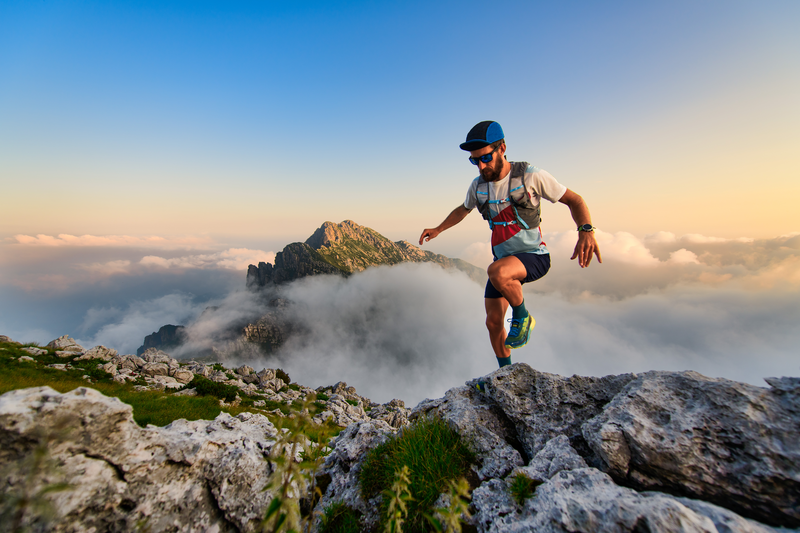
94% of researchers rate our articles as excellent or good
Learn more about the work of our research integrity team to safeguard the quality of each article we publish.
Find out more
REVIEW article
Front. Toxicol. , 05 July 2021
Sec. Developmental and Reproductive Toxicology
Volume 3 - 2021 | https://doi.org/10.3389/ftox.2021.663372
This article is part of the Research Topic Getting to the Heart of Developmental Toxicities View all 5 articles
Developmental origin of health and disease postulates that the footprints of early life exposure are followed as an endowment of risk for adult diseases. Epidemiological and experimental evidence suggest that an adverse fetal environment can affect the health of offspring throughout their lifetime. Exposure to endocrine disrupting chemicals (EDCs) during fetal development can affect the hormone system homeostasis, resulting in a broad spectrum of adverse health outcomes. In the present review, we have described the effect of prenatal EDCs exposure on cardio-metabolic-renal health, using the available epidemiological and experimental evidence. We also discuss the potential mechanisms of their action, which include epigenetic changes, hormonal imprinting, loss of energy homeostasis, and metabolic perturbations. The effect of prenatal EDCs exposure on cardio-metabolic-renal health, which is a complex condition of an altered biological landscape, can be further examined in the case of other environmental stressors with a similar mode of action.
Environmental toxicants comprise a wide range of chemical agents released through natural or anthropogenic sources. They contaminate the abiotic components of the ecosystem and affect the health of the biotic components (Gore et al., 2015; Trasande et al., 2016). Endocrine disrupting chemicals (EDCs) include phenols, phthalates, parabens, flame retardants, heavy metals, pesticides, perfluorinated chemicals, UV filter components, triclosan, and organochlorines. Among these, of particular concern are polychlorinated biphenyls (PCBs), polybrominated biphenyls (PBBs), dioxins, bisphenols, dichlorodiphenyltrichloroethane (DDT), vinclozolin, diethylstilbestrol (DES), and heavy metals, such as cadmium, mercury, arsenic, lead, manganese, and zinc. On a daily basis, people could be exposed to EDCs through packaged foods, plastics, cosmetics, and pharmaceuticals in multiple personal and occupational settings (Tchounwou et al., 2012; Gore et al., 2015; Marcoccia et al., 2017). Cumulative exposure to mixtures of EDCs can lead to adverse effects on the health of the exposed individuals (Crews et al., 2003). Multiple studies, including the studies of the National Health and Nutrition Examination Survey (NHANES), have shown that about 75–97% of US and Asian adults have detectable levels of phthalates and phenols [bisphenol A (BPA) and polyfluoroalkyl chemicals] in their urine (Silva et al., 2004; Calafat et al., 2007, 2008; Vandenberg et al., 2010; Zhang et al., 2011; Husøy et al., 2019). Epidemiological and experimental studies have also linked adult exposure to EDCs with abnormal male and female reproductive health, diabetes, obesity, cardiovascular and metabolic disorders, thyroid function, and hormone sensitive cancers (Howard and Lee, 2012; Bodin et al., 2015; Heindel et al., 2015, 2017). Children are also vulnerable to EDCs (Calafat et al., 2017; Hendryx and Luo, 2018), making EDC exposure a major health concern for all age groups.
While adult exposure to EDCs has been proved to promote adverse health effects, the developing fetus could have greater susceptibility due to its higher rate of growth and cellular differentiation (Barouki et al., 2012; Grandjean et al., 2015). Adverse health effects can be highly pronounced in the developing fetus at concentrations of EDCs much lower than the permissible limits (Welshons et al., 2003). Multiple epidemiological studies provide evidence that exposure to EDCs in pregnant women is nearly universal (Woodruff et al., 2011; Arbuckle et al., 2015; Lee et al., 2017; Rosofsky et al., 2017; Philips et al., 2018). Gestational exposure to EDCs may occur by way of daily care products, such as cosmetics, the use of electronic devices, and consumption of the animal, plant, or processed foods (Caserta et al., 2011; Rouillon et al., 2017).
Apart from gestation, childhood and adolescence are also highly vulnerable periods. The kidney and liver of infants are immature and have relatively poor glomerular filtration and capacity to detoxify drugs and chemicals (Seely, 2017). A growing body of evidence suggests that in utero exposure to EDCs, such as BPA, phthalates, polyfluoroalkyl chemicals, and heavy metals, is associated with preterm birth and fetal growth restrictions (Wolff et al., 2008; Govarts et al., 2012; Bach et al., 2015; Veiga-Lopez et al., 2015; Birks et al., 2016; Lenters et al., 2016; Lauritzen et al., 2017). These two conditions are known risk factors for the early onset of renal, cardiovascular, and metabolic dysfunction (Woodruff et al., 2011; Barker, 2012; Mierzynski et al., 2016; Martin et al., 2017).
In the present review, the effects of prenatal, perinatal, and early-life exposure to EDCs on the cardio-metabolic-renal health have been described based on epidemiological and experimental evidence available. The common and unifying mechanisms, which may be involved across multiple EDCs, are also discussed.
Metabolic syndrome can be defined as the state of metabolic perturbation, which includes at least three of the following five symptoms: elevated waist circumference, elevated triglycerides, reduced high-density lipoprotein cholesterol (HDL-C), elevated fasting glucose, and hypertension (Alberti et al., 2009). Metabolic syndrome (MetS) was reported to be associated with an increased risk of chronic diseases, including type 2 diabetes (T2D), non-alcoholic fatty liver disease (NAFLD), various cancer forms, and cardiovascular diseases (Mendrick et al., 2018). As the endocrine system homeostasis is crucial for normal development and metabolism, the endocrine disrupting chemicals can also be referred to as “metabolism disrupting chemicals.” The link between developmental exposure to endocrine disruptors and the onset of metabolic perturbations was pioneered by Grun and Blumberg who described the obesogenic action of organotins (Grün and Blumberg, 2006).
The epidemiological studies on maternal exposure to EDCs and the risk of metabolic disorders have been summarized in Table 1. The experimental studies on this are summarized in Table 2. Prenatal exposure to BPA causes hyperleptinemia, high blood pressure, and enhanced weight gain during early childhood (Ashley-Martin et al., 2014; Bae et al., 2017; Guo et al., 2020). Metabolic disruption after maternal BPA exposure has been reported in rodent models where it promoted hyperlipidemia, altered glucose homeostasis, impaired energy expenditure, and increased adiposity (Miyawaki et al., 2007; Alonso-Magdalena et al., 2010; MacKay et al., 2013; Li et al., 2014; García-Arévalo et al., 2016; Bansal et al., 2017; Desai et al., 2018b; Manukyan et al., 2019; Diamante et al., 2020).
Table 2. Major experimental studies describing maternal exposure to EDCs and risk of metabolic disorders in offspring.
Pthalates are plasticizers generally used in many personal care and medical use products. Maternal exposure to phthalates was reported to cause metabolic disruptions, elevate body mass index (BMI), and leptin levels in the exposed population (Ashley-Martin et al., 2014; Buckley et al., 2016a,b; Maresca et al., 2016). Experimental studies have reported multiple mechanisms for phthalates-associated metabolic disruption, including damage and changes to hepatic metabolism (Zhang et al., 2018; Wen et al., 2020), enhanced adipose differentiation (Hunt et al., 2017), alteration in genes associated with β-cell developmental (Rajesh and Balasubramanian, 2015), and dysbiosis of gut microbiota (Fan et al., 2020). Altered phosphorylation of endothelial nitric oxide synthase and induction of angiotensin type 1 receptor could elevate blood pressure (Lee et al., 2016) and further contribute to metabolic disruption by phthalates.
Diethylstilbestrol is a non-steroidal chemical with estrogenic activity. It has been reported to act as an obesogen and cause metabolic disruption through enhanced weight gain in prenatally exposed human females (Hatch et al., 2015). However, in rodent models, gestational low dose (0.1 ppm) exposure to diethylstilbestrol (DES) resulted in reduced litter size and decreased body weight of the offspring (Cagen et al., 1999a,b).
Organochlorines, a different class of endocrine disruptors, have also been reported to cause metabolic deregulation after maternal exposure (Tables 1, 2). Human cohort studies on maternal exposure to dichlorodiphenyl-dichloroethylene (DDE) and PCBs showed an increase in the growth rate and childhood obesity in offspring (Tang-Péronard et al., 2014; Valvi et al., 2014). High maternal urinary DDE levels were also associated with increased body weight in infants (Karmaus et al., 2009; Valvi et al., 2014; Iszatt et al., 2015). Hyperinsulinemia has also been reported in some 5 year-old females exposed to persistent organic pollutants (Tang-Péronard et al., 2015). Experimental studies on prenatal exposure to DDE have also reported impaired cold tolerance, high body fat content, and the carryover of an obese phenotype up to F3 generation (Skinner et al., 2013; La Merrill et al., 2014). Female offspring, which were perinatally exposed to 2,3,7,8-Tetrachlorodibenzodioxin (TCDD), had increased fat while exposed males showed decreased fat content. In the same study, exposure to PCB was associated with increased glucagon levels in females, while males showed hyperglycemia (Van Esterik et al., 2015).
Many heavy metals, including cadmium, lead, mercury, and arsenic, are also reported to act through endocrine disruption at relatively low doses. In human birth cohort studies, cadmium and lead exposure during early lifetime resulted in juvenile obesity and hyperleptinemia (Ashley-Martin et al., 2015; Green et al., 2018). However, lower blood cholesterol levels were observed in male children after maternal lead (lead > 5 μg/dL) exposure (Liu et al., 2019, 2020). Maternal cadmium levels were also associated with juvenile obesity in the offspring (Green et al., 2018). Gestational exposure to cadmium has been reported to promote glucose intolerance, pancreatic damage, liver steatosis, and adiposity in the animal offspring up to F2 generation through endocrine disruption of glucocorticoid (Castillo et al., 2012) and retinoic acid signaling (Jackson et al., 2020). Arsenic exposure to both the mother and the offspring was associated with metabolic disorders, including T2D in the Strong Heart Family Study (Tinkelman et al., 2020). Multiple studies have reported metabolic disruption after gestational arsenic exposure, including increased glucose intolerance, adiposity, and insulin resistance (Rodriguez et al., 2016; Huang et al., 2018). High-fat diet has been found to exaggerate liver steatosis after maternal arsenic exposure in offspring (Ditzel et al., 2016). Cumulatively, all the above studies provide evidence that prenatal and early-life exposure to EDCs can cause metabolic dysfunction.
Chronic kidney disease is a growing health problem among children and adults. The incidence and the prevalence of chronic kidney disease (CKD) among children have been steadily increasing since the 1980s (Baum, 2010; Harambat et al., 2012; Becherucci et al., 2016). A number of traditional risk factors associated with CKD in children include hypertension, obesity, diabetes, and aberrant divalent mineral metabolism (Wong et al., 2006; Staples et al., 2010; Harambat et al., 2012; Warady et al., 2015). There is growing evidence that links exposure to EDCs with early progression to end-stage renal disease (ESRD) (Kataria et al., 2015). However, early-life exposure to EDCs and their association with chronic kidney disease have not been extensively studied. Some of the studies on gestational and early-life exposure to EDCs and their effect on the kidney are summarized in Table 3. Early-life exposure to EDCs was associated with elevated levels of kidney toxicity markers such as albumin-to-creatinine ratio (ACR), estimated glomerular filtration rate (eGFR), and urinary protein-to-creatinine ratio (UPCR) in some human population studies (Li et al., 2012; Trasande et al., 2013a, 2014; Malits et al., 2018).
Detectable bisphenol A levels were reported in a study on children correlated with increased levels of ACR (Trasande et al., 2013a). Furthermore, in some animal studies, gestational exposure to BPA was associated with glomerular abnormalities, including changes in the glomerular number and density (Nuñez et al., 2018). Early-life exposure to BPA and phthalates is also associated with increased tubular injury and oxidative stress, which can affect renal function (Jacobson et al., 2020). In a study on children, exposure to high molecular weight phthalates was associated with higher ACR (Trasande et al., 2014). Exposure to di-(2-ethylhexyl) phthalate (DEHP) tainted food was associated with micro-albuminuria in children (Tsai et al., 2016; Wu et al., 2018a).
Growing evidence suggests a strong association between dioxin exposure and renal dysfunction in adults (Huang et al., 2016), although there is less information on prenatal dioxin exposure and renal abnormalities (Table 3). Perinatal exposure to TCDD can affect renal morphology (Aragon et al., 2008b) and promote hydronephrosis (Aragon et al., 2008a). Similarly, exposure to flame retardants, such as organophosphate esters (OPEs) and tetrabromobisphenol A (TBBPA), was associated with CKD in adult epidemiological studies (Kang et al., 2019). In an in utero study, exposure to TBBPA was associated with renal tubule atrophy and cyst in the kidney (Fukuda et al., 2004; Tada et al., 2006).
The heavy metals, which might modulate the endocrine system, are cadmium, arsenic, mercury, lead, manganese, and zinc. However, not all heavy metals are associated with kidney diseases. While there are several adult studies on exposure to heavy metals and nephrotoxicity, the studies on gestational and perinatal exposure are sparse. A cross-sectional study on preschool girls (age range: 4.4–5.4 years) showed that cadmium exposure during childhood was inversely correlated with estimated glomerular filtration rate (eGFR), which can adversely affect kidney function (Skroder et al., 2015). In an animal study, increased levels of albumin, osteopontin, vascular endothelial growth factor, and tissue inhibitor of metalloproteinases-1 were observed in the amniotic fluid of cadmium-exposed mothers (Jacobo-Estrada et al., 2016). In the same study, histopathological assessment of the kidney of the fetus showed tubular damage and precipitations in the renal pelvis. In a related study, gestational and lactational exposure to cadmium led to the reduced relative weight of liver and kidneys in the female offspring (Luo et al., 2015; Hamidian et al., 2020). Gestational exposure to cadmium also caused a significant decrease in the glomerular filtration rate (GFR) with a disorganized expression of tight-junction proteins, such as claudin-2 and claudin-5 in rat offspring (Jacquillet et al., 2007). In another study, rat offspring, which were prenatally exposed to cadmium chloride (CdCl2), had increased β2-microglobulin (β2M) levels, suggesting some kidney damage (Saillenfait et al., 1991).
The effect of early exposure to arsenic on the progression and development of kidney diseases has been assessed in few human studies (Smith et al., 2012; Hawkesworth et al., 2013; Zheng et al., 2014; Weidemann et al., 2015). Exposure to arsenic has also been linked with increased mortality in young adults due to multiorgan cancers and chronic renal diseases (Smith et al., 2012). The nephrotoxic effects following prenatal arsenic exposure could also be due to dysregulated autophagy (Tian et al., 2019b) and increased oxidative stress and mitochondrial damage (Tian et al., 2019a,b).
Lead-induced nephropathy in young adults was first reported in children in Queensland, Australia (Nye, 1929). Chronic poisoning with lead (blood lead levels > 60 μg/dL) has been associated with nephropathy in children and adults (Ekong et al., 2006), and was characterized by tubulointerstitial fibrosis, tubular atrophy, glomerular sclerosis, and reduced eGFR (Morgan et al., 1966; Loghman-Adham, 1997). Childhood lead poisoning can also promote hypertension (Moel et al., 1985; Hu, 1991; Fadrowski et al., 2010), prolong partial Fanconi syndrome (Loghman-Adham, 1998), and lead to abnormal renal function (Moel and Sachs, 1992; Filler et al., 2012; Fadrowski et al., 2013). In a prospective Yugoslavian birth-cohort study, high blood pressure and proteinuria were observed in offspring born to mothers living near lead-contaminated environment (Factor-Litvak et al., 1999). This was also associated with increased levels of lead in the blood. Hyperfiltration, which is often linked with albuminuria and a transient increase in GFR, was also associated with early-life lead exposure and may be the cause of kidney injury during adulthood (Khalil-Manesh et al., 1992; Weaver et al., 2003; Ekong et al., 2006; Helal et al., 2012). Early-life exposure to lead was positively associated with serum cystatin C levels (Staessen et al., 2001). However, in another study, early-life exposure to lead was negatively associated with serum creatinine and cystatin C (de Burbure et al., 2006). In another population study on children exposed to lead, lead levels in blood were positively associated with multiple urinary renal injury biomarkers, including retinol-binding protein (Bernard et al., 1995), β2M and Clara cell protein (Fels et al., 1998), and N-acetyl-beta-D-glucosaminidase (NAG) (Verberk et al., 1996).
Exposure to all forms of mercury is nephrotoxic (Zalups and Lash, 1994). In the kidneys, the pars recta segment of the proximal tubule is highly susceptible to mercury. Unfortunately, there are very limited studies on the effect of gestational mercury exposure on the kidneys of the offspring. The accumulation of high mercury levels in the kidney of offspring, following exposure during the gestation period, has been reported (Drasch et al., 1994). Animal studies showed transient renal dysfunction in mothers, as well as the offspring with a significant increase in urinary β2M and albumin levels after mercury exposure (Bernard et al., 1992). An unpublished study from our lab has also associated prenatal methyl mercury exposure with decreased glomerulus numbers in the offspring.
Cardiovascular diseases (CVD) cause an estimated 17.9 million deaths annually (Wang et al., 2016). To date, the majority of epidemiological and animal studies connecting environmental stressors and CVD have focused on a narrow group of EDCs.
In an in vivo study, exposure to ioxynil (IOX) and DES during the embryonic stage led to disrupted cardiovascular development (Li et al., 2019). The study showed increased heartbeat frequency and reduced ventricle volume and an aorta diameter, following IOX and DES exposure during the embryonic stage.
In a similar study, zebrafish embryos, which were exposed to BPA, had impaired cardiogenesis with an altered cardiac phenotype, upregulation of hand 2, estrogen receptor (esr2b), histone acetyltransferase (kat6a), and histone acetylation (Lombó et al., 2019). Furthermore, the increased rate of heart failures in the progeny was observed when male zebrafish was exposed to BPA during spermatogenesis (Lombó et al., 2015). This continued till the F2 generation. The study also showed a significant decrease in five key genes involved in cardiac development in the embryos of the F1 generation (myh6, cmlc2, atp2a2b, sox2, and insrb). A study on apes, which were orally administered BPA during the gestation period, suggested its impact on the cardiovascular fitness of the developing fetus (Chapalamadugu et al., 2014). A significant decrease in the expression of myosin heavy chain, cardiac isoform alpha (Myh6), was observed in the left ventricle. Similarly, overexpression of ′A Disintegrin and Metalloprotease 12′, long isoform (Adam12-l), was observed in both the ventricles and the right atrium of the heart of the exposed fetus. A study on sheep showed that prenatal BPA exposure followed by postnatal overfeeding leads to a significant increase in interventricular septal thickness and affects the morphological and functional parameters of the heart when the exposed animals become obese later in life (Mohan Kumar et al., 2017).
Animal studies on perinatal BPA exposure have reported enhanced male and reduced female sex specific differences in velocity of the circumferential shortening and ascending aorta velocity time integral. Elevated diastolic blood pressure was observed in all the perinatally exposed female offspring (Cagampang et al., 2012; Patel et al., 2013). Several calcium homeostasis proteins (sarcoendoplasmic reticulum ATPase 2a (SERCA2a), sodium calcium exchanger-1, phospholamban (PLB), phospho-PLB, and calsequestrin 2, which are involved in contraction and relaxation of cardiac muscles were altered (Cagampang et al., 2012; Patel et al., 2013). Fibrosis was also observed in the heart of the fetus prenatally exposed to BPA. This was associated with a significant change in miR-17-5p,−208-3p, and−210-3p expression in the fetal heart (Rasdi et al., 2020). In a study, using PXR-humanized apolipoprotein E-deficient (huPXR•ApoE−/−) mouse model, perinatal exposure to BPA worsened atherosclerosis in adult male huPXR•ApoE−/− offspring (Sui et al., 2018). However, no significant atherosclerotic changes were observed in female offspring.
In a cross-sectional study, early-life exposure to BPA was associated with elevated diastolic blood pressure (Khalil et al., 2014). In another cross-sectional study on children and adolescents, dietary phthalate (DEHP) exposure was associated with elevated systolic blood pressure (Trasande et al., 2013b). Perinatal exposure to hexachlorobenzene (HCB) was associated with an elevated systolic blood pressure, and early-life exposure to DDE was associated with an increase in diastolic blood pressure (Vafeiadi et al., 2015). A combined cohort study demonstrated that women prenatally exposed to DES are at higher risk of coronary artery disease (CAD), myocardial infarction (MI), high cholesterol levels, hypertension, and elevated blood pressure (Troisi et al., 2013, 2018). All these factors are major signs of metabolic disorders.
In a cross-sectional study on children, exposure to phthalates, such as monobenzyl, monocarboxyoctyl, and monocarboxynonyl during the gestation period, was associated with decreased levels of 8-isoprostane at 9 years of age (Tran et al., 2017). 8-isoprostane is a known marker for oxidative stress, which can promote detrimental metabolic changes later in life. Whereas, at 14 years of age, a positive association (increase) was observed between 8-isoprostane and two other metabolites of high molecular weight phthalates [mono(2-ethylhexyl) and mono (2-ethyl-5-carboxypentyl) phthalate]. A positive association was also observed between 8-isoprostane and total cholesterol levels, as well as systolic and diastolic blood pressure (Tran et al., 2017). Exposure to DEHP during lactation altered the expression of insulin-signaling molecules in the cardiac tissue of the offspring (Mangala Priya et al., 2014). In utero exposure to DEHP can also reduce locomotor activity at postnatal day (PND) 60. At later-life stages (PND 200), both systolic and diastolic systemic arterial pressure and locomotor activity were reduced in adult rats perinatally exposed to DEHP (Martinez-Arguelles et al., 2013). Wu and coworkers observed an inverse correlation between early-life exposure to phthalate and systolic blood pressure in boys (Wu et al., 2018b). Another group of researchers identified a notable correlation between different phthalate metabolites such as mono-butyl phthalate (MBP), mono-benzyl phthalate (MBzP), and mono-2-ethylhexyl phthalate (MEHP), and elevated blood pressure in children and adolescents aged 6–18 years (Amin et al., 2018). As per the Spanish INMA-Sabadell Birth Cohort Study, high- and low-molecular weight phthalate metabolites were associated with lower systolic blood pressure in girls but not in boys (Valvi et al., 2015).
Perfluorononanoic acid (PFNA), a perfluoroalkyl substance (PFASs), was associated with elevated systolic blood pressure, low-density lipoprotein cholesterol (LDL-C), and total cholesterol (Khalil et al., 2018). Perfluorooctanoic acid (PFOA) and perfluorooctane sulfonic acid (PFOS) were associated with increased LDL-C. PFOA exposure was also positively correlated with total cholesterol (Khalil et al., 2018). Elevated blood pressure was observed in offspring born to rats exposed to DEX, PFOS, atrazine, and PFNA (Rogers et al., 2014).
Nearly five decades ago, a set of autopsy case reports established an association between perinatal arsenic exposure and cardiovascular conditions, such as myocardial infarction, vascular lesions, and thickening of the arteries in young children (Rosenberg, 1973, 1974). Children with these severe cardiovascular issues had resided in regions of Chile, who were highly contaminated with arsenic (average levels of 870 μg/L) from 1958 to 1970. Young adults (30–49 years) born during this period were at three times higher risk of mortality due to myocardial infarction as compared with the rest of the Chile (Yuan et al., 2007). Several epidemiological studies have also associated in utero and early-life arsenic exposure with an increased risk of childhood cardiovascular disease (Table 4). Increased childhood (5–18 years old) mortality due to CVD was observed in arsenic-exposed children from Bangladesh (Rahman et al., 2013). The risk was comparatively higher in girls and in adolescents. According to MINIMat cohort study in Bangladesh, higher in utero arsenic exposure was associated with increased blood pressure in children at 18 months of age (Hawkesworth et al., 2013). Elevation in blood pressure from an early age may have detrimental effects later in life, particularly in a genetically susceptible population. In a cross-sectional study of children (3–14 years) in Zimapan, Mexico, a positive correlation was established between total urinary arsenic and carotid intima-media thickness (cIMT), a subclinical indicator of CVD (Osorio-Yanez et al., 2013). The study also showed an association of total urinary arsenic with increased plasma levels of asymmetric dimethylarginine (ADMA), an endogenous inhibitor of nitric oxide production and predictive of CVD. The mothers of the participating children were reported living in the highly contaminated areas during their pregnancy, suggesting a possible contribution of prenatal arsenic exposure in the onset of observed effects. Apolipoprotein E deficient (ApoE−/−) mice are highly vulnerable to atherosclerosis. The male offspring of pregnant ApoE−/− mice, which were exposed to arsenic, showed accelerated atherosclerotic plaque and loss of endothelial cell function and vascular tone at 10 and 16 weeks of age (Srivastava et al., 2007). The follow-up study showed a more profound effect, following early postnatal exposure to arsenic (Srivastava et al., 2009).
EDCs might cause similar modes of action, transport, and storage within tissues and activate or antagonize nuclear hormone receptors (Casals-Casas and Desvergne, 2011; Heindel et al., 2017). During pregnancy, exposure to EDCs has been associated with an abnormal gestational endocrine milieu, including altered levels of sex hormones (Sathyanarayana et al., 2014; Johns et al., 2015). Multiple mechanisms have been proposed for the action of EDCs, including epigenetic modulations, altered inflammatory and oxidative stress responses, hormonal imprinting and fundamental changes in energy storage, and glucose homeostasis pathways. The common mechanisms across multiple classes of EDCs are summarized in Figure 1.
Figure 1. Multiple effects of exposure to endocrine disrupting chemicals (EDCs). EDCs might act through multiple mechanisms to alter cell fate and function. Early-life exposure to EDCs might cause epigenetic dysregulation, pro-inflammatory changes, and changes in energy homeostasis and glucose regulation. These could adversely affect the cardiometabolic renal health of offspring.
Abnormal maternal environment may alter the developmental trajectory of the fetus via epigenetic modulations (Walker, 2016). Prenatal exposure to phthalates [particularly to DEHP, MEHP, benz-butyl phthalate (BBP), DBP, and MBP] has been shown to modulate genes through their effect on DNA methylation, histone modifications (acetylation, methylation, phosphorylation, ubiquitination, sumoylation, and ADP ribosylation), non-coding RNAs and micro RNAs (miRNAs) (Martinez-Arguelles and Papadopoulos, 2016; Solomon et al., 2017; Dutta et al., 2020). Paternal factors such as sperm DNA changes can also affect fetal development (Day et al., 2016). Prenatal cadmium exposure was associated with hypermethylation in the promoter of glucocorticoid receptor (GR) gene, resulting in increased expression of hepatic GR, leading to dysregulated hepatic metabolism (Castillo et al., 2012).
Several studies link early-life epigenetic changes with dysregulation of metabolic parameters. Early-life exposure to BPA can promote hypermethylation of imprinted genes like IGF2, resulting in impairment of β-cell function in the pancreas of offspring (Mao et al., 2017) and pregnant mothers (Bateman et al., 2017). Maternal exposure to BPA might inhibit the expression of pancreatic and duodenal homeobox-1(Pdx1) gene through deacetylation, demethylation of histone 3 lysine 4 (H3K4), and methylation of histone 3 lysine 9 (H3K9), leading to impaired β-cell development in the offspring (Chang et al., 2016). Maternal BPA exposure also inhibits hepatic glucokinase (Gck) expression through hypermethylation (Maet al. 2013; Liet al., 2014). BPA might exert hyperlipidemic effects through epigenetic modifications of hepatic genes Fasn, Nrf2, and SREBP-1C, which are involved in lipid metabolism (Strakovsky et al., 2015; Shimpi et al., 2017). Similarly, prenatal exposure to DES caused developmental programming of obesity through hypermethylation of the homeobox gene HOXA10 and other region-specific alterations (Bromer et al., 2009). Prenatal BPA exposure could also promote long-term weight gain through its effect on imprinted genes, such as MEST (Junge et al., 2018) and IGF2R (Choi et al., 2020).
Mono-(2-ethylhexyl)phthalate exposure in RAW 264.7 cells was found to cause inflammation through sirtuins (Park et al., 2019a). Phthalates might also induce miRNAs modifications to alter the cholesterol efflux in RAW 264.7 cells (Park et al., 2019b). Maternal exposure to phthalates (DEHP) was also associated with altered DNA methylation in liver (Wen et al., 2020) and hypermethylation of genes (Pdx1, Pax4/6, and HNF-4α) involved in pancreatic β-cell development (Rajesh and Balasubramanian, 2015). All these could promote diabetes and obesity phenotype, which was closely linked with metabolic syndrome.
Non-epigenetic effects on glucose metabolism and adipogenesis have also been observed. Adult exposure to cadmium was found to inhibit key glucose metabolizing enzymes involved in glycolysis, a pentose pathway, gluconeogenesis, and a glycogenesis process through its binding to cysteine-SH residues (Viselina and Luk'Yanova, 2000; Ramírez-Bajo et al., 2014). Cadmium also affects the synthesis, transportation, and metabolism of lipids (Lucia et al., 2010; Yang et al., 2013). Arsenic might also interfere with glucose metabolism through binding with the thiol-containing enzymes (Kannan and Flora, 2004). It can also affect the binding of substrate to insulin receptors and alter adipogenic differentiation and glucose homeostasis (Garciafigueroa et al., 2013; Castriota et al., 2020). Arsenic might also interfere and replace the phosphate group in enzymes involved in glycolysis and oxidative phosphorylation through a process known as “arsenolysis” (Kannan and Flora, 2004).
The promotion of persistent inflammation and oxidative stress is another common theme across multiple EDCs. Cadmium is associated with oxidative stress in the pancreas (Lei et al., 2005). Arsenic can also promote oxidative stress through both mitochondria-dependent (Naranmandura et al., 2011) and independent mechanisms (Kannan and Flora, 2004; Shi et al., 2004; Naranmandura et al., 2011). Upon exposure to arsenic, there could be the production of nitric oxide and superoxide anions, which can subsequently be converted to reactive species to cause oxidative stress and cellular damage through mitochondria-independent mechanisms. Many human and animal studies have demonstrated that EDCs might modulate the immune system and alter the inflammatory cytokine milieu in both the mother and the fetus (Ferguson et al., 2011; Dietert, 2012; Song et al., 2017). Cohort studies on pregnant mothers have demonstrated that detectable levels of paraben and phenols in the urine were associated with abnormal inflammatory cytokine levels in the blood (Watkins et al., 2015; Zota et al., 2018). Aberrant maternal proinflammatory state, in turn, is associated with adverse birth outcomes, including complete loss, preterm labor, preeclampsia, and fetal growth restriction (Cotechini and Graham, 2015; Amaral et al., 2017; Boyle et al., 2017; Catalano and Shankar, 2017).
Several signaling intermediates have been associated with early-life exposure to EDCs and inflammation. Maternal cadmium exposure might activate NF-κB to stimulate the expression of inflammatory cytokines (Ronco et al., 2011). Chronic inflammation after BPA exposure was associated with activation of JNK and NF-kB-signaling pathways that was followed by upregulation of inflammatory cytokines (Savastano et al., 2015; Liao et al., 2016). Exogenous exposure to BPA induced mitochondrial damage in INS-1 cells (Lin et al., 2013; Shirani et al., 2019), along with induction of other proapoptotic proteins (Gong et al., 2017; Wang et al., 2017; Kaur et al., 2018) and cytochrome C release (Hwang et al., 2013).
Many EDCs might modulate physiological processes and hormonal action in a non-monotonic manner (Casals-Casas and Desvergne, 2011; Vandenberg et al., 2012; MacKay and Abizaid, 2018) through their effect on hormone receptors. BPA can promote metabolic disorders through its endocrine-disrupting effect on multiple nuclear receptors, including the estrogen (Ohlstein et al., 2014; Acconcia et al., 2015), glucocorticoid (GR) (Zhang et al., 2019), and aryl hydrocarbon receptor (AhR) (Nishizawa et al., 2005). BPA might act as anestrogen receptor alpha (ERα) agonist through activation of ERα ligand bonding domain (LBD), although it cannot activate the LBD of estrogen receptor beta (ERβ). Thus, it acts as an ERβ antagonist through inhibition of the downstream p38/MAPK pathway (Ascenzi et al., 2006; Bolli et al., 2010). BPA can also enhance adipogenesis through GR activation and translocation in 3T3L1 cells (Sargis et al., 2010; Atlas et al., 2014). Some in silico studies also demonstrate its activity on GR (Prasanth et al., 2010; Zhang et al., 2017). Although BPA cannot directly activate the nuclear receptor PPARγ (Ohlstein et al., 2014), its analogs are known to act through activation of PPARγ-RXR complex (Riu et al., 2011). In utero exposure to BPA has been also shown to increase the expression of AhR at both RNA and protein levels (Nishizawa et al., 2005). Phthalates are also known to act on hormone-signaling agents to enhance cell proliferation (Jin et al., 2008; Chen et al., 2016). They can either activate the downstream estrogen signaling (Lee et al., 2014) or stimulate AhR and downstream signaling (Hsieh et al., 2012). Adult exposure to phthalates is also reported to cause estrogen receptor stress and autophagy in zebrafish liver through activation of the IRE-XBP1 pathway (Zhang et al., 2021).
Heavy metals, including arsenic and cadmium, can also alter hormone signaling by interacting with ERα (Stoica et al., 2000) and GR (Simons et al., 1990). Mercury is also known to inhibit the endogenous hormone binding of GR and mineralocorticoid receptor (MR) (Galigniana and Piwien-Pilipuk, 1999; Brkljačić et al., 2004). Arsenic can target the DNA-binding domain (DBD) of GR to inhibit gene expression in rat EDR3 hepatoma cells at higher doses, while the effects are stimulatory at lower doses (Bodwell et al., 2004). Perinatal exposure to arsenic can interfere with MAPK signaling through the reduction in Ras and Raf expression, which are downstream of GR (Martinez-Finley et al., 2011; Caldwell et al., 2015). Chronic exposure to arsenic is known to reduce the circulatory estradiol levels as well as the expression of estrogen receptor and the estrogen-responsive genes (VEGF, cyclin D1, and CDK4) in the uterus (Chatterjee and Chatterji, 2010). Cadmium is also reported to act as a metalloestrogen (Aquino et al., 2012). In vitro studies have shown that cadmium could affect the estrogen receptor either through binding to LBD (Stoica et al., 2000) or replacing the Zn2+ in DNA binding domain (DBD) of ERα (Low et al., 2002; Nesatyy et al., 2005). In vivo studies on the zebrafish brain have also shown that the estrogen receptor antagonistic activity of cadmium can be ameliorated by Zn treatment (Chouchene et al., 2016).
The clustering of metabolic and cardiovascular risk factors, which was previously known as “syndrome X,” is now referred to as “cardiometabolic syndrome” (CMS). Prenatal exposure to EDCs could cause adverse metabolic alterations, which are closely linked with CKD and CVD. The possible unifying mechanism could involve fundamental changes in the hormonal and epigenetic imprints caused due to EDCs exposure during fetal development. This could permanently alter the baseline inflammatory state and affect adipocyte development in the offspring. All these factors could increase the overall risk for cardiovascular disease, diabetes, and CKD in offspring. Developmental exposure to EDCs may thus be imprinting a risk phenotype for overall cardio-metabolic-renal health (CMR-health) in later life, which needs to be studied further.
RS and KK conducted the comprehensive literature review. RS, KK, and VSh wrote the manuscript. RT, HK, and VSh helped in formulation of tables and figure and revised the article for important intellectual content. VSr and VSh designed the concept of the manuscript. All authors read and approved the final manuscript.
The authors declare that the research was conducted in the absence of any commercial or financial relationships that could be construed as a potential conflict of interest.
Acconcia, F., Pallottini, V., and Marino, M. (2015). Molecular mechanisms of action of BPA. Dose Resp. 13:1559325815610582. doi: 10.1177/1559325815610582
Alberti, K., Eckel, R. H., Grundy, S. M., Zimmet, P. Z., Cleeman, J. I., Donato, K. A., and Smith, S. C. Jr. (2009). Harmonizing the metabolic syndrome: a joint interim statement of the International Diabetes Federation Task Force on Epidemiology and Prevention; National Heart, Lung, and blood Institute; American Heart Association; World Heart Federation; International Atherosclerosis Society; and International Association for the study of obesity. Circulation 120, 1640–1645. doi: 10.1161/CIRCULATIONAHA.109.192644
Alonso-Magdalena, P., Vieira, E., Soriano, S., Menes, L., Burks, D., Quesada, I., et al. (2010). Bisphenol A exposure during pregnancy disrupts glucose homeostasis in mothers and adult male offspring. Environ. Health Perspect. 118, 1243–1250. doi: 10.1289/ehp.1001993
Amaral, L. M., Wallace, K., Owens, M., and LaMarca, B. (2017). Pathophysiology and current clinical management of preeclampsia. Curr. Hypertens. Rep. 19, 1–6. doi: 10.1007/s11906-017-0757-7
Amin, M. M., Ebrahimpour, K., Parastar, S., Shoshtari-Yeganeh, B., Hashemi, M., Mansourian, M., et al. (2018). Association of urinary concentrations of phthalate metabolites with cardiometabolic risk factors and obesity in children and adolescents. Chemosphere 211, 547–556. doi: 10.1016/j.chemosphere.2018.07.172
Angle, B. M., Do, R. P., Ponzi, D., Stahlhut, R. W., Drury, B. E., Nagel, S. C., et al. (2013). Metabolic disruption in male mice due to fetal exposure to low but not high doses of bisphenol A (BPA): evidence for effects on body weight, food intake, adipocytes, leptin, adiponectin, insulin and glucose regulation. Reprod. Toxicol. 42, 256–268. doi: 10.1016/j.reprotox.2013.07.017
Aquino, N. B., Sevigny, M. B., Sabangan, J., and Louie, M. C. (2012). The role of cadmium and nickel in estrogen receptor signaling and breast cancer: metalloestrogens or not? J. Environ. Sci. Health Part C 30, 189–224. doi: 10.1080/10590501.2012.705159
Aragon, A. C., Goens, M. B., Carbett, E., and Walker, M. K. (2008a). Perinatal 2. 3, 7, 8-tetrachlorodibenzo-p-dioxin exposure sensitizes offspring to angiotensin II-induced hypertension. Cardiovasc. Toxicol. 8:145. doi: 10.1007/s12012-008-9023-1
Aragon, A. C., Kopf, P. G., Campen, M. J., Huwe, J. K., and Walker, M. K. (2008b). In utero and lactational 2. 3, 7, 8-tetrachlorodibenzo-p-dioxin exposure: effects on fetal and adult cardiac gene expression and adult cardiac and renal morphology. Toxicol. Sci. 101, 321–330 doi: 10.1093/toxsci/kfm272
Arbuckle, T. E., Marro, L., Davis, K., Fisher, M., Ayotte, P., Bélanger, P., et al. (2015). Exposure to free and conjugated forms of bisphenol A and triclosan among pregnant women in the MIREC cohort. Environ. Health Perspect. 123, 277–284. doi: 10.1289/ehp.1408187
Ascenzi, P., Bocedi, A., and Marino, M. (2006). Structure–function relationship of estrogen receptor α and β: impact on human health. Mol. Aspects Med. 27, 299–402. doi: 10.1016/j.mam.2006.07.001
Ashley-Martin, J., Dodds, L., Arbuckle, T. E., Ettinger, A. S., Shapiro, G. D., Fisher, M., et al. (2014). A birth cohort study to investigate the association between prenatal phthalate and bisphenol A exposures and fetal markers of metabolic dysfunction. Environ. Health 13:84. doi: 10.1186/1476-069X-13-84
Ashley-Martin, J., Dodds, L., Arbuckle, T. E., Ettinger, A. S., Shapiro, G. D., Fisher, M., et al. (2015). Maternal blood metal levels and fetal markers of metabolic function. Environ. Res. 136, 27–34. doi: 10.1016/j.envres.2014.10.024
Atlas, E., Pope, L., Wade, M. G., Kawata, A., Boudreau, A., and Boucher, J. G. (2014). Bisphenol A increases aP2 expression in 3T3L1 by enhancing the transcriptional activity of nuclear receptors at the promoter. Adipocyte 3, 170–179. doi: 10.4161/adip.28436
Bach, C. C., Bech, B. H., Brix, N., Nohr, E. A., Bonde, J. P. E., and Henriksen, T. B. (2015). Perfluoroalkyl and polyfluoroalkyl substances and human fetal growth: a systematic review. Crit. Rev. Toxicol. 45, 53–67. doi: 10.3109/10408444.2014.952400
Bae, S., Lim, Y.-H., Lee, Y. A., Shin, C. H., Oh, S.-Y., and Hong, Y.-C. (2017). Maternal urinary bisphenol A concentration during midterm pregnancy and children's blood pressure at age 4. Hypertension 69, 367–374. doi: 10.1161/HYPERTENSIONAHA.116.08281
Bansal, A., Rashid, C., Xin, F., Li, C., Polyak, E., Duemler, A., et al. (2017). Sex-and dose-specific effects of maternal bisphenol A exposure on pancreatic islets of first-and second-generation adult mice offspring. Environ. Health Perspect. 125:097022. doi: 10.1289/EHP1674
Barker, D. J. (2012). Developmental origins of chronic disease. Public Health 126, 185–189. doi: 10.1016/j.puhe.2011.11.014
Barouki, R., Gluckman, P. D., Grandjean, P., Hanson, M., and Heindel, J. J. (2012). Developmental origins of non-communicable disease: implications for research and public health. Environ. Health 11, 1–9. doi: 10.1186/1476-069X-11-42
Bateman, M. E., Strong, A. L., McLachlan, J. A., Burow, M. E., and Bunnell, B. A. (2017). The effects of endocrine disruptors on adipogenesis and osteogenesis in mesenchymal stem cells: a review. Front. Endocrinol. 7:171. doi: 10.3389/fendo.2016.00171
Baum, M. (2010). Overview of chronic kidney disease in children. Curr. Opin. Pediatr. 22:158. doi: 10.1097/MOP.0b013e32833695cb
Becherucci, F., Roperto, R. M., Materassi, M., and Romagnani, P. (2016). Chronic kidney disease in children. Clin. Kidney J. 9, 583–591. doi: 10.1093/ckj/sfw047
Bernard, A. M., Collette, C., and Lauwerys, R. (1992). Renal effects of in utero exposure to mercuric chloride in rats. Arch. Toxicol. 66, 508–513. doi: 10.1007/BF01970677
Bernard, A. M., Vyskocil, A., Roels, H., Kriz, J., Kodl, M., and Lauwerys, R. (1995). Renal effects in children living in the vicinity of a lead smelter. Environ. Res. 68, 91–95. doi: 10.1006/enrs.1995.1012
Birks, L., Casas, M., Garcia, A. M., Alexander, J., Barros, H., Bergström, A., et al. (2016). Occupational exposure to endocrine-disrupting chemicals and birth weight and length of gestation: a European meta-analysis. Environ. Health Perspect. 124, 1785–1793. doi: 10.1289/EHP208
Bodin, J., Stene, L. C., and Nygaard, U. C. (2015). Can exposure to environmental chemicals increase the risk of diabetes type 1 development?. Biomed. Res. Int. 2015:208947. doi: 10.1155/2015/208947
Bodwell, J. E., Kingsley, L. A., and Hamilton, J. W. (2004). Arsenic at very low concentrations alters glucocorticoid receptor (GR)-mediated gene activation but not GR-mediated gene repression: complex Dose– Response effects are closely correlated with levels of activated GR and require a functional GR DNA binding domain. Chem. Res. Toxicol. 17, 1064–1076. doi: 10.1021/tx0499113
Bolli, A., Bulzomi, P., Galluzzo, P., Acconcia, F., and Marino, M. (2010). Bisphenol A impairs estradiol-induced protective effects against DLD-1 colon cancer cell growth. IUBMB Life 62, 684–687. doi: 10.1002/iub.370
Boyle, A. K., Rinaldi, S. F., Norman, J. E., and Stock, S. J. (2017). Preterm birth: inflammation, fetal injury and treatment strategies. J. Reprod. Immunol. 119, 62–66. doi: 10.1016/j.jri.2016.11.008
Brkljačić, J., Milutinović, D. V., Dundjerski, J., and Matić, G. (2004). Mercury inhibits rat liver and kidney glucocorticoid receptor hormone binding activity. Cell Biol. Toxicol. 20, 171–182. doi: 10.1023/B:CBTO.0000029467.21231.12
Bromer, J. G., Wu, J., Zhou, Y., and Taylor, H. S. (2009). Hypermethylation of homeobox A10 by in utero diethylstilbestrol exposure: an epigenetic mechanism for altered developmental programming. Endocrinology 150, 3376–3382. doi: 10.1210/en.2009-0071
Buckley, J. P., Engel, S. M., Mendez, M. A., Richardson, D. B., Daniels, J. L., Calafat, A. M., et al. (2016a). Prenatal phthalate exposures and childhood fat mass in a New York City cohort. Environ. Health Perspect. 124, 507–513. doi: 10.1289/ehp.1509788
Buckley, J. P., Herring, A. H., Wolff, M. S., Calafat, A. M., and Engel, S. M. (2016b). Prenatal exposure to environmental phenols and childhood fat mass in the Mount Sinai Children's Environmental Health Study. Environ. Int. 91, 350–356. doi: 10.1016/j.envint.2016.03.019
Cagampang, F. R., Torrens, C., Anthony, F. W., and Hanson, M. A. (2012). Developmental exposure to bisphenol A leads to cardiometabolic dysfunction in adult mouse offspring. J. Dev. Orig. Health Dis. 3, 287–292. doi: 10.1017/S2040174412000153
Cagen, S., Waechter, J Jr, Dimond, S., Breslin, W., Butala, J., Jekat, F., and Harris, L. (1999a). Normal reproductive organ development in CF-1 mice following prenatal exposure to bisphenol A. Toxicol. Sci. 50, 36–44. doi: 10.1093/toxsci/50.1.36
Cagen, S., Waechter, J. Jr., Dimond, S., Breslin, W., Butala, J., Jekat, F., and Harris, L. (1999b). Normal reproductive organ development in Wistar rats exposed to bisphenol A in the drinking water. Regul. Toxicol. Pharmacol. 30, 130–139. doi: 10.1006/rtph.1999.1340
Calafat, A. M., Wong, L. Y., Kuklenyik, Z., Reidy, J. A., and Needham, L. L. (2007). Polyfluoroalkyl chemicals in the US population: data from the National Health and Nutrition Examination Survey (NHANES) 2003–2004 and comparisons with NHANES 1999–2000. Environ. Health Perspect. 115, 1596–1602. doi: 10.1289/ehp.10598
Calafat, A. M., Ye, X., Valentin-Blasini, L., Li, Z., Mortensen, M. E., and Wong, L. Y. (2017). Co-exposure to non-persistent organic chemicals among American pre-school aged children: a pilot study. Int. J. Hyg. Environ. Health 220, 55–63. doi: 10.1016/j.ijheh.2016.10.008
Calafat, A. M., Ye, X., Wong, L. Y., Reidy, J. A., and Needham, L. L. (2008). Exposure of the US population to bisphenol A and 4-tertiary-octylphenol: 2003–2004. Environ. Health Perspect. 116, 39–44. doi: 10.1289/ehp.10753
Caldwell, K. E., Labrecque, M. T., Solomon, B. R., Ali, A., and Allan, A. M. (2015). Prenatal arsenic exposure alters the programming of the glucocorticoid signaling system during embryonic development. Neurotoxicol. Teratol. 47, 66–79. doi: 10.1016/j.ntt.2014.11.006
Camsari, C., Folger, J. K., Rajput, S. K., McGee, D., Latham, K. E., and Smith, G. W. (2019). Transgenerational effects of periconception heavy metal administration on adipose weight and glucose homeostasis in mice at maturity. Toxicol. Sci. 168, 610–619. doi: 10.1093/toxsci/kfz008
Casals-Casas, C., and Desvergne, B. (2011). Endocrine disruptors: from endocrine to metabolic disruption. Annu. Rev. Physiol. 73, 135–162. doi: 10.1146/annurev-physiol-012110-142200
Caserta, D., Mantovani, A., Marci, R., Fazi, A., Ciardo, F., La Rocca, C., et al. (2011). Environment and women's reproductive health. Hum. Reprod. Update 17, 418–433. doi: 10.1093/humupd/dmq061
Castillo, P., Ibáñez, F., Guajardo, A., Llanos, M. N., and Ronco, A. M. (2012). Impact of cadmium exposure during pregnancy on hepatic glucocorticoid receptor methylation and expression in rat fetus. PLoS ONE 7:e44139. doi: 10.1371/journal.pone.0044139
Castriota, F., Rieswijk, L., Dahlberg, S., La Merrill, M. A., Steinmaus, C., Smith, M. T., et al. (2020). A state-of-the-science review of arsenic's effects on glucose homeostasis in experimental models. Environ. Health Perspect. 128:016001. doi: 10.1289/EHP4517
Catalano, P. M., and Shankar, K. (2017). Obesity and pregnancy: mechanisms of short term and long term adverse consequences for mother and child. BMJ 356:j1. doi: 10.1136/bmj.j1
Chang, H., Wang, D., Xia, W., Pan, X., Huo, W., Xu, S., et al. (2016). Epigenetic disruption and glucose homeostasis changes following low-dose maternal bisphenol A exposure. Toxicol. Res. 5, 1400–1409. doi: 10.1039/C6TX00047A
Chapalamadugu, K. C., Vandevoort, C. A., Settles, M. L., Robison, B. D., and Murdoch, G. K. (2014). Maternal bisphenol a exposure impacts the fetal heart transcriptome. PLoS ONE 9:e89096. doi: 10.1371/journal.pone.0089096
Chatterjee, A., and Chatterji, U. (2010). Arsenic abrogates the estrogen-signaling pathway in the rat uterus. Reprod. Biol. Endocrinol. 8, 1–11. doi: 10.1186/1477-7827-8-80
Chen, F.-P., Chien, M.-H., and Chern, I. Y.-Y. (2016). Impact of low concentrations of phthalates on the effects of 17β-estradiol in MCF-7 breast cancer cells. Taiwanese J. Obstetr. Gynecol. 55, 826–834. doi: 10.1016/j.tjog.2015.11.003
Choi, Y.-J., Lee, Y. A., Hong, Y.-C., Cho, J., Lee, K.-S., Shin, C. H., et al. (2020). Effect of prenatal bisphenol A exposure on early childhood body mass index through epigenetic influence on the insulin-like growth factor 2 receptor (IGF2R) gene. Environ. Int. 143:105929. doi: 10.1016/j.envint.2020.105929
Chouchene, L., Pellegrini, E., Gueguen, M. M., Hinfray, N., Brion, F., Piccini, B., et al. (2016). Inhibitory effect of cadmium on estrogen signaling in zebrafish brain and protection by zinc. J. Appl. Toxicol. 36, 863–871. doi: 10.1002/jat.3285
Cotechini, T., and Graham, C. H. (2015). Aberrant maternal inflammation as a cause of pregnancy complications: a potential therapeutic target? Placenta 36, 960–966. doi: 10.1016/j.placenta.2015.05.016
Crews, D., Putz, O., Thomas, P., Hayes, T., and Howdeshell, K. (2003). Animal models for the study of the effects of mixtures, low doses, and the embryonic environment on the action of endocrine disrupting chemicals. Pure Appl. Chem. 75, 2305–2320. doi: 10.1351/pac200375112305
Day, J., Savani, S., Krempley, B. D., Nguyen, M., and Kitlinska, J. B. (2016). Influence of paternal preconception exposures on their offspring: through epigenetics to phenotype. Am. J. Stem Cells 5, 11–18.
de Burbure, C., Buchet, J. P., Leroyer, A., Nisse, C., Haguenoer, J. M., Mutti, A., et al. (2006). Renal and neurologic effects of cadmium, lead, mercury, and arsenic in children: evidence of early effects and multiple interactions at environmental exposure levels. Environ. Health Perspect. 114, 584–590. doi: 10.1289/ehp.8202
Desai, M., Ferrini, M. G., Han, G., Jellyman, J. K., and Ross, M. G. (2018a). In vivo maternal and in vitro BPA exposure effects on hypothalamic neurogenesis and appetite regulators. Environ. Res. 164, 45–52. doi: 10.1016/j.envres.2018.02.011
Desai, M., Ferrini, M. G., Jellyman, J. K., Han, G., and Ross, M. G. (2018b). In vivo and in vitro bisphenol A exposure effects on adiposity. J. Dev. Orig. Health Dis. 9, 678–687. doi: 10.1017/S2040174418000600
Diamante, G., Cely, I., Zamora, Z., Ding, J., Blencowe, M., Lang, J., et al. (2020). Systems toxicogenomics of prenatal low-dose BPA exposure on liver metabolic pathways, gut microbiota, and metabolic health in mice. Environ. Int. 146:106260. doi: 10.1016/j.envint.2020.106260
Dietert, R. R. (2012). Misregulated inflammation as an outcome of early-life exposure to endocrine-disrupting chemicals. Rev. Environ. Health 27, 117–131. doi: 10.1515/reveh-2012-0020
Ditzel, E. J., Nguyen, T., Parker, P., and Camenisch, T. D. (2016). Effects of arsenite exposure during fetal development on energy metabolism and susceptibility to diet-induced fatty liver disease in male mice. Environ. Health Perspect. 124, 201–209. doi: 10.1289/ehp.1409501
Drasch, G., Schupp, I., Höfl, H., Reinke, R., and Roider, G. (1994). Mercury burden of human fetal and infant tissues. Eur. J. Pediatr. 153, 607–610. doi: 10.1007/BF02190671
Dutta, S., Haggerty, D. K., Rappolee, D. A., and Ruden, D. M. (2020). Phthalate exposure and long-term epigenomic consequences: a review. Front. Genet. 11:405. doi: 10.3389/fgene.2020.00405
Ekong, E. B., Jaar, B. G., and Weaver, V. M. (2006). Lead-related nephrotoxicity: a review of the epidemiologic evidence. Kidney Int. 70, 2074–2084. doi: 10.1038/sj.ki.5001809
Factor-Litvak, P., Wasserman, G., Kline, J. K., and Graziano, J. (1999). The Yugoslavia prospective study of environmental lead exposure. Environ. Health Perspect. 107, 9–15. doi: 10.1289/ehp.991079
Fadrowski, J. J., Abraham, A. G., Navas-Acien, A., Guallar, E., Weaver, V. M., and Furth, S. L. (2013). Blood lead level and measured glomerular filtration rate in children with chronic kidney disease. Environ. Health Perspect. 121, 965–970. doi: 10.1289/ehp.1205164
Fadrowski, J. J., Navas-Acien, A., Tellez-Plaza, M., Guallar, E., Weaver, V. M., and Furth, S. L. (2010). Blood lead level and kidney function in US adolescents: The Third National Health and Nutrition Examination Survey. Arch. Intern. Med. 170, 75–82. doi: 10.1001/archinternmed.2009.417
Fan, Y., Qin, Y., Chen, M., Li, X., Wang, R., Huang, Z., et al. (2020). Prenatal low-dose DEHP exposure induces metabolic adaptation and obesity: role of hepatic thiamine metabolism. J. Hazard. Mater. 385:121534. doi: 10.1016/j.jhazmat.2019.121534
Fels, L. M., Wünsch, M., Baranowski, J., Norska-Borówka, I., Price, R. G., Taylor, S. A., et al. (1998). Adverse effects of chronic low level lead exposure on kidney function–a risk group study in children. Nephrol. Dial. Transpl. 13, 2248–2256. doi: 10.1093/ndt/13.9.2248
Ferguson, K. K., Loch-Caruso, R., and Meeker, J. D. (2011). Urinary phthalate metabolites in relation to biomarkers of inflammation and oxidative stress: NHANES 1999–2006. Environ. Res. 111, 718–726. doi: 10.1016/j.envres.2011.02.002
Filler, G., Roach, E., Yasin, A., Sharma, A. P., Blake, P. G., and Yang, L. (2012). High prevalence of elevated lead levels in pediatric dialysis patients. Pediatr. Nephrol. 27, 1551–1556. doi: 10.1007/s00467-012-2150-8
Fukuda, N., Ito, Y., Yamaguchi, M., Mitumori, K., Koizumi, M., Hasegawa, R., et al. (2004). Unexpected nephrotoxicity induced by tetrabromobisphenol A in newborn rats. Toxicol. Lett. 150, 145–155. doi: 10.1016/j.toxlet.2004.01.001
Galigniana, M. D., and Piwien-Pilipuk, G. (1999). Comparative inhibition by hard and soft metal ions of steroid-binding capacity of renal mineralocorticoid receptor cross-linked to the 90-kDa heat-shock protein heterocomplex. Biochem. J. 341, 585–592. doi: 10.1042/bj3410585
García-Arévalo, M., Alonso-Magdalena, P., Servitja, J.-M., Boronat-Belda, T., Merino, B., Villar-Pazos, S., et al. (2016). Maternal exposure to bisphenol-A during pregnancy increases pancreatic β-cell growth during early life in male mice offspring. Endocrinology 157, 4158–4171. doi: 10.1210/en.2016-1390
Garciafigueroa, D. Y., Klei, L. R., Ambrosio, F., and Barchowsky, A. (2013). Arsenic-stimulated lipolysis and adipose remodeling is mediated by G-protein-coupled receptors. Toxicol. Sci. 134, 335–344. doi: 10.1093/toxsci/kft108
Gong, X., Xie, H., Li, X., Wu, J., and Lin, Y. (2017). Bisphenol A induced apoptosis and transcriptome differences of spermatogonial stem cells in vitro. Acta Biochim. Biophys. Sin. 49, 780–791. doi: 10.1093/abbs/gmx075
Gore, A. C., Chappell, V. A., Fenton, S. E., Flaws, J. A., Nadal, A., Prins, G. S., et al. (2015). EDC-2: the Endocrine Society's second scientific statement on endocrine-disrupting chemicals. Endocr. Rev. 36, E1–E150. doi: 10.1210/er.2015-1093
Govarts, E., Nieuwenhuijsen, M., Schoeters, G., Ballester, F., Bloemen, K., De Boer, M., et al. (2012). Birth weight and prenatal exposure to polychlorinated biphenyls (PCBs) and dichlorodiphenyldichloroethylene (DDE): a meta-analysis within 12 European Birth Cohorts. Environ. Health Perspect. 120, 162–170. doi: 10.1289/ehp.1103767
Grandjean, P., Barouki, R., Bellinger, D. C., Casteleyn, L., Chadwick, L. H., Cordier, S., et al. (2015). Life-long implications of developmental exposure to environmental stressors: new perspectives. Endocrinology 156, 3408–3415. doi: 10.1210/en.2015-1350
Green, A. J., Hoyo, C., Mattingly, C. J., Luo, Y., Tzeng, J.-Y., Murphy, S. K., et al. (2018). Cadmium exposure increases the risk of juvenile obesity: a human and zebrafish comparative study. Int. J. Obes. 42, 1285–1295. doi: 10.1038/s41366-018-0036-y
Grün, F., and Blumberg, B. (2006). Environmental obesogens: organotins and endocrine disruption via nuclear receptor signaling. Endocrinology 147, s50–s55. doi: 10.1210/en.2005-1129
Guo, J., Zhang, J., Wu, C., Xiao, H., Lv, S., Lu, D., et al. (2020). Urinary bisphenol A concentrations and adiposity measures at age 7 years in a prospective birth cohort. Chemosphere 251:126340. doi: 10.1016/j.chemosphere.2020.126340
Hamidian, G., Mirdar, S., Raee, P., Asghari, K., and Jarrahi, M. (2020). Silymarin protects the structure of kidney in the neonatal rats exposed to maternal cadmium toxicity: a stereological study. Vet. Res. Forum 11, 143–152. doi: 10.30466/vrf.2019.84202.2108
Harambat, J., Van Stralen, K. J., Kim, J. J., and Tizard, E. J. (2012). Epidemiology of chronic kidney disease in children. Pediatr. Nephrol. 27, 363–373. doi: 10.1007/s00467-011-1939-1
Hatch, E., Troisi, R., Palmer, J., Wise, L., Titus, L., Strohsnitter, W., et al. (2015). Prenatal diethylstilbestrol exposure and risk of obesity in adult women. J. Dev. Orig. Health Dis. 6, 201–207. doi: 10.1017/S2040174415000033
Hawkesworth, S., Wagatsuma, Y., Kippler, M., Fulford, A. J., Arifeen, S. E., Persson, L. A., et al. (2013). Early exposure to toxic metals has a limited effect on blood pressure or kidney function in later childhood, rural Bangladesh. Int. J. Epidemiol. 42:176. doi: 10.1093/ije/dys215
Heindel, J. J., Blumberg, B., Cave, M., Machtinger, R., Mantovani, A., Mendez, M. A., et al. (2017). Metabolism disrupting chemicals and metabolic disorders. Reprod. Toxicol. 68, 3–33. doi: 10.1016/j.reprotox.2016.10.001
Heindel, J. J., Vom Saal, F. S., Blumberg, B., Bovolin, P., Calamandrei, G., Ceresini, G., et al. (2015). Parma consensus statement on metabolic disruptors. Environ. Health 14, 1–7. doi: 10.1186/s12940-015-0042-7
Helal, I., Fick-Brosnahan, G. M., Reed-Gitomer, B., and Schrier, R. W. (2012). Glomerular hyperfiltration: definitions, mechanisms and clinical implications. Nat. Rev. Nephrol. 8, 293–300. doi: 10.1038/nrneph.2012.19
Hendryx, M., and Luo, J. (2018). Children's environmental chemical exposures in the USA, NHANES 2003–2012. Environ. Sci. Pollut. Res. 25, 5336–5343. doi: 10.1007/s11356-017-0874-5
Howard, S. G., and Lee, D. H. (2012). What is the role of human contamination by environmental chemicals in the development of type 1 diabetes?. J. Epidemiol. Commun. Health 66, 479–481. doi: 10.1136/jech.2011.133694
Hsieh, T. H., Tsai, C. F., Hsu, C. Y., Kuo, P. L., Lee, J. N., Chai, C. Y., et al. (2012). Phthalates induce proliferation and invasiveness of estrogen receptor-negative breast cancer through the AhR/HDAC6/c-Myc signaling pathway. FASEB J. 26, 778–787. doi: 10.1096/fj.11-191742
Hu, H. (1991). A 50-year follow-up of childhood plumbism: hypertension, renal function, and hemoglobin levels among survivors. Am. J. Dis. Child. 145, 681–687. doi: 10.1001/archpedi.1991.02160060099029
Huang, C. Y., Wu, C. L., Wu, J. S., Chang, J. W., Cheng, Y. Y., Kuo, Y. C., et al. (2016). Association between blood dioxin level and chronic kidney disease in an endemic area of exposure. PLoS ONE 11:e0150248. doi: 10.1371/journal.pone.0150248
Huang, M. C., Douillet, C., Dover, E. N., and Stýblo, M. (2018). Prenatal arsenic exposure and dietary folate and methylcobalamin supplementation alter the metabolic phenotype of C57BL/6J mice in a sex-specific manner. Arch. Toxicol. 92, 1925–1937. doi: 10.1007/s00204-018-2206-z
Hunt, B. G., Wang, Y.-L., Chen, M.-S., Wang, S.-C., and Waltz, S. E. (2017). Maternal diethylhexyl phthalate exposure affects adiposity and insulin tolerance in offspring in a PCNA-dependent manner. Environ. Res. 159, 588–594. doi: 10.1016/j.envres.2017.09.004
Husøy, T., Andreassen, M., Hjertholm, H., Carlsen, M. H., Norberg, N., Sprong, C., et al. (2019). The Norwegian biomonitoring study from the EU project EuroMix: levels of phenols and phthalates in 24-hour urine samples and exposure sources from food and personal care products. Environ. Int. 132:105103. doi: 10.1016/j.envint.2019.105103
Hwang, J. K., Min, K. H., Choi, K. H., Hwang, Y. C., Jeong, I.-K., Ahn, K. J., et al. (2013). Bisphenol A reduces differentiation and stimulates apoptosis of osteoclasts and osteoblasts. Life Sci. 93, 367–372. doi: 10.1016/j.lfs.2013.07.020
Iszatt, N., Stigum, H., Verner, M.-A., White, R. A., Govarts, E., Murinova, L. P., et al. (2015). Prenatal and postnatal exposure to persistent organic pollutants and infant growth: a pooled analysis of seven European birth cohorts. Environ. Health Perspect. 123, 730–736. doi: 10.1289/ehp.1308005
Jackson, T. W., Ryherd, G. L., Scheibly, C. M., Sasser, A. L., Guillette, T., and Belcher, S. M. (2020). Gestational Cd exposure in the CD-1 mouse induces sex-specific hepatic insulin insensitivity, obesity, and metabolic syndrome in adult female offspring. Toxicol. Sci. 178, 264–280. doi: 10.1093/toxsci/kfaa154
Jacobo-Estrada, T., Cardenas-Gonzalez, M., Santoyo-Sánchez, M., Parada-Cruz, B., Uria-Galicia, E., Arreola-Mendoza, L., et al. (2016). Evaluation of kidney injury biomarkers in rat amniotic fluid after gestational exposure to cadmium. J. Appl. Toxicol. 36, 1183–1193. doi: 10.1002/jat.3286
Jacobson, M. H., Wu, Y., Liu, M., Attina, T. M., Naidu, M., Karthikraj, R., et al. (2020). Serially assessed bisphenol A and phthalate exposure and association with kidney function in children with chronic kidney disease in the US and Canada: a longitudinal cohort study. PLoS Med. 17:e1003384. doi: 10.1371/journal.pmed.1003384
Jacquet, A., Barbeau, D., Arnaud, J., Hijazi, S., Hazane-Puch, F., Lamarche, F., et al. (2019). Impact of maternal low-level cadmium exposure on glucose and lipid metabolism of the litter at different ages after weaning. Chemosphere 219, 109–121. doi: 10.1016/j.chemosphere.2018.11.137
Jacquillet, G., Barbier, O., Rubera, I., Tauc, M., Borderie, A., Namorado, M. C., et al. (2007). Cadmium causes delayed effects on renal function in the offspring of cadmium-contaminated pregnant female rats. Am. J. Physiol. Renal Physiol. 293, F1450–F1460. doi: 10.1152/ajprenal.00223.2007
Jin, Q., Sun, Z., and Li, Y. (2008). Estrogenic activities of di-2-ethylhexyl phthalate. Front. Med. China 2, 303–308. doi: 10.1007/s11684-008-0058-2
Johns, L. E., Ferguson, K. K., Soldin, O. P., Cantonwine, D. E., Rivera-González, L. O., Del Toro, L. V. A., et al. (2015). Urinary phthalate metabolites in relation to maternal serum thyroid and sex hormone levels during pregnancy: a longitudinal analysis. Reprod. Biol. Endocrinol. 13, 1–12. doi: 10.1186/1477-7827-13-4
Junge, K. M., Leppert, B., Jahreis, S., Wissenbach, D. K., Feltens, R., Grützmann, K., et al. (2018). MEST mediates the impact of prenatal bisphenol A exposure on long-term body weight development. Clin. Epigenet. 10, 1–12. doi: 10.1186/s13148-018-0478-z
Kang, H., Lee, J., Lee, J. P., and Choi, K. (2019). Urinary metabolites of organophosphate esters (OPEs) are associated with chronic kidney disease in the general US population, NHANES 2013-2014. Environ. Int. 131:105034. doi: 10.1016/j.envint.2019.105034
Kannan, G. M., and Flora, S. J. (2004). Chronic arsenic poisoning in the rat: treatment with combined administration of succimers and an antioxidant. Ecotoxicol. Environ. Saf. 58, 37–43. doi: 10.1016/S0147-6513(03)00096-4
Karmaus, W., Osuch, J. R., Eneli, I., Mudd, L. M., Zhang, J., Mikucki, D., et al. (2009). Maternal levels of dichlorodiphenyl-dichloroethylene (DDE) may increase weight and body mass index in adult female offspring. Occup. Environ. Med. 66, 143–149. doi: 10.1136/oem.2008.041921
Kataria, A., Trasande, L., and Trachtman, H. (2015). The effects of environmental chemicals on renal function. Nat. Rev. Nephrol. 11:610. doi: 10.1038/nrneph.2015.94
Kaur, S., Saluja, M., and Bansal, M. (2018). Bisphenol A induced oxidative stress and apoptosis in mice testes: modulation by selenium. Andrologia 50:e12834. doi: 10.1111/and.12834
Khalil, N., Ebert, J. R., Honda, M., Lee, M., Nahhas, R. W., Koskela, A., et al. (2018). Perfluoroalkyl substances, bone density, and cardio-metabolic risk factors in obese 8-12 year old children: a pilot study. Environ. Res. 160, 314–321. doi: 10.1016/j.envres.2017.10.014
Khalil, N., Ebert, J. R., Wang, L., Belcher, S., Lee, M., Czerwinski, S. A., et al. (2014). Bisphenol A and cardiometabolic risk factors in obese children. Sci. Tot. Environ. 470–471, 726–732. doi: 10.1016/j.scitotenv.2013.09.088
Khalil-Manesh, F., Gonick, H. C., Cohen, A. H., Alinovi, R., Bergamaschi, E., Mutti, A., et al. (1992). Experimental model of lead nephropathy. I. Continuous high-dose lead administration. Kidney Int. 41, 1192–1203. doi: 10.1038/ki.1992.181
La Merrill, M., Karey, E., Moshier, E., Lindtner, C., La Frano, M. R., Newman, J. W., et al. (2014). Perinatal exposure of mice to the pesticide DDT impairs energy expenditure and metabolism in adult female offspring. PloS ONE. 9:e103337. doi: 10.1371/journal.pone.0103337
Lauritzen, H. B., Larose, T. L., Øien, T., Sandanger, T. M., Odland, J. Ø., Van De Bor, M., et al. (2017). Maternal serum levels of perfluoroalkyl substances and organochlorines and indices of fetal growth: a Scandinavian case–cohort study. Pediatr. Res. 81, 33–42. doi: 10.1038/pr.2016.187
Lee, H.-R., Hwang, K.-A., and Choi, K.-C. (2014). The estrogen receptor signaling pathway activated by phthalates is linked with transforming growth factor-β in the progression of LNCaP prostate cancer models. Int. J. Oncol. 45, 595–602. doi: 10.3892/ijo.2014.2460
Lee, K.-I., Chiang, C.-W., Lin, H.-C., Zhao, J.-F., Li, C.-T., Shyue, S.-K., et al. (2016). Maternal exposure to di-(2-ethylhexyl) phthalate exposure deregulates blood pressure, adiposity, cholesterol metabolism and social interaction in mouse offspring. Arch. Toxicol. 90, 1211–1224. doi: 10.1007/s00204-015-1539-0
Lee, W. C., Fisher, M., Davis, K., Arbuckle, T. E., and Sinha, S. K. (2017). Identification of chemical mixtures to which Canadian pregnant women are exposed: the MIREC study. Environ. Int. 99, 321–330. doi: 10.1016/j.envint.2016.12.015
Lei, L., Jin, T., and Zhou, Y. (2005). The toxic effects of cadmium on pancreas. Chin. J. Indust. Hyg. Occup. Dis. 23, 45–49.
Lenters, V., Portengen, L., Rignell-Hydbom, A., Jönsson, B. A., Lindh, C. H., Piersma, A. H., et al. (2016). Prenatal phthalate, perfluoroalkyl acid, and organochlorine exposures and term birth weight in three birth cohorts: multi-pollutant models based on elastic net regression. Environ. Health Perspect. 124, 365–372. doi: 10.1289/ehp.1408933
Li, G., Chang, H., Xia, W., Mao, Z., Li, Y., and Xu, S. (2014). F0 maternal BPA exposure induced glucose intolerance of F2 generation through DNA methylation change in Gck. Toxicol. Lett. 228, 192–199. doi: 10.1016/j.toxlet.2014.04.012
Li, M., Bi, Y., Qi, L., Wang, T., Xu, M., Huang, Y., et al. (2012). Exposure to bisphenol A is associated with low-grade albuminuria in Chinese adults. Kidney Int. 81, 1131–1139. doi: 10.1038/ki.2012.6
Li, Y. F., Canario, A. V. M., Power, D. M., and Campinho, M. A. (2019). Ioxynil and diethylstilbestrol disrupt vascular and heart development in zebrafish. Environ. Int. 124, 511–520. doi: 10.1016/j.envint.2019.01.009
Liao, S.-L., Tsai, M.-H., Lai, S.-H., Yao, T.-C., Hua, M.-C., Yeh, K.-W., et al. (2016). Prenatal exposure to bisphenol-a is associated with toll-like receptor–induced cytokine suppression in neonates. Pediatr. Res. 79, 438–444. doi: 10.1038/pr.2015.234
Lin, Y., Sun, X., Qiu, L., Wei, J., Huang, Q., Fang, C., et al. (2013). Exposure to bisphenol A induces dysfunction of insulin secretion and apoptosis through the damage of mitochondria in rat insulinoma (INS-1) cells. Cell Death Dis. 4:e460. doi: 10.1038/cddis.2012.206
Liu, Y., Ettinger, A. S., Téllez-Rojo, M., Sánchez, B. N., Zhang, Z., Cantoral, A., et al. (2020). Prenatal lead exposure, type 2 diabetes, and cardiometabolic risk factors in mexican children at age 10–18 years. J. Clin. Endocrinol. Metab. 105, 210–218. doi: 10.1210/clinem/dgz038
Liu, Y., Peterson, K. E., Montgomery, K., Sánchez, B. N., Zhang, Z., Afeiche, M. C., et al. (2019). Early lead exposure and childhood adiposity in Mexico City. Int. J. Hyg. Environ. Health 222, 965–970. doi: 10.1016/j.ijheh.2019.06.003
Loghman-Adham, M. (1997). Renal effects of environmental and occupational lead exposure. Environ. Health Perspect. 105, 928–938. doi: 10.1289/ehp.97105928
Loghman-Adham, M. (1998). Aminoaciduria and glycosuria following severe childhood lead poisoning. Pediatr. Nephrol. 12, 218–221. doi: 10.1007/s004670050441
Lombó, M., Fernández-Díez, C., González-Rojo, S., Navarro, C., Robles, V., and Herráez, M. P. (2015). Transgenerational inheritance of heart disorders caused by paternal bisphenol A exposure. Environ. Pollut. 206, 667–678. doi: 10.1016/j.envpol.2015.08.016
Lombó, M., González-Rojo, S., Fernández-Díez, C., and Herráez, M. P. (2019). Cardiogenesis impairment promoted by bisphenol A exposure is successfully counteracted by epigallocatechin gallate. Environ. Pollut. 246, 1008–1019. doi: 10.1016/j.envpol.2019.01.004
Low, L. Y., Hernández, H., Robinson, C. V., O'Brien, R., Grossmann, J. G., Ladbury, J. E., et al. (2002). Metal-dependent folding and stability of nuclear hormone receptor DNA-binding domains. J. Mol. Biol. 319, 87–106. doi: 10.1016/S0022-2836(02)00236-X
Lucia, M., André, J.-M., Gonzalez, P., Baudrimont, M., Bernadet, M.-D., Gontier, K., et al. (2010). Effect of dietary cadmium on lipid metabolism and storage of aquatic bird Cairina moschata. Ecotoxicology 19, 163–170. doi: 10.1007/s10646-009-0401-7
Luo, X., Li, L., Ma, M., and Li, R. (2015). Effects of low-dose cadmium exposure during gestation and lactation on development and reproduction in rats. Environ. Sci. Pollut. Res. 22, 10569–10579. doi: 10.1007/s11356-015-4249-5
MacKay, H., and Abizaid, A. (2018). A plurality of molecular targets: the receptor ecosystem for bisphenol-A (BPA). Horm. Behav. 101, 59–67. doi: 10.1016/j.yhbeh.2017.11.001
MacKay, H., Patterson, Z. R., Khazall, R., Patel, S., Tsirlin, D., and Abizaid, A. (2013). Organizational effects of perinatal exposure to bisphenol-A and diethylstilbestrol on arcuate nucleus circuitry controlling food intake and energy expenditure in male and female CD-1 mice. Endocrinology 154, 1465–1475. doi: 10.1210/en.2012-2044
Malits, J., Attina, T. M., Karthikraj, R., Kannan, K., Naidu, M., Furth, S., et al. (2018). Renal Function and exposure to Bisphenol A and phthalates in children with Chronic Kidney Disease. Environ. Res. 167, 575–582. doi: 10.1016/j.envres.2018.08.006
Mangala Priya, V., Mayilvanan, C., Akilavalli, N., Rajesh, P., and Balasubramanian, K. (2014). Lactational exposure of phthalate impairs insulin signaling in the cardiac muscle of F1 female albino rats. Cardiovasc. Toxicol. 14, 10–20. doi: 10.1007/s12012-013-9233-z
Manukyan, L., Dunder, L., Lind, P. M., Bergsten, P., and Lejonklou, M. H. (2019). Developmental exposure to a very low dose of bisphenol A induces persistent islet insulin hypersecretion in Fischer 344 rat offspring. Environ. Res. 172, 127–136. doi: 10.1016/j.envres.2019.02.009
Mao, Z., Xia, W., Huo, W., Zheng, T., Bassig, B. A., Chang, H., et al. (2017). Pancreatic impairment and Igf2 hypermethylation induced by developmental exposure to bisphenol A can be counteracted by maternal folate supplementation. J. Appl. Toxicol. 37, 825–835. doi: 10.1002/jat.3430
Marcoccia, D., Pellegrini, M., Fiocchetti, M., Lorenzetti, S., and Marino, M. (2017). Food components and contaminants as (anti) androgenic molecules. Genes Nutr. 12, 1–16. doi: 10.1186/s12263-017-0555-5
Maresca, M. M., Hoepner, L. A., Hassoun, A., Oberfield, S. E., Mooney, S. J., Calafat, A. M., et al. (2016). Prenatal exposure to phthalates and childhood body size in an urban cohort. Environ. Health Perspect. 124, 514–520. doi: 10.1289/ehp.1408750
Martin, A., Connelly, A., Bland, R. M., and Reilly, J. J. (2017). Health impact of catch-up growth in low-birth weight infants: systematic review, evidence appraisal, and meta-analysis. Matern. Child Nutr. 13, 1–13. doi: 10.1111/mcn.12297
Martinez-Arguelles, D. B., McIntosh, M., Rohlicek, C. V., Culty, M., Zirkin, B. R., and Papadopoulos, V. (2013). Maternal in utero exposure to the endocrine disruptor di-(2-ethylhexyl) phthalate affects the blood pressure of adult male offspring. Toxicol. Appl. Pharmacol. 266, 95–100. doi: 10.1016/j.taap.2012.10.027
Martinez-Arguelles, D. B., and Papadopoulos, V. (2016). Prenatal phthalate exposure: epigenetic changes leading to lifelong impact on steroid formation. Andrology 4, 573–584. doi: 10.1111/andr.12175
Martinez-Finley, E. J., Goggin, S. L., Labrecque, M. T., and Allan, A. M. (2011). Reduced expression of MAPK/ERK genes in perinatal arsenic-exposed offspring induced by glucocorticoid receptor deficits. Neurotoxicol. Teratol. 33, 530–537. doi: 10.1016/j.ntt.2011.07.003
Mendrick, D. L., Diehl, A. M., Topor, L. S., Dietert, R. R., Will, Y., La Merrill, M. A., et al. (2018). Metabolic syndrome and associated diseases: from the bench to the clinic. Toxicol. Sci. 162, 36–42. doi: 10.1093/toxsci/kfx233
Mierzynski, R., Dluski, D., Darmochwal-Kolarz, D., Leszczynska-Gorzelak, B., Kimber-Trojnar, Z., and Oleszczuk, J. (2016). Intra-uterine growth retardation as a risk factor of postnatal metabolic disorders. Curr. Pharm. Biotechnol. 17, 587–596. doi: 10.2174/1389201017666160301104323
Miyawaki, J., Sakayama, K., Kato, H., Yamamoto, H., and Masuno, H. (2007). Perinatal and postnatal exposure to bisphenol a increases adipose tissue mass and serum cholesterol level in mice. J. Atheroscler. Thromb. 14, 245–252. doi: 10.5551/jat.E486
Moel, D. I., and Sachs, H. K. (1992). Renal function 17 to 23 years after chelation therapy for childhood plumbism. Kidney Int. 42, 1226–1231. doi: 10.1038/ki.1992.408
Moel, D. I., Sachs, H. K., Cohn, R. A., and Drayton, M. A. (1985). Renal function 9 to 17 years after childhood lead poisoning. J. Pediatr. 106, 729–733. doi: 10.1016/S0022-3476(85)80344-9
Mohan Kumar, S. M., Rajendran, T. D., Vyas, A. K., Hoang, V., Asirvatham-Jeyaraj, N., Veiga-Lopez, A., et al. (2017). Effects of prenatal bisphenol-A exposure and postnatal overfeeding on cardiovascular function in female sheep. J. Dev. Orig. Health Dis. 8:65. doi: 10.1017/S204017441600057X
Morgan, J. M., Hartley, M. W., and Miller, R. E. (1966). Nephropathy in chronic lead poisoning. Arch. Intern. Med. 118, 17–29. doi: 10.1001/archinte.1966.00290130019005
Naranmandura, H., Xu, S., Sawata, T., Hao, W. H., Liu, H., Bu, N., et al. (2011). Mitochondria are the main target organelle for trivalent monomethylarsonous acid (MMAIII)-induced cytotoxicity. Chem. Res. Toxicol. 24, 1094–1103. doi: 10.1021/tx200156k
Nesatyy, V. J., Rutishauser, B. V., Eggen, R. I., and Suter, M. J.-F. (2005). Identification of the estrogen receptor Cd-binding sites by chemical modification. Analyst 130, 1087–1097. doi: 10.1039/b501192b
Nishizawa, H., Imanishi, S., and Manabe, N. (2005). Effects of exposure in utero to bisphenol a on the expression of aryl hydrocarbon receptor, related factors, and xenobiotic metabolizing enzymes in murine embryos. J. Reprod. Dev. 51, 593–605. doi: 10.1262/jrd.17026
Nuñez, P., Fernandez, T., García-Arévalo, M., Alonso-Magdalena, P., Nadal, A., Perillan, C., et al. (2018). Effects of bisphenol A treatment during pregnancy on kidney development in mice: a stereological and histopathological study. J. Dev. Orig. Health Dis. 9, 208–214. doi: 10.1017/S2040174417000939
Nye, L. (1929). An investigation of the extraordinary incidence of chronic nephritis in young people in Queensland. Med. J. Aust. 2. doi: 10.5694/j.1326-5377.1929.tb15729.x
Ohlstein, J. F., Strong, A. L., McLachlan, J. A., Gimble, J. M., Burow, M. E., and Bunnell, B. A. (2014). Bisphenol A enhances adipogenic differentiation of human adipose stromal/stem cells. J. Mol. Endocrinol. 53:345. doi: 10.1530/JME-14-0052
Osorio-Yanez, C., Ayllon-Vergara, J. C., Aguilar-Madrid, G., Arreola-Mendoza, L., Hernandez-Castellanos, E., Barrera-Hernandez, A., et al. (2013). Carotid intima-media thickness and plasma asymmetric dimethylarginine in Mexican children exposed to inorganic arsenic. Environ. Health Perspect. 121, 1090–1096. doi: 10.1289/ehp.1205994
Osorio-Yanez, C., Ayllon-Vergara, J. C., Arreola-Mendoza, L., Aguilar-Madrid, G., Hernandez-Castellanos, E., Sanchez-Pena, L. C., et al. (2015). Blood pressure, left ventricular geometry, and systolic function in children exposed to inorganic arsenic. Environ. Health Perspect. 123, 629–635. doi: 10.1289/ehp.1307327
Park, M. H., Gutiérrez-García, A. K., and Choudhury, M. (2019a). Mono-(2-ethylhexyl) phthalate aggravates inflammatory response via Sirtuin regulation and Inflammasome activation in RAW 264.7 cells. Chem. Res. Toxicol. 32, 935–942. doi: 10.1021/acs.chemrestox.9b00101
Park, M. H., Jeong, E., and Choudhury, M. (2019b). Mono-(2-ethylhexyl) phthalate regulates cholesterol efflux via microRNAs regulated m6A RNA methylation. Chem. Res. Toxicol. 33, 461–469. doi: 10.1021/acs.chemrestox.9b00367
Patel, B. B., Raad, M., Sebag, I. A., and Chalifour, L. E. (2013). Lifelong exposure to bisphenol a alters cardiac structure/function, protein expression, and DNA methylation in adult mice. Toxicol. Sci. 133, 174–185. doi: 10.1093/toxsci/kft026
Philips, E. M., Jaddoe, V. W. V., Asimakopoulos, A. G., Kannan, K., Steegers, E. A. P., Santos, S., et al. (2018). Bisphenol and phthalate concentrations and its determinants among pregnant women in a population-based cohort in the Netherlands. 2004-5. Environ. Res. 161, 562–572. doi: 10.1016/j.envres.2017.11.051
Prasanth, G., Divya, L., and Sadasivan, C. (2010). Bisphenol-A can bind to human glucocorticoid receptor as an agonist: an in silico study. J. Appl. Toxicol. 30, 769–774. doi: 10.1002/jat.1570
Rahman, M., Sohel, N., Yunus, M., Chowdhury, M. E., Hore, S. K., Zaman, K., et al. (2013). Increased childhood mortality and arsenic in drinking water in Matlab, Bangladesh: a population-based cohort study. PLoS ONE 8:e55014. doi: 10.1371/journal.pone.0055014
Rajesh, P., and Balasubramanian, K. (2015). Gestational exposure to di (2-ethylhexyl) phthalate (DEHP) impairs pancreatic β-cell function in F1 rat offspring. Toxicol. Lett. 232, 46–57. doi: 10.1016/j.toxlet.2014.09.025
Ramírez-Bajo, M. J., de Atauri, P., Ortega, F., Westerhoff, H. V., Gelpí, J. L., Centelles, J. J., et al. (2014). Effects of cadmium and mercury on the upper part of skeletal muscle glycolysis in mice. PLoS ONE 9:e80018. doi: 10.1371/journal.pone.0080018
Rasdi, Z., Kamaludin, R., Rahim, S. A., Fuad, S. B. S. A., Othman, M. H. D., Siran, R., et al. (2020). The impacts of intrauterine Bisphenol A exposure on pregnancy and expression of miRNAs related to heart development and diseases in animal model. Sci. Rep. 10, 1–13. doi: 10.1038/s41598-020-62420-1
Riu, A., Le Maire, A., Grimaldi, M., Audebert, M., Hillenweck, A., Bourguet, W., et al. (2011). Characterization of novel ligands of ERα, ERβ, and PPARγ: the case of halogenated bisphenol A and their conjugated metabolites. Toxicol. Sci. 122, 372–382. doi: 10.1093/toxsci/kfr132
Rodriguez, K. F., Ungewitter, E. K., Crespo-Mejias, Y., Liu, C., Nicol, B., Kissling, G. E., et al. (2016). Effects of in utero exposure to arsenic during the second half of gestation on reproductive end points and metabolic parameters in female CD-1 mice. Environ. Health Perspect. 124, 336–343. doi: 10.1289/ehp.1509703
Rogers, J. M., Ellis-Hutchings, R. G., Grey, B. E., Zucker, R. M., Norwood, J. Jr., Grace, C. E., et al. (2014). Elevated blood pressure in offspring of rats exposed to diverse chemicals during pregnancy. Toxicol. Sci. 137, 436–446. doi: 10.1093/toxsci/kft248
Ronco, A. M., Montenegro, M., Castillo, P., Urrutia, M., Saez, D., Hirsch, S., et al. (2011). Maternal exposure to cadmium during gestation perturbs the vascular system of the adult rat offspring. Toxicol. Appl. Pharmacol. 251, 137–145. doi: 10.1016/j.taap.2011.01.001
Rosenberg, H. G. (1973). Systemic arterial disease with myocardial infarction. Report on two infants. Circulation 47, 270–275. doi: 10.1161/01.CIR.47.2.270
Rosenberg, H. G. (1974). Systemic arterial disease and chronic arsenicism in infants. Arch. Pathol. 97, 360–365.
Rosofsky, A., Janulewicz, P., Thayer, K. A., McClean, M., Wise, L. A., Calafat, A. M., et al. (2017). Exposure to multiple chemicals in a cohort of reproductive-aged Danish women. Environ. Res. 154, 73–85. doi: 10.1016/j.envres.2016.12.011
Rouillon, S., Deshayes-Morgand, C., Enjalbert, L., Rabouan, S., Hardouin, J. B., Migeot, V., et al. (2017). Endocrine disruptors and pregnancy: knowledge, attitudes and prevention behaviors of French women. Int. J. Environ. Res. Public Health 14:1021. doi: 10.3390/ijerph14091021
Saillenfait, A. M., Payan, J. P., Brondeau, M. T., Zissu, D., and de Ceaurriz, J. (1991). Changes in urinary proximal tubule parameters in neonatal rats exposed to cadmium chloride during pregnancy. J. Appl. Toxicol. 11, 23–27. doi: 10.1002/jat.2550110105
Sargis, R. M., Johnson, D. N., Choudhury, R. A., and Brady, M. J. (2010). Environmental endocrine disruptors promote adipogenesis in the 3t3-l1 cell line through glucocorticoid receptor activation. Obesity 18, 1283–1288. doi: 10.1038/oby.2009.419
Sathyanarayana, S., Barrett, E., Butts, S., Wang, C., and Swan, S. H. (2014). Phthalate exposure and reproductive hormone concentrations in pregnancy. Reproduction 147, 401–409. doi: 10.1530/REP-13-0415
Savastano, S., Tarantino, G., D'Esposito, V., Passaretti, F., Cabaro, S., Liotti, A., et al. (2015). Bisphenol-A plasma levels are related to inflammatory markers, visceral obesity and insulin-resistance: a cross-sectional study on adult male population. J. Transl. Med. 13, 1–7. doi: 10.1186/s12967-015-0532-y
Seely, J. C. (2017). A brief review of kidney development, maturation, developmental abnormalities, and drug toxicity: juvenile animal relevancy. J. Toxicol. Pathol. 30, 125–133. doi: 10.1293/tox.2017-0006
Shi, H., Shi, X., and Liu, K. J. (2004). Oxidative mechanism of arsenic toxicity and carcinogenesis. Mol. Cell. Biochem. 255, 67–78. doi: 10.1023/B:MCBI.0000007262.26044.e8
Shimpi, P. C., More, V. R., Paranjpe, M., Donepudi, A. C., Goodrich, J. M., Dolinoy, D. C., et al. (2017). Hepatic lipid accumulation and Nrf2 expression following perinatal and peripubertal exposure to bisphenol A in a mouse model of nonalcoholic liver disease. Environ. Health Perspect. 125:087005. doi: 10.1289/EHP664
Shirani, M., Alizadeh, S., Mahdavinia, M., and Dehghani, M. A. (2019). The ameliorative effect of quercetin on bisphenol A-induced toxicity in mitochondria isolated from rats. Environ. Sci. Pollut. Res. 26, 7688–7696. doi: 10.1007/s11356-018-04119-5
Silva, M. J., Barr, D. B., Reidy, J. A., Malek, N. A., Hodge, C. C., Caudill, S. P., et al. (2004). Urinary levels of seven phthalate metabolites in the U.S. population from the National Health and Nutrition Examination Survey (NHANES) 1999-2000. Environ. Health Perspect. 112, 331–338. doi: 10.1289/ehp.6723
Simons, S. S. Jr., Chakraborti, P. K., and Cavanaugh, A. H. (1990). Arsenite and cadmium (II) as probes of glucocorticoid receptor structure and function. J. Biol. Chem. 265, 1938–1945. doi: 10.1016/S0021-9258(19)39922-3
Skinner, M. K., Manikkam, M., Tracey, R., Guerrero-Bosagna, C., Haque, M., and Nilsson, E. E. (2013). Ancestral dichlorodiphenyltrichloroethane (DDT) exposure promotes epigenetic transgenerational inheritance of obesity. BMC Med. 11:228. doi: 10.1186/1741-7015-11-228
Skroder, H., Hawkesworth, S., Kippler, M., El Arifeen, S., Wagatsuma, Y., Moore, S. E., et al. (2015). Kidney function and blood pressure in preschool-aged children exposed to cadmium and arsenic–potential alleviation by selenium. Environ. Res. 140, 205–213. doi: 10.1016/j.envres.2015.03.038
Smith, A. H., Marshall, G., Liaw, J., Yuan, Y., Ferreccio, C., and Steinmaus, C. (2012). Mortality in young adults following in utero and childhood exposure to arsenic in drinking water. Environ. Health Perspect. 120, 1527–1531. doi: 10.1289/ehp.1104867
Solomon, O., Yousefi, P., Huen, K., Gunier, R. B., Escudero-Fung, M., Barcellos, L. F., et al. (2017). Prenatal phthalate exposure and altered patterns of DNA methylation in cord blood. Environ. Mol. Mutagen 58, 398–410. doi: 10.1002/em.22095
Song, H., Park, J., Bui, P. T. C., Choi, K., Gye, M. C., Hong, Y. C., et al. (2017). Bisphenol A induces COX-2 through the mitogen-activated protein kinase pathway and is associated with levels of inflammation-related markers in elderly populations. Environ. Res. 158, 490–498. doi: 10.1016/j.envres.2017.07.005
Srivastava, S., D'Souza, S. E., Sen, U., and States, J. C. (2007). In utero arsenic exposure induces early onset of atherosclerosis in ApoE–/– mice. Reprod. Toxicol. 23, 449–456. doi: 10.1016/j.reprotox.2007.01.005
Srivastava, S., Vladykovskaya, E. N., Haberzettl, P., Sithu, S. D., D'Souza, S. E., and States, J. C. (2009). Arsenic exacerbates atherosclerotic lesion formation and inflammation in ApoE-/-mice. Toxicol. Appl. Pharmacol. 241, 90–100. doi: 10.1016/j.taap.2009.08.004
Staessen, J. A., Nawrot, T., Den Hond, E., Thijs, L., Fagard, R., Hoppenbrouwers, K., et al. (2001). Renal function, cytogenetic measurements, and sexual development in adolescents in relation to environmental pollutants: a feasibility study of biomarkers. Lancet 357, 1660–1669. doi: 10.1016/S0140-6736(00)04822-4
Staples, A. O., Greenbaum, L. A., Smith, J. M., Gipson, D. S., Filler, G., Warady, B. A., et al. (2010). Association between clinical risk factors and progression of chronic kidney disease in children. Clin. J. Am. Soc. Nephrol. 5, 2172–2179. doi: 10.2215/CJN.07851109
Stoica, A., Pentecost, E., and Martin, M. B. (2000). Effects of arsenite on estrogen receptor-α expression and activity in MCF-7 breast cancer cells. Endocrinology 141, 3595–3602. doi: 10.1210/endo.141.10.7704
Strakovsky, R. S., Wang, H., Engeseth, N. J., Flaws, J. A., Helferich, W. G., Pan, Y.-X., et al. (2015). Developmental bisphenol A (BPA) exposure leads to sex-specific modification of hepatic gene expression and epigenome at birth that may exacerbate high-fat diet-induced hepatic steatosis. Toxicol. Appl. Pharmacol. 284, 101–112. doi: 10.1016/j.taap.2015.02.021
Stratakis, N., Conti, D. V., Borras, E., Sabido, E., Roumeliotaki, T., Papadopoulou, E., et al. (2020). Association of fish consumption and mercury exposure during pregnancy with metabolic health and inflammatory biomarkers in children. JAMA Netw. Open 3:e201007. doi: 10.1001/jamanetworkopen.2020.1007
Sui, Y., Park, S. H., Wang, F., and Zhou, C. (2018). Perinatal bisphenol A exposure increases atherosclerosis in adult male PXR-humanized mice. Endocrinology 159, 1595–1608. doi: 10.1210/en.2017-03250
Tada, Y., Fujitani, T., Yano, N., Takahashi, H., Yuzawa, K., Ando, H., et al. (2006). Effects of tetrabromobisphenol A, brominated flame retardant, in ICR mice after prenatal and postnatal exposure. Food Chem. Toxicol. 44, 1408–1413. doi: 10.1016/j.fct.2006.03.006
Tang-Péronard, J. L., Heitmann, B. L., Andersen, H. R., Steuerwald, U., Grandjean, P., Weihe, P., et al. (2014). Association between prenatal polychlorinated biphenyl exposure and obesity development at ages 5 and 7 y: a prospective cohort study of 656 children from the Faroe Islands. Am. J. Clin. Nutr. 99, 5–13. doi: 10.3945/ajcn.113.066720
Tang-Péronard, J. L., Heitmann, B. L., Jensen, T. K., Vinggaard, A. M., Madsbad, S., Steuerwald, U., et al. (2015). Prenatal exposure to persistent organochlorine pollutants is associated with high insulin levels in 5-year-old girls. Environ. Res. 142, 407–413. doi: 10.1016/j.envres.2015.07.009
Tchounwou, P. B., Yedjou, C. G., Patlolla, A. K., and Sutton, D. J. (2012). Heavy metal toxicity and the environment. Mol. Clin. Environ. Toxicol. 133–164. doi: 10.1007/978-3-7643-8340-4_6
Tian, X., Feng, J., Dong, N., Lyu, Y., Wei, C., Li, B., et al. (2019a). Subchronic exposure to arsenite and fluoride from gestation to puberty induces oxidative stress and disrupts ultrastructure in the kidneys of rat offspring. Sci. Tot. Environ. 686, 1229–1237. doi: 10.1016/j.scitotenv.2019.04.409
Tian, X., Xie, J., Chen, X., Dong, N., Feng, J., Gao, Y., et al. (2019b). Deregulation of autophagy is involved in nephrotoxicity of arsenite and fluoride exposure during gestation to puberty in rat offspring. Arch. Toxicol. 94, 749–760. doi: 10.1007/s00204-019-02651-y
Tinkelman, N. E., Spratlen, M. J., Domingo-Relloso, A., Tellez-Plaza, M., Grau-Perez, M., Francesconi, K. A., et al. (2020). Associations of maternal arsenic exposure with adult fasting glucose and insulin resistance in the Strong Heart Study and Strong Heart Family Study. Environ. Int. 137:105531. doi: 10.1016/j.envint.2020.105531
Tokar, E. J., Diwan, B. A., and Waalkes, M. P. (2012). Renal, hepatic, pulmonary and adrenal tumors induced by prenatal inorganic arsenic followed by dimethylarsinic acid in adulthood in CD1 mice. Toxicol. Lett. 209, 179–185. doi: 10.1016/j.toxlet.2011.12.016
Tran, V., Tindula, G., Huen, K., Bradman, A., Harley, K., Kogut, K., et al. (2017). Prenatal phthalate exposure and 8-isoprostane among Mexican-American children with high prevalence of obesity. J. Dev. Orig. Health Dis. 8:196. doi: 10.1017/S2040174416000763
Trasande, L., Attina, T. M., and Trachtman, H. (2013a). Bisphenol A exposure is associated with low-grade urinary albumin excretion in children of the United States. Kidney Int. 83, 741–748. doi: 10.1038/ki.2012.422
Trasande, L., Sathyanarayana, S., Spanier, A. J., Trachtman, H., Attina, T. M., and Urbina, E. M. (2013b). Urinary phthalates are associated with higher blood pressure in childhood. J. Pediatr. 163, 747–753. doi: 10.1016/j.jpeds.2013.03.072
Trasande, L., Sathyanarayana, S., and Trachtman, H. (2014). Dietary phthalates and low-grade albuminuria in US children and adolescents. Clin. J. Am. Soc. Nephrol. 9, 100–109. doi: 10.2215/CJN.04570413
Trasande, L., Zoeller, R. T., Hass, U., Kortenkamp, A., Grandjean, P., Myers, J. P., et al. (2016). Burden of disease and costs of exposure to endocrine disrupting chemicals in the European Union: an updated analysis. Andrology 4, 565–572. doi: 10.1111/andr.12178
Troisi, R., Hyer, M., Hatch, E. E., Titus-Ernstoff, L., Palmer, J. R., Strohsnitter, W. C., et al. (2013). Medical conditions among adult offspring prenatally exposed to diethylstilbestrol. Epidemiology 24, 430–438. doi: 10.1097/EDE.0b013e318289bdf7
Troisi, R., Titus, L., Hatch, E. E., Palmer, J. R., Huo, D., Strohsnitter, W. C., et al. (2018). A prospective cohort study of prenatal diethylstilbestrol exposure and cardiovascular disease risk. J. Clin. Endocrinol. Metab. 103, 206–212. doi: 10.1210/jc.2017-01940
Tsai, H. J., Chen, B. H., Wu, C. F., Wang, S. L., Huang, P. C., Tsai, Y. C., et al. (2016). Intake of phthalate-tainted foods and microalbuminuria in children: the 2011 Taiwan food scandal. Environ. Int. 89–90, 129–137. doi: 10.1016/j.envint.2016.01.015
Vafeiadi, M., Georgiou, V., Chalkiadaki, G., Rantakokko, P., Kiviranta, H., Karachaliou, M., et al. (2015). Association of prenatal exposure to persistent organic pollutants with obesity and cardiometabolic traits in early childhood: the Rhea mother-child cohort (Crete, Greece). Environ. Health Perspect. 123, 1015–1021. doi: 10.1289/ehp.1409062
Valvi, D., Casas, M., Romaguera, D., Monfort, N., Ventura, R., Martinez, D., et al. (2015). Prenatal phthalate exposure and childhood growth and blood pressure: evidence from the spanish inma-sabadell birth cohort study. Environ. Health Perspect. 123, 1022–1029. doi: 10.1289/ehp.1408887
Valvi, D., Mendez, M. A., Garcia-Esteban, R., Ballester, F., Ibarluzea, J., Goni, F., et al. (2014). Prenatal exposure to persistent organic pollutants and rapid weight gain and overweight in infancy. Obesity 22, 488–496. doi: 10.1002/oby.20603
Van Esterik, J., Verharen, H., Hodemaekers, H., Gremmer, E., Nagarajah, B., Kamstra, J., et al. (2015). Compound-and sex-specific effects on programming of energy and immune homeostasis in adult C57BL/6JxFVB mice after perinatal TCDD and PCB 153. Toxicol. Appl. Pharmacol. 289, 262–275. doi: 10.1016/j.taap.2015.09.017
Vandenberg, L. N., Chahoud, I., Heindel, J. J., Padmanabhan, V., Paumgartten, F. J., and Schoenfelder, G. (2010). Urinary, circulating, and tissue biomonitoring studies indicate widespread exposure to bisphenol A. Environ. Health Perspect. 118, 1055–1070. doi: 10.1289/ehp.0901716
Vandenberg, L. N., Colborn, T., Hayes, T. B., Heindel, J. J., Jacobs, D. R. Jr, Lee, D. H., et al. (2012). Hormones and endocrine-disrupting chemicals: low-dose effects and nonmonotonic dose responses. Endocr. Rev. 33, 378–455. doi: 10.1210/er.2011-1050
Veiga-Lopez, A., Kannan, K., Liao, C., Ye, W., Domino, S. E., and Padmanabhan, V. (2015). Gender-specific effects on gestational length and birth weight by early pregnancy BPA exposure. J. Clin. Endocrinol. Metab. 100, E1394–E1403. doi: 10.1210/jc.2015-1724
Verberk, M. M., Willems, T. E., Verplanke, A. J., and Wolff, F. A. D. (1996). Environmental lead and renal effects in children. Arch. Environ. Health Int. J. 51, 83–87. doi: 10.1080/00039896.1996.9935998
Viselina, T., and Luk'Yanova, O. (2000). Cadmium-induced changes in the activity of carbohydrate metabolism enzymes in mollusks. Russ. J. Mar. Biol. 26, 289–291. doi: 10.1007/BF02759511
Walker, C. L. (2016). Minireview: epigenomic plasticity and vulnerability to EDC exposures. Mol. Endocrinol. 30, 848–855. doi: 10.1210/me.2016-1086
Wang, C., Zhang, J., Li, Q., Zhang, T., Deng, Z., Lian, J., et al. (2017). Low concentration of BPA induces mice spermatocytes apoptosis via GPR30. Oncotarget 8:49005. doi: 10.18632/oncotarget.16923
Wang, G., DiBari, J., Bind, E., Steffens, A. M., Mukherjee, J., Bartell, T. R., et al. (2019). In utero exposure to mercury and childhood overweight or obesity: counteracting effect of maternal folate status. BMC Med. 17, 1–10. doi: 10.1186/s12916-019-1442-2
Wang, H., Naghavi, M., Allen, C., Barber, R. M., Bhutta, Z. A., Carter, A., et al. (2016). Global, regional, and national life expectancy, all-cause mortality, and cause-specific mortality for 249 causes of death. 1980–2015: a systematic analysis for the Global Burden of Disease Study 2015. Lancet 388, 1459–1544. doi: 10.1016/S0140-6736(16)31012-1
Warady, B. A., Abraham, A. G., Schwartz, G. J., Wong, C. S., Muñoz, A., Betoko, A., et al. (2015). Predictors of rapid progression of glomerular and nonglomerular kidney disease in children and adolescents: the chronic kidney disease in children (CKiD) cohort. Am. J. Kidney Dis. 65, 878–888. doi: 10.1053/j.ajkd.2015.01.008
Watkins, D. J., Ferguson, K. K., Del Toro, L. V. A., Alshawabkeh, A. N., Cordero, J. F., and Meeker, J. D. (2015). Associations between urinary phenol and paraben concentrations and markers of oxidative stress and inflammation among pregnant women in Puerto Rico. Int. J. Hyg. Environ. Health 218, 212–219. doi: 10.1016/j.ijheh.2014.11.001
Weaver, V. M., Lee, B. K., Ahn, K. D., Lee, G. S., Todd, A. C., Stewart, W. F., et al. (2003). Associations of lead biomarkers with renal function in Korean lead workers. Occup. Environ. Med. 60, 551–562. doi: 10.1136/oem.60.8.551
Weidemann, D., Kuo, C. C., Navas-Acien, A., Abraham, A. G., Weaver, V., and Fadrowski, J. (2015). Association of arsenic with kidney function in adolescents and young adults: results from the National Health and Nutrition Examination Survey 2009-2012. Environ. Res. 140, 317–324. doi: 10.1016/j.envres.2015.03.030
Welshons, W. V., Thayer, K. A., Judy, B. M., Taylor, J. A., Curran, E. M., and vom Saal, F. S. (2003). Large effects from small exposures. I. Mechanisms for endocrine-disrupting chemicals with estrogenic activity. Environ. Health Perspect. 111, 994–1006. doi: 10.1289/ehp.5494
Wen, Y., Rattan, S., Flaws, J. A., and Irudayaraj, J. (2020). Multi and transgenerational epigenetic effects of di-(2-ethylhexyl) phthalate (DEHP) in liver. Toxicol. Appl. Pharmacol. 402:115123. doi: 10.1016/j.taap.2020.115123
Wolff, M. S., Engel, S. M., Berkowitz, G. S., Ye, X., Silva, M. J., Zhu, C., et al. (2008). Prenatal phenol and phthalate exposures and birth outcomes. Environ. Health Perspect. 116, 1092–1097. doi: 10.1289/ehp.11007
Wong, H., Mylrea, K., Feber, J., Drukker, A., and Filler, G. (2006). Prevalence of complications in children with chronic kidney disease according to KDOQI. Kidney Int. 70, 585–590. doi: 10.1038/sj.ki.5001608
Woodruff, T. J., Zota, A. R., and Schwartz, J. M. (2011). Environmental chemicals in pregnant women in the United States: NHANES 2003-2004. Environ. Health Perspect. 119, 878–885. doi: 10.1289/ehp.1002727
Wu, C. F., Hsiung, C. A., Tsai, H. J., Tsai, Y. C., Hsieh, H. M., Chen, B. H., et al. (2018a). Interaction of melamine and di-(2-ethylhexyl) phthalate exposure on markers of early renal damage in children: the 2011 Taiwan food scandal. Environ. Pollut. 235, 453–461. doi: 10.1016/j.envpol.2017.12.107
Wu, W., Wu, P., Yang, F., Sun, D. L., Zhang, D. X., and Zhou, Y. K. (2018b). Association of phthalate exposure with anthropometric indices and blood pressure in first-grade children. Environ. Sci. Pollut. Res. 25, 23125–23134. doi: 10.1007/s11356-018-2447-7
Yang, J., Liu, D., Jing, W., Dahms, H.-U., and Wang, L. (2013). Effects of cadmium on lipid storage and metabolism in the freshwater crab Sinopotamon henanense. PLoS ONE 8:e77569. doi: 10.1371/journal.pone.0077569
Yuan, Y., Marshall, G., Ferreccio, C., Steinmaus, C., Selvin, S., Liaw, J., et al. (2007). Acute myocardial infarction mortality in comparison with lung and bladder cancer mortality in arsenic-exposed region II of Chile from 1950 to 2000. Am. J. Epidemiol. 166, 1381–1391. doi: 10.1093/aje/kwm238
Zalups, R. K., and Lash, L. H. (1994). Advances in understanding the renal transport and toxicity of mercury. J. Toxicol. Environ. Health 42, 1–44. doi: 10.1080/15287399409531861
Zhang, J., Yang, Y., Liu, W., Schlenk, D., and Liu, J. (2019). Glucocorticoid and mineralocorticoid receptors and corticosteroid homeostasis are potential targets for endocrine-disrupting chemicals. Environ. Int. 133:105133. doi: 10.1016/j.envint.2019.105133
Zhang, J., Zhang, T., Guan, T., Yu, H., and Li, T. (2017). In vitro and in silico assessment of the structure-dependent binding of bisphenol analogues to glucocorticoid receptor. Anal. Bioanal. Chem. 409, 2239–2246. doi: 10.1007/s00216-016-0168-7
Zhang, Y., Jiao, Y., Tao, Y., Li, Z., Yu, H., Han, S., et al. (2021). Monobutyl phthalate can induce autophagy and metabolic disorders by activating the ire1a-xbp1 pathway in zebrafish liver. J. Hazard. Mater. 412:125243. doi: 10.1016/j.jhazmat.2021.125243
Zhang, Y., Zhang, W., Fu, X., Zhou, F., Yu, H., and Na, X. (2018). Transcriptomics and metabonomics analyses of maternal DEHP exposure on male offspring. Environ. Sci. Pollut. Res. 25, 26322–26329. doi: 10.1007/s11356-018-2596-8
Zhang, Z., Alomirah, H., Cho, H. S., Li, Y. F., Liao, C., Minh, T. B., et al. (2011). Urinary bisphenol A concentrations and their implications for human exposure in several Asian countries. Environ. Sci. Technol. 45, 7044–7050. doi: 10.1021/es200976k
Zheng, L., Kuo, C. C., Fadrowski, J., Agnew, J., Weaver, V. M., and Navas-Acien, A. (2014). Arsenic and chronic kidney disease: a systematic review. Curr. Environ. Health Rep. 1, 192–207. doi: 10.1007/s40572-014-0024-x
Zota, A. R., Geller, R. J., Romano, L. E., Coleman-Phox, K., Adler, N. E., Parry, E., et al. (2018). Association between persistent endocrine-disrupting chemicals (PBDEs, OH-PBDEs, PCBs, and PFASs) and biomarkers of inflammation and cellular aging during pregnancy and postpartum. Environ. Int. 115, 9–20. doi: 10.1016/j.envint.2018.02.044
Keywords: prenatal exposure, DOHAD, cardio-metabolic-renal health, endocrine disrupting chemicals, cardiovascular disorder
Citation: Singh RD, Koshta K, Tiwari R, Khan H, Sharma V and Srivastava V (2021) Developmental Exposure to Endocrine Disrupting Chemicals and Its Impact on Cardio-Metabolic-Renal Health. Front. Toxicol. 3:663372. doi: 10.3389/ftox.2021.663372
Received: 02 February 2021; Accepted: 10 May 2021;
Published: 05 July 2021.
Edited by:
Stephane Louis Bourque, University of Alberta, CanadaReviewed by:
Alison Holloway, McMaster University, CanadaCopyright © 2021 Singh, Koshta, Tiwari, Khan, Sharma and Srivastava. This is an open-access article distributed under the terms of the Creative Commons Attribution License (CC BY). The use, distribution or reproduction in other forums is permitted, provided the original author(s) and the copyright owner(s) are credited and that the original publication in this journal is cited, in accordance with accepted academic practice. No use, distribution or reproduction is permitted which does not comply with these terms.
*Correspondence: Vineeta Sharma, dmluZWV0YS5wYWx3YWxAZ21haWwuY29t
†These authors have contributed equally to this work
Disclaimer: All claims expressed in this article are solely those of the authors and do not necessarily represent those of their affiliated organizations, or those of the publisher, the editors and the reviewers. Any product that may be evaluated in this article or claim that may be made by its manufacturer is not guaranteed or endorsed by the publisher.
Research integrity at Frontiers
Learn more about the work of our research integrity team to safeguard the quality of each article we publish.