- 1Programa Graduado em Área da Biologia Básica e Aplicada (GABBA), Instituto de Ciências Biomédicas Abel Salazar (ICBAS), Universidade do Porto (UP), Porto, Portugal
- 2Global Health and Tropical Medicine (GHTM), Unidade de Parasitologia Médica, Instituto de Higiene e Medicina Tropical (IHMT), Universidade Nova de Lisboa (UNL), Lisboa, Portugal
- 3Instituto de Química de São Carlos (IQSC), Universidade de São Paulo (USP), São Carlos, Brazil
- 4Instituto de Engenharia e Ciências do Mar (ISECMAR), Universidade Técnica do Atlântico (UTA), São Vicente, Cape Verde
- 5Instituto de Engenharia e Ciências do Mar (ISECMAR), West African Science Service Center on Climate Change and Adapted Land-Use (WASCAL), Universidade Técnica do Atlântico (UTA), São Vicente, Cape Verde
- 6Instituto de Biologia (IB), Departamento de Biologia Animal, Universidade Estadual de Campinas (UNICAMP), São Paulo, Brazil
- 7Global Health and Tropical Medicine (GHTM), Unidade de Saúde Pública Internacional e Bioestatística, Instituto de Higiene e Medicina Tropical (IHMT), Universidade Nova de Lisboa (UNL), Lisboa, Portugal
- 8Laboratório de Inflamação e Doenças Infecciosas (LIDI), Departamento de Morfologia e Patologia (DMP), Universidade Federal de São Carlos (UFSCar), São Carlos, Brazil
- 9Instituto Nacional de Ciência e Tecnologia de Bioanalítica (INCTBio), Universidade Estadual de Campinas (UNICAMP), Campinas, Brazil
- 10Laboratório de Parasitologia, Instituto Nacional de Investigação Agrária e Veterinária, I.P., (INIAV), Oeiras, Portugal
Schistosomiasis is a chronic neglected tropical disease saddling millions of people in the world, mainly children living in poor rural areas. Praziquantel (PZQ) is currently the only drug used for the treatment and control of this disease. However, the extensive use of this drug has brought concern about the emergence of PZQ-resistance/tolerance by Schistosoma mansoni. Studies of Schistosoma spp. genome, transcriptome, and proteome are crucial to better understand this situation. In this in vitro study, we compare the proteomes of a S. mansoni variant strain stably resistant to PZQ and isogenic to its fully susceptible parental counterpart, identifying proteins from male and female adult parasites of PZQ-resistant and PZQ-susceptible strains, exposed and not exposed to PZQ. A total of 60 Schistosoma spp. proteins were identified, some of which present or absent in either strain, which may putatively be involved in the PZQ-resistance phenomenon. These proteins were present in adult parasites not exposed to PZQ, but some of them disappeared when these adult parasites were exposed to the drug. Understanding the development of PZQ-resistance in S. mansoni is crucial to prolong the efficacy of the current drug and develop markers for monitoring the potential emergence of drug resistance.
Introduction
Schistosomiasis is one important intravascular parasitic infection, being endemic in 76 countries with more than 97% of infected people living in Africa and parts of South America. This disease infects an estimative of 290 million people worldwide, especially children, living in poor rural areas of low and middle-income countries (1–4). Schistosoma mansoni and S. haematobium are the two major endemic parasite species causing Schistosomiasis. S. mansoni cercariae (shredded by snail hosts) penetrate the human skin and migrate into the vascular system, where mature male and female worms mate and produce ≈ 300 eggs per day (5). S. mansoni eggs provoke inflammatory responses and liver damages, which can lead to liver cirrhosis and portal hypertension, as well as an inability to perform a daily activity, headache, fever, syncope, nausea, elevated liver enzymes, malaise, fatigue and nocturnal sweats (4, 5).
Despite many efforts to control the transmission of this disease (6–8), essentially after the introduction of chemotherapeutic treatment in the 1980s, schistosomiasis is still highly prevalent (9). Its control is based on chemotherapy treatment using Praziquantel (PZQ) (10–16), which is effective, inexpensive, and easy to use (10, 16). However, frequent schistosome reinfection occurs, PZQ has minor activity against juvenile parasite forms but has a strong impact on adult worms (10, 17, 18). Because of its high prevalence, schistosomiasis has earned a Category II disease, ranking next to malaria, for importance as a target tropical disease by the World Health Organization special program for research and training in tropical diseases (1–3). Although the impact of schistosomiasis could be dramatically reduced by improvement in education and population awareness, basic sanitation conditions, as well as elimination of the intermediate host snails, such methods are not sufficient to control or eradicate this parasitosis. In the absence of vaccines (4), the control of this disease relies on chemotherapy to ease symptoms and reduce transmission. It is possible to induce resistance of S. mansoni and S. japonicum to PZQ in mice under laboratorial conditions, and reduced susceptibility to PZQ in the field isolates of S. mansoni has been found in many foci. Also, there are several schistosomiasis cases caused by S. haematobium infections in which repeated standard treatment fails to clear the infection (10, 19, 20). Understanding the development of PZQ-resistance in S. mansoni is crucial to prolong the efficacy of the current drug and develop markers for monitoring drug resistance (19, 20).
The identification of proteins is important for understanding how schistosomes regulate host immune systems to establish chronic infections and also elucidate other aspects of parasite–host interaction (21). Furthermore, a comprehensive deciphering of the schistosome genome, transcriptome, and proteome has become increasingly central for understanding the complex parasite–host interplay (12, 22–24). Therefore, such information can be expected to facilitate the discovery of vaccines and new therapeutic drug targets, as well as new diagnostic reagents for schistosomiasis control (21–23), and may aid the development of protein probes for selective and sensitive diagnosis of schistosomiasis (25). Proteomics approaches encompass the most efficient and powerful set of tools for the identification of protein complexes (26–28) and have been widely used to decipher the proteome of parasites such as nematodes (29) and trematodes (30–33). For Schistosoma spp., the proteome has been studied in many developmental life stages (34), including lung-schistosomula (35, 36), cercariae (36–38), egg (36, 39) and, adult worms (24, 25, 36, 40–42).
Two-dimensional electrophoresis (2-DE) is a technique for the separation and identification of proteins, based on pI values (isoelectric focusing, IEF) in one dimension and molecular mass by SDS-PAGE (sodium dodecyl sulfate-polyacrylamide gel electrophoresis) in the other (43). Liquid chromatography coupled with tandem mass spectrometry (LC-MS/MS) is one of the most commonly used methods to identify and characterize peptides and proteins in complex samples (44). To our knowledge, the S. mansoni PZQ-resistant strain proteome has not been yet reported thus an examination of global changes in gene expression following drug treatment or between isolates showing differential drug susceptibility may provide an entrée into the identification of genes underlying drug action or resistance.
Based on the need to identify the genes that underly drug action or resistance, this work aimed to compare the proteomes of a S. mansoni variant strain stably resistant to PZQ and isogenic to its fully susceptible parental counterpart, identifying proteins from male and female adult parasites of PZQ-resistant and PZQ-susceptible strains, exposed and not exposed to PZQ. This information represents substantial progress towards deciphering the adult parasite proteome. Furthermore, these data may constitute an informative source for further investigations into PZQ-resistance and increase the possibility of identifying proteins related to this condition, possibly contributing to avoid or decrease the likelihood of development and spread of PZQ-resistance. Improving the understanding of PZQ-resistant and PZQ-susceptible proteome would increase the possibilities of clarification of PZQ mode of action and resistant phenotype.
Material and Methods
Chemical and Reagents
Acetic acid, acetonitrile (ACN), ammonium bicarbonate (NH4HCO3), bovine serum albumin (BSA), chloroform, dithiothreitol (DDT), fetal bovine serum (FBS), formic acid (FA), glycerol, iodoacetamide (IAA), isopropyl alcohol, L-glutamine, penicillin–streptomycin solution, phenol, polyacrylamide gel, Praziquantel (C19H24N2O2), RPMI-1640 medium, sodium dodecyl sulfate (SDS), thiourea, triton X-100, tris(hydroxymethyl)aminomethane hydrochloride (Tris–HCl), urea, 1,2-diheptanoyl-sn-glycero-3-phosphatidylcholine (DHPC), 3-[(3-cholamidopropyl)dimethylammonio]-1-propanesulfonate (CHAPS), and 4-(2-Hydroxyethyl)piperazine-1-ethanesulfonic acid (HEPES) were purchased from Sigma-Aldrich/Merck (Darmstadt, Germany). Agarose and Trizol Invitrogen® reagent were by Thermo Fisher Scientific® (Waltham, Massachusetts, USA). Complete mini protease inhibitor cocktail tablets were obtained from Roche Diagnostics GmbH® (Mannheim, Germany). Bromophenol blue, Coomassie brilliant blue R-350, IPG buffer, and immobiline dry strip IPG (13 cm; pH 3−10 nonlinear gradient) were obtained from GE Healthcare® (Chicago, IL). Methanol and trifluoracetic acid (TFA) were by Panreac Química S.L.U. ® (Barcelona, Spain). Trypsin modified sequencing grade and trypsin came from Promega Corporation® (Madison, WI). Water was previously distilled and further deionized using a Milli-Q system Millipore (Millipore, Bedford, MA). All solutions were prepared on the day of the experiments.
Ethical Statement
The research study was reviewed and approved by the Ethics Committee and Animal, Faculty of Veterinary Medicine, UTL (Ref. 0421/2013). Animals were maintained and handled following the National and European Legislation (DL 276/2001 and DL 314/2003; 2010/63/EU adopted on September 22, 2010), concerning the protection and animal welfare, and all procedures were performed according to the National and European Legislation. Anesthetics and other techniques were used to reduce the pain and adverse effect on animals.
Parasite Samples
A resistant strain (RS) of S. mansoni that tolerates up to 1,200 mg PZQ/kg of mouse body weight (this dose compares to a dose of a single-dose treatment regime of 80 mg/kg in humans) was developed recently (45). As previously described in this strain the female and male parasites phenotypically presented different reactions to PZQ both in vivo and in vitro (males being more resistant than female parasites). This S. mansoni variant strain was selected from a fully susceptible parasite strain, by stepwise drug pressure, and is isogenic, except for the genetic determinants of PZQ-resistance phenotypes, and significantly different from the counterpart S. mansoni susceptible strain. As such, this S. mansoni PZQ-resistant strain represents a distinct and valuable model for the study of PZQ-resistance.
Thus, two different parasite isolates were used in this study: S. mansoni BH PZQ-susceptible strain (SS) from Belo Horizonte (BH), Minas Gerais state, Brazil, and a stable PZQ-resistant strain (RS) (IHMT/UNL) obtained from the same BH strain, as described by Pinto-Almeida et al. (45). These two parasite strains are routinely kept in their intermediate host Biomphalaria glabrata snails at the IHMT/UNL (Instituto de Higiene e Medicina Tropical, IHMT; Universidade Nova de Lisboa, UNL, Lisboa, Portugal). Mus musculus CD1 line male mice was chosen as the animal model for S. mansoni infection because it is a good host for this parasite mimicking the S. mansoni human infection (46). Mice infection occurred by natural transdermal penetration of cercariae, by exposing mice tails to about 100 cercariae of S. mansoni each.
Eight- to ten-week old adult parasites were recovered by perfusion of the hepatic portal system and mesenteric veins, according to Lewis (47), and washed twice in RPMI-1640, to remove contaminating hair and blood clots. Males and females of S. mansoni (resistant strain—RS and susceptible strain—SS to PZQ) were analyzed separately. Regarding the groups of adult parasites exposed to PZQ (EPZQ), after collecting, the parasites were transferred to 24-well culture plates containing RPMI-1640 culture medium, 0.2 mol/L L-glutamine, 0.01 mol/L HEPES, 0.024 mol/L NaHCO3, 10,000 units penicillin, and 10 mg streptomycin/ml, pH 7 and supplemented with 15% FBS. Five adult parasites were added on each well for each studied group for PZQ treatment: 1) PZQ-susceptible male (SM); 2) PZQ-susceptible female (SF); 3) PZQ-resistant male (RM), and 4) PZQ-resistant female (RF). Adult parasites were treated in culture, with 3.0 × 10−5 mol/L of PZQ during 24 h (this dose was chosen and used because it was optimized in our previous published work (45), it allowed us to differentiate between susceptible and resistant parasites in culture, it has an effect on the parasites but that it does not kill them) and, then washed twice with saline solution to clean any traces of culture medium and stored in Trizol at −80°C, for protein extraction later.
For the groups of adult parasites not exposed to PZQ (NEPZQ), adult parasites were kept in RPMI-1640 medium with no addition of drug during 24 h and then washed twice with saline solution to clean any traces of culture medium and, also stored in Trizol at −80°C, for post protein extraction. There are three experimental variables; (1) exposure (E) to Praziquantel (PZQ) or non-exposure (NE), (2) the strain of S. mansoni, susceptible (S) or resistant (R), and (3) the sex of the parasite (M/F). Accordingly, the experimental set up consisted of eight sample groups, four for parasites not exposed to PZQ (RM-NEPZQ, RF-NEPZQ, SM-NEPZQ, and SF-NEPZQ), and four for parasites exposed to PZQ (RM-EPZQ, RF-EPZQ, SM-EPZQ, and SF-EPZQ), all experimental groups were done in technical triplicate (2-DE gels were run three times on a single drug treated sample). After the treatment period, the proteins were immediately extracted, all worms were alive prior the protein extraction. In each experimental group proteins were extracted in bulk. The effect of male and female separation on parasite development was not tested in vitro in this work.
Preparation of Protein Extracts
S. mansoni adult parasite protein extracts were obtained using Trizol protocol, according to the manufacturer’s instructions (48). Briefly, the parasites were lysed and homogenized directly in Trizol reagent at room temperature (≈25°C). The homogenized samples were incubated at room temperature to permit complete dissociation of the nucleoprotein complex. After homogenization, we proceeded to the separation phase, adding chloroform and centrifugation of samples. The aqueous phase was removed and the interphase and organic phenol–chloroform phase was used for the protein isolation procedure. Next, isopropyl alcohol precipitation was performed and the pellet was solubilized in SBI buffer (7 mol/L urea, 2 mol/L thiourea, 0.015 mol/L DHPC, 0.5% Triton X-100, 0.02 mol/L DTT, and complete mini protease inhibitor cocktail tablets), according to Babu et al. (49) and stored at −80°C until use. Protein concentration in protein extracts was measured by Bradford assay (50), and the quality of the extract was verified in 12% uniform SDS-PAGE gels (42).
Two-Dimensional Electrophoresis
Two-dimensional electrophoresis (2-DE) gels was performed in triplicate with 240 μg of protein extracts, for each eight sample group (four not exposed and four parasites exposed to PZQ). To prepare samples for 2-DE, protein samples were diluted in rehydration solution containing 7 mol/L urea, 2 mol/L thiourea, 4% CHAPS, 0.5% IPG buffer, 1% DTT, and 0.002% bromophenol blue. The rehydration was carried out passively overnight for 12 h in a 13 cm pH 3–10 strip. The strips were then applied on an Ettan IPGphor 3 System (GE Healthcare, Piscataway, NJ, USA), for protein separation by isoelectric focusing (IEF), using the following conditions developed for this work: at a constant current 50 μA/strip, the voltage program started with a gradient up to 3.5 kV in 3 h, then a step of 3 h at 3.5 kV, then a total voltage of 64.0 kVh to the end.
After focusing, the strips containing proteins were reduced in an equilibration solution (50 mol/L Tris–HCl, pH 8.8, 6 mol/L urea, 20% glycerol, 2% SDS) containing 2% DTT, and then alkylated in the same solution containing 2.5% IAA. The Immobilized pH gradient (IPG) strips and molecular weight standards were then transferred to the top of 12% uniform SDS-PAGE gels, and sealed with 0.5% agarose. The second dimension was carried out using a Protein Plus Dodeca cell system (Bio-Rad Laboratories, Inc., Hercules, CA) under an initial current of 15 mA/gel for 15 min, followed by increasing the current to 50 mA/gel until the end of the run.
For 2-DE experiments, three biological sample replicas were done for the two-dimensional polyacrylamide gel electrophoresis for each group, thus making our experimental procedure more reproducible. Gels were fixed in 40% methanol/10% acetic acid solution and stained with coomassie brilliant blue R-350. The spots were visually normalized and evaluated using the ImageMaster® 2D Platinum 7.0 software (GE Healthcare Bio-Sciences, Uppsala, Sweden), according to the number of spots matching, and the results were exported and evaluated in SIMCA-P software (Umetrics, Umeå, Sweden) as previously described. Here, we built PCA models, from which it was possible to obtain statistically valid spots between groups using t-test and Jackknife. This was done in a way that the software would inform us on the proteins spots of interest (51).
In-Gel Digestion and Peptide Preparation for Mass Spectrometry Analysis
We selected spots that were consistently found in all replicates for each condition, and were large enough to excise and retain sufficient material for downstream processing. The protein spots selected for each group were manually excised, destained, reduced, alkylated, and digested in-gel with trypsin from the corresponding 2-DE gel for mass spectrometry (MS) identification. First, spots were washed in ultrapure water and then destained in a solution containing 50% methanol/2.5% acetic acid, for 2 h at room temperature. This step was repeated until clear of blue stain. The gel fragments were incubated in 100% ACN with occasional vortexing until gel pieces became white and shrank. Then, the solution was removed and spots were completely dried and ready for digestion. The in-gel digestion with trypsin modified sequencing grade reagents was done according to Shevchenko et al. (52). Briefly, protein digestion was conducted at 37°C overnight. After the incubation, the supernatant was transferred to a clean tube, and 30 μl of 5% FA/60% ACN solution was added to gel spots for the extraction of tryptic peptides. This procedure was performed two times for 30 min, under constant agitation. The supernatant was pooled to the respective tube containing the initial peptide solution. This solution was dried in a Speedvac concentrator Thermo Fisher Scientific (Waltham, MA) and the peptides were re-suspended in 8 μl of 0.1% FA solution. The peptides were desalted in reverse phase micro-columns ZipTip® C18 (Millipore Corporation, Billerica, MA), according to the manufacturer’s instructions (53). Peptides were dried again and resuspended in 50% ACN/0.1% TFA solution.
Nanoflow LC-MS/MS Analysis and Protein Identification
The digested peptides (50 µg) were analyzed in an Easy-nLC II nanoflow liquid chromatography system (nano-LC-MS/MS) (Thermo Fisher Scientific, Waltham, MA) in tandem with an LTQ-Orbitrap Velos mass spectrometer (Thermo Fisher Scientific), which was equipped with a nanoelectrospray Nano-Flex II (Thermo Fisher Scientific) operating in a positive ion mode (24). Nanoelectrospray voltage was set to 4.5 kV and source temperature at 220°C. The precursor ion was isolated using the data-dependent acquisition mode with a 2 m/z isolation width and sequentially the ten most intense ions were selected for fragmentation event by collision-induced dissociation (CID), at 35% normalized collision energy and 10 ms activation time. Maximum ion injection times were set to 100 ms for MS and 500 ms for MS/MS, with a resolution of 60,000 and a scan within m/z range from 400 to 2,000. Chromatographic separation was carried out a Thermo C18 capillary column (10 cm × 75 µm, 3 µm, 120 Å) protected by a guard Thermo C18 capillary column (2 cm × 100 µm i.d., 5 µm particle, 120 Å pore size). Ultrapure water containing 0.1% FA (solvent A) and ACN containing 0.1% FA (solvent B) was used as mobile phase. The separation was performed at room temperature with a constant flow rate of 0.3 µl/min, with a total acquisition time of 90 min, by employing the elution program as follows: linear gradient of 5% of solvent B during 5 min, ranging to 80% of solvent B over 85 min. Data acquisition was controlled by Xcalibur® 2.0.7 Software (Thermo Fisher Scientific) and converted in mgf files using MassMatrix® MS Data file conversion version 3.9 software.
Bioinformatics
The list of peptide and fragment mass values generated by the mass spectrometer for each spot were submitted to an MS/MS ion search using the Mascot® 2.0 software online search engine (Matrix Science Inc, Boston, MA, USA) to search the Nanoflow LC-MS/MS data against the NCBInr database Schistosoma_mansoni_NCBI_112014, November 2014. Mascot® software (used at the Mass Spectrometry Laboratory at Brazilian Biosciences National Laboratory, CNPEM, Campinas, Brazil) and also against WormBase ParaSite, was set with two tryptic missed cleavages, a peptide ion mass tolerance of 10 ppm, a fragment ion mass tolerance of 0.02 Da, a peptide charge state of 2+ and 3+, variable modifications of methionine (oxidation), and fixed modifications of cysteine (carbamidomethylation).
During the analysis, our samples were checked against a contaminant database supplied by the Global Proteome Machine [GPM-web-based, an open-source user interface for analyzing and displaying protein identification data by Craig and Beavis (54)]. All validated proteins had at least two independent spectra, with greater than 95.0% probability estimated by the Peptide Prophet algorithm of being present in the S. mansoni database as at least one unique peptide. To avoid random matches, only ions with an individual score above the indicated by the Mascot® to identity or extensive homology (p <0.05) were considered for protein identification. However, when the Mascot® score was not significant, but the percentage coverage and root mean squared error (RMSE) were in the same range as those of proteins with a significant match, proteins were deemed identified if additional parameters, such as its calculated pI and Mw, were in agreement with those observed for the actual gel spot and the species matched was S. mansoni.
The molecular function and biological process were assigned for the proteins identified according to information obtained from the Gene Ontology® (GO) database (55). The exact annotation for each protein was used in most cases. However, the catalytic activity category was used for all proteins with molecular functions associated with (GTPase, hydrolase, isomerase, kinase, ligase, lyase, oxidoreductase, transcription, and transferase activities). Binding category was used for all types of ligand identified (actin, ATP, calcium, GTP, magnesium ion, metal ion, protein domain-specific, and nucleotide bindings). Furthermore, there were other molecular function categories classified such as chaperone, motor, regulation of muscle contraction, structural, and transport. The proteins that had no associated known function were classified as “unknown”.
The list of proteins that were identified in all the experiments was analysed using the webserver g:Profiler, to perform functional enrichment analysis. Briefly, the g:GOSt functionality was used, considering a g:SCS threshold <0.05 for statistical significance (56).
Results
2-DE Separation of Proteins From S. mansoni PZQ-Resistant and PZQ-Susceptible Adult Parasites
Eight protein extracts were analyzed: four from parasites not exposed to PZQ (NEPZQ) [resistant males and females (assigned as RM-NEPZQ and RF-NEPZQ, respectively), and susceptible males and females (assigned as SM-NEPZQ and SF-NEPZQ, respectively)] and another four samples from parasites exposed to PZQ (EPZQ) [resistant males and females (assigned as RM-EPZQ and RF-EPZQ, respectively), and susceptible males and females (assigned as SM-EPZQ and SF-EPZQ, respectively)]. All protein extracts presented high purity and good quality for posterior 2-DE (Figure 1) and nano-LC-MS/MS analysis. The quality/purity of the protein extract was assessed as previously described by Rayna et al. (57), the quality/purity of the extract was verified in 12% uniform SDS-PAGE gels.
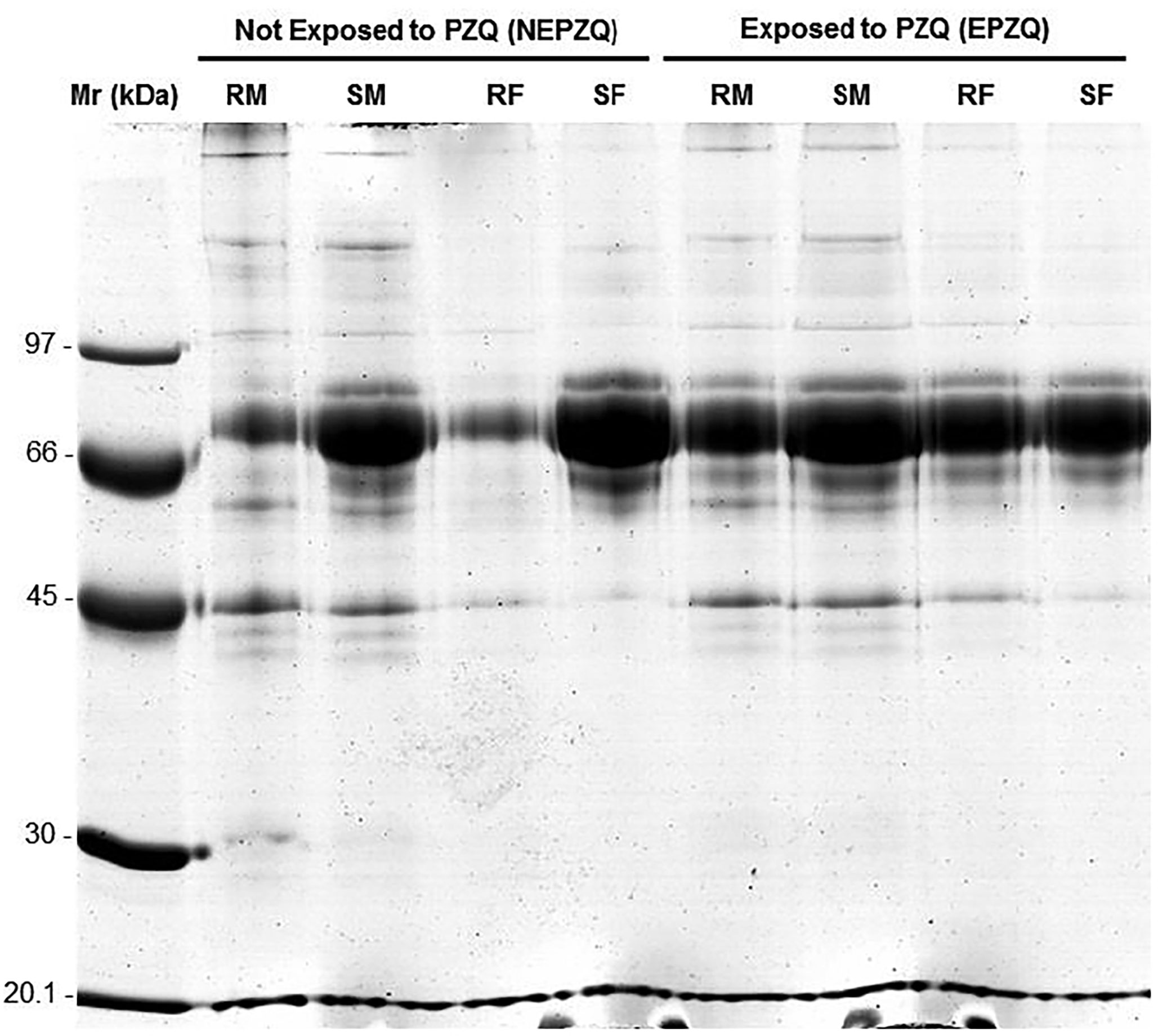
Figure 1 SDS-PAGE gel of the protein preparations in all eight conditions, improving the quality of the protein extracts studied. RM, resistant males; SM, susceptible males; RF, resistant females; SF, susceptible females; Mr, Molecular marker reference.
Analytical 2-DE gels were produced, to reproducibly resolve protein spots in a broad pH range and molecular weight, and to compare the protein pattern of S. mansoni proteome from the two strains (PZQ-resistant and PZQ-susceptible) not exposed (Figure 2) and exposed to PZQ (Figure 3). See Figures 2, 3, and Supplementary Data.
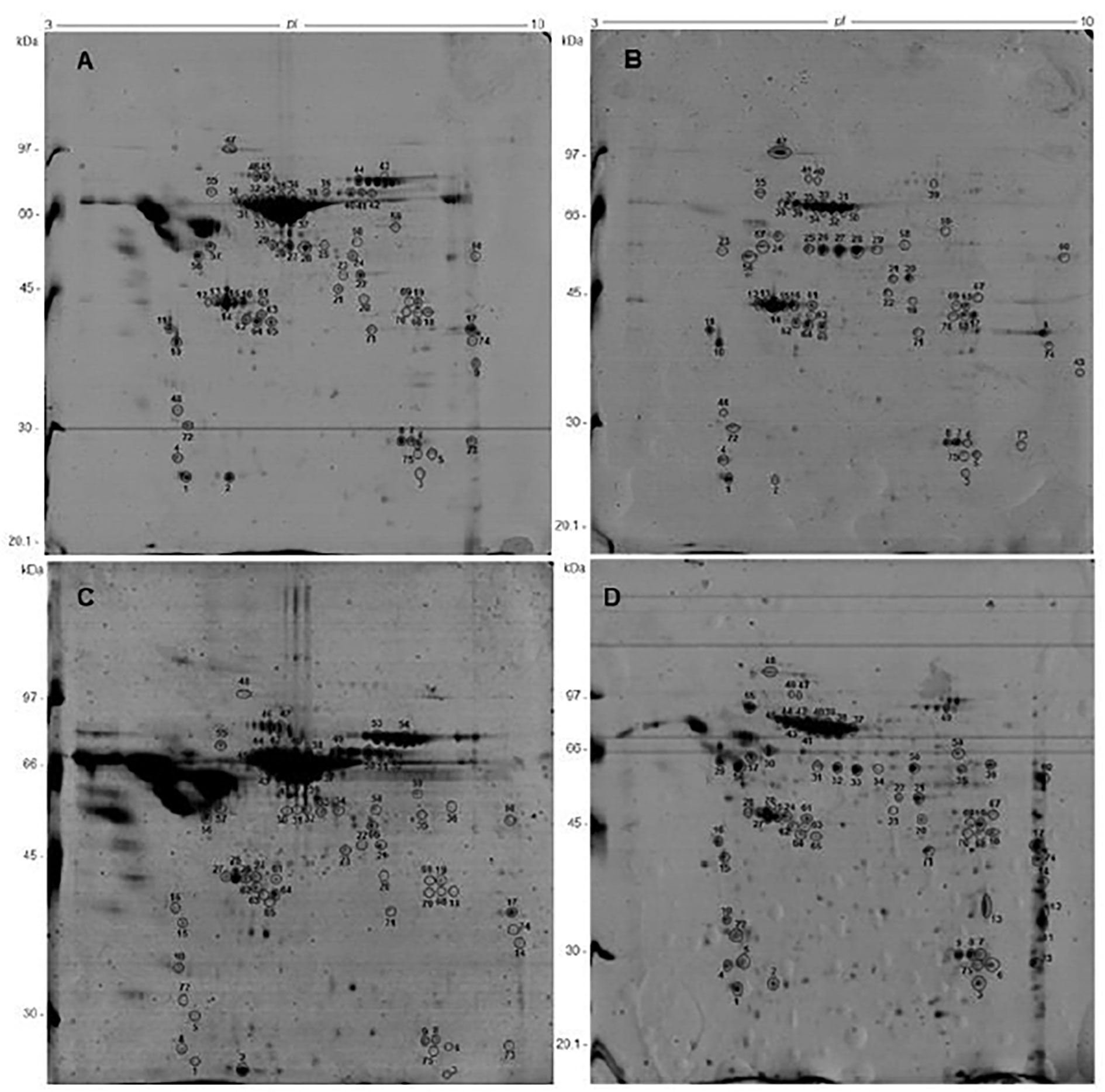
Figure 2 Comparative two-dimensional gel electrophoresis of protein samples from S. mansoni adult parasites not exposed to PZQ (baseline experiment), using 13 cm, pH 3-10NL strips and SDS-PAGE 12%, stained by coomassie brilliant blue: (A) SM-NEPZQ; (B) RM-NEPZQ; (C) SF-NEPZQ; (D) RF-NEPZQ. The identified spots, which were analyzed and identified by Nanoflow LC-MS/MS. All the identified proteins are listed in Supplementary Material Tables 1–3.
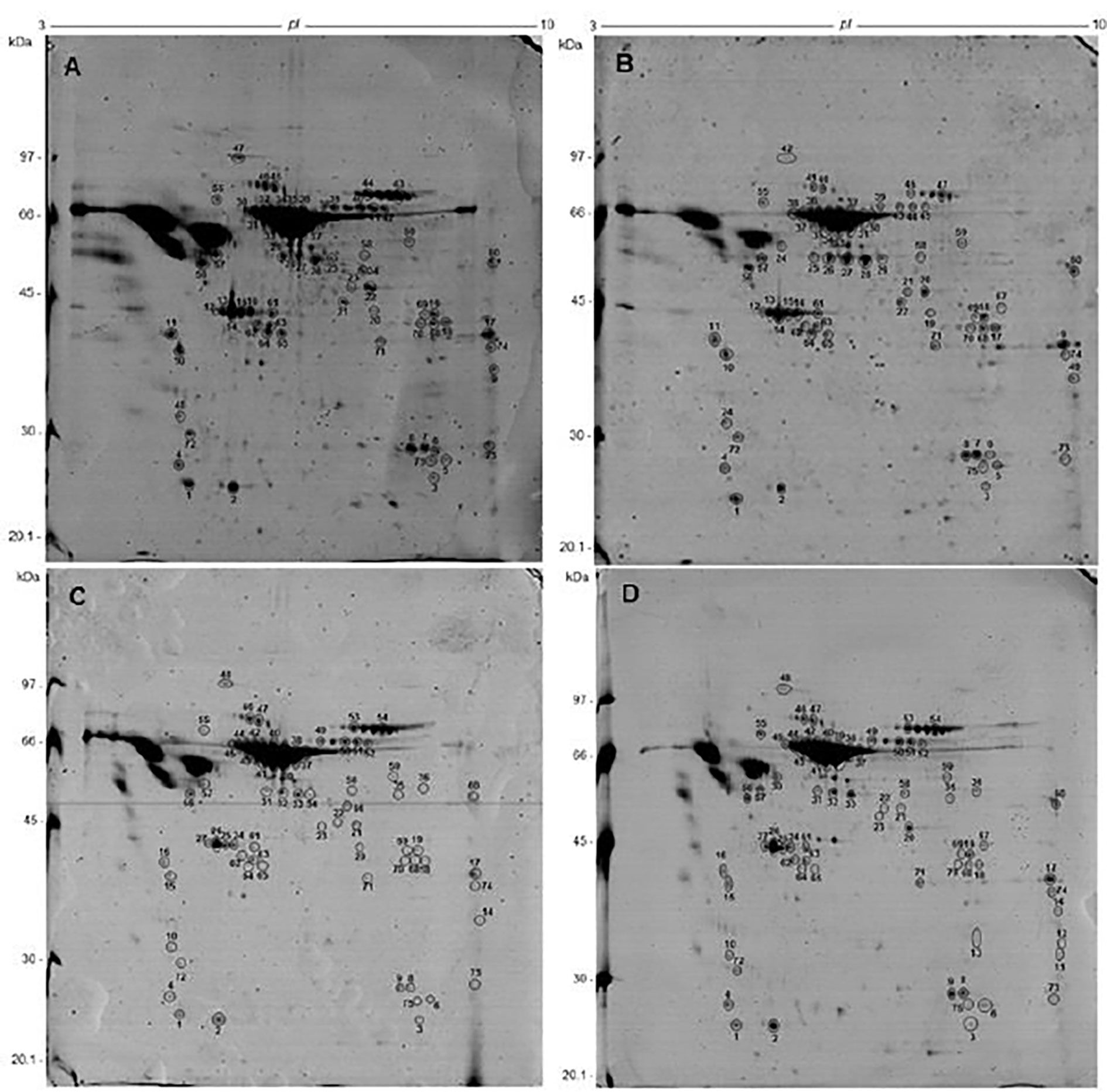
Figure 3 Comparative two-dimensional gel electrophoresis of protein samples from S. mansoni adult parasites exposed to PZQ using 13 cm, pH 3-10NL strips and SDS-PAGE 12%, stained by coomassie brilliant blue. (A) SM-EPZQ; (B) RM-EPZQ; (C) SF-EPZQ; (D) RF-EPZQ. The identified spots, which were analyzed and identified by Nanoflow LC-MS/MS. All the identified proteins are listed in Supplementary Material Tables 1–3.
Two-DE maps constructed with coomassie blue-stained gels showed reasonably comparable numbers of spots in all the samples. In total 133 ± 14, 265 ± 20, 142 ± 8, and 188 ± 34 spots were detected in proteins from RM-NEPZQ, RF-NEPZQ, SM-NEPZQ, and SF-NEPZQ, respectively (Table 1). For parasites exposed to PZQ, 203 ± 14, 133 ± 9, 220 ± 34, and 99 ± 19 spots were detected in RM-EPZQ, RF-EPZQ, SM-EPZQ, and SF-EPZQ, respectively (Table 1). Table 1 includes the data from the replicates. The spots that were validated were the same in each replicate. The only protein spots whose analysis was continued, were those where a protein spot is consistently expressed in the three replicates, if the protein spot is only expressed in one or two replicas then this spot is excluded from the subsequent analysis.
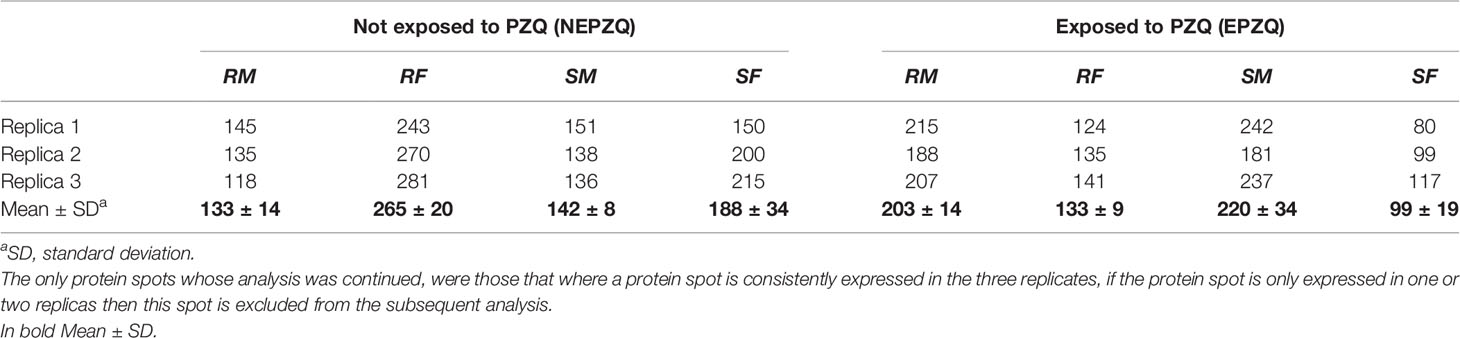
Table 1 Summary comparison of the number of protein spots in the 2-DE maps for the eight different proteome samples analyzed.
Nanoflow LC-MS/MS Analysis and Protein Identification
Gels were compared between replica and the only spots that were chosen to be excised were the ones that were more intense and that were present in all three replicas. The spots were excised from preparative gels for each sample, digested by trypsin, and identified by nanoflow LC-MS/MS. For RM-NEPZQ, 64 spots were successfully analyzed by nano-LC-MS/MS, as well as 69 from RF-NEPZQ, 67 from SM-NEPZQ, 68 from SF-NEPZQ, 68 from RM-EPZQ, 69 from RF-EPZQ, 67 from SM-EPZQ, and 66 spots from SF-EPZQ (Supplementary Material Tables 1–3). The nano-LC-MS/MS results were employed to search the database (NCBInr) by the Mascot® search engine, and the matched proteins are listed in Supplementary Tables 1, 2. Some proteins were identified in only one individual spot, but on several occasions, more than one spot in a gel corresponded to the same protein, or better, same protein species (Figures 2, 3, and Supplementary Material Tables 1–3).
Sixty individual protein species were identified on samples from parasites not exposed to PZQ, of which 45 were present in RM-NEPZQ, 52 in RF-NEPZQ, 45 in SM-NEPZQ, and 47 proteins in SF-NEPZQ (Supplementary Material). In this group of NEPZQ parasites, 35 proteins were common to all four protein extracts, eight occurred only in resistant adult parasites, eight only in susceptible adult parasites, four in female parasites, and three were only present in resistant females (Figure 4). Interestingly, two proteins that have shown to be common in RM-NEPZQ, RF-NEPZQ, and SM-NEPZQ preparations, namely serine/threonine phosphatase and troponin I, did not appear in SF-NEPZQ (Table 2).
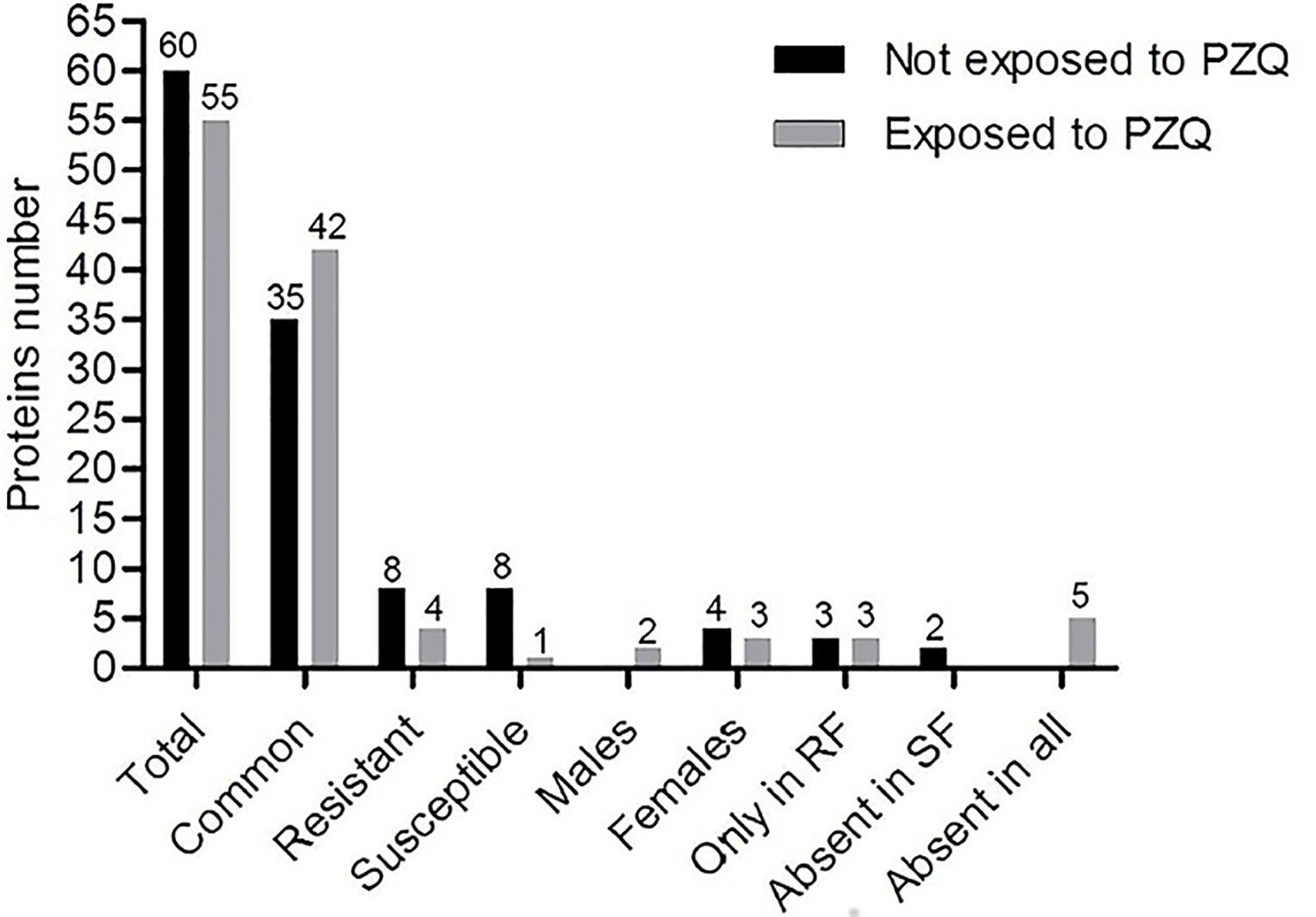
Figure 4 Number of shared proteins identified from parasites not exposed and exposed to Praziquantel.
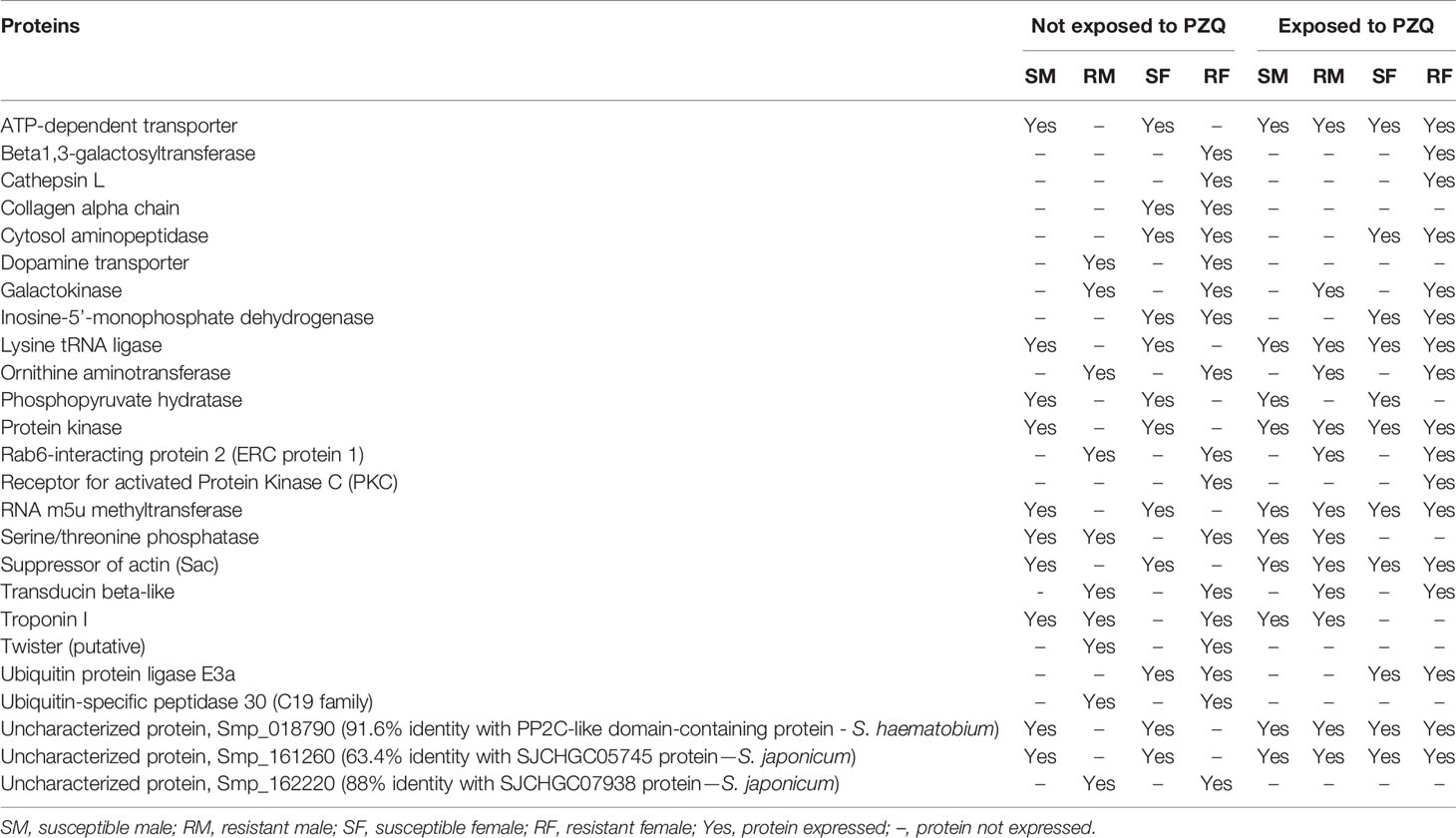
Table 2 Summary comparison of the specific proteins identified from parasites not exposed (NEPZQ) and exposed (EPZQ) to Praziquantel.
The total number of proteins identified was reduced to 55 proteins on the protein extracts from parasites exposed to PZQ. Forty-eight of those proteins were present in RM-EPZQ, 52 in RF-EPZQ, 45 in SM-EPZQ, and 46 in SF-EPZQ (Supplementary Material Tables 1–3). Forty-two proteins appeared to be common to all the four protein extracts of EPZQ parasites. Besides, four proteins were present only in resistant strain, one in susceptible strain, three were exclusive of female parasites, two proteins were present only in male parasites, and three were only in resistant females (Figure 4). Five proteins that were present on parasites not exposed to PZQ, namely, collagen alpha chain, dopamine transporter, twister (putative), ubiquitin-specific peptidase 30 (C19 family), and Smp_162220, did not appear here (Table 2).
Molecular Function of Identified Proteins
The proteins identified by nano-LC-MS/MS, summarized in Supplementary Tables 1, 2, were generally categorized by their molecular function, according to information obtained from the GO database (Table 3), we consider that this general classification in large groups of protein functions is fundamental as a basis for future proteomics and transcriptomic tests. When proteins had another function annotation, they were shown in brackets. The biological process and subcellular localization assigned to each protein in that database are also included in Table 3. The results allowed the identification of proteins categorized as binding, catalytic, transport, regulation of muscle contraction, chaperone, motor, structural activities, and proteins of unknown functions. Among the molecules identified as binding proteins, most of them were ATP, nucleotide, protein, and ion binding proteins. The proteins categorized correspond to a variety of biological processes, nevertheless, most of them were glycolytic enzymes and proteins related to the metabolic process.
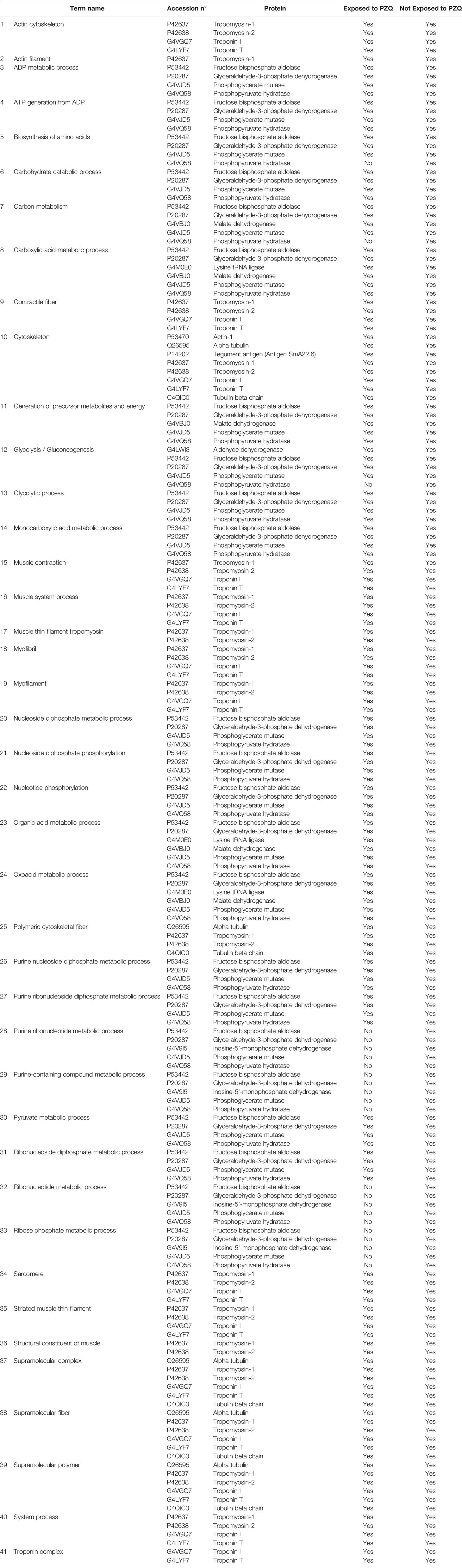
Table 3 Proteins identified by MS/MS and categorized by their molecular function according to information obtained from GO database.
Regarding the subcellular localization, the proteins identified were classified, as cytoskeletal, cytosolic, nuclear, membrane proteins, and some of them were located on extracellular matrix. Among them, those most abundantly identified were cytosolic proteins. There were fifteen and seven proteins whose biological process and subcellular localization, respectively, were not predicted (Table 3).
Functional Enrichment Analysis
In all experimental conditions, except in both exposed or not exposed susceptible females, the GO Molecular Processes with the highest p-values were related to muscle contraction (GO:0006936, GO:0003012, GO:0003008), corresponding to UniProt geneIDs P42637, P42638, G4VGQ7, G4LYF7. However, in susceptible females, both exposed and unexposed, the Molecular Processes with the highest p-values were unique and related to ADP metabolic process and ATP generation from ADP (GO:0046031, GO:0006757, GO:0009135, GO:0006096, GO:0009179, GO:0009185, GO:0006165, GO:0046939, GO:0006090, and GO:0009132). These GO terms were associated with gene IDs P53442, P20287, G4VJD5, and G4VQ58 (Table 4) (Supplementary Tables 1, 3).
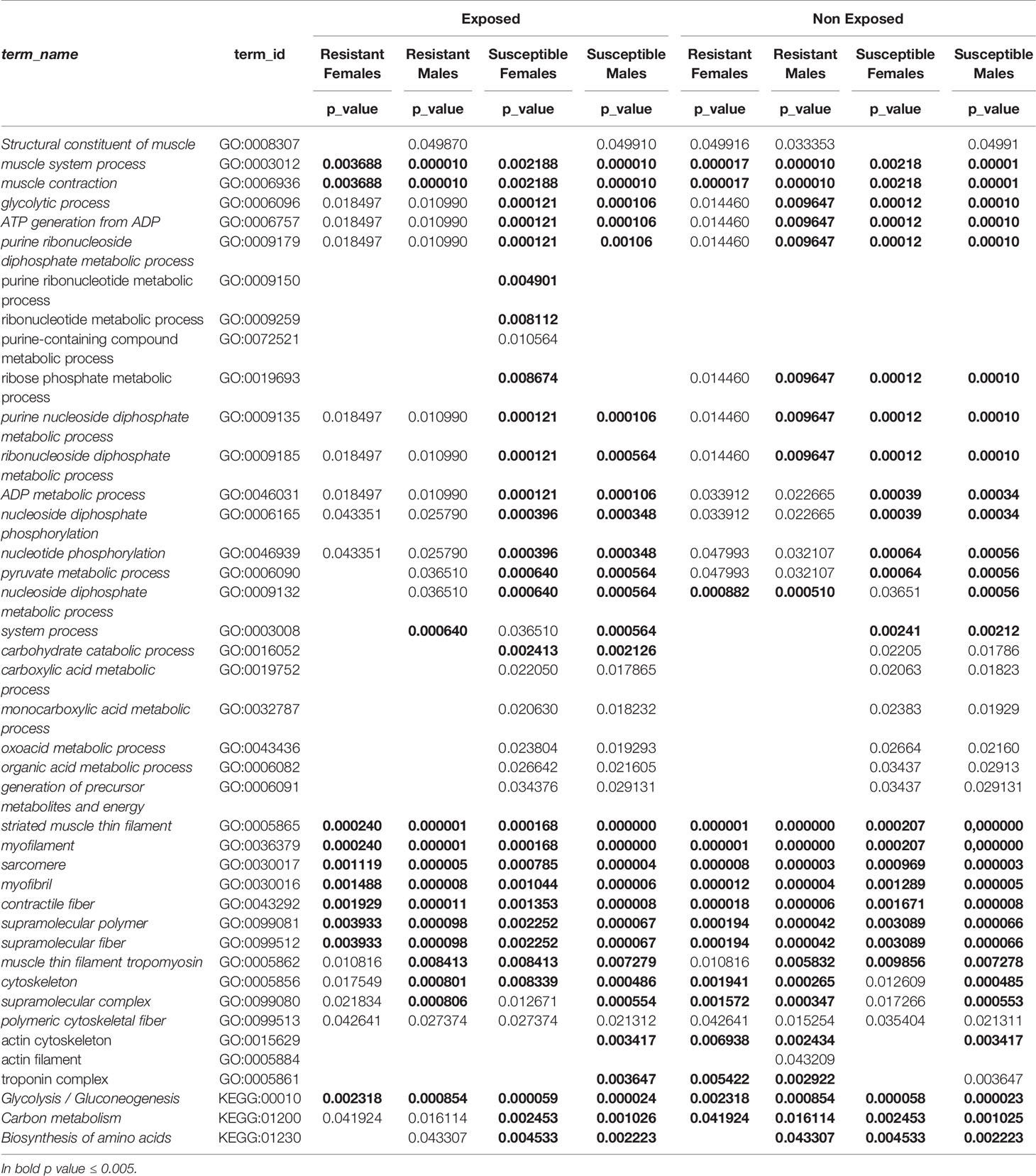
Table 4 Summary comparison of the specific proteins identified from parasites not exposed (NEPZQ) and exposed (EPZQ) to Praziquantel on gene ontology analyses.
Discussion
Nowadays the control of schistosomiasis is based on PZQ, which is the only drug available for its treatment. The WHO strategy for schistosomiasis control focuses on reducing disease through periodic, targeted treatment with PZQ through the large-scale treatment of affected populations. It involves regular treatment of all at-risk groups (6, 8, 12, 16). However, the existence of reports of PZQ-resistance cases by S. mansoni (as well as by S. haematobium) has become a serious problem that needs to be solved. Some studies have suggested that resistance to Schistosoma infection can be acquired naturally or induced by the drug (20, 45, 58–60). For example, Sanchez et al. (20) examined the effect of PZQ in strains of S. mansoni. The authors noted a reduced miracidia susceptibility after exposure to 2 × 50 mg/Kg of PZQ. It has also been reported the susceptibility of worms in vivo (mice infected with schistosomes) after a lethal dose of 3 × 300 mg/kg of PZQ, reducing in only 10% the worm number in infected rodents. Besides experimental evidence, reports of treatment failure in Senegal and Egypt with reduced susceptibility to PZQ were obtained (61, 62) and, further in vitro experiments have confirmed the development of PZQ-resistance (9, 10, 43, 61–64).
In the present study we identified 60 different proteins on S. mansoni proteome, this corresponds to 0.42% of the full S. mansoni proteome (https://www.uniprot.org/proteomes/UP000008854). All those proteins were present in adult parasites not exposed to PZQ, but some of them disappeared when these adult parasites were exposed to the drug. This result could indicate the involvement of PZQ exposure on those protein expressions in resistant and susceptible strains. With this, we are not unequivocally stating that these proteins are the only ones or that they are at all responsible for the phenotype of PZQ drug resistance but this work opens the door to new studies using proteomics, transcriptomics, and metabolomics on the importance of these proteins in the phenotype and genotype of resistance to PZQ.
Although previous studies of Schistosoma proteome had been performed using protein extracts and Schistosoma species different from ours, some proteins, such as 14-3-3 protein, HSP-70, GAPDH, glutathione S-transferase 28 kDa, enolase, fructose-bisphosphate aldolase, actin, triosephosphate isomerase, calponin, elongation factor 1-α, phosphoglycerate kinase, phosphoglycerate mutase, myosin, and paramyosin were commonly identified (36, 42, 65–70). In addition, some proteins that have already been tested as vaccine candidates, as glutathione S-transferase 28 kDa (71), triosephosphate isomerase (71, 72), and paramyosin (71), were also identified in the present study. Major egg antigen, troponin T, disulphide-isomerase ER-60, and actin, proteins that we also found, have already been clustered as immunoreactive proteins in serum pools of infected or non-infected individuals from endemic area (42).
Looking at the proteomes from both genders, in this survey, four proteins were only present in females from both strains, even under exposure to PZQ, namely, cytosol aminopeptidase, inosine-5′-monophosphate dehydrogenase (IMPDH), ubiquitin protein ligase E3a, and collagen alpha chain. Cytosol/leucine aminopeptidase catalyzes the hydrolysis of amino acid residues from the N-terminus of proteins and peptides (73), and it has already been assessed as a vaccine candidate against the infection of Fasciola hepatica (74). This protein has previously been identified in S. mansoni eggs (75). Regarding IMPDH, this protein is responsible for the rate-limiting step in guanine nucleotide biosynthesis (76), and it has previously been identified in the Schistosoma genome and transcriptome (77). E3 ligase enzyme catalyzes protein ubiquitination, which regulates various biological processes through covalent modification of proteins and transcription factors, and ubiquitin is the most important protein of this process (78, 79). Ubiquitination is of interest in S. mansoni because this process could be a potential target for the design of new drugs (80), being ubiquitin protein ligase E3a a good target to be studied. Concerning collagen alpha chain, Yang et al. (81) described that silencing the expression of a type of collagen (type V collagen) significantly affects the spawning and egg hatching of S. japonicum, and it also affects the morphology of the adult parasites (81). Therefore, it would be very interesting to evaluate the role of each of these proteins in PZQ-resistance, especially, collagen alpha chain, since it seems to produce morphological alterations in eggs and adult parasites of S. mansoni PZQ-resistant strain (10, 11). Moreover, females of this species, the only gender in which those proteins were found in this work, are more tolerant to PZQ treatment than males (45).
Another large difference between the proteome of both genders was the expression of troponin I and serine/threonine-protein phosphatase. These proteins were present in males independently of drug exposure, but in females, they were only present in resistant females not exposed to PZQ. Troponin I belongs to the troponin complex that mediates Ca2+ regulation that governs the actin-activated myosin motor function in striated muscle contraction (82). On the other side, protein kinases and phosphatases, as is the case of serine/threonine-protein phosphatase, are essential for the normal functioning of signaling pathways since it is well known that reversible phosphorylation of proteins is a ubiquitous mechanism crucial for the regulation of most cellular functions (83). In S. mansoni, a phosphatase 2B (calcineurin) has been described as a heterodimer with a catalytic subunit and a regulatory subunit, which bind to Ca2+ increased the phosphatase activity (84). Thus, protein phosphatases represent crucial molecules for the parasite and hence potential chemotherapeutic targets (85).
Regarding resistant strain parasites, it is notable the finding of eight proteins exclusively present in those S. mansoni adult parasites. From those eight proteins, dopamine transporter, twister (putative), ubiquitin-specific peptidase 30 (C19 family), and uncharacterized protein smp_162220 were not detected in the proportion of the proteome we analysed in S. mansoni PZQ-exposed adult parasites. However, galactokinase, ornithine aminotransferase, Rab6-interacting protein 2 (ERC protein 1), and transducin beta-like remained after drug exposure. Dopamine/norepinephrine transporter (SmDAT) gene transcript, characterized in S. mansoni, is essential for the survival of the parasite as it causes muscular relaxation and a lengthening in the parasite, controlling movement (86). Galactokinase catalyzes the second step of the Leloir pathway, a metabolic pathway found in most organisms for the catabolism of β-D-galactose to glucose 1-phosphate (87). Galactokinase and hexokinase have a similar enzymatic function on sugar phosphorylation (88), and characterization of schistosome hexokinase has been described as pertinent to understanding the metabolic response of S. mansoni cercariae to an increased glucose availability (89). Ornithine aminotransferase was already identified in S. mansoni (90) and it has been characterized as playing a central role in ornithine biosynthesis (91). It seems responsible for catalyzing the transfer of the delta-amino group of L-ornithine to 2-oxoglutarate, producing L-glutamate-gamma-semialdehyde, which in turn spontaneously cyclizes to pyrroline-5-carboxylate, and L-glutamate (92). Rab6-interacting protein 2 is a member of a family of RIM-binding proteins, which are presynaptic active zone proteins that regulate neurotransmitter release (93). Ubiquitin-specific peptidase 30 (C19 family) belongs to a metabolic pathway that had previously been associated with the development of artemisinin and artesunate-resistance in Plasmodium chabaudi (94), which is a very interesting result. All those proteins specifically found in the resistant strain should be further studied to better understand if they could have a fundamental role in PZQ-resistance development.
Yet, for the resistant strain parasites, there are three proteins, beta 1,3-galactosyl-transferase, cathepsin L, and receptor for activated Protein Kinase C (PKC) that are exclusive to resistant females, even after exposure to PZQ. Beta 1,3-galactosyltransferase has previously been identified in the Schistosoma genome and transcriptome (77). Cathepsin L activity is believed to be involved in hemoglobin digestion by adult schistosomes (95), and these authors suggested the involvement of cathepsin proteinases in several key functions render them as potential targets to novel antiparasitic chemotherapy and immunoprophylaxis. Putative PKC exists in kinomes of the blood flukes S. mansoni (20, 96, 97), S. japonicum (98), S. haematobium (99), and regulates movement, attachment, pairing, and egg release in S. mansoni, being considered a potential target for chemotherapeutic treatment against schistosomiasis (100). These results need further investigation thus considering that the PZQ mode of action is not completely clarified nor is its genetic process of susceptibility lost, our results might help in the process of clarification of a possible relationship between those proteins and PZQ-resistance in S. mansoni females (20, 45). Concerning the PZQ-susceptible strain, we noted eight proteins that only appeared in this strain. Phosphopyruvate hydratase (enolase), an important glycolytic enzyme that has the functions of activating the plasminogen, involving in the processes of infection and migration of parasites, reducing the immune function of the host as well as preventing parasites from the immune attack of the host (101), is the only protein from those eight proteins that continued to be present after PZQ exposure.
Our gene enrichment analyses also provided functional differences when comparing males and females. The results identified in susceptible females, either exposed or not exposed, a significantly higher p-value for GO terms that were related to ATP generation from ADP and other GO terms within the ancestor chart of this term. On the other hand, susceptible males presented significantly higher p-values for GO terms associated with muscle contraction. This give us an indication of a higher fitness and active metabolic status of susceptible females both in the presence or absence of Praziquantel when compared to susceptible males. Also, processes related to monocarboxylic acid metabolic processes were identified only in susceptible males and females, both exposed and unexposed. This could also suggest that loss of susceptibility/resistance to Prazinquantel come at the compromise of the fitness level, with lower metabolic levels both in males and females.
These results altogether represent an important finding for the study of PZQ-resistance/susceptibility in S. mansoni, since they allow comparing directly the proteome under both conditions. We believe that the most promising candidates are proteins that appeared associated only to one of the strains, especially those with functions possibly related to the phenotypic alterations observed by Pinto-Almeida et al. (11), or previously associated with resistance by other parasites to different drugs. These candidates require special attention in more studies, assessing for instance the level of protection induced by these proteins in animal models infected by both S. mansoni strains, as they may have some involvement in the PZQ-resistance phenomenon, although further studies are needed to deepen this knowledge.
Conclusions
Here, we investigated the proteome of a PZQ-resistant S. mansoni strain and the respective isogenic susceptible strain, an important step towards a full clarification on the genetic process of PZQ-resistance considering that we were able to compare a PZQ-resistant parasite line with the susceptible one from with it was obtained allowing a more accurate comparison between strains that differ solely on PZQ susceptibility phenotype. This study allowed us to identify proteins that in our proteome analyses differ between PZQ-susceptible and PZQ-resistant parasites, however, their relationship or contribution towards the mechanism of resistance remains unclear and strongly needs further clarification. Since these proteins may be involved with the PZQ-resistance phenomenon, their functional characterization should be pursued in future studies aiming at the discovery of new drug targets for schistosomiasis control and also studies that could clarify the PZQ mode of action.
The identification of the proteins putatively associated with PZQ-resistance in S. mansoni permits also to investigate the possibility of developing a diagnostic test to distinguish patients carrying PZQ-resistant strains from those with PZQ-susceptible S. mansoni. The development of such a test would constitute a major step towards schistosomiasis control as it would render it possible to adjust drug administration to increase treatment efficacy, perhaps even by combining PZQ with efflux pump inhibitors as suggested previously (45). The proteome analysis made it also possible to identify proteins that were present only in females, being them good targets to identify the mechanisms underlying the decreased PZQ-susceptibility of females, when compared to males. Furthermore, some of these proteins may constitute targets, for schistosomiasis control. They should, therefore, be the object of further analysis. In this context, the development and use of other techniques, such as genetic manipulation methods, will be crucial to further unravel the phenomenon/problem of PZQ-resistance/tolerance/loss of susceptibility. Therefore, this work opens doors to other PZQ-resistance studies, and could represent a basis to find a solution to the PZQ-resistance problem in a disease that affects millions of people worldwide.
Data Availability Statement
The original contributions presented in the study are publicly available. This data can be found here: https://datadryad.org/stash/share/_nzZNotPFuNIsBY1R5x1XjDy3XUFKGAEsGBDFNl3yWM.
Ethics Statement
The research project was reviewed and approved by the Ethics Committee and Animal Welfare, Faculty of Veterinary Medicine, UTL (Ref. 0421/2013). Animals were maintained and handled in accordance with National and European legislation (DL 276/2001 and DL 314/2003; 2010/63/EU adopted on 22nd September 2010), with regard to the protection and animal welfare, and all procedures were performed according to National and European Legislation. The anesthetics and other techniques were used to reduce the pain and adverse effect of animal.
Author Contributions
AP-A: Conceptualization, methodology, software, validation, formal analysis, investigation, resources, data curation, writing - original draft, writing - review and editing, and visualization. TM: Conceptualization, methodology, investigation, resources, writing - original draft, and writing - review and editing. PF: Writing - original draft, writing - review and editing, and experimental studies. ABA: Writing - review and editing, and gene ontology analysis. SB: Conceptualization, writing - original draft, and writing - review and editing. FA: Writing - original draft, writing - review and editing, and funding acquisition. SA: Resources, writing - original draft, and writing - review and editing. CG: Writing - review and editing. EC: Conceptualization, methodology, software, validation, formal analysis, data curation, writing - original draft, writing - review and editing, visualization, supervision, project administration, and funding acquisition. AA: Conceptualization, methodology, software, validation, formal analysis, investigation, research, data curation, writing - original draft, writing - review and editing, visualization, supervision, project administration, and funding acquisition. All authors contributed to the article and approved the submitted version.
Funding
This work was initially supported by Fundação para a Ciência e a Tecnologia de Portugal (FCT) by grant PEst-OE/SAU/UI0074/2014. AP-A was initially funded by Graduate Program in Areas of Basic and Applied Biology (GABBA) from the Instituto de Ciências Biomédicas Abel Salazar, Universidade do Porto and FCT (SFRH/BD/51697/2011) meanwhile received the António Coutinho Science Award (11/BI-PD/20) by the Instituto Gulbenkian de Ciência (IGC) of the Fundação Calouste Gulbenkian, and Fundação Familia Merk and Câmara Municipal de Oeiras, and nowadays is funded by the German Federal Ministry of Education and Research (BMBF), the West African Science Service Centre on Climate Change and Adapted Land Use (WASCAL) through WASCAL Graduate Studies Programme in Climate Change and Marine Sciences at the Institute for Enginneering and Marine Sciences, Atlantic Technical University, Cabo Verde. The Brazilian agencies that were involved in this project are Conselho Nacional de Desenvolvimento Científico e Tecnológico - CNPq Proc. Nrs 400168/2013-8, and 375781/2013-7 that funded AA, and Fundação de Amparo a Pesquisa no Estado de São Paulo – FAPESP Proc. Nrs 2009/54040-8, 2009/16598-7, and 2008/04050-4 that funded infrastructure to EC. Project FAPESP 2014/07331-5 funded infrastructure to FA. This study was financed in part by the Coordenação de Aperfeiçoamento de Pessoal de Nível Superior – Brasil (CAPES) – Finance Code 1742613 and 88882.317690/2019-01, as a fellowship to CG and Finance Code 001 as a fellowship to TM. AA is now being funded by Fundação para a Ciência e Tecnologia (FCT) of Portugal PTDC/CVT-CVT/28798/2017. Fundação para a Ciência e Tecnologia (FCT) of Portugal provided funds to GHTM (UID/Multi/04413/2020) funded PF, ABA, and SB.
Conflict of Interest
The authors declare that the research was conducted in the absence of any commercial or financial relationships that could be construed as a potential conflict of interest.
Acknowledgments
We acknowledge the Mass Spectrometry Laboratory at Brazilian Biosciences National Laboratory, CNPEM, Campinas, Brazil for their support with the mass spectrometry analysis. The authors would like to thank Professor Ana Tomás from IBMC and GABBA program (Porto, Portugal), for very helpful suggestions that improved greatly this paper.
Supplementary Material
The Supplementary Material for this article can be found online at: https://www.frontiersin.org/articles/10.3389/fitd.2021.664642/full#supplementary-material and https://datadryad.org/stash/share/_nzZNotPFuNIsBY1R5x1XjDy3XUFKGAEsGBDFNl3yWM
References
1. Hay SI. Global, Regional, and National Disability-Adjusted Life-Years (DALYs) for 333 Diseases and Injuries and Healthy Life Expectancy (HALE) for 195 Countries and Territories, 1990–2016: A Systematic Analysis for the Global Burden of Disease Study 2016. Lancet (2017) 390:1260–344. doi: 10.1016/S0140-6736(18)32335-3
2. Global Health Metrics. Global, Regional, and National Incidence, Prevalence, and Years Lived With Disability for 328 Diseases and Injuries for 195 Countries, 1990–2016: A Systematic Analysis for the Global Burden of Disease Study 2016. Lancet (2017) 390:1211–59. doi: 10.1016/S0140-6736(17)32154-2
3. WHO. Schistosomiasis and Soil-Transmitted Helminthiases: Number of People Treated in 2016. Wkly. Epidemiol. Rec. (2017) 49:749–60.
4. Langenberg MCC, Hoogerwerf MA, Koopman JPR, Janse JJ, Kos-van Oosterhoud J, Feijt C, et al. A Controlled Human Schistosoma mansoni Infection Model to Advance Novel Drugs, Vaccines and Diagnostics. Nat Med (2020) 26:326–32. doi: 10.1038/s41591-020-0759-x
5. Colley DG, Bustinduy AL, Secor WE, King CH. Human Schistosomiasis. Lancet (2014) 383:2253–64. doi: 10.1016/S0140-6736(13)61949-2
6. Doenhoff MJ, Kimani G, Cioli D. Praziquantel and the Control of Schistosomiasis. Parasitol Today (2000) 16:364–6. doi: 10.1016/S0169-4758(00)01749-X
7. Fenwick A, Savioli L, Engels D, Bergquist NR, Todd MH. Drugs for the Control of Parasitic Diseases: Current Status and Development in Schistosomiasis. Trends Parasitol (2003) 19:509–15. doi: 10.1016/j.pt.2003.09.005
8. Hagan P, Appleton CC, Coles GC, Kusel JR, Tchuem-Tchuenté LA. Schistosomiasis Control: Keep Taking the Tablets. Trends Parasitol (2004) 20:92–7. doi: 10.1016/j.pt.2003.11.010
9. King CH. Toward the Elimination of Schistosomiasis. N Engl J Med (2009) 360:106–9. doi: 10.1056/nejmp0808041
10. Cioli D, Pica-Mattoccia L, Basso A, Guidi A. Schistosomiasis Control: Praziquantel Forever? Mol Biochem Parasitol (2014) 195:23–9. doi: 10.1016/j.molbiopara.2014.06.002
11. Pinto-Almeida A, Mendes T, de Oliveira RN, de A.P. Corrêa S, Allegretti SM, Belo S, et al. Morphological Characteristics of Schistosoma mansoni PZQ -Resistant and -Susceptible Strains are Different in Presence of Praziquantel. Front Microbiol (2016) 7:2016.00594. doi: 10.3389/fmicb.2016.00594
12. Panic G, Coulibaly JT, Harvey N, Keiser J, Swann J. Characterizing the Biochemical Response to Schistosoma mansoni Infection and Treatment With Praziquantel in Preschool and School Aged Children. J Proteome Res (2018) 17:2028–33. doi: 10.1021/acs.jproteome.7b00910
13. Schneeberger PHH, Coulibaly JT, Panic G, Daubenberger C, Gueuning M, Frey JE, et al. Investigations on the Interplays Between Schistosoma mansoni, Praziquantel and the Gut Microbiome. Parasites Vectors (2018) 11:1–12. doi: 10.1186/s13071-018-2739-2
14. Mduluza T, Mutapi F. Putting the Treatment of Paediatric Schistosomiasis Into Context. Infect Dis Poverty (2017) 6:1–6. doi: 10.1186/s40249-017-0300-8
15. Montresor A, Garba A. Treatment of Preschool Children for Schistosomiasis. Lancet Glob Heal (2017) 5:e640–1. doi: 10.1016/s2214-109x(17)30202-4
16. Cioli D, Pica-Mattoccia L. Praziquantel. Parasitol Res (2003) 90:S3–9. doi: 10.1007/s00436-002-0751-z
17. Xiao SH, Sun J, Chen MG. Pharmacological and Immunological Effects of Praziquantel Against Schistosoma japonicum: A Scoping Review of Experimental Studies. Exp Parasitol (2018) 183:56–63. doi: 10.1186/s40249-018-0391-x
18. de Oliveira CNF, Frezza TF, Lúcia Garcia V, Figueira GM, Mendes TMF, Allegretti SM. Schistosoma mansoni: In Vivo Evaluation of Phyllanthus amarus Hexanic and Ethanolic Extract. Exp Parasitol (2017) 183:56–63. doi: 10.1016/j.exppara.2017.10.008
19. Wang W, Wang L, Liang YS. Susceptibility or Resistance of Praziquantel in Human Schistosomiasis: A Review. Parasitol Res (2012) 111:1871–7. doi: 10.1007/s00436-012-3151-z
20. Sanchez MC, Cupit PM, Bu L, Cunningham C. Transcriptomic Analysis of Reduced Sensitivity to Praziquantel in Schistosoma mansoni. Mol Biochem Parasitol (2019) 228:6–15. doi: 10.1016/j.molbiopara.2018.12.005
21. Knudsen GM, Medzihradszky KF, Lim K-C, Hansell E, McKerrow JH. Proteomic Analysis of Schistosoma mansoni Cercarial Secretions. Mol Cell Proteomics (2005) 4:1862–75. doi: 10.1074/mcp.m500097-mcp200
22. Hu W, Brindley PJ, McManus DP, Feng Z, Han Z-G. Schistosome Transcriptomes: New Insights Into the Parasite and Schistosomiasis. Trends Mol Med (2004) 10:217–25. doi: 10.1016/j.molmed.2004.03.002
23. Verjovski-Almeida S, Leite LCC, Dias-Neto E, Menck CFM, Wilson RA. Schistosome Transcriptome: Insights and Perspectives for Functional Genomics. Trends Parasitol (2004) 20:304–8. doi: 10.1016/j.pt.2004.04.011
24. Mendes TMF, Carrilho E, Afonso AJPFS, Galinaro CA, Cabral FJ, Allegretti SM. Proteomic, Metabolic and Immunological Changes in Biomphalaria glabrata Infected With Schistosoma mansoni. Int J Parasitol (2019) 49:1049–60. doi: 10.1016/j.ijpara.2019.08.001
25. Wang Z-Q, Cui S-J, Liu F, Hu W, Feng Z, Han Z-G. Excretory/secretory Proteome of the Adult Developmental Stage of Human Blood Fluke, Schistosoma japonicum. Mol Cell Proteomics (2009) 8:1236–51. doi: 10.1074/mcp.m800538-mcp200
26. Clauser KR, Baker P, Burlingame AL. Role of Accurate Mass Measurement (± 10 Ppm) in Protein Identification Strategies Employing MS or MS/MS and Database Searching. Anal Chem (1999) 71:2871–82. doi: 10.1021/ac9810516
27. Perkins DN, Pappin DJC, Creasy DM, Cottrell JS. Probability-Based Protein Identification by Searching Sequence Databases Using Mass Spectrometry Data. Electrophoresis (1999) 20:3551–67. doi: 10.1002/(SICI)1522-2683(19991201)20:18<3551::AID-ELPS3551>3.0.CO;2-2
28. Groth D, Lehrach H, Hennig S. GOblet: A Platform for Gene Ontology Annotation of Anonymous Sequence Data. Nucleic Acids Res (2004) 32:W313–7. doi: 10.1093/nar/gkh406
29. Stirewalt MA. Skin Penetration Mechanisms of Helminths. New York: New York & London: Academic Press Inc. (1996).
30. Al-Sherbiny M, Osmana A, Barakat R, El Morshedy H, Bergquist R, Olds R. In Vitro Cellular and Humoral Responses to Schistosoma mansoni Vaccine Candidate Antigens. Acta Trop (2003) 88:117–30. doi: 10.1016/s0001-706x(03)00195-5
31. Smyth D, Mcmanus DP, Smout MJ, Laha T, Zhang W, Loukas A. Isolation of cDNAs Encoding Secreted and Transmembrane Proteins From Schistosoma mansoni by a Signal Sequence Trap Method. Infect Immun (2003) 71:2548–54. doi: 10.1128/IAI.71.5.2548
32. van Balkom BWM, van Gestel RA, Brouwers JFHM, Krijgsveld J, Tielens AGM, Heck AJR, et al. Mass Spectrometric Analysis of the Schistosoma mansoni Tegumental Sub-Proteome. J Proteome Res (2005) 289:958–66. doi: 10.1074/jbc.M113.531558
33. DeMarco R, Verjovski-Almeida S. Schistosomes-Proteomics Studies for Potential Novel Vaccines and Drug Targets. Drug Discovery Today (2009) 14:472–8. doi: 10.1016/j.drudis.2009.01.011
34. Verjovski-Almeida S, DeMarco R. Current Developments on Schistosoma Proteomics. Acta Trop (2008) 108:183–5. doi: 10.1016/j.actatropica.2008.04.017
35. Harrop R, Coulson PS, Wilson RA. Characterization, Cloning and Immunogenicity of Antigens Released by Transforming Cercariae of Schistosoma mansoni. Parasitology (1999) 118:583–94. doi: 10.1017/s003118209900640x
36. Curwen RS, Ashton PD, Johnston DA, Wilson RA. The Schistosoma mansoni Soluble Proteome: A Comparison Across Four Life-Cycle Stages. Mol Biochem Parasitol (2004) 138:57–66. doi: 10.1016/j.molbiopara.2004.06.016
37. Dvořák J, Mashiyama ST, Braschi S, Sajid M, Knudsen GM, Hansell E, et al. Differential Use of Protease Families for Invasion by Schistosome Cercariae. Biochimie (2008) 90:345–58. doi: 10.1016/j.biochi.2007.08.013
38. Hansell E, Braschi S, Medzihradszky KF, Sajid M, Debnath M, Ingram J, et al. Proteomic Analysis of Skin Invasion by Blood Fluke Larvae. PloS Negl Trop Dis (2008) 2:e262. doi: 10.1371/journal.pntd.0000262
39. Cass CL, Johnson JR, Califf LL, Xu T, Hernandez HJ, Stadecker MJ, et al. Proteomic Analysis of Schistosoma mansoni Egg Secretions. Mol Biochem Parasitol (2007) 155:84–93. doi: 10.1016/j.molbiopara.2007.06.002
40. Braschi S, Wilson RA. Proteins Exposed at the Adult Schistosome Surface Revealed by Biotinylation. Mol Cell Proteomics (2006) 5:347–56. doi: 10.1074/mcp.m500287-mcp200
41. Ferreira MS, de Oliveira DN, de Oliveira RN, Allegretti SM, Vercesic AE, Catharino RR. Mass Spectrometry Imaging: A New Vision in Differentiating Schistosoma mansoni Strains. J Mass Spectrom (2014) 49:86–92. doi: 10.1002/jms.3308
42. Ludolf F, Patrocíınio PR, Corrêa-Oliveira R, Gazzinelli A, Falcone FH, Teixeira-Ferreira A, et al. Serological Screening of the Schistosoma mansoni Adult Wormproteome. PloS Negl Trop Dis (2014) 8:e2745. doi: 10.1371/journal.pntd.0002745
43. O’Farrell PH. High Resolution Two-Dimensional Electrophoresis of Proteins, J. Biol Chem (1975) 250:4007–21. doi: 10.1016/S0021-9258(19)41496-8
44. Bensimon A, Heck AJR, Aebersold R. Mass Spectrometry – Based Proteomics and Network Biology. Annu Rev Biochem (2012) 81:379–405. doi: 10.1146/annurev-biochem-072909-100424
45. Pinto-Almeida A, Mendes T, Armada A, Belo S, Carrilho E, Viveiros M, et al. The Role of Efflux Pumps in Schistosoma mansoni Praziquantel Resistant Phenotype. PloS One (2015) 10:1–20. doi: 10.1371/journal.pone.0140147
46. Katz N, Coelho PMZ. Clinical Therapy of Schistosomiasis Mansoni: The Brazilian Contribution. Acta Trop (2008) 108:72–8. doi: 10.1016/j.actatropica.2008.05.006
47. Lewis F. Schistosomiasis. Coligan JE, Kruisbeek AM, Margulies DH, Shevach EM, W. Strober, Coico R, editors. Hoboken, New Jersey: Curr. Protoc. Immunol., Wiley Inte (1998) p. 19.1.1–19.1.28.
48. Thermo Ficher Scientific. TRIzol Reagent, Experimental Protocol for DNA Isolation. Thermo Ficher Scientific Invitrogen User Guide (2016). Available at: https://assets.thermofisher.com/TFS-Assets/LSG/manuals/trizol_reagent.pdf.
49. Babu GJ, Wheeler D, Alzate O, Periasamy M. Solubilization of Membrane Proteins for Two-Dimensional Gel Electrophoresis: Identification of Sarcoplasmic Reticulum Membrane Proteins. Anal Biochem (2004) 325:121–5. doi: 10.1016/j.ab.2003.10.024
50. Marion M. Bradford, A Rapid and Sensitive Method for the Quantitation of Microgram Quantities of Protein Utilizing the Principle of Protein-Dye Binding. Anal Biochem (1976) 72:248–54. doi: 10.1016/0003-2697(76)90527-3
51. Cheng G-F, Lin J-J, Feng X-G, Fu Z-Q, Jin Y-M, Yuan C-X, et al. Proteomic Analysis of Differentially Expressed Proteins Between the Male and Female Worm of Schistosoma japonicum After Pairing. Proteomics (2005) 5:511–21. doi: 10.1002/pmic.200400953
52. Shevchenko A, Tomas H, Havlis J, Olsen JV, Mann M. In-Gel Digestion for Mass Spectrometric Characterization of Proteins and Proteomes. Nat Protoc (2006) 1:2856–60. doi: 10.1038/nprot.2006.468
53. Millipore Corporation. Sample Preparation of Oligonucleotides Prior to MALDI-TOF MS Using ZipTip C18 and ZipTip µ-C18 Pipette Tips. Millipore Technical Note (2010).
54. Robertson C, Cortens JP, Beavis RC. Open Source System for Analyzing, Validating, and Storing Protein Identification Data. J Proteome Res (2004) 3:1234–42. doi: 10.1021/pr049882h
55. Huntley RP, Sawford T, Mutowo-Meullenet P, Shypitsyna A, Bonilla C, Martin MJ, et al. The GOA Database: Gene Ontology Annotation Updates for 2015. Nucleic Acids Res (2015) 43:D1057–63. doi: 10.1093/nar/gku1113
56. Raudvere U, Kolberg L, Kuzmin I, Arak T, Adler P, Peterson H, et al. g:Profiler: A Web Server for Functional Enrichment Analysis and Conversions of Gene Lists (2019 Update). Nucleic Acids Res (2019) 47:W191–8. doi: 10.1093/nar/gkz369
57. Raynal B, Lenormand P, Baron B, Hoos S, England P. Quality Assessment and Optimization of Purified Protein Samples: Why and How? Microb Cell Fact (2014) 13:180. doi: 10.1186/s12934-014-0180-6
58. Kabatereine NB, Vennervald JB, Ouma JH, Kemijumbi J, Butterworth AE, Dunne DW, et al. Adult Resistance to Schistosomiasis Mansoni: Age-Dependence of Reinfection Remains Constant in Communities With Diverse Exposure Patterns. Parasitology (2002) 118:101–5. doi: 10.1017/s0031182098003576
59. Corrêa-Oliveira R, Rodrigues Caldas I, Gazzinelli G. Natural Versus Drug-Induced Resistance in Schistosoma mansoni Infection. Parasitol Today (2000) 16:397–9. doi: 10.1016/S0169-4758(00)01740-3
60. Black CL, Mwinzi PNM, Muok EMO, Abudho B, Fitzsimmons CM, Dunne DW, et al. Influence of Exposure History on the Immunology and Development of Resistance to Human Schistosomiasis Mansoni. PloS Negl Trop Dis (2010) 4:e637. doi: 10.1371/journal.pntd.0000637
61. Stelma EF, Tallas I, Sow S, Kongs A, Niang M, Polman K, et al. Efficacy and Side Effects of Praziquantel in an Epidemic Focus of Schistosoma mansoni. Am J Trop Med Hyg (1995) 53:167–70. doi: 10.4269/ajtmh.1995.53.167
62. Ismail M, Metwally A, Farghaly A, Bruce J, Tao L-F, Bennett JL. Characterization of Isolates of Schistosoma mansoni From Egyptian Villagers That Tolerate High Doses of Praziquantel. Am J Trop Med Hyg (1996) 55:214–8. doi: 10.4269/ajtmh.1996.55.214
63. Fallon PG, Doenhoff AJ. Drug-Resistant Schistosomiasis: Resistance to Praziquantel and Oxamniquine Induced in Schistosoma mansoni in Mice is Drug Specific. Am J Trop Med Hyg (1994) 51:83–8. doi: 10.4269/ajtmh.1994.51.83
64. Ismail M, Botros S, Metwally A, William S, Farghally A, Tao L-F, et al. Resistance to Praziquantel: Direct Evidence From Schistosoma mansoni Isolated From Egyptian Villagers. Am J Trop Med Hyg (1999) 60:932–5. doi: 10.4269/ajtmh.1999.60.932
65. Mutapi F, Burchmore R, Mduluza T, Foucher A, Harcus Y, Nicoll G, et al. Praziquantel Treatment of Individuals Exposed to Schistosoma haematobium Enhances Serological Recognition of Defined Parasite Antigens. J Infect Dis (2005) 192:1108–18. doi: 10.1086/432553
66. Braschi S, Curwen RS, Ashton PD, Verjovski-Almeida S, Wilson A. The Tegument Surface Membranes of the Human Blood Parasite Schistosoma mansoni: A Proteomic Analysis After Differential Extraction. Proteomics (2006) 6:1471–82. doi: 10.1002/pmic.200500368
67. Guillou F, Roger E, Moné Y, Rognon A, Grunau C, Théron A, et al. Excretory-Secretory Proteome of Larval Schistosoma mansoni and Echinostoma caproni, Two Parasites of Biomphalaria glabrata. Mol Biochem Parasitol (2007) 155:45–56. doi: 10.1016/j.molbiopara.2007.05.009
68. Pérez-Sánchez R, Valero ML, Ramajo-Hernández A, Siles-Lucas M, Ramajo-Martín V, Oleaga A. A Proteomic Approach to the Identification of Tegumental Proteins of Male and Female Schistosoma bovis Worms. Mol Biochem Parasitol (2008) 161:112–23. doi: 10.1016/j.molbiopara.2008.06.011
69. Zhong Z, Zhoud H, Li X, Luo Q, Songa X, Wang W, et al. Serological Proteome-Oriented Screening and Application of Antigens for the Diagnosis of Schistosomiasis Japonica. Acta Trop (2010) 116:1–8. doi: 10.1016/j.actatropica.2010.04.014
70. Boukli NM, Delgado B, Ricaurte M, Espino AM. Fasciola hepatica and Schistosoma mansoni: Identification of Common Proteins by Comparative Proteomic Analysis. J Parasitol (2011) 97:852–61. doi: 10.1645/GE-2495.1
71. Pearce EJ. Progress Towards a Vaccine for Schistosomiasis. Acta Trop (2003) 86:309–13. doi: 10.1016/S0001-706X(03)00062-7
72. Reynolds SR, Dahl CE, Harm DA. T and B Epitope Determination and Analysis of Multiple Antigenic Peptides for the Schistosoma mansoni Experimental Vaccine Triose-Phosphate Isomerase. J Immunol (1994) 152:193–200.
73. Piacenza L, Acosta D, Basmadjian I, Dalton JP, Carmona C. Vaccination Against Infection With Fasciola hepatica in Sheep With Cathepsin L Proteinases and With Leucine Aminopeptidase Induce High Levels of Protection. Infect Immun (1999) 67:1954–61. doi: 10.1016/s1383-5769(98)80739-3
74. Acosta D, Cancela M, Piacenza L, Roche L, Carmona C, Tort JF. Fasciola hepatica Leucine Aminopeptidase, a Promising Candidate for Vaccination Against Ruminant Fasciolosis. Mol Biochem Parasitol (2008) 158:52–64. doi: 10.1016/j.molbiopara.2007.11.011
75. Rinaldi G, Morales ME, Alrefaei YN, Cancela M, Dalton JP, Tort JF, et al. RNA Interference Targeting Leucine Aminopeptidase Blocks Hatching of Schistosoma mansoni Eggs. Mol Biochem Parasitol (2009) 167:118–26. doi: 10.1016/j.molbiopara.2009.05.002
76. Prosise GL, Luecke H. Crystal Structures of Tritrichomonas foetus Inosine Monophosphate Dehydrogenase in Complex With Substrate, Cofactor and Analogs: A Structural Basis for the Random-in Ordered-Out Kinetic Mechanism. J Mol Biol (2003) 326:517–27. doi: 10.1016/S0022-2836(02)01383-9
77. Protasio AV, Tsai IJ, Babbage A, Nichol S, Hunt M, Aslett MA, et al. A Systematically Improved High Quality Genome and Transcriptome of the Human Blood Fluke Schistosoma mansoni. PloS Negl Trop Dis (2012) 6:e1455. doi: 10.1371/journal.pntd.0001455
78. Sun L, Chen ZJ. The Novel Functions of Ubiquitination in Signaling. Curr Opin Cell Biol (2004) 16:119–26. doi: 10.1016/j.ceb.2004.02.005
79. Santos DN, Aguiar PHN, Lobo FP, Mourão MM, Tambor JHM, Valadão AF, et al. Schistosoma mansoni: Heterologous Complementation of a Yeast Null Mutant by SmRbx, a Protein Similar to a RING Box Protein Involved in Ubiquitination. Exp Parasitol (2007) 116:440–9. doi: 10.1016/j.exppara.2007.02.012
80. Guerra-Sá R, Castro-Borges W, Evangelista EA, Kettelhut IC, Rodrigues V. Schistosoma mansoni: Functional Proteasomes Are Required for Development in the Vertebrate Host. Exp Parasitol (2005) 109:228–36. doi: 10.1016/j.exppara.2005.01.002
81. Yang Y, Jin Y, Liu P, Shi Y, Cao Y, Liu J, et al. RNAi Silencing of Type V Collagen in Schistosoma japonicum Affects Parasite Morphology, Spawning, and Hatching. Parasitol Res (2012) 111:1251–7. doi: 10.1007/s00436-012-2959-x
82. Wei B, Jin J-P. Troponin T Isoforms and Posttranscriptional Modifications: Evolution, Regulation and Function. Arch Biochem Biophys (2011) 505:144–54. doi: 10.1016/j.abb.2010.10.013
83. Luan S. Protein Phosphatases in Plants. Annu Rev Plant Biol (2003) 54:63–92. doi: 10.1007/978-1-4939-2648-0_2
84. Mecozzi B, Rossi A, Lazzaretti P, Kady M, Kaiser S, Valle C, et al. Molecular Cloning of Schistosoma mansoni Calcineurin Subunits and Immunolocalization to the Excretory System. Mol Biochem Parasitol (2000) 110:333–43. doi: 10.1016/s0166-6851(00)00287-5
85. Daher W, Cailliau K, Takeda K, Pierrot C, Khayath N, Dissous C, et al. Characterization of Schistosoma mansoni Sds Homologue, a Leucine-Rich Repeat Protein That Interacts With Protein Phosphatase Type 1 and Interrupts a G2/M Cell-Cycle Checkpoint. Biochem J (2006) 395:433–41. doi: 10.1042/bj20051597
86. Larsen MB, Fontana ACK, Magalhães LG, Rodriguesd V, Mortensen OV. A Catecholamine Transporter From the Human Parasite Schistosoma mansoni With Low Affinity for Psychostimulants. Mol Biochem Parasitol (2011) 177:35–41. doi: 10.1016/j.molbiopara.2011.01.006
87. Frey PA. The Leloir Pathway: A Mechanistic Imperative for Three Enzymes to Change the Stereochemical Configuration of a Single Carbon in Galactose. FASEB J (1996) 10:461–70. doi: 10.1096/fasebj.10.4.8647345
88. Bork P, Sander C, Valencia A. Convergent Evolution of Similar Enzymatic Function on Different Protein Folds: The Hexokinase, Ribokinase, and Galactokinase Families of Sugar Kinases. Protein Sci (1993) 2:31–40. doi: 10.1002/pro.5560020104
89. Tielens AGM, van den Heuvel JM, van Mazijk HJ, J.E. Wilson CB. Shoemaked, The 50-kDa Glucose 6-Phosphate-Sensitive Hexokinase of Schistosoma mansoni. J Biol Chem (1994) 269:24736–41. doi: 10.1016/S0021-9258(17)31453-9
90. Roger E, Mitta G, Moné Y, Bouchut A, Rognon A, Grunau C, et al. Molecular Determinants of Compatibility Polymorphism in the Biomphalaria glabrata/Schistosoma mansoni Model: New Candidates Identified by a Global Comparative Proteomics Approach. Mol Biochem Parasitol (2008) 157:205–16. doi: 10.1016/j.molbiopara.2007.11.003
91. Gafan C, Wilson J, Berger LC, Berger BJ. Characterization of the Ornithine Aminotransferase From Plasmodium falciparum. Mol Biochem Parasitol (2001) 118:1–10. doi: 10.1016/S0166-6851(01)00357-7
92. Haslett MR, Pink D, Walters B, Brosnan ME. Assay and Subcellular Localization of Pyrroline-5-Carboxylate Dehydrogenase in Rat Liver. Biochim Biophys Acta - Gen Subj (2004) 1675:81–6. doi: 10.1016/j.bbagen.2004.08.008
93. Wang Y, Liu X, Biederer T, Sudhof TC. A Family of RIM-Binding Proteins Regulated by Alternative Splicing: Implications for the Genesis of Synaptic Active Zones. Proc Natl Acad Sci (2002) 99:14464–9. doi: 10.1073/pnas.182532999
94. Hunt P, Afonso A, Creasey A, Culleton R, Sidhu ABS, Logan J, et al. Gene Encoding a Deubiquitinating Enzyme Is Mutated in Artesunate- and Chloroquine-Resistant Rodent Malaria Parasites. Mol Microbiol (2007) 65:27–40. doi: 10.1111/j.1365-2958.2007.05753.x
95. Dalton JP, Clough KA, Jones MK, Brindley PJ. Characterization of the Cathepsin-Like Cysteine Proteinases of Schistosoma mansoni. Infect Immun (1996) 64:1328–34. doi: 10.1128/iai.64.4.1328-1334.1996
96. Berriman M, Haas BJ, LoVerde PT, Wilson RA, Dillon GP, Cerqueira GC, et al. The Genome of the Blood Fluke Schistosoma mansoni. Nature (2009) 460:352–8. doi: 10.1038/nature08160
97. Andrade LF, Nahum LA, Avelar LGA, Silva LL, Zerlotini A, Ruiz JC, et al. Eukaryotic Protein Kinases (ePKs) of the Helminth Parasite Schistosoma mansoni. BMC Genomics (2011) 12:215. doi: 10.1186/1471-2164-12-215
98. Zhou Y, Zheng H, Chen X, Zhang L, Wang K, Guo J, et al. The Schistosoma japonicum Genome Reveals Features of Host- Parasite Interplay. Nature (2009) 460:345–51. doi: 10.1038/nature08140
99. Young ND, Jex AR, Li B, Liu S, Yang L, Xiong Z, et al. Whole-Genome Sequence of Schistosoma haematobium. Nat Genet (2012) 44:221–5. doi: 10.1038/ng.1065
100. Ressurreição M, De Saram P, Kirk RS, Rollinson D, Emery AM, Page NM, et al. And Extracellular Signal-Regulated Kinase Regulate Movement, Attachment, Pairing and Egg Release in Schistosoma mansoni. PloS Negl Trop Dis (2014) 8:e2924. doi: 10.1371/journal.pntd.0002924
Keywords: praziquantel resistance, Schistosoma mansoni, proteomics, 2D-electrophoresis, mass spectrometry, liquid chromatography
Citation: Pinto-Almeida A, Mendes TMF, Ferreira P, Abecasis AB, Belo S, Anibal FF, Allegretti SM, Galinaro CA, Carrilho E and Afonso A (2021) A Comparative Proteomic Analysis of Praziquantel-Susceptible and Praziquantel-Resistant Schistosoma mansoni Reveals Distinct Response Between Male and Female Animals. Front. Trop. Dis 2:664642. doi: 10.3389/fitd.2021.664642
Received: 05 February 2021; Accepted: 28 June 2021;
Published: 21 July 2021.
Edited by:
Deirdre Hollingsworth, University of Oxford, United KingdomReviewed by:
Thomas Crellen, Big Data Institute (BDI), United KingdomStephen R. Doyle, Wellcome Sanger Institute (WT), United Kingdom
Samar Nagah El-Beshbishi, Mansoura University, Egypt
Copyright © 2021 Pinto-Almeida, Mendes, Ferreira, Abecasis, Belo, Anibal, Allegretti, Galinaro, Carrilho and Afonso. This is an open-access article distributed under the terms of the Creative Commons Attribution License (CC BY). The use, distribution or reproduction in other forums is permitted, provided the original author(s) and the copyright owner(s) are credited and that the original publication in this journal is cited, in accordance with accepted academic practice. No use, distribution or reproduction is permitted which does not comply with these terms.
*Correspondence: Emanuel Carrilho, ZW1hbnVlbEBpcXNjLnVzcC5icg==; Ana Afonso, YW5hYWZvbnNvNzRAaG90bWFpbC5jb20=