8%; 1,074/1,765) and arthralgia (58.6%; 1,034/1,765; Table 2). Interestingly, the percentage of participants with a rash (16.4%) and conjunctivitis hyperemic/non-purulent (16.9%) appeared higher in Puerto Rico than at other sites (Table 2). Three (0.2%) neurologic/neuroinflammatory disorders were reported, two of which had cranial nerve disorder (both in Mexico) and one had right inferior limb paresthesia and axillary temperature ≥37°C (in Honduras). None of these cases required hospitalization and all cases recovered. All had a negative ZIKV RT-PCR test.
3.2.3 Description of Zika seroprevalence at baseline and incidence of Zika infection based on seroconversion by the end of the study
Zika seroprevalence at baseline and seroconversion after 1 year of follow-up as determined by Zika BOB ELISA and Zika MN assay are summarized in Table 3. Zika seroprevalence at baseline ranged from 32.3% (129/400) in Puerto Rico to 81.3% (325/400) in Mexico (Acapulco) with the Zika BOB ELISA, and from 30.3% (121/400) to 85.2% (340/399) with Zika MN assay, respectively. Zika seroconversion rates after 1 year in those seronegative at baseline ranged from 1.1% (4/352) in Colombia to 13.2% (9/68) in Mexico (Acapulco) with Zika BOB ELISA and from 1.3% (4/316) to 11.1% (6/54) with Zika MN assay, respectively (Table 3).
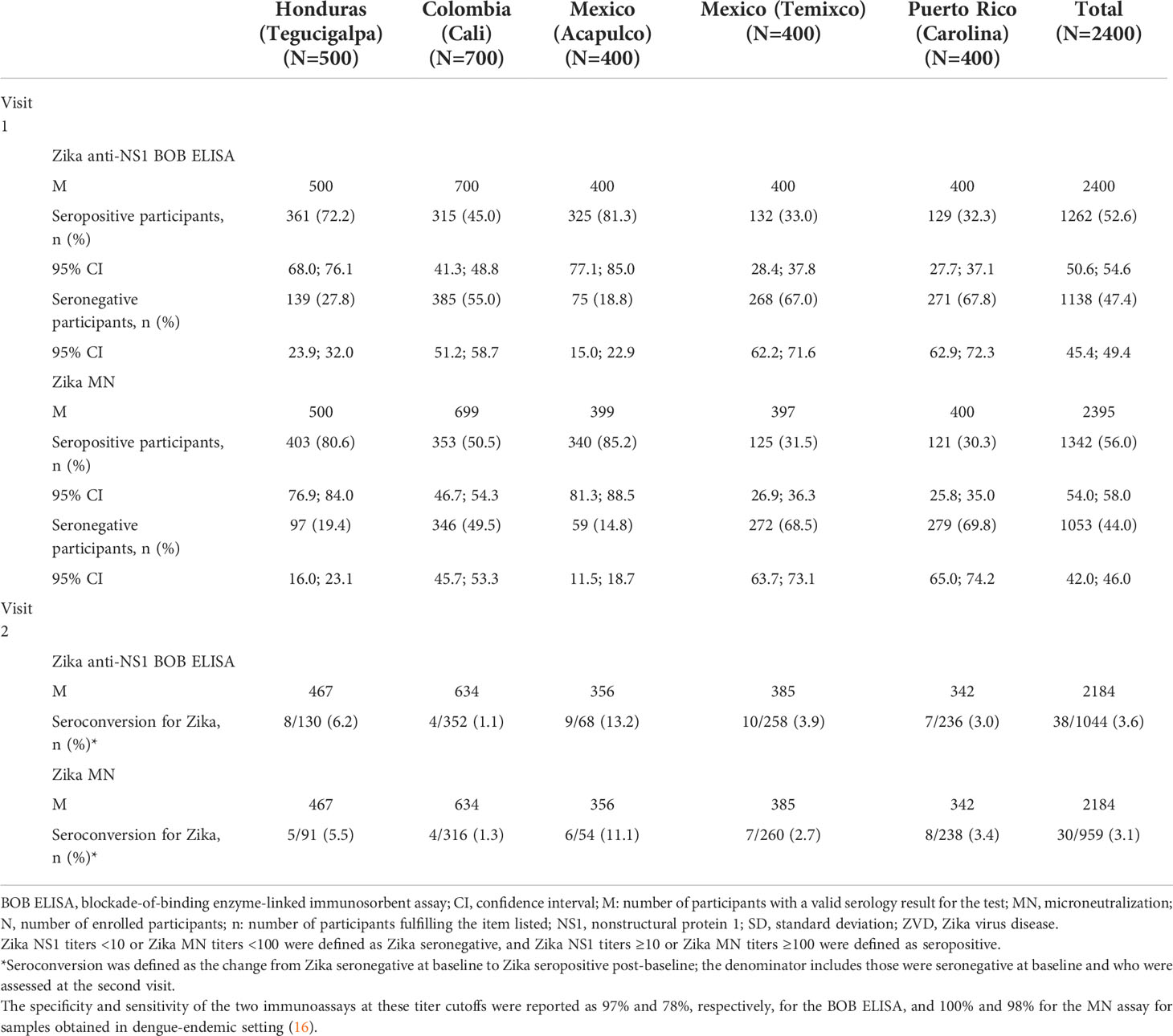
Table 3 Summary of ZIKV seroprevalence at baseline and seroconversion rates after 1 year of follow-up as determined by Zika NS1 BOB ELISA and MN assay (FAS).
Of the 41 participants with PCR-positive dengue infection reported above, 13 were Zika seronegative at baseline and all the others were both dengue and Zika seropositive at baseline. Of the 13 participants Zika seronegative at baseline, 4 (31%) seroconverted to Zika as determined by the Zika MN and/or Zika NS1 BOB ELISA.
3.2.4 Validation of the surveillance operational performance
The number and percentage of samples collected in the specific time windows after the onset of suspected ZVD is summarized in Table 4. Overall, 90.9% (1,604/1,765) and 94.1% (1,660/1,765) of participants with suspected ZVD were tested during the acute (within 7 days of symptom onset) and convalescent (7–14 days after acute sample) phases, respectively.
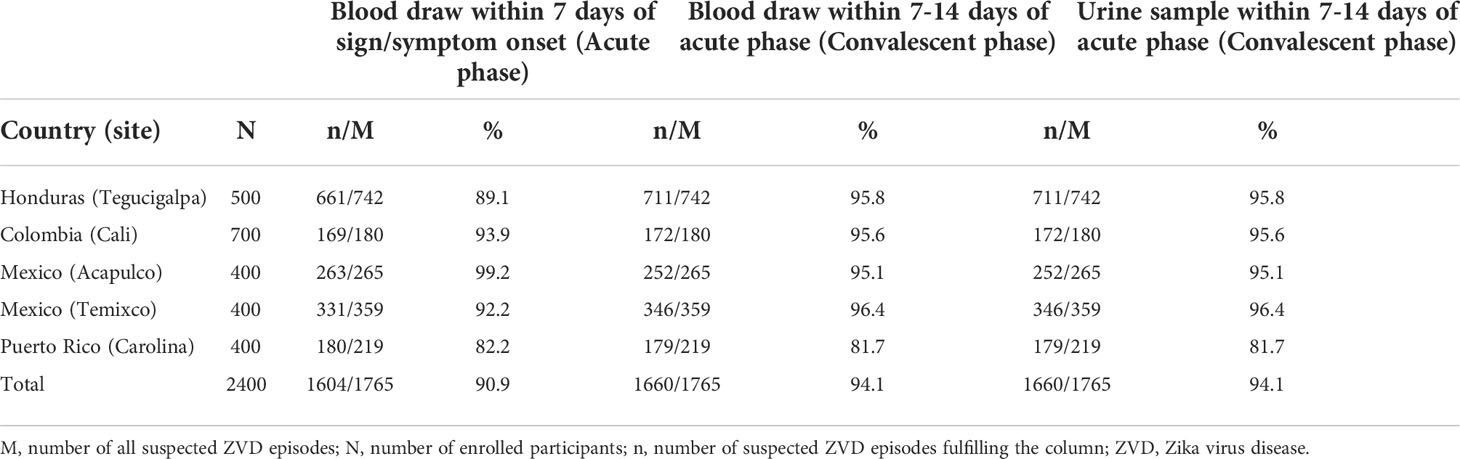
Table 4 Number and percentage of samples collected during the acute and convalescent phases following sign/symptoms onset (FAS).
3.2.5 Pregnancies during the study
A total of 104 (4.3%) participants became pregnant during the study; of these, 52 (50%) and 59 (56.7%) were Zika seropositive at baseline as determined by Zika BOB ELISA and Zika MN assay, respectively. Overall, 46 (44.2%) pregnant participants had at least one episodes of reportable signs/symptoms. However, after 1 year of follow-up, only 1 (2.2%) pregnant participant had seroconverted as determined by Zika MN assay (no seroconversions determined by Zika BOB ELISA). There were no pregnant women with VCZ.
4 Discussion
This surveillance study was designed to assess the circulation of ZIKV in four countries in the Americas immediately after the 2015–2016 outbreak (18), aiming to assess a case definition that could be employed in vaccine clinical trials, as well as to identify suitable locations to conduct such trials. The diagnostic confirmation of ZVD in these countries may be difficult due to the co-circulation of other arboviruses endemic to the region, such as dengue and chikungunya (19–21), thus many countries opted to report “probable cases” based on clinical presentation instead (22). In addition, previous reports have documented that most Zika infections are asymptomatic and those that are symptomatic may have a variety of mild to moderate symptoms (23). We aimed to maximize the detection rate of VCZ cases (using RT-PCR) by targeting those with signs or symptoms reported in the literature to be common in suspected ZVD cases (2, 24–26).
A significant proportion of participants in our study were found to be Zika seropositive at baseline, despite specifically excluding those with a self-reported diagnosed history of ZVD (although there was no screening for Zika-specific antibodies prior to enrolment). It is likely that most of these Zika seropositive participants were asymptomatically infected with ZIKV prior to enrolment. Notably, low seroconversion rates were observed by the end of the study in those Zika seronegative at baseline. Unexpectedly, among participants reporting signs/symptoms compatible with ZVD, only three (0.17%) VCZ cases were confirmed by RT-PCR. Our study suggests that the initial widespread Zika outbreak in the region during 2015–2016 depleted the pool of Zika-susceptible individuals, and thus decreased Zika virus circulation during 2017–2018 in the region as observed in our study. Zika seroprevalence during the same time period as in our study in the participating countries, Colombia, Honduras, Mexico, and Puerto Rico were reported to be 48%, 80%, 39%, and 25%, respectively (16). A Zika seroprevalence of approximately 50–60% has been proposed as sufficient herd immunity to quench outbreaks (27, 28), and it is generally accepted that the Zika seroprevalence in Latin America following the initial outbreak in 2015 was sufficiently high to quench the epidemic, and suppress the virus in the subsequent years (27–29). Thus, it is feasible that sufficient herd immunity may have been reached in the countries participating in our study and, in part, led to the suppression of the initial 2015–2016 epidemic and subsequent low circulation of the virus in the regions assessed.
A mathematical modelling study of Zika cases reported from 2015 to mid-2017 in 35 countries in Latin America and the Caribbean reported subsequent depletion of the susceptible population in most major cities across the region, accounting for the low incidence of infections by 2018 (30). Furthermore, the low number of VCZ cases in our study is consistent with the substantially reduced Zika reports from the WHO and the National Surveillance Systems in the region for 2017–2018 relative to the preceding year (31, 32). Consequently, it has been suggested that herd immunity may have been achieved in most populations (30), with only residual low level local transmission occurring. However, other sources postulate that herd immunity may not yet have occurred, and suggest that the reduced circulation could probably be due to vector seasonal features (33). Indeed, supporting this hypothesis is the observation that seropositivity at baseline was not homogeneously high across the geographic areas included in this study that could explain the steep decline in Zika incidence across the whole continent. In addition, there was a reported reduction in dengue cases reported during 2017 and 2018 in all participating countries compared with the preceding two years with reductions >50% in Colombia, Honduras and Puerto Rico, but not in Mexico (20). Arguably, it is also possible that unprecedented widespread ZIKV outbreak in the preceding years may also have provided cross-immunity against dengue to a large section of the population, thereby resulting in a significant decline in the number of dengue cases reported in the subsequent years (34, 35). Interestingly, two of the three suspected ZVD cases in our study were reported at the Temixco site in Mexico where Zika seropositivity at baseline was the highest. This appears consistent with an increase in confirmed Zika cases reported by the Mexican Ministry of Health during weeks 16–29 of 2017 (32).
Although we aimed to assess a combination of symptoms that would increase specificity for identifying ZVD during surveillance, it is clear that it would be difficult to differentiate ZVD from dengue based on symptoms in the absence of a known outbreak of the former. Of note, 41 (2.3%) of the symptomatic suspected ZVD cases in our study were DENV positive (RT-PCR in acute phase samples). In addition, ZIKV infections are usually difficult to detect since about 80% are asymptomatic or result in mild illness (7, 36).
The Zika seroconversion rates in our study, although low, indicate that the ZIKV still circulated albeit undetected in the areas and during the time period selected for this study. Until the reasons underlying the sudden fall in Zika cases are established, it remains prudent to continue exploring ZIKV surveillance and control measures. In addition, the scope of the complications associated with ZIKV infection are not fully known because of the large knowledge gap concerning ZIKV pathogenesis (14). Of concern, modeling the distribution of the transmission vectors suggests that over 2.17 billion people living in the tropics and subtropics would be at risk of ZIKV infection, based mainly on suitable vector environmental conditions (37), in addition to travel-mediated rapid dissemination of infection (38).
In conclusion, the large Zika outbreak during 2015–2016 in the Americas and associated congenital sequelae observed was cause of great public health concern throughout the affected region. Whether the Zika epidemic depleted the pool of Zika-susceptible individuals or the decrease in ZIKV circulation was secondary to an unrelated depletion of the vector is not well understood. It is, nonetheless, possible that future ZIKV outbreaks may occur when the susceptible population increases.
Data availability statement
Qualified researchers may request access to patient-level data and related documents [including, e.g., the clinical study report, study protocol with any amendments, blank case report form, statistical analysis plan, and dataset specifications]. Patient-level data will be anonymized, and study documents will be redacted to protect the privacy of trial participants. Further details on Sanofi’s data sharing criteria, eligible studies, and process for requesting access can be found at https://vivli.org/.
Ethics statement
The studies involving human participants were reviewed and approved by Comité de Ética en Investigación Biomédica de la Unidad de investigación Científica (CEIB UIC), Universidad Nacional Autónoma de Honduras, Primer Piso Edificio Facultad de Ciencias, Calle la Salud Contiguo al Hospital Materno Infantil, Tegucigalpa, Honduras. Comité de Etica en Investigación Biomedica Corporación Científica Pediátrica (CEIB CEIP), Independent EC office Cali, Colombia. Comité de Ética en Investigación del Instituto Nacional de Pediatría (CEI INP), Comité de Investigación del Instituto Nacional de Pediatría (CI INP), and Comité de Bioseguridad del Instituto Nacional de Pediatría (CB INP), all at Colonia Insurgentes Cuicuilco, Delegación Coyoacán, Ciudad de México, México, Oficina para la Protección de Participantes Humanos en Investigación (OPPHI), and School of Health Professions, San Juan, Puerto Rico. Written informed consent to participate in this study was provided by the participants’ legal guardian/next of kin.
Author contributions
JO, LB, ER, SG, JH, GA, and FN contributed to the conception and/or design of the study. EG-R, DR, JA, EM, and FA contributed to data acquisition. JO, ZC, CP, and FN contributed to the analysis and interpretation of data. All authors were involved in the drafting and critical review of the manuscript and approved the final version. All are accountable for accuracy and integrity of the data presented in this manuscript.
Funding
The project was funded and sponsored by Sanofi and in whole or partly funded with Federal funds from the Department of Health and Human Services; Office of the Assistant Secretary for Preparedness and Response; Biomedical Advanced Research and Development Authority, under Contract No. HHSO100201600039C.
Acknowledgments
The authors wish to thank the participants (as well as the parents of those under the legal age of 18 years), the investigators, coordinators and study teams who were involved in this study: Matthew Bonaparte, Kathi Hudzina, Nichole Webb, and Allison Wehrkamp (Sanofi, Swiftwater, USA), Catherine Moreau, Corinne Terle, and Jesus Garrido (Sanofi, Marcy l’Etoile, France), Marcela Cangrejo, Juan Ortiz, and Andrey Rojas (Sanofi, Colombia), Alma Flores and Nuria Pillado (Sanofi, Mexico), and Nishita Hebbar (PRA Health Science, Canada). Editorial assistance with the preparation of the manuscript was provided by Sam Xavier Hijazi, inScience Communications, Springer Healthcare Ltd., UK, and was funded by Sanofi. The authors would also like to thank Anirban Sanyal for editorial assistance and manuscript coordination on behalf of Sanofi.
Conflict of interest
DR, EG-R, EM, and JA received funds/grants from Sanofi through their institution to undertake this study. ZC, CP, ER, SG, JH, and FN are all employees of Sanofi and may hold shares and/or stock options in the company. GA, JO, and LB were employees of Sanofi at the time of the study and may also hold shares and/or stock options in the company. Author FA was employed by Centro de Investigación Clínica del Pacífico S.A. de C.V.
The remaining author declares that the research was conducted in the absence of any commercial or financial relationships that could be construed as a potential conflict of interest.
Publisher’s note
All claims expressed in this article are solely those of the authors and do not necessarily represent those of their affiliated organizations, or those of the publisher, the editors and the reviewers. Any product that may be evaluated in this article, or claim that may be made by its manufacturer, is not guaranteed or endorsed by the publisher.
References
1. White MK, Wollebo HS, David Beckham J, Tyler KL, Khalili K. Zika virus: An emergent neuropathological agent. Ann Neurol (2016) 80:479–89. doi: 10.1002/ana.24748
3. World Health Organization. Zika virus outbreak global response (2016). Available at: https://www.who.int/emergencies/zika-virus/response/report/en/ (Accessed 5 June 2020).
4. Anez G, Chancey C, Grinev A, Rios M. Dengue virus and other arboviruses: a global view of risks. ISBT Sci Ser (2012) 7:274–82. doi: 10.1111/j.1751-2824.2012.01602.x
5. Dupont-Rouzeyrol M, Biron A, O'Connor O, Huguon E, Descloux E. Infectious zika viral particles in breastmilk. Lancet (2016) 387:1051. doi: 10.1016/S0140-6736(16)00624-3
6. Medina FA, Torres G, Acevedo J, Fonseca S, Casiano L, De Leon-Rodriguez CM, et al. Duration of the presence of infectious zika virus in semen and serum. J Infect Dis (2019) 219:31–40. doi: 10.1093/infdis/jiy462
7. Ladhani SN, O'Connor C, Kirkbride H, Brooks T, Morgan D. Outbreak of zika virus disease in the americas and the association with microcephaly, congenital malformations and Guillain-Barré syndrome. Arch Dis Child (2016) 101:600–2. doi: 10.1136/archdischild-2016-310590
8. Hills SL, Fischer M, Petersen LR. Epidemiology of zika virus infection. J Infect Dis (2017) 216:S868–s74. doi: 10.1093/infdis/jix434
9. Rasmussen SA, Jamieson DJ, Honein MA, Petersen LR. Zika virus and birth defects–reviewing the evidence for causality. N Engl J Med (2016) 374:1981–7. doi: 10.1056/NEJMsr1604338
10. Teixeira MG, Costa Mda C, de Oliveira WK, Nunes ML, Rodrigues LC. The epidemic of zika virus-related microcephaly in brazil: detection, control, etiology, and future scenarios. Am J Public Health (2016) 106:601–5. doi: 10.2105/AJPH.2016.303113
11. Ikejezie J, Shapiro CN, Kim J, Chiu M, Almiron M, Ugarte C, et al. Zika virus transmission - region of the americas, may 15, 2015-December 15, 2016. MMWR (2017) 66:329–34. doi: 10.15585/mmwr.mm6612a4
12. Pan American Health Organization/World Health Organization. Zika cumulative cases 2015-2016 updated (2016). Available at: https://www.paho.org/hq/dmdocuments/2016/2016-nov-17-phe-ZIKV-cases.pdf (Accessed 5 June 2020).
13. Illiois Public Health Association. WHO announces a public health emergency of international concern (2016). Available at: https://www.ipha.com/news/post/215/who-announces-a-public-health-emergency-of-international-concern (Accessed 5 June 2020).
14. Song BH, Yun SI, Woolley M, Lee YM. Zika virus: History, epidemiology, transmission, and clinical presentation. J Neuroimmunol (2017) 308:50–64. doi: 10.1016/j.jneuroim.2017.03.001
15. World Health Organization. Zika epidemiology update 2019 (2019). Available at: https://www.who.int/emergencies/diseases/zika/zika-epidemiology-update-july-2019.pdf?ua=1 (Accessed 5 June 2020).
16. Nascimento EJM, Bonaparte MI, Luo P, Vincent TS, Hu B, George JK, et al. Use of a blockade-of-binding ELISA and microneutralization assay to evaluate zika virus serostatus in dengue-endemic areas. Am J Trop Med Hyg (2019) 101:708–15. doi: 10.4269/ajtmh.19-0270
17. Boaz M, Janosczyk H, Garg S, Yost S, Hildreth SW, Dayan G, et al. Virological confirmation of suspected dengue in a phase 2 Latin American vaccine trial: Implications for vaccine efficacy evaluation. Trials Vaccinol (2014) 3:127–33. doi: 10.1016/j.trivac.2014.07.002
18. Pan American Health Organization. Zika virus infection: data, maps and statistics (2016). Available at: https://www.paho.org/hq/index.php?option=com_topics&view=rdmore&cid=8095&item=zika-virus-infection&type=statistics&Itemid=41484 (Accessed 3 July 2020).
19. Paixão ES, Teixeira MG, Rodrigues LC. Zika, chikungunya and dengue: the causes and threats of new and re-emerging arboviral diseases. BMJ Glob Health (2018) 3:e000530. doi: 10.1136/bmjgh-2017-000530
20. Pan American Health Organization. Dengue fever in the americas number of reported cases by country and territory (2015). Available at: https://www.paho.org/data/index.php/en/mnu-topics/indicadores-dengue-en/dengue-nacional-en/252-dengue-pais-ano-en.html (Accessed 5 June 2020).
21. Pan American Health Organization. Chikungunya: geographic distribution (2017). Available at: https://www.paho.org/hq/index.php?option=com_topics&view=rdmore&cid=6917&item=chikungunya&cat=statistics&type=geographic-distribution-6917&Itemid=40931 (Accessed 3 July 2020).
22. Pan American Health Organization. Zika resources: case definitions (2016). Available at: https://wwwpahoorg/hq/indexphp?option=com_content&view=article&id=11117:zika-resources-case-definitions&Itemid=41532&lang=en (Accessed 5 June 2020).
23. Centers for Disease Control and Prevention. Zika and blood transfusion (2018). Available at: https://www.cdc.gov/zika/transmission/blood-transfusion.html (Accessed 3 July 2020).
24. Cerbino-Neto J, Mesquita EC, Souza TM, Parreira V, Wittlin BB, Durovni B, et al. Clinical manifestations of zika virus infection, Rio de Janeiro, Brazil, 2015. Emerg Infect Dis (2016) 22:1318–20. doi: 10.3201/eid2207.160375
25. Hennessey M, Fischer M, Staples JE. Zika virus spreads to new areas - region of the americas, may 2015-January 2016. MMWR (2016) 65:55–8. doi: 10.15585/mmwr.mm6503e1
26. Pan American Health Organization / World Health Organization. Zika: case definitions (2016). Available at: https://www.paho.org/hq/index.php?option=com_contenYt&view=article&id=11117:zika-resources-case-definitions&Itemid=41532 (Accessed 3 July 2020).
27. Netto EM, Moreira-Soto A, Pedroso C, Hoser C, Funk S, Kucharski AJ, et al. High zika virus seroprevalence in Salvador, northeastern Brazil limits the potential for further outbreaks. mBio (2017) 8:e01390–17. doi: 10.1128/mBio.01390-17
28. Ribeiro GS, Hamer GL, Diallo M, Kitron U, Ko AI, Weaver SC. Influence of herd immunity in the cyclical nature of arboviruses. Curr Opin Virol (2020) 40:1–10. doi: 10.1016/j.coviro.2020.02.004
29. Lourenco J, Maia de Lima M, Faria NR, Walker A, Kraemer MU, Villabona-Arenas CJ, et al. Epidemiological and ecological determinants of zika virus transmission in an urban setting. Elife (2017) 6:e29820. doi: 10.7554/eLife.29820
30. O’Reilly KM, Lowe R, Edmunds WJ, Mayaud P, Kucharski A, Eggo RM, et al. Projecting the end of the zika virus epidemic in Latin America: A modelling analysis. BMC Med (2018) 16:180. doi: 10.1186/s12916-018-1158-8
31. Pan American Health Organization. Cases of zika virus disease by country or territory (2020). Available at: https://www.paho.org/data/index.php/en/?option=com_content&view=article&id=524:zika-weekly-en&Itemid=352 (Accessed 3 July 2020).
32. Pan American Health Organization. Regional zika epidemiological update (2017). Available at: https://www.paho.org/hq/index.php?option=com_content&view=article&id=11599:regional-zika-epidemiological-update-americas&Itemid=41691&lang=en (Accessed 5 June 2020).
33. Silva JVJJ, Lopes TRR, Oliveira-Filho EF, Oliveira RADS, Gil LHVG. Perspectives on the zika outbreak: Herd immunity, antibody-dependent enhancement and vaccine. Rev Inst Med Trop Sao Paulo (2017) 59:e21. doi: 10.1590/s1678-9946201759021
34. Ngono AE, Shresta S. Immune response to dengue and zika. Annu Rev Immunol (2018) 36:279–308. doi: 10.1146/annurev-immunol-042617-053142
35. Wen J, Shresta S. Antigenic cross-reactivity between zika and dengue viruses: is it time to develop a universal vaccine? Curr Opin Immunol (2019) 59:1–8. doi: 10.1016/j.coi.2019.02.001
36. Haby MM, Pinart M, Elias V, Reveiz L. Prevalence of asymptomatic zika virus infection: a systematic review. Bull World Health Organ (2018) 96:402–13d. doi: 10.2471/BLT.17.201541
37. Messina JP, Kraemer MUG, Brady OJ, Pigott DM, Shearer FM, Weiss DJ, et al. Mapping global environmental suitability for zika virus. eLife (2016) 5:e15272. doi: 10.7554/eLife.15272
Keywords: Latin America, seroconversion, seroprevalence, Zika virus, case definition, surveillance
Citation: Rivas E, Ojeda J, Garcia-Rivera EJ, Rivera DM, Arredondo JL, Medina EL, Aguirre F, Bernal L, Chen Z, Petit C, Guranathan S, Heinrichs JH, Áñez G and Noriega F (2022) Prospective surveillance of Zika virus at the end of the Americas’ outbreak: An unexpected outcome. Front. Trop. Dis. 3:1027908. doi: 10.3389/fitd.2022.1027908
Received: 25 August 2022; Accepted: 03 October 2022;
Published: 24 October 2022.
Edited by:
Carlos Brites, Federal University of Bahia, BrazilReviewed by:
Marli Cordeiro, Fiocruz Pernambuco, BrazilEduardo Netto, Federal University of Bahia, Brazil
Copyright © 2022 Rivas, Ojeda, Garcia-Rivera, Rivera, Arredondo, Medina, Aguirre, Bernal, Chen, Petit, Guranathan, Heinrichs, Áñez and Noriega. This is an open-access article distributed under the terms of the Creative Commons Attribution License (CC BY). The use, distribution or reproduction in other forums is permitted, provided the original author(s) and the copyright owner(s) are credited and that the original publication in this journal is cited, in accordance with accepted academic practice. No use, distribution or reproduction is permitted which does not comply with these terms.
*Correspondence: Enrique Rivas, Enrique.Rivas@sanofi.com
†Deceased
‡Present address: Luis Bernal, Escuela de Medicina y Ciencias de la Salud, Universidad del Rosario, Bogotá, Colombia
Germán Áñez, Novavax, Inc., Gaithersburg, MD, United States