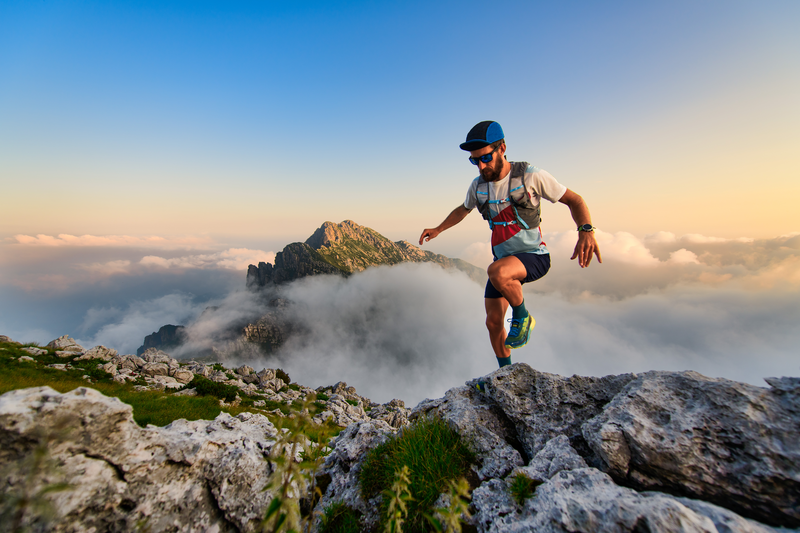
95% of researchers rate our articles as excellent or good
Learn more about the work of our research integrity team to safeguard the quality of each article we publish.
Find out more
REVIEW article
Front. Sens. , 08 November 2024
Sec. Sensor Devices
Volume 5 - 2024 | https://doi.org/10.3389/fsens.2024.1456669
In this review, the forefront of biosensor development has been marked by a profound exploration of carbon nanostructured materials for the specific application of glucose detection. Moreover, this progressive line of inquiry capitalizes on the distinctive attributes of carbon nanostructured materials such as carbon nanotubes, carbon quantum dots, and graphene which exhibit unique characteristics in the development of biosensor engineering design. It also enhanced analytical performances regarding the limit of detection, selectivity, sensitivity, and reproducibility towards glucose detection in biological samples. Most importantly, the strategic integration of carbon nanostructured-based biosensor architectures has played a significant role in advancements, characterized by heightened sensitivity, exquisite selectivity, and augmented stability in glucose detection processes. Furthermore, utilizing these advanced materials has engendered a transformative impact on electrochemical properties, propelling the biosensors to achieve rapid and precise glucose-sensing capabilities. The confluence of carbon nanostructures with biosensor technology has not only elevated the scientific understanding of glucose detection mechanisms. Still, it has also paved the way for miniaturized and portable biosensors. This transformative shift holds great promise for the realization of point-of-care diagnostics, representing a pivotal step towards durability and efficient glucose monitoring in health/medical care. These advancements emphasize the crucial role of carbon nanostructured-based biosensors in opening the way to a new avenue of superiority and effectiveness in diabetes management. Conclusively, the challenges and, in a forward-looking stance, the prospective futures of glucose biosensors anchored on carbon nanostructured frameworks were considered.
Diabetes is one of the leading fatal diseases globally, and thus directly causes more than 1.5 million deaths in 2019 alone (Xu et al., 2024). However, if it is detected on time, it can be prevented and managed through continuous monitoring of glucose sugar levels. The optimal blood glucose range for a healthy individual is between 70 and 100 mg/dL (Rodriguez Ayala et al., 2024). Elevated blood glucose levels can lead to diabetes-related complications such as neuropathy, retinopathy, and cardiovascular diseases (Kakoti et al., 2024). Moreover, deviations from this acceptable range, whether hyperglycemia or hypoglycemia, can precipitate severe health issues (Lorber et al., 2024). Chronic hyperglycemia can be associated with microvascular and macrovascular complications but not limited to retinopathy, nephropathy, and cardiovascular diseases (Hossain et al., 2024). The delicate balance of glucose homeostasis is crucial, as fluctuations beyond the acceptable range can compromise the integrity of vital organs such as the heart, kidneys, and eyes, ultimately leading to significant morbidity (Veerapandi and Sekar, 2024). Analytical methods have proven useful in reliably monitoring glucose levels in the blood (Arias-Rivera et al., 2024). For example, the indirect detection of enzymatic byproducts resulting from the glucose oxidation detection method has been used. Moreover, based on the type of enzyme used, the most widely used method are glucose oxidase (GOx) method (Lee et al., 2024), which uses the enzyme glucose oxidase to catalyze glucose oxidation and measure hydrogen peroxide, and the hexokinase method (Dickson et al., 2019). Another method is the detection of glucose using hexokinase to phosphorylate glucose and measure dihydronicotinamide adenine dinucleotide (NADH production). Additionally, optical methods that utilize a dip-stick (urine glucose test strips), have been adopted as rapid analytical kits, that detect glucose levels by visual or photometric analysis (Nikitina et al., 2023). High-performance liquid chromatography (HPLC) (Tiwari et al., 2023), are also amongst techniques that have been used for glucose detection, through separating glucose molecules from other components as well as separating glucose based on its charge-to-size ratio, respectively (Sarkozy et al., 2023). However, these conventional glucose detection methods face several drawbacks. For instance, glucose oxidase and hexokinase methods conflict with other coexisting substances and require expensive reagents. Tests like Benedict’s and Fehling’s are non-specific and only provide semi-quantitative results. Similarly, HPLC and capillary electrophoresis require expensive, sophisticated equipment and are time-consuming.
Electrochemical sensors have emerged as alternative glucose detection systems (Welegergs et al., 2024; Welegergs et al., 2024; Govindaraj et al., 2023). Traditionally, the detection of glucose using electrochemical sensors involves bio-recognition elements such as enzymes (Harun-Or-Rashid et al., 2024). Even though, they are intricate and costly to extract, purify, and stabilize (Zheng et al., 2024); to obtain accurate readings, the utilization of enzymes generally leads to low limits of detection (Harun-Or-Rashid et al., 2024). The emergence of nanotechnology with nanomaterials has further improved the performance of glucose sensors, since these materials can act as electron shuttles during glucose oxidation, leading to high analytical signals (Dua et al., 2024). Additionally, some nanomaterials such as metal nanoparticles have been reported to exhibit redox couples/species Pt, Ru, Pd, Ag, Cu, and Fe in an electrochemical environment, thereby allowing them to undergo oxidation-reduction reactions (Liu et al., 2024; Yang et al., 2024). However, these metal nanoparticles are still expensive to produce and upscale for industrial applications. On the other hand, carbon-based nanomaterials are the best alternative nanomaterials due to their excellent conductivity and fast electron transfer kinetics, which are crucial in obtaining enhanced signals quickly (Hegde et al., 2024). Moreover, electrochemical sensors stand out due to their specificity, sensitivity, portability, and cost-effectiveness, making them ideal for a wide range of applications, particularly in fields requiring rapid and on-site analysis (Gezahegn et al., 2024; Ambaye et al., 2023a; Demeke et al., 2017; Ambaye et al., 2023b). In this review, the focus is on the application of specific carbon-based nanostructures in electrochemical biosensors for glucose detection across various sample types. Furthermore, this review highlights the role of carbon nanostructured materials in glucose sensing, emphasizing their potential advancements in healthcare. However, the review mainly focuses on graphene, carbon nanotubes (CNTs), and carbon quantum dots (CQDs), with only a brief mention of other promising carbon forms, such as mesoporous carbon and carbon nanofibers. Moreover, a broader examination of these materials would provide a more comprehensive understanding of carbon-based nanostructures in glucose sensing (Yuan et al., 2020).
More importantly, a comprehensive search in the Scopus database was performed using the query (title (glucose) and keyword (electrochemical and sensor)) covering the period from 2013 to 2023. This search yielded a total of 2,529 documents, as illustrated in Figure 1. An analysis of the publication trends reveals a consistent increase in the number of research articles over the years, reflecting the growing interest and advancements in the field of glucose electrochemical sensors. The rising trend can be attributed to the increasing demand for accurate and reliable glucose monitoring systems, driven by the global prevalence of diabetes and the need for improved healthcare solutions. Notably, significant spikes in publications were observed in recent years, indicating accelerated research and development efforts in this domain. Thus, requires the need to highlight insights and perspectives/trends in the developing trends of analytically monitoring glucose where carbon-based nanostructured biosensors are gaining popularity (Gupta and Tai, 2023).
Figure 1. Advancements in glucose detection via electrochemical sensors: A Comprehensive Analysis from 2013 to 2023 based on scopus database extracted.
The first-generation glucose biosensor was invented by Clark and Lyons (1962) in 1961. It consisted of an oxygen electrode, an inner semipermeable layer for oxygen, a thin layer of glucose oxidase (GOx), and an outer dialysis membrane. Updike and Hicks later simplified this method by immobilizing and stabilizing GOx in a polyacrylamide gel on an oxygen electrode, allowing glucose concentration to be measured in biofluids (Hicks and Updike, 1966). This advancement led to the introduction of the first commercial glucose sensors, which employed Clark’s method and were based on the amperometric detection of hydrogen peroxide (Juska and Pemble, 2020). These developments paved the way for the manufacture of glucose test strips and the commercialization of glucometer devices currently available on the market. However, conventional glucose test strips still have some drawbacks (Ortiz-Martínez et al., 2021). Recently, there has been growing interest in the direct measurement of glucose at the electrode layer (Bruen et al., 2017). The following sections evaluate various carbon-based materials that can provide improved analytical signals at different applied voltages or currents. Due to their structural, morphological, and electrochemical properties, carbon-based materials are well-suited for non-enzymatic glucose detection (Malode et al., 2024). The emergence of these materials has also facilitated the development of non-invasive techniques for continuous and point-of-care blood glucose monitoring (Harun-Or-Rashid et al., 2024), as well as glucose detection in various biofluids such as sweat, tears, urine, saliva, and interstitial fluids (Majdinasab, 2024). Therefore, it is crucial to assess the performance of these carbon nanomaterials to understand their electrochemical behavior throughout materials development, device fabrication, and system performance.
Carbon nanostructured-based electrode materials, particularly graphene, carbon nanotubes (CNTs), and carbon quantum dots (CQDs), have been employed for glucose detection due to their attractive properties (Ambaye et al., 2021; Ambaye et al., 2023c). Graphene offers an extensive surface area and excellent conductivity, facilitating efficient electron transfer and high sensitivity in electrochemical sensors (Ambaye et al., 2022). Typically, CNTs provide similar benefits with their tubular structure, enhancing the interaction with glucose molecules and improving the sensor’s responsiveness (Fall et al., 2022). Likewise, CQDs with their unique optical and electronic properties, enable precise and selective glucose detection when integrated with nanomaterials (Gao et al., 2023). These materials could be functionalized with enzymes or employed in non-enzymatic sensors, significantly improving their performance and stability. Hence, carbon nanostructured-based electrode materials are utilized in electrochemical and biosensing applications through functionalizing and immobilizing various electrode types, including printed electrodes, carbon paste electrodes, glassy carbon electrodes, and gold electrodes, as depicted in Figure 2. Interestingly, they contribute to the development of highly sensitive, selective, and rapid glucose sensors suitable for on-spot as well as wearable and point-of-care devices, addressing the growing need for effective diabetes management tools (Safarkhani et al., 2023). The development of nanotechnology to enhance bare electrodes shows significant promise in amplifying output signals for glucose detection (Biswas et al., 2023). This review discusses in detail the use of carbon nanostructured materials such as carbon nanotubes, graphene, and carbon quantum dots for electrode modification and their analytical performances (Liao et al., 2023).
Figure 2. Illustration and basic principles of the electrochemical sensor of glucose at carbon nanostructured-based electrodes.
CNT-based electrode materials have revolutionized glucose detection technologies due to their outstanding physicochemical properties (Thangamuthu et al., 2018). The high surface-to-volume ratio of CNTs offers an extensive active surface area, promoting increased interaction with glucose molecules and significantly enhancing detection sensitivity (Yuwen et al., 2023). Furthermore, their excellent electrical conductivity enables efficient electron transfer during the electrochemical oxidation of glucose, which is crucial for generating a robust and reliable signal. Moreover, the attractive mechanical strength and flexibility of CNTs contribute to the durability and resilience of glucose sensors, while their chemical stability ensures consistent performance across various environments. The functionalization of these CNT-based materials further amplifies their effectiveness in glucose detection applications (Tang et al., 2004). Importantly, they can be functionalized/immobilized with enzymes like glucose oxidase or metallic nanoparticles to enhance their specificity and sensitivity, thereby enhancing the total efficiency of glucose analysis systems and accurate point-of-care testing (Yuwen et al., 2023). Several CNT-based electrode materials have been reported for glucose detection in various biological matrices. For example, Xia et al., have reported the electrode material composed of CNTs, multi-walled carbon nanotubes (MWCNTs), and polydimethylsiloxane (PDMS) (CMP) has demonstrated impressive performance in wearable glucose detection in sweat, as reported by using the amperometry electrochemical technique as described in Figure 3. Moreover, the sensor exhibits a wider linear range from 20 μmol L−1–1.1 mmol L−1, with a notable sensitivity of 71.62 μA mM−1 cm−2 and a low detection limit of 6.78 μM (Xia et al., 2023). This innovative approach highlights the potential for reliable, glucose monitoring in wearable sensing applications.
Figure 3. (A) Schematic illustration of the bimetallic MOF coated CNT/PDMS film electrode for detection of glucose in sweat, (B) schematic representation of the NCMP glucose sensor fabrication, (C) time-current response curve of the NCMP film electrode to various glucose concentrations, and (D) calibration curve showing the current response of different glucose concentrations (Xia et al., 2023).
Recently, Huo et al. (2023), introduced a glucose sensor using gold nanoparticle (Au NP), synthesized through the seed-mediated growth process, and solution-processed zirconia nanoparticles (ZrO2 NP), on modified MWCNTs. This Au/ZrO2@MWCNTs composite shows a wide linear detection range from 10 μM to 13 mM, as illustrated in Figure 4, high sensitivity of 162.52 μA mM−1 cm−2, and a low detection limit of 0.95 μM. The sensor retains over 90% of its initial current after 15 days of continuous use, demonstrating excellent long-term stability. The study further validates the practical applicability of the sensor in simulated serum samples, indicating its potential for real-world clinical use. The integration of Au and ZrO2 nanoparticles on MWCNTs enhances the sensor’s catalytic efficiency and contributes to its durability, making it a robust choice for glucose detection in various settings.
Figure 4. (A) The timing current curves of Au/ZrO2@MWCNTs/GCE for different concentrations of glucose (the inset shows the timing current curve at glucose) (B) the calibration curve between current and glucose concentration, and (C) selectivity study of the electrode (Huo et al., 2023).
Similarly, Choi et al. (2019) have synthesized hydrophilic and biocompatible chitosan (Chit) core-shelled CNTs. Electrodes with three different Chit: CNT weight ratios were prepared and characterized using FT-IR, XPS, XRD, SEM, and TEM. The Chit-CNT85 composite (85 wt% Chit) showed optimal performance and was covalently modified with glucose oxidase (GOx) to form the GOx-Chit-CNT85/ITO electrode as described in Figures 5A, B. This electrode demonstrated a fast electron-transfer constant (ks) of 8.2 s−1 and responded selectively to glucose without interference from ascorbic acid and uric acid, indicating its potential for mediator-free glucose sensing. Further, in a groundbreaking study by Nascimento et al. (2024), development of a modified NiFe2O4@MWCNT composite electrode material for amperometric sensing was reported (Figures 5C, D). This innovative electrode, as used to analyze urine samples, exhibited an impressive concentration detection range between 50 and 600 μmol L−1, with a 3-fold boasting of sensitivity (0.018 μA L µmol−1) compared to unmodified MWCNT electrode and an exceptionally low detection limit of 36 μmol L−1.
Figure 5. (A) Preparation of GOxChit-CNT85/ITO and (B) GOxChit-CNT85/ITO application for glucose sensing (Choi et al., 2019); (C) amperometric response interfering species, (D) BIA amperogram for injections of standard solutions of glucose (Nascimento et al., 2024); (E) preparation of (CH-GOx/PPy-Nf-fMWCNTs/GCE), and (F) amperometric response of the CH-GOx/PPy-Nf-fMWCNTs/GCE, and corresponding calibration curve (Shrestha et al., 2016).
Nascimento et al., have employed a novel bio-nanohybrid film comprising polypyrrole (PPy), Nafion (Nf), and functionalized fMWCNTs, expertly developed on a glassy carbon electrode (GCE) as depicted in Figures 5E, F. The electrochemical characteristics of this state-of-the-art biosensor were meticulously analyzed using cyclic voltammetry (CV), electrochemical impedance spectroscopy (EIS), and amperometry. The biosensor, as demonstrated in blood serum, exhibited extraordinary sensitivity, achieving 2860.3 μA mM−1 cm−2, with an extensive linear range of up to 4.7 mM (R2 0.99) and an exceptionally low detection limit of 5 μM (Shrestha et al., 2016). It was noted that CNT-based glucose sensors, whether enzymatic or nonenzymatic, proved effective by facilitating fast electron transfer, stable immobilization of enzymes, and deposition or dispersion of highly catalytic metal respectively.
CQDs are carbon nanoparticles less than 10 nm in size, known for their remarkable properties (Liu et al., 2020; Li et al., 2020). Discovered accidentally in 2004, during the purification of single-walled carbon nanotubes using gel electrophoresis (Xu et al., 2004), CQDs were later named and synthesized by Sun et al. (2006). Since then, CQDs have emerged as promising candidates for various applications in fields such as bioimaging, drug delivery, sensors, and optoelectronics due to their unique properties (Patel et al., 2019). Their highly tunable bandgap, excellent photoluminescence, good biocompatibility, and large surface area make them desirable for a wide range of uses (Yadav et al., 2023). CQDs can be easily functionalized with different surface groups, allowing for tailored interactions with biological systems or specific target molecules (Dua et al., 2023). This has led to significant interest in exploring their potential in medical diagnostics and therapeutics, where their small size and low toxicity are advantageous (Zhang et al., 2021). Additionally, CQDs can be synthesized using relatively simple and cost-effective methods, making them more accessible for various research and industrial applications (Wang et al., 2019). CQDs have emerged as a promising nanomaterial in the field of biosensing due to their unique properties (Ambaye et al., 2022; Safarkhani et al., 2023), better biocompatibility, photostability, and lower toxicity compared to traditional materials (Dua et al., 2023; Wang et al., 2022). One of the key advantages of CQDs in biosensing is their fluorescent labels (Lim et al., 2015). CQDs can be specifically functionalized to bind to specific biomolecules, such as glucose, allowing for real-time monitoring of their levels (Pourmadadi et al., 2023). This fluorescent property enables sensitive and accurate detection of biomolecules, providing a valuable tool in biosensing applications. Furthermore, CQDs enable non-enzymatic sensing (Wang et al., 2014), which simplifies sensor design and reduces interference. Unlike traditional enzymatic sensors, CQDs directly interact with biomolecules without relying on enzymes, offering high sensitivity and selectivity (Ji et al., 2020). This makes them suitable for the detection of a wide range of biomolecules, including proteins, DNA (Azam et al., 2021), and heavy metal ions (Huang et al., 2019). The small size and large surface area of CQDs also make them ideal for enhancing the electron transfer rate, which is crucial for biosensing applications (Kumar et al., 2023). This property allows for efficient and rapid detection of biomolecules, contributing to the overall effectiveness of CQDs in biosensing.
CQDs have shown great potential in glucose sensing due to their high biocompatibility and photostability (Chacko et al., 2023). The unique optical properties of CQDs make them attractive for use as fluorescent probes for glucose sensing (Yang et al., 2023). They can be easily integrated into bioelectronic devices for real-time and continuous monitoring of glucose levels in biological fluids (Cortes et al., 2024). Additionally, the tunable surface chemistry of CQDs allows for functionalization with various biomolecules, enabling specific detection of glucose in complex biological samples (Chen et al., 2020). Potential applications of CQDs in glucose sensing include wearable glucose monitoring devices, implantable glucose sensors, and non-invasive glucose sensing methods using CQDs as fluorescent probes (Kim et al., 2024). In recent years, CQDs have been used as materials for detecting glucose, showing promising results. Research studies have demonstrated the use of CQDs for detecting glucose with high sensitivity and selectivity (Lv et al., 2024). For example, a recent study by Huang discussed the specific and selective detection of saccharide species and provided the mechanism of glucose detection using quantum dots along with other materials (Aygun et al., 2024; Huang et al., 2023). The correlation of CQDs and the sugar molecule arises from corrosion reactions, resulting in a modification in glow intensity or electroanalytical signals that deliver a trustworthy method of ascertaining the level of the sugar (Kong et al., 2024). One of the unique features of using CQDs for glucose sensing is their ability to offer enhanced biocompatibility and reduced cytotoxicity compared to other sensing platforms, such as metal-based nanoparticles or organic dyes (Zhu et al., 2012). Combining specific detection parts such as nucleic acid ligands or biological catalysts with CQDs substantially augments their precision and discrimination towards glucose compounds. These improvements make CQDs a promising candidate for next-generation glucose sensors. Furthermore, CQDs can be easily functionalized with specific recognition elements, such as enzymes or aptamers, to further enhance their selectivity and specificity toward glucose molecules (Wang et al., 2014; Lim et al., 2015). Therefore, several CQDs-based electrodes have been employed for glucose detection in various sample matrices.
For example, a glucose sensor has been engineered by immobilizing zinc oxide quantum dots (ZnO QDs) onto fMWCNTs, forming a ZnO QDs/MWCNTs nanocomposite. Transmission electron microscopy (TEM) confirmed the precise monodispersed and anchoring of ZnO QDs on the thiol-functionalized MWCNTs (Figure 6A). The use of this composite architecture to detect glucose concentration from human urine samples exhibited impressive electrocatalytic properties, achieving a high sensitivity of 9.36 μA μM−1, with a detection limit of 0.208 µM and a linear detection range spanning from 0.1 to 2.5 µM (Figure 6B) (Vinoth et al., 2021). The exceptional sensitivity and low detection threshold ascribed the potential of the ZnO QDs/MWCNTs nanocomposite sensor for advanced biomedical diagnostic applications, particularly in the accurate and efficient monitoring of glucose levels in biological specimens. In another study, Khan et al., developed a novel electrode system using in-situ deposition of carbon quantum dots (C-dots) over copper oxide (CuO) (Figure 6C). This innovative electrode demonstrated remarkable analytical performance. The sensor operates effectively within a concentration range of 15–225 nM and exhibits a low limit of detection (LOD) of 1.4 nM (Figure 6D). Additionally, this electrochemical sensing system shows a high sensitivity of 17142.86 μA mM−1 cm−2 (Ullah khan et al., 2024). They showed that this advanced electrode design maintained its performance even after repeated use indicating cyclic stability in the detection of glucose blood serum samples. Buk et al. (2019) developed a novel electrode system biosensor, engineered utilizing a composite of CQDs and gold nanoparticles (AuNPs) conjugated with glucose oxidase (GOx) as described in Figure 6E. This biosensor’s analytical prowess was meticulously assessed through chronoamperometry. The CQDs/AuNPs-GOx biosensor exhibited remarkable sensitivity, registering at 47.24 μA mM−1 cm−2, and demonstrated a detection limit of 17 µM. It provided a linear response to glucose concentrations within the range of 0.05–2.85 mM (Figure 6F), with high accuracy and reliability, reproducibility, and reusability. Importantly, the biosensor also showcased the feasibility of precisely detecting glucose levels in real human serum in the presence of the interfering species (ascorbic acid, uric acid, and acetaminophen) indicating significant potential for clinical diagnostic applications.
Figure 6. (A) TEM image of MWCNT/ZnO QDs including EDS spectra of the materials, (B) amperometric response as a function of glucose concentration (Vinoth et al., 2021); (C) preparation of CuO/CQD and their application, (D) DPV response of glucose concentrations, and its calibration graph (Ullah khan et al., 2024); (E) the chronoamperometric response of the CQD/AuNP-GOx biosensor, and (F) the corresponding calibration curve (Buk et al., 2019).
Graphene-based electrode materials represent a revolutionary approach for sensing applications, attributed to their distinct and superior properties (Li et al., 2024). Graphene, a monolayer of carbon atoms configured in a two-dimensional honeycomb structure, offers an exceptionally large surface area, enhancing the interaction with glucose molecules and thereby increasing the sensor’s sensitivity (Huang et al., 2015). Its outstanding electrical conductivity enables efficient electron transfer during the electrochemical oxidation of glucose, producing strong and reliable signals essential for precise glucose measurement (Goodrum et al., 2024). Glucose biosensing mechanisms are broadly categorized into enzymatic and non-enzymatic approaches, both hinging on the electrooxidation reactions of glucose molecules (Mohamad Nor et al., 2022). Enzymatic sensors detect glucose indirectly, while non-enzymatic sensors, which utilize nanomaterials with increased specific surface areas and electrochemically active sites, achieve direct detection. The fundamental principle for these sensors lies in the oxidation/reduction reactions of glucose (He et al., 2024). Interestingly, higher sensitivity and biocompatibility have driven the innovation of novel graphene-based nanomaterials as electrode materials for glucose sensing. Since its first characterization in 2004 (Shahriary and Athawale, 2014), produced through the mechanical exfoliation of highly oriented pyrolytic graphite, graphene has garnered significant attention due to its exceptional chemical and physical properties. Its two-dimensional monolayer structure offers a high specific surface area (∼2,600 m2/g), which enhances its ability to bind target analytes of glucose, thereby improving sensitivity and lowering detection limits (Petit and Bandosz, 2009). Despite being only one carbon atom thick, graphene exhibits remarkable mechanical strength, with a Young’s modulus of ∼1.1 TPa and a tensile strength of 130 GPa, making it resistant to mechanical stress (Lee et al., 1979). Apart from pure graphene, reduced graphene oxide (rGO), a derivative of graphene, maintains flexibility even after 5,000 bending cycles, with no significant increase in resistance proving its robustness in wearable devices including glucose biosensors (Sandeep et al., 2024).
Enhanced electron transfer between the electrode and the target molecule is crucial for the performance of electrochemical biosensors (Fu et al., 2021). Fortunately, graphene’s sp2-hybridized structure that forms σ-bonds with a trigonal planar geometry, allows high charge carrier mobility, translating to high electrical conductivity (Wu et al., 2013). Experimental measurements and theoretical studies have reported electron mobility ranging from 10³ to 10⁶ cm2/(V·s) and electrical conductivity from 102 to 10⁶ S/m, demonstrating graphene’s promise for glucose sensing (Bolotin, 2014). Nanoporous graphene, with its exceptional transport properties, is also used for detecting single molecules, including various carbohydrates (Kammarchedu et al., 2024). Graphene and graphene oxide improve electrochemiluminescence (ECL) sensor efficiency by providing numerous sites for luminophores and increased binding sites for target analytes (Qi and Zhang, 2020). A sensitive ECL glucose sensor using graphene quantum dots (GQDs) prepared from GO, with hydrogen peroxide as the only reagent, has shown excellent sensitivity with detection limits as low as pmol·L−1 (Yang et al., 2020). In general, enzymatic glucose sensors rely on direct or mediated electron transfer between the active sites of the enzymes and the electrode surface. By increasing enzyme concentration and accelerating direct electron transfer (DET), the sensitivity of these sensors can be significantly enhanced (Naseri et al., 2018). Graphene materials, with their high charge mobility, electrical conductivity, and specific surface area, have been reported to improve the efficiency of these sensors (Ji et al., 2024). Further, the strong electrostatic interaction of nanostructured graphene with enzymes enhances contact at the enzyme-electrode interface (Ji et al., 2024). Functionalizing the electrode surface with appropriate groups facilitates enzyme immobilization, improving interaction with substrates and enhancing sensor performance (Fenoy et al., 2022). The presence of oxygenated functional groups in graphene provides additional reaction sites for further functionalization, accelerating enzyme immobilization on the biosensor’s electrode surface (Ferlazzo et al., 2024). Interestingly, GO electrodes, with their high surface area and oxygen-rich functional groups, enhance glucose sensing by providing numerous active sites for glucose oxidation and improving enzyme immobilization. The electrocatalytic properties of GO facilitate efficient electron transfer during glucose oxidation, whether in enzyme-based or non-enzymatic sensors. These features make GO a highly effective material for enhancing the sensitivity and performance of glucose biosensors (Figure 7) (Chakrovorty et al., 2021).
Figure 7. Illustration of the structure and mechanism of graphene oxide-based non-enzymatic biosensor for blood glucose detection (Chakrovorty et al., 2021).
Functionalization of graphene with enzymes like glucose oxidase results in catalytic oxidation of glucose, resulting in the production of hydrogen peroxide, detectable through electrochemical means (Durgalakshmi et al., 2019). This functionalization significantly boosts the specificity and sensitivity of glucose sensors. Moreover, non-enzymatic sensors that utilize graphene’s electrocatalytic properties can directly oxidize glucose, obviating the need for enzymes and thereby enhancing stability and extending the sensor’s lifespan (Zhou et al., 2024; Sakr et al., 2020). Graphene’s biocompatibility further positions it as an ideal material for in vivo applications, including continuous glucose monitoring systems (Li et al., 2012). Its integration into wearable and implantable devices has led to the development of advanced, non-invasive glucose monitoring technologies. The combination of high sensitivity, rapid response time, and robustness positions graphene-based electrodes at the cutting edge of glucose detection technology, offering an efficient solution for diabetes management. Wearable electrochemical glucose sensor, fabricated by the screen-printing technology, in sweat samples, reported by Zheng et al. (2021). It performed well with linear the range of 0.05–1 mM, a sensitivity of 105.93 μA mM−1 cm−2 demonstrated for constant 9 h of monitoring. Due to these attractive properties, graphene-based electrode materials have been utilized for glucose sensing. For example, Awada et al. (2023) have reported on the synthesis and preparation of 4-NTP functionalized heterogeneous layers of gold/graphene/Cu toward electrochemical sensing of glucose. Interestingly, the developed electrode material ascribed a moderate sensitivity, (179.6 μA mM−1 cm−2) with a limit of detection of 0.5 mM within a concentration range of 1–20 mM. This might be due to the attached 4-nitrophenol into the gold/graphene/cu composite electrode.
Advanced electrode material NiO–Co3O4/rGO has shown exceptional performance in glucose detection within serum samples, as reported by Mustafa et al. (2022). Utilizing amperometry for sensing, this sensor achieves a wide linear range from 1.23 × 10−⁴ mM to 10 mM, with an outstanding sensitivity of 345 μA mM−1 cm−2 and an impressive limit of detection of 0.13 μM. The interference study, conducted with 100 µM concentrations of fructose (FR), lactose (LA), uric acid (UA), and ascorbic acid (AA), further validates its accuracy and reliability. This cutting-edge technology demonstrates significant potential for precise glucose monitoring in clinical applications. In another study, the ZIF-67@GO/Co(OH)2 electrode material, as reported by Tao et al. (2023) featuring in an extensive concentration range from 0.001 to 8.546 mM, ensuring its applicability across diverse testing scenarios (Figures 8A, B). The electrode demonstrates exceptional sensitivity at 2412.7 μA mM−1 cm−2, allowing for highly precise glucose measurements, even at low concentrations. Its detection limit is impressively low at 0.934 μM. The ZIF-67@GO/Co(OH)2 electrode also excels in selectivity, effectively distinguishing glucose from common interfering substances such as DA, AA, UA, fructose, sucrose, lactose, and maltose, even when these intrusive substances are present in large concentrations as depicted in Figures 8C–F. This robustness is further highlighted by its consistent accuracy in complex sample environments, where it successfully retains its performance following the successive addition of these interfering agents to a glucose solution. These attributes underscore the superior capabilities of the ZIF-67@GO/Co(OH)2 electrode material in glucose detection applications.
Figure 8. ZIF-67@GO/Co(OH)2 electrode for (A) glucose detection, and (B) its calibration graph; (C) glucose LSV measurements for samples with different proportions of MWCNTs, (D) 0 wt%, (E) 15 wt%, (E) 30 wt%, and (F) 60 wt% (Liao et al., 2023).
Latiful et al., have developed a chronoamperometric glucose sensor using ZnO-graphene (G)-SnO2 ternary nanocomposite electrode materials, achieving a notable sensitivity for the detection of glucose concentration as shown in Figures 9A, B. The sensor utilizes ZnO-G-SnO2 nanocomposites for glucose sensing via cyclic voltammetry. These nanocomposites were synthesized using a self-assembly method and are deposited on nickel foam electrodes. The sensor operates effectively at low current densities (0.0010 mA/cm2) and demonstrates capability in detecting glucose concentrations as precise as 0.5 mmol L−1 across a potential range from −1.0 to 1.0 V (Kabir et al., 2024). Similarly, a highly stable glucose sensor based on graphene on annealed copper was reported by Luo et al. (2024) with two linear dynamic ranges of 0–1.39 mM and 2.78–11.11 mM, with a sensitivity of 97 μA mM−1 and 3 μA mM−1, respectively. Furthermore, they reported that the as-prepared sensor was reliable in detecting glucose in blood samples showing resistance to interfering substances such as NaCl, urea, and lactic acid. Gijare et al. (2024) reported rGO-based glucose sensing device which achieved a sensitivity of 19.17 μA cm−2mM−1 with a low detection limit of 1.9 µM, and a linear range of 0.2–10 mM synthesized using cow urine as a reducing agent, (Figures 9C, D) (Gijare et al., 2024).
Figure 9. (A) preparation of ZnO-G-SnO2 electrode, (B) glucose sensing mechanism (Kabir et al., 2024); (C) preparation of rGO, and (D) LSV of rGO-10 based on glucose concentrations and its calibration curve (Gijare et al., 2024).
In another study, they used cow urine for the removal of oxygen-containing groups in GO, confirmed through various analyses (UV Vis, Fourier transform infrared, and X-ray diffraction). Their findings showed that the variation in current response values (Figures 9C, D) towards glucose in human serum was insignificant even after five repetitions of testing. In a relevant study by Nugba et al. (2023), an amperometric glucose sensor was developed which was prepared through an electrodeposition approach. It demonstrated glucose detection in saliva samples, achieving a detection limit of 0.023 µM and a wide linear range of 0.03–4.5 mM. This amperometric glucose sensor showed a detection accuracy of 97% which is in close agreement with measurements taken from blood glucose. Further, they showed that their design shows good selectivity towards glucose in the presence of interfering species such as ascorbic acid and urea with a sensitivity of 2665 µA mM−1 cm−2.
The analytical performance of the carbon nanomaterials of the scope of this review is summarized in Table 1. These CNT, CQD, and graphene-based nanomaterial electrodes are applied. It was observed from the vast quantity of literature that the synergistic incorporation of these materials benefits optimizing the performance, cost, and user-friendliness of glucose detection through a non-enzymatic and enzymatic sensing approach.
Table 1. Examples of carbon nanomaterial-based electrodes for the detection of glucose through a non-enzymatic/enzymatic sensing approach.
Other types of carbon such as mesoporous carbons provide high surface areas and efficient mass transport, while carbon nanofibers offer excellent conductivity and improved electron transfer. Together, they enable better sensitivity and stability in detection of biological and chemical analytes. This review focuses on recent advancements in these carbon-based biosensors, including their fabrication methods and applications (Iftikhar et al., 2023; Tang et al., 2017).
This carbon nanofibers have been used as modifiers during fabrication of highly specific and stable glucose biosensor characterized with high flexibility. The CNF modified flexible electrode platform consisted a novel conjugated polymer, P-BDT-BTz, which includes benzotriazole, benzodithiophene, and benzenediamine moieties. The fabrication process involved electrospinning polyacrylonitrile (PAN) nanofiber mats, which were then stabilized and carbonized to form CNFs. These nanofibers were applied onto a flexible polyethylene terephthalate (PET) substrate. The synthesized and characterized conjugated polymer, P-BDT-BTz, was utilized as a surface modifier for the CNF-coated transducer to enhance glucose detection. The biosensor’s performance was optimized by evaluating the influence of different parameters, resulting in a linear glucose detection range from 20 µM to 500 μM, with a detection limit of 8.5 µM. Additionally, the biosensor exhibited strong glucose-sensing capabilities in beverage samples. Overall, this amperometric glucose oxidase (GOx)--based biosensor demonstrates excellent specificity, a low detection limit, and a broad linear detection range, highlighting its potential as a reliable tool for glucose monitoring (Bulut et al., 2023).
While many glucose sensors are currently available, there remains significant interest in developing non-enzymatic devices for glucose detection across various applications. This study presents an innovative enzyme-free electrochemical glucose sensor based on carbon nanofibers (CNFs) functionalized with an aromatic diamine through a simple wet chemistry approach. The electrochemical properties of the modified carbon-based screen-printed electrodes (SPCEs) were evaluated using electrical impedance spectroscopy (EIS), showcasing excellent selectivity for glucose over competing sugars such as fructose and sucrose. The sensor exhibited two distinct linear response ranges: one at lower glucose concentrations (0–1,200 μM) and another from 1,200 to 5,000 μM, with a limit of detection (LOD) calculated at 18.64 μM. The study underscores the sensor’s precise and reliable glucose detection, positioning this novel non-enzymatic electrochemical sensor as a promising tool for selective glucose sensing in diverse applications (Ferlazzo et al., 2024).
The demand for electrochemical sensors is growing for real-time health monitoring, particularly in measuring temperature, heart rate, and blood glucose levels. This study introduces a fiber-like microelectrode composed of copper oxide-modified carbon nanotubes (CuO@CNTFs), designed as a flexible and wearable glucose sensor with exceptional catalytic activity. The one-dimensional structure of CNT fibers enhances conductivity and mechanical strength, making them superior to alternative fibrous materials. Employing a binder-free electrodeposition method, CuO nanoparticles were successfully deposited on the CNT fibers, followed by thermal treatment to improve sensor performance.
Characterization techniques, including scanning electron microscopy and energy-dispersive X-ray analysis, confirmed the effective integration of CuO nanoparticles. The CuO@CNTF microelectrodes demonstrated impressive electrochemical properties, with a sensitivity of approximately 3,000 μA mM−1 cm−2, a detection limit of 1.4 μM, and a broad linear detection range of up to 13 mM. The microelectrode’s superior performance is attributed to the synergistic effects of CuO’s electrocatalytic activity and the high conductivity of CNT fibers. Additionally, electrochemical impedance spectroscopy revealed a low charge transfer resistance, further validating the electrode’s efficiency. This research offers a promising strategy for creating flexible and disposable non-enzymatic glucose sensors through CNT fiber-based microelectrodes (Muqaddas et al., 2023).
Non-enzymatic glucose sensors are essential for detecting glucose in complex biological systems. This study presents a streamlined method to create highly stable and sensitive glucose sensors by depositing cobalt phthalocyanine (CoPc) onto mesoporous carbon screen-printed electrodes (MCs). The use of electrochemical activation (aMC) followed by phase inversion (PI) significantly enhanced the sensor’s performance. Among the tested configurations, the aMC-CoPc/PI electrode showed the highest electrocatalytic activity, with a sensitivity of 22.3 μA mM−1, a detection limit of 27.4 μM, and a linear range from 0.1 to 3.5 mM. The sensor also demonstrated excellent selectivity, stability, and reproducibility in complex samples such as horse serum and cell culture media, highlighting its potential for practical glucose detection in real-world applications (González-Sánchez et al., 2024).
Accurate monitoring of blood glucose levels is essential for the effective diagnosis and management of diabetes. While traditional methods rely on enzymatic and non-enzymatic electrochemical biosensors for glucose quantification in serum or plasma, recent research has shifted towards non-invasive detection methods in sweat, aiming to improve sensitivity, accuracy, and stability. This study presents an innovative approach using nanostructured mesoporous carbon integrated with glucose oxidase (GOx) to enhance direct electron transfer to the electrode surface. A CuNi alloy nanoparticle-coated mesoporous carbon (CuNi-MC) catalyst was synthesized through a hydrothermal process, followed by annealing at 700°C under argon gas. The structural and morphological properties of the catalyst were thoroughly analyzed using X-ray diffraction and high-resolution transmission electron microscopy. Electrochemical assessments, including cyclic voltammetry (CV) and amperometry, revealed outstanding electrocatalytic activity, with a rapid response time of 4 s and a broad linear detection range from 0.005 to 0.45 mM. Furthermore, the electrode exhibited high sensitivity at 11.7 ± 0.061 mA mM−1 cm−2 in selective media. This study underscores the potential of CuNi-MC catalysts for developing highly sensitive, non-invasive glucose biosensors with rapid response times, making significant advancements in glucose monitoring technology (Radwan et al., 2021).
Overall, among carbon-based nanomaterials, graphene oxide (GO) stands out for its excellent biocompatibility and practical applicability in glucose electrochemical sensing. Its oxygen-containing functional groups improve dispersibility in biological environments, reducing cytotoxicity compared to pure graphene, carbon quantum dots, and carbon nanotubes. These functional groups also allow easy attachment of glucose oxidase and other biomolecules, enhancing sensor performance. GO’s high surface area boosts sensitivity, while its stable and simple fabrication makes it ideal for practical applications. GO-based sensors have been widely researched for real-time glucose monitoring, offering a promising solution for wearable and flexible biosensors in medical diagnostics.
In this review, the recent advances in carbon nanostructured-based biosensors have been discussed, including carbon quantum dots, carbon nanotubes, graphene, mesoporous carbons, and carbon nanofibers for glucose detection. Each of these nanostructured materials offers unique benefits and challenges related to their synthesis, functionalization, cost-effectiveness, and stability. Recent developments in these materials have shown significant potential for enhancing glucose sensor performance through improved sensitivity, selectivity, and lower detection limits. The exceptional properties and numerous advantages of carbon nanostructured materials, such as high surface area, excellent electrical conductivity, mechanical strength, and chemical stability, make them highly valuable in biosensing applications. The flexibility and versatility of these materials allow for their integration into various sensor designs, further enhancing their applicability and performance. Therefore, advancing carbon nanostructured-based biosensors is essential to replace conventional glucose detection methods. Continued research is necessary to develop environmentally friendly, stable, highly selective, and sensitive materials. The application of carbon nanostructured materials in glucose sensors presents a holistic approach to addressing the global challenge of diabetes management. However, most research has focused on fabricating these sensors and evaluating their analytical performance in controlled environments. To move towards commercialization, it is crucial to test these sensors in real-world conditions, which involve complex biological matrices. Additionally, the long-term stability and environmental impact of carbon nanostructured materials need to be thoroughly assessed before considering large-scale production. The selected carbon nanostructures, each with unique features such as high surface-to-volume ratio (carbon quantum dots), exceptional electrical conductivity (carbon nanotubes), and outstanding mechanical and chemical properties (graphene), have been extensively applied in glucose sensing. These materials, whether used individually or in combination, are expected to drive a significant shift from traditional glucose detection methods to more advanced, flexible, and low-cost biosensors. Carbon nanostructured-based biosensors, through suitable functionalization or modification, have greatly enhanced the sensitivity, selectivity, and detection limits for glucose. However, challenges remain, such as ensuring stability, optimizing functionalization processes, and integrating these materials into practical sensor designs. To fully harness the potential of carbon nanostructured-based biosensors, interdisciplinary research efforts should focus on developing scalable, eco-friendly synthesis methods, and improving sensor functionalization for real-world application. Researchers should also prioritize extensive in vivo testing to validate performance in more complex environments. Through these efforts, the field can move closer to realizing commercially viable, high-performance glucose sensors capable of revolutionizing diabetes management. Additionally, scaling up production while ensuring consistent quality and minimizing environmental impact and toxicity study presents a significant challenge. Progress in functionalization methods and sensor integration will be vital in addressing these issues, ensuring that glucose sensors based on carbon nanostructures remain not only highly sensitive and selective but also stable, reproducible, and cost-effective. Furthermore, fostering interdisciplinary collaborations and driving ongoing innovation will be crucial to overcoming these challenges, paving the way for the commercialization of advanced glucose sensors that could transform diabetes management. Despite these challenges, carbon quantum dots, carbon nanotubes, and graphene show immense potential for developing highly efficient glucose sensors. Moreover, this review may open new avenues for advancing the application of carbon nanostructured materials in glucose detection, paving the way for innovative and practical biosensing solutions.
AA: Writing–review and editing, Writing–original draft, Methodology, Data curation, Conceptualization. MM: Writing–review and editing, Writing–original draft, Data curation. YZ: Visualization, Writing–review and editing, Writing–original draft, Data curation. WM: Writing–review and editing, Writing–original draft, Methodology, Data curation. JF: Methodology, Conceptualization, Writing–review and editing, Writing–original draft. TM: Writing–review and editing, Methodology, Project administration, Supervision, Validation. BN: Writing–review and editing, Writing–original draft, Methodology, Conceptualization.
The author(s) declare that no financial support was received for the research, authorship, and/or publication of this article.
The authors express their gratitude to the Bio and Emerging Technology Institute (BETin), Addis Ababa, Ethiopia.
The authors declare that the research was conducted in the absence of any commercial or financial relationships that could be construed as a potential conflict of interest.
All claims expressed in this article are solely those of the authors and do not necessarily represent those of their affiliated organizations, or those of the publisher, the editors and the reviewers. Any product that may be evaluated in this article, or claim that may be made by its manufacturer, is not guaranteed or endorsed by the publisher.
Ambaye, A. D., Fito, J., Nxumalo, E., and Nkambule, T. T. I. (2023b). The challenges of micropollutants and their environmental detection through biochar-based nanostructured electrochemical sensors: a review. Int. J. Environ. Sci. Technol. 20, 11741–11762. doi:10.1007/s13762-023-04896-8
Ambaye, A. D., Kebede, T. G., Ntsendwana, B., and Nxumalo, E. N. (2023a). Fe-MOF derived graphitic carbon nitride nanocomposites as novel electrode materials for the electrochemical sensing of 2, 4-dichlorophenol in wastewater. Synth. Met. 299, 117452. doi:10.1016/j.synthmet.2023.117452
Ambaye, A. D., Kefeni, K. K., Kebede, T. G., Ntsendwana, B., Mishra, S. B., and Nxumalo, E. N. (2022). Cu-MOF/N-doped GO nanocomposites modified screen-printed carbon electrode towards detection of 4-nitrophenol. J. Electroanal. Chem. 919, 116542. doi:10.1016/j.jelechem.2022.116542
Ambaye, A. D., Kefeni, K. K., Mishra, S. B., Nxumalo, E. N., and Ntsendwana, B. (2021). Recent developments in nanotechnology-based printing electrode systems for electrochemical sensors. Talanta 225, 121951. doi:10.1016/j.talanta.2020.121951
Ambaye, A. D., Muchindu, M., Jijana, A., Mishra, S., and Nxumalo, E. (2023c). Screen-printed electrode system based on carbon black/copper-organic framework hybrid nanocomposites for the electrochemical detection of nitrite. Mater Today Commun. 35, 105567. doi:10.1016/j.mtcomm.2023.105567
Arias-Rivera, S., Raurell-Torredà, M., Fernández-Castillo, R. J., Campos-Asensio, C., Thuissard-Vasallo, I. J., Andreu-Vázquez, C., et al. (2024). Blood glucose monitoring in critically ill adult patients: type of sample and method of analysis. Systematic review and meta-analysis. Enfermería Intensiva (Engl. ed.) 35 (1), 45–72. doi:10.1016/j.enfie.2023.02.002
Awada, C., Hammud, H. H., Bukhamsin, H. A., Al-Hudairi, D. E., and Ruffino, F. (2023). Development of ultrasensitive new sensors based on thio-functionalized heterogeneous layers of gold/graphene/copper for glucose detection. Appl. Surf. Sci. 638, 158012. doi:10.1016/j.apsusc.2023.158012
Aygun, A., Cobas, I., Tiri, R. N. E., and Sen, F. (2024). Hydrothermal synthesis of B, S, and N-doped carbon quantum dots for colorimetric sensing of heavy metal ions. RSC Adv. 14 (16), 10814–10825. doi:10.1039/d4ra00397g
Azam, N., Najabat Ali, M., and Javaid Khan, T. (2021). Carbon quantum dots for biomedical applications: review and analysis. Front. Mater 8. doi:10.3389/fmats.2021.700403
Biswas, P., Polash, S. A., Dey, D., Kaium, M. A., Mahmud, A. R., Yasmin, F., et al. (2023). Advanced implications of nanotechnology in disease control and environmental perspectives. Biomed. and Pharmacother. 158, 114172. doi:10.1016/j.biopha.2022.114172
Bolotin, K. I. (2014). Electronic transport in graphene: towards high mobility. Graphene, 199–227. doi:10.1533/9780857099334.3.199
Bruen, D., Delaney, C., Florea, L., and Diamond, D. (2017). Glucose sensing for diabetes monitoring: recent developments. Sensors 17 (8), 1866. doi:10.3390/s17081866
Buk, V., and Pemble, M. E. (2019). A highly sensitive glucose biosensor based on a microdisk array electrode design modified with carbon quantum dots and gold nanoparticles. Electrochim Acta 298, 97–105. doi:10.1016/j.electacta.2018.12.068
Buk, V., Pemble, M. E., and Twomey, K. (2019). Fabrication and evaluation of a carbon quantum dot/gold nanoparticle nanohybrid material integrated onto planar micro gold electrodes for potential bioelectrochemical sensing applications. Electrochim Acta 293, 307–317. doi:10.1016/j.electacta.2018.10.038
Bulut, U., Oyku Sayin, V., Altin, Y., Can Cevher, S., Cirpan, A., Celik Bedeloglu, A., et al. (2023). A flexible carbon nanofiber and conjugated polymer-based electrode for glucose sensing. Microchem. J. 184, 108148. doi:10.1016/j.microc.2022.108148
Chacko, A. R., Narayanan, R., Korah, B. K., Mathew, S., Abraham, T., and Mathew, B. (2023). Solution-based carbon quantum dots as electrochemical ‘on–off’ switch in aqueous medium: engineering of a sustainable green sensor and its computational investigation. Microchem. J. 191, 108853. doi:10.1016/j.microc.2023.108853
Chakrovorty, A., Bhattacharjee, B., Dey, R., Samadder, A., and Nandi, S. (2021). Graphene: the magic carbon derived biological weapon for human welfare. Int. Acad. Publ. House (IAPH), 9–17. doi:10.52756/ijerr.2021.v25.002
Chen, L., Dotzert, M., Melling, C. W. J., and Zhang, J. (2020). Tunable photoluminescence of carbon dots used for homogeneous glucose sensing assay. Biochem. Eng. J. 159, 107580. doi:10.1016/j.bej.2020.107580
Choi, Y. B., Kim, H. S., Jeon, W. Y., Lee, B. H., Shin, U. S., and Kim, H. H. (2019). The electrochemical glucose sensing based on the chitosan-carbon nanotube hybrid. Biochem. Eng. J. 144, 227–234. doi:10.1016/j.bej.2018.10.021
Clark, L. C., and Lyons, C. (1962). Electrode systems for continuous monitoring in cardiovascular surgery. Ann. N. Y. Acad. Sci. 102 (1), 29–45. doi:10.1111/j.1749-6632.1962.tb13623.x
Cortes, F. R. U., Falomir, E., Lancis, J., and Mínguez-Vega, G. (2024). Pulsed laser fragmentation synthesis of carbon quantum dots (CQDs) as fluorescent probes in non-enzymatic glucose detection. Appl. Surf. Sci. 665, 160326. doi:10.1016/j.apsusc.2024.160326
Demeke, A., Chekol, F., Mehretie, S., and Admassie, S. (2017). Simultaneous determination of pyridine-2-aldoxime methochloride and dopamine at poly(3,4-ethylenedioxythiophene) modified glassy carbon electrode. Anal. and Bioanal. Electrochem. 9 (6), 704–717.
Dickson, L. M., Buchmann, E. J., Van Rensburg, C. J., and Norris, S. A. (2019). The impact of differences in plasma glucose between glucose oxidase and hexokinase methods on estimated gestational diabetes mellitus prevalence. Sci. Rep. 9, 7238. doi:10.1038/s41598-019-43665-x
Dua, A., Debnath, A., Kumar, K., Mazumder, R., Mazumder, A., Singh, R., et al. (2024). Advancements of glucose monitoring biosensor: current state, generations of technological progress, and innovation dynamics. Curr. Pharm. Biotechnol. 25. doi:10.2174/0113892010305386240625072535
Dua, S., Kumar, P., Pani, B., Kaur, A., Khanna, M., and Bhatt, G. (2023). Stability of carbon quantum dots: a critical review. RSC Adv. 13 (20), 13845–13861. doi:10.1039/d2ra07180k
Durgalakshmi, D., Rakkesh, R. A., and Mohanraj, J. (2019). Graphene – metal – organic framework-modified electrochemical sensors. Graphene-Based Electrochem. Sensors Biomol., 275–296. doi:10.1016/B978-0-12-815394-9.00011-X
Fall, B., Karim, A., Diaw, D., Fall, M., Randriamahazaka, H., and Thomas, S. (2022). Highly efficient non-enzymatic electrochemical glucose sensor based on carbon nanotubes functionalized by molybdenum disulfide and decorated with nickel nanoparticles (GCE/CNT/MoS2/NiNPs). Sensors Actuators Rep. J. 5, 100136. doi:10.1016/j.snr.2022.100136
Fenoy, G. E., Marmisollé, W. A., Knoll, W., and Azzaroni, O. (2022). Highly sensitive urine glucose detection with graphene field-effect transistors functionalized with electropolymerized nanofilms. Sensors and Diagnostics 1 (1), 139–148. doi:10.1039/D1SD00007A
Ferlazzo, A., Celesti, C., Iannazzo, D., Ampelli, C., Giusi, D., Costantino, V., et al. (2024). Functionalization of carbon nanofibers with an aromatic diamine: toward a simple electrochemical-based sensing platform for the selective sensing of glucose. ACS Omega 9 (25), 27085–27092. doi:10.1021/acsomega.4c00525
Fu, K., Seo, J. W., Kesler, V., Maganzini, N., Wilson, B. D., Eisenstein, M., et al. (2021). Accelerated electron transfer in nanostructured electrodes improves the sensitivity of electrochemical biosensors. Advance Science. doi:10.1101/2021.04.13.439686
Gao, F., Hong, W., Xu, B., Zhao, Z., Wang, Y., Lu, L., et al. (2023). Tin dioxide quantum dots-modified sensing electrode for selective detection of luteolin. Microchem. J. 193, 109244. doi:10.1016/j.microc.2023.109244
Gezahegn, T. F., Ambaye, A. D., Mekoyete, T. M., Malefane, M. E., Oyedotun, K. O., and Mokrani, T. (2024). Breakthroughs in nanostructured-based chemical sensors for the detection of toxic metals. Talanta Open 10, 100354. doi:10.1016/j.talo.2024.100354
Gijare, M. S., Chaudhari, S. R., Ekar, S., Shaikh, S. F., Al-Enizi, A. M., Pandit, B., et al. (2024). Green synthesis of reduced graphene oxide (rGO) and its applications in non-enzymatic electrochemical glucose sensors. J. Photochem Photobiol. A Chem. 450, 115434. doi:10.1016/j.jphotochem.2023.115434
González-Sánchez, M. I., Khadhraoui, H., Jiménez-Pérez, R., Iniesta, J., and Valero, E. (2024). Non-enzymatic glucose sensor using mesoporous carbon screen-printed electrodes modified with cobalt phthalocyanine by phase inversion. Microchem. J. 200, 110314. doi:10.1016/j.microc.2024.110314
Goodrum, R., Weldekidan, H., Li, H., Mohanty, A. K., and Misra, M. (2024). Graphene-based nanostructures from green processes and their applications in biomedical sensors. Adv. Industrial Eng. Polym. Res. 7 (1), 37–53. doi:10.1016/j.aiepr.2023.03.001
Govindaraj, M., Srivastava, A., Muthukumaran, M. K., Tsai, P. C., Lin, Y. C., Raja, B. K., et al. (2023). Current advancements and prospects of enzymatic and non-enzymatic electrochemical glucose sensors. Int. J. Biol. Macromol. 253, 126680. doi:10.1016/j.ijbiomac.2023.126680
Gupta, S., and Tai, N.-H. (2023). Carbon nanomaterials and their composites for electrochemical glucose biosensors: a review on fabrication and sensing properties. J. Taiwan Inst. Chem. Eng. 154, 104957. doi:10.1016/j.jtice.2023.104957
Harun-Or-Rashid, M., Aktar, M. N., Preda, V., and Nasiri, N. (2024). Advances in electrochemical sensors for real-time glucose monitoring. Sensors and Diagnostics 3, 893–913. doi:10.1039/d4sd00086b
He, S., Ma, Y., Maulik, G., Jellicoe, M., Nag, A., Powell, W., et al. (2024). A review on graphene-based sensors for tactile applications. Sens. Actuators A Phys. 372, 115363. doi:10.1016/j.sna.2024.115363
Hegde, V., Bhat, M. P., Lee, J.-H., Kurkuri, M. D., Kim, C. S., and Lee, K.-H. (2024). Carbon-based nanomaterials: Multifaceted role in agrochemical recognition, remediation, and release. Nano Today 57, 102388. doi:10.1016/j.nantod.2024.102388
Hicks, G. P., and Updike, S. J. (1966). The preparation and characterization of lyophilized polyacrylamide enzyme gels for chemical Analysis. Anal. Chem. 38, 726–730. doi:10.1021/ac60238a014
Hossain, Md. J., Al-Mamun, Md., and Islam, Md. R. (2024). Diabetes mellitus, the fastest growing global public health concern: early detection should be focused. Health Sci. Rep. 7, e2004. doi:10.1002/hsr2.2004
Huang, B., Wang, Q., Li, Y., Zhang, M., and Wei, X. (2015). Preparation and characterisation of graphene. Mater. Res. Innovations 19, S9-344–S9-350. doi:10.1179/1432891715Z.0000000002010
Huang, C.-C., Hung, Y.-S., Weng, Y.-M., Chen, W., and Lai, Y.-S. (2019). Sustainable development of carbon nanodots technology: natural products as a carbon source and applications to food safety. Trends Food Sci. Technol. 86, 144–152. doi:10.1016/j.tifs.2019.02.016
Huang, X., Han, Y., Li, J., Tang, M., and Qing, G. (2023). Sensitive and specific detection of saccharide species based on fluorescence: update from 2016. Anal. Bioanal. Chem. 415 (18), 4061–4077. doi:10.1007/s00216-023-04703-w
Huo, Y., Li, R., Xiu, S., Wang, Y., Zhang, L., Jin, A., et al. (2023). A high–performance non–enzymatic glucose sensor based Au and ZrO2 nanoparticles supported on multi-walled carbon nanotubes. Diam. Relat. Mater 140, 110455–110458. doi:10.1016/j.diamond.2023.110455
Iftikhar, F. J., Shah, A., Wali, Q., and Kokab, T. (2023). Advancements in nanofiber-based electrochemical biosensors for diagnostic applications. Biosens. (Basel) 13, 416. doi:10.3390/bios13040416
Ji, C., Zhou, Y., Leblanc, R. M., and Peng, Z. (2020). Recent developments of carbon dots in biosensing: a Review. ACS Sens. 5 (9), 2724–2741. doi:10.1021/acssensors.0c01556
Ji, X., Jin, H., Qian, W., Wang, Z., Zhang, Z., Yang, Z., et al. (2024). Flexible, scalable hierarchical graphene foam decorated with nickel layer for highly sensitive enzyme-free glucose sensing. J. Alloys Compd. 1004, 175902. doi:10.1016/j.jallcom.2024.175902
Juska, V. B., and Pemble, M. E. (2020). A critical review of electrochemical glucose sensing: evolution of biosensor platforms based on advanced nanosystems. Sensors 20, 6013. doi:10.3390/s20216013
Kabir, L., Joo, Y. J., Cho, K. Y., and Oh, W. C. (2024). Electrochemical approach for nonenzymatic glucose sensing with noble metal-free 2D graphene-based ternary nanocomposite. Sens. Actuators A Phys. 372, 115367. doi:10.1016/j.sna.2024.115367
Kakoti, B. B., Alom, S., Deka, K., and Halder, R. K. (2024). AMPK pathway: an emerging target to control diabetes mellitus and its related complications. J. Diabetes Metab. Disord. 23, 441–459. doi:10.1007/s40200-024-01420-8
Kammarchedu, V., Asgharian, H., Zhou, K., Khamsi, P. S., and Ebrahimi, A. (2024). Recent advances in graphene-based electroanalytical devices for healthcare applications. Nanoscale 16, 12857–12882. doi:10.1039/d3nr06137j
Kim, T. Y., De, R., Choi, I., Kim, H., and Hahn, S. K. (2024). Multifunctional nanomaterials for smart wearable diabetic healthcare devices. Biomaterials 310, 122630. doi:10.1016/j.biomaterials.2024.122630
Kong, J., Wei, Y., Zhou, F., Shi, L., Zhao, S., Wan, M., et al. (2024). Carbon quantum dots: properties, preparation, and applications. Molecules 29 (9), 2002. doi:10.3390/molecules29092002
Kumar, S., Wang, Z., Zhang, W., Liu, X., Li, M., Li, G., et al. (2023). Optically active nanomaterials and its biosensing applications—a Review. Biosens. (Basel) 13 (1), 85–38. doi:10.3390/bios13010085
Lee, C., Wei, X., Kysar, J. W., and Hone, J. (1979). Measurement of the elastic properties and intrinsic strength of monolayer graphene. Science 321 (5887), 385–388. doi:10.1126/science.1157996
Lee, S. M., Kim, H., Li, P., and Park, H. G. (2024). A label-free and washing-free method to detect biological thiols on a personal glucose meter utilizing glucose oxidase-mimicking activity of gold nanoparticles. Biosens. Bioelectron. 250, 116019. doi:10.1016/j.bios.2024.116019
Li, C., Yang, W., Wang, M., Yu, X., Fan, J., Xiong, Y., et al. (2020). A review of coating materials used to improve the performance of optical fiber sensors. Sensors Switz. 20 (15), 4215–4224. doi:10.3390/s20154215
Li, M., Li, D., Xiu, G., and Long, Y. (2012). Applications of screen-printed electrodes in current environmental analysis. Curr. Opin. Electrochem 3 (1), 137–143. doi:10.1016/j.coelec.2017.08.016
Li, Z., Huang, L., Cheng, L., Guo, W., and Ye, R. (2024). Laser-induced graphene-based sensors in health monitoring: progress, sensing mechanisms, and applications. Small Methods 2400118, e2400118–e2400120. doi:10.1002/smtd.202400118
Liao, S., Shiau, K., Wang, F., and Yang, C. F. (2023). Fabrications of the flexible non-enzymatic glucose sensors using Au-CuO-rGO and Au-CuO-rGO-MWCNTs nanocomposites as carriers. Sensors 23, 8029. doi:10.3390/s23198029
Liaqat, I., Iqbal, N., Aslam, M., Nasir, M., Hayat, A., Han, D. X., et al. (2020). Co3O4 nanocubes decorated single-walled carbon nanotubes for efficient electrochemical non-enzymatic glucose sensing. SN Appl. Sci. 2 (10), 1756–1812. doi:10.1007/s42452-020-03531-2
Lim, S. Y., Shen, W., and Gao, Z. (2015). Carbon quantum dots and their applications. Chem. Soc. Rev. 44 (1), 362–381. doi:10.1039/C4CS00269E
Liu, J., Li, M., Liu, W., Hao, Z., Zhang, F., Pang, H., et al. (2024). Advances in non-enzymatic electrochemical materials for H2O2 sensing. J. Electroanal. Chem. 954, 118060. doi:10.1016/j.jelechem.2024.118060
Liu, W., Jia, H., Zhang, J., Tang, J., Wang, J., and Fang, D. (2020). Preparation of nitrogen-doped carbon quantum dots (NCQDs) and application for non-enzymatic detection of glucose. Microchem. J. 158, 105187. doi:10.1016/j.microc.2020.105187
Lorber, D. L., ElSayed, N. A., Bannuru, R. R., Shah, V., Puisis, M., Crandall, J., et al. (2024). Diabetes management in detention facilities: a statement of the American Diabetes Association. Diabetes Care 47, 544–555. doi:10.2337/dci24-0015
Luo, M., Zhu, Z., Huang, L., and Gao, Y. (2024). Optimization growth of graphene on annealed copper and its application as glucose sensor material. Mater Sci. Semicond. Process 173, 108147. doi:10.1016/j.mssp.2024.108147
Lv, Y., Zhou, C., Li, M., Huo, Z., Wei, Z., Wang, N., et al. (2024). A dual-mode sensing system based on carbon quantum dots and Fe nanozymes for the detection of α-glucosidase and its inhibitors. Talanta 268 (P1), 125328. doi:10.1016/j.talanta.2023.125328
Majdinasab, M. (2024). Wearable electrochemical biosensors for glucose monitoring: wearable biosensing in medicine and healthcare. Berlin, Heidelberg: Springer, 35–66.
Malode, S. J., Pandiaraj, S., Alodhayb, A., and Shetti, N. P. (2024). Carbon nanomaterials for biomedical applications: progress and outlook. ACS Appl. Bio Mater 7 (2), 752–777. doi:10.1021/acsabm.3c00983
Mohamad Nor, N., Ridhuan, N. S., and Abdul Razak, K. (2022). Progress of Enzymatic and Non-enzymatic electrochemical glucose biosensor based on nanomaterial-modified electrode. Biosens. (Basel) 12 (12), 1136. doi:10.3390/bios12121136
Mustafa, A., Alsafari, I. A., Somaily, H., Yousaf, S., Din, M. I., Rahman, J., et al. (2022). Fabrication, characterization of NiO–Co3O4/rGO based nanohybrid and application in the development of non-enzymatic glucose sensor. Phys. B Phys. Condens. Matter 648, 414404. doi:10.1016/j.physb.2022.414404
Muqaddas, S., Javed, M., Nadeem, S., Asghar, M. A., Haider, A., Ahmad, M., et al. (2023). Carbon nanotube fiber-based flexible microelectrode for electrochemical glucose sensors. ACS Omega 8 (2), 2272–2280. doi:10.1021/ACSOMEGA.2C06594
Nascimento, A. B., De Faria, L. V., Matias, T. A., Lopes, O. F., and Muñoz, R. A. A. (2024). Batch-injection amperometric determination of glucose using a NiFe2O4/carbon nanotube composite enzymeless sensor. Chemosensors 12, 112. doi:10.3390/chemosensors12060112
Naseri, M., Fotouhi, L., and Ehsani, A. (2018). Nanostructured metal organic framework modified glassy carbon electrode as a high efficient non-enzymatic amperometric sensor for electrochemical detection of H2O2. J. Electrochem. Sci. Technol. 9 (1), 28–36. doi:10.33961/jecst.2018.9.1.28
Nikitina, V. N., Karastsialiova, A. R., and Karyakin, A. A. (2023). Glucose test strips with the largest linear range made via single step modification by glucose oxidase-hexacyanoferrate-chitosan mixture. Biosens. Bioelectron. 220, 114851. doi:10.1016/j.bios.2022.114851
Nugba, B. E., El-Moneim, A. A., Mousa, N. O., and Osman, A. (2023). A nonenzymatic laser-induced flexible amperometric graphene electrode for glucose detection in saliva. Carbon Lett. 33 (6), 1767–1780. doi:10.1007/s42823-023-00489-0
Ortiz-Martínez, M., Flores-DelaToba, R., González-González, M., and Rito-Palomares, M. (2021). Current challenges and future trends of enzymatic paper-based point-of-care testing for diabetes mellitus type 2. Biosens. (Basel) 11 (12), 482. doi:10.3390/bios11120482
Patel, K. D., Singh, R. K., and Kim, H. W. (2019). Carbon-based nanomaterials as an emerging platform for theranostics. Mater Horiz. 6 (3), 434–469. doi:10.1039/c8mh00966j
Petit, B. C., and Bandosz, T. J. (2009). MOF–graphite oxide composites: combining the uniqueness of graphene layers and metal–organic frameworks. Adv. Mater. 21, 4753–4757. doi:10.1002/adma.200901581
Pourmadadi, M., Rahmani, E., Rajabzadeh-Khosroshahi, M., Samadi, A., Behzadmehr, R., Rahdar, A., et al. (2023). Properties and application of carbon quantum dots (CQDs) in biosensors for disease detection: a comprehensive review. J. Drug Deliv. Sci. Technol. 80, 104156. doi:10.1016/j.jddst.2023.104156
Qi, H., and Zhang, C. (2020). Electrogenerated chemiluminescence biosensing. Anal. Chem. 92 (1), 524–534. doi:10.1021/acs.analchem.9b03425
Radwan, A. B., Paramparambath, S., Cabibihan, J. J., Al-Ali, A. K., Kasak, P., Shakoor, R. A., et al. (2021). Superior non-invasive glucose sensor using bimetallic cuni nanospecies coated mesoporous carbon. Biosens. (Basel) 11 (11), 463. doi:10.3390/bios11110463
Rodriguez Ayala, F., Leñini, C., Cardinali, N., and Ricardo Grau, R. (2024). A double blind, placebo-controlled study to evaluate the efficacy of probiotic Bacillus subtilis DG101 in maintaining blood sugar homeostasis in healthy adults. Ann. Clin. Med. Cas. Rep. Rev. ACMCRR- 118. doi:10.47991/2834-5231/ACMCRR-118
Safarkhani, M., Aldhaher, A., Heidari, G., Zare, E. N., Warkiani, M. E., Akhavan, O., et al. (2023). Nanomaterial-assisted wearable glucose biosensors for noninvasive real-time monitoring: pioneering point-of-care and beyond. Nano Mater. Sci. 263–283. doi:10.1016/j.nanoms.2023.11.009
Sakr, M. A., Elgammal, K., Delin, A., and Serry, M. (2020). Performance-enhanced non-enzymatic glucose sensor based on graphene-heterostructure. Sensors Switz. 20 (1), 145. doi:10.3390/s20010145
Sandeep, R., Chandrasekar, N., Balaji, R., Alexander, P. S., Humaidi, N. Z. N., and Hwang, M. T. (2024). A comprehensive review on graphene-based materials: from synthesis to contemporary sensor applications. Mater. Sci. and Eng. R 159, 100805. doi:10.1016/j.mser.2024.100805
Sarkozy, D., Farsang, R., Szigeti, M., Austin, S., Lock, S., and Guttman, A. (2023). Capillary electrophoresis analysis of industrial galactooligosaccharides. J. Pharm. Biomed. Anal. 233, 115434. doi:10.1016/j.jpba.2023.115434
Shahriary, L., and Athawale, A. A. (2014). Graphene oxide synthesized by using modified hummers Approach. Int. J. Renew. Energy Environ. Eng. 02 (01), 2348–0157.
Shrestha, B. K., Ahmad, R., Mousa, H. M., Kim, I. G., Kim, J. I., Neupane, M. P., et al. (2016). High-performance glucose biosensor based on chitosan-glucose oxidase immobilized polypyrrole/nafion functionalized multi-walled carbon nanotubes bio-nanohybrid film. J. Colloid Interface Sci. 482, 39–47. doi:10.1016/j.jcis.2016.07.067
Sun, Y. P., Zhou, B., Lin, Y., Wang, W., Fernando, K. A. S., Pathak, P., et al. (2006). Quantum-sized carbon dots for bright and colorful photoluminescence. J. Am. Chem. Soc. 128 (24), 7756–7757. doi:10.1021/ja062677d
Tang, H., Chen, J., Yao, S., Nie, L., Deng, G., and Kuang, Y. (2004). Amperometric glucose biosensor based on adsorption of glucose oxidase at platinum nanoparticle-modified carbon nanotube electrode. Anal. Biochem. 331, 89–97. doi:10.1016/j.ab.2004.05.005
Tang, L., Xie, X., Zhou, Y., Zeng, G., Tang, J., Wu, Y., et al. (2017). A reusable electrochemical biosensor for highly sensitive detection of mercury ions with an anionic intercalator supported on ordered mesoporous carbon/self-doped polyaniline nanofibers platform. Biochem. Eng. J. 117, 7–14. doi:10.1016/j.bej.2016.09.011
Tao, B., Zheng, H., Li, J., Miao, F., and Zhang, P. (2023). Co-MOF@GO nanotubes constructed from nickel foam loaded with Co(OH)2: a non-enzymatic high-performance electrochemical sensor for glucose. Mater. Sci. Eng. B 298, 116895. doi:10.1016/j.mseb.2023.116895
Thangamuthu, M., Gabriel, W. E., Santschi, C., and Martin, O. J. F. (2018). Electrochemical sensor for bilirubin detection using screen printed electrodes functionalized with carbon nanotubes and graphene. sensors 18, 800. doi:10.3390/s18030800
Tiwari, M., Mhatre, S., Vyas, T., Bapna, A., and Govindarajan, R. (2023). A validated HPLC-RID method for quantification and optimization of total sugars: fructose, glucose, sucrose, and lactose in eggless mayonnaise. Separations 10, 199. doi:10.3390/separations10030199
Ullah khan, N., Prasad Sharma, B., Hussain Tumrani, S., Zahoor, M., Ali Soomro, R., Küçükdeniz, T., et al. (2024). Enhanced detection of glucose with carbon quantum dot-modified copper oxide: computational insight and machine learning modeling of electrochemical sensing. Microchem. J. 204, 110936. doi:10.1016/j.microc.2024.110936
Veerapandi, G., and Sekar, C. (2024). Investigation of glucose concentration in banana as a function of ripening time using Ni-Ca2CuO3 based electrochemical sensor. J. Food Compos. Analysis 126, 105888. doi:10.1016/j.jfca.2023.105888
Vinoth, V., Subramaniyam, G., Anandan, S., Valdés, H., and Manidurai, P. (2021). Non-enzymatic glucose sensor and photocurrent performance of zinc oxide quantum dots supported multi-walled carbon nanotubes. Mater. Sci. Eng. B 265, 115036. doi:10.1016/j.mseb.2020.115036
Wang, B., Cai, H., Waterhouse, G. I. N., Qu, X., Yang, B., and Lu, S. (2022). Carbon dots in bioimaging, biosensing and therapeutics: a comprehensive review. Small Sci. 2 (6). doi:10.1002/smsc.202200012
Wang, L., Yin, Y., Jain, A., and Susan Zhou, H. (2014). Aqueous phase synthesis of highly luminescent, nitrogen-doped carbon dots and their application as bioimaging agents. Langmuir 30 (47), 14270–14275. doi:10.1021/la5031813
Wang, X., Feng, Y., Dong, P., and Huang, J. (2019). A Mini Review on carbon quantum dots: preparation, properties, and electrocatalytic application. Front. Chem. 7, 671–679. doi:10.3389/fchem.2019.00671
Welegergs, G. G., Ambaye, A. D., Jokazi, M., Nwahara, N., and Nyokong, T. (2024). Bioengineering of one dimensional hierarchical Cu7S4 hollow nanotubes for non-enzymatic glucose sensing applications. RSC Adv. 14 (37), 27122–27131. doi:10.1039/d4ra05199h
Wu, S., He, Q., Tan, C., Wang, Y., and Zhang, H. (2013). Graphene-based electrochemical sensors. small 9 (8), 1160–1172. doi:10.1002/smll.201202896
Xia, J., Zou, B., Liu, F., Wang, P., and Yan, Y. (2022). Sensitive glucose biosensor based on cyclodextrin modified carbon nanotubes for detecting glucose in honey. J. Food Compos. Analysis 105 (301), 104221. doi:10.1016/j.jfca.2021.104221
Xia, Y., Su, T., Mi, Z., Feng, Z., Hong, Y., Hu, X., et al. (2023). Wearable electrochemical sensor based on bimetallic MOF coated CNT/PDMS film electrode via a dual-stamping method for real-time sweat glucose analysis. Anal. Chim. Acta 1278, 341754. doi:10.1016/j.aca.2023.341754
Xu, X., Ray, R., Gu, Y., Ploehn, H. J., Gearheart, L., Raker, K., et al. (2004). Electrophoretic analysis and purification of fluorescent single-walled carbon nanotube fragments. J. Am. Chem. Soc. 126 (40), 12736–12737. doi:10.1021/ja040082h
Xu, Z., Feng, J., Xing, S., Liu, Y., Chen, Y., Li, J., et al. (2024). Global trends and spatial drivers of diabetes mellitus mortality, 1990-2019: a systematic geographical analysis. Front. Endocrinol. (Lausanne) 15, 1370489. doi:10.3389/fendo.2024.1370489
Yadav, P. K., Chandra, S., Kumar, V., Kumar, D., and Hasan, S. H. (2023). Carbon quantum dots: synthesis, structure, properties, and catalytic applications for organic synthesis. Catalysts 13 (422), 422. doi:10.3390/catal13020422
Yang, H. L., Bai, L. F., Geng, Z. R., Chen, H., Xu, L. T., Xie, Y. C., et al. (2023). Carbon quantum dots: preparation, optical properties, and biomedical applications. Mater Today Adv. 18, 100376. doi:10.1016/j.mtadv.2023.100376
Yang, S., Chu, M., Du, J., Li, Y., Gai, T., Tan, X., et al. (2020). Graphene quantum dot electrochemiluminescence increase by bio-generated H2O2 and its application in direct biosensing. R. Soc. Open Sci. 7 (1), 191404. doi:10.1098/rsos.191404
Yang, Z., Guo, J., Wang, L., Zhang, J., Ding, L., Liu, H., et al. (2024). Nanozyme-enhanced electrochemical biosensors: mechanisms and applications. Small 20 (14), 2307815. doi:10.1002/smll.202307815
Yuan, C., Qin, X., Xu, Y., Jing, Q., Shi, R., and Wang, Y. (2020). High sensitivity detection of H2O2 and glucose based on carbon quantum dots-catalyzed 3, 3′, 5, 5′-tetramethylbenzidine oxidation. Microchem. J. 159, 105365. doi:10.1016/j.microc.2020.105365
Yuwen, T., Shu, D., Zou, H., Yang, X., Wang, S., Zhang, S., et al. (2023). Carbon nanotubes: a powerful bridge for conductivity and flexibility in electrochemical glucose sensors. J. Nanobiotechnology 21 (1), 320. doi:10.1186/s12951-023-02088-7
Zhang, S., Zeng, J., Wang, C., Feng, L., Song, Z., Zhao, W., et al. (2021). The Application of wearable glucose sensors in point-of-Care Testing. Front. Bioeng. Biotechnol. 9, 1–14. doi:10.3389/fbioe.2021.774210
Zheng, L., Cao, M., Du, Y., Liu, Q., Emran, M. Y., Kotb, A., et al. (2024). Artificial enzyme innovations in electrochemical devices: advancing wearable and portable sensing technologies. Nanoscale 16 (1), 44–60. doi:10.1039/D3NR05728C
Zheng, L., Liu, Y., and Zhang, C. (2021). A sample-to-answer, wearable cloth-based electrochemical sensor (WCECS) for point-of-care detection of glucose in sweat. Sens. Actuators B Chem. 343, 130131. doi:10.1016/j.snb.2021.130131
Zhou, Y., Wei, W., Lei, W., Li, F., Shu, J., Deng, Z., et al. (2024). Synergistically enhancing electrocatalysis and non-enzymatic sensing for glucose by iridium single-atom/nickel oxide/N-doped graphene. Anal. Bioanal. Chem. doi:10.1007/s00216-024-05226-8
Keywords: sensors, nanocomposites, electrodes, detection, selectivity, diabetes
Citation: Ambaye AD, Mamo MD, Zigyalew Y, Mengistu WM, Fito Nure J, Mokrani T and Ntsendwana B (2024) The development of carbon nanostructured biosensors for glucose detection to enhance healthcare services: a review. Front. Sens. 5:1456669. doi: 10.3389/fsens.2024.1456669
Received: 28 June 2024; Accepted: 23 October 2024;
Published: 08 November 2024.
Edited by:
Nagaraja Kodihalli, Manipal Institute of Technology, IndiaReviewed by:
Aarti Bhatt, Nitte Mahalinga Adyanthaya Memorial Institute of Technology, IndiaCopyright © 2024 Ambaye, Mamo, Zigyalew, Mengistu, Fito Nure, Mokrani and Ntsendwana. This is an open-access article distributed under the terms of the Creative Commons Attribution License (CC BY). The use, distribution or reproduction in other forums is permitted, provided the original author(s) and the copyright owner(s) are credited and that the original publication in this journal is cited, in accordance with accepted academic practice. No use, distribution or reproduction is permitted which does not comply with these terms.
*Correspondence: Abera Demeke Ambaye, YWJlcmFkYTEwQGdtYWlsLmNvbQ==
Disclaimer: All claims expressed in this article are solely those of the authors and do not necessarily represent those of their affiliated organizations, or those of the publisher, the editors and the reviewers. Any product that may be evaluated in this article or claim that may be made by its manufacturer is not guaranteed or endorsed by the publisher.
Research integrity at Frontiers
Learn more about the work of our research integrity team to safeguard the quality of each article we publish.