Explore article hub
- 1Department of Biology, University of Naples Federico II, Naples, Italy
- 2Institute for Sustainable Plant Protection, National Research Council of Italy, Florence, Italy
A Viewpoint on the Frontiers in Science Lead Article
Adapting crops for climate change: regaining lost abiotic stress tolerance in crops
Key points
- The rapid and human-driven changes happening to the climate are forcing plants to adapt quickly; this adaptation is not always possible and causes loss of genetic diversity.
- Advancements in biotechnology and phenotyping are crucial for accelerating plant adaptation to climate change, though cultural and commercial challenges may pose significant barriers.
- In addition to rewilding and de novo domestication, studies that investigate plant interactions with soil and airborne organisms can be leveraged to accelerate plant adaptation to climate change.
The Earth’s climate has continuously fluctuated throughout its history, with many of the most significant changes linked to changing levels of atmospheric carbon dioxide (CO2) and other greenhouse gases, such as nitrous oxide and methane, during cycles of glacial and interglacial periods (roughly every 100 thousand years since the Mid-Pleistocene). However, the current rise in CO2 is mainly due to human activities and is unprecedented in both its speed and concentration. For example, CO2 concentration now exceeds 400 ppm, which is well above the ~300 ppm seen in the interglacial periods before anthropogenic pollution. This rapid increase in CO2 levels is far beyond what organisms have experienced over the last million years and poses serious challenges to their existence and functioning.
While plants thrive under rising CO2 levels (1), rapid and continuous warming caused by the accumulation of greenhouse gases could push the Earth into a “hothouse” state (2). Rising temperatures, along with concurrent stresses such as prolonged or recurrent droughts and soil salinization, threaten global agricultural productivity and food security. Organisms that endure permanent or recurrent stresses associated with climate change may (i) adapt to the stress, (ii) migrate to areas more suitable for growth and reproduction, or, in absence of either of these two responses, (iii) undergo extinction.
Plants are sessile organisms. They can migrate through trans-generation seed dispersal, but this process is very slow, limited, and inefficient. Despite observed migration of plant communities in response to warming climates, many plants have limited options for migration. Moreover, only migration at speeds greater than 1 km per year would allow plants to successfully escape the impacts of climate change, as suggested by Corlett and Westcott (3). The potential for the plant migration strategy to cope with climate change is therefore clearly limited.
Plants possess the ability to adapt to a wide range of environmental conditions due to their phenotypic plasticity. However, the phenotypes of plants also react rather slowly to environmental pressures. Indeed, any environmental change needs to be sensed first by plants for transcriptional, post-transcriptional, or even epigenetic regulatory mechanisms to be activated. These responses lead to phenotypic changes associated with improved tolerance and/or resilience or the capacity to escape incoming stress. However, are these responses fast enough to cope with the current pace of climate warming? Previous studies cast doubts on this capacity (4, 5). Indeed, a fundamental assumption of paleoecology has been that the rate of evolution is far slower than the rate of current climate change. In addition, more extreme phenotypes in populations will likely be lost the faster this change occurs (6) (Figure 1).
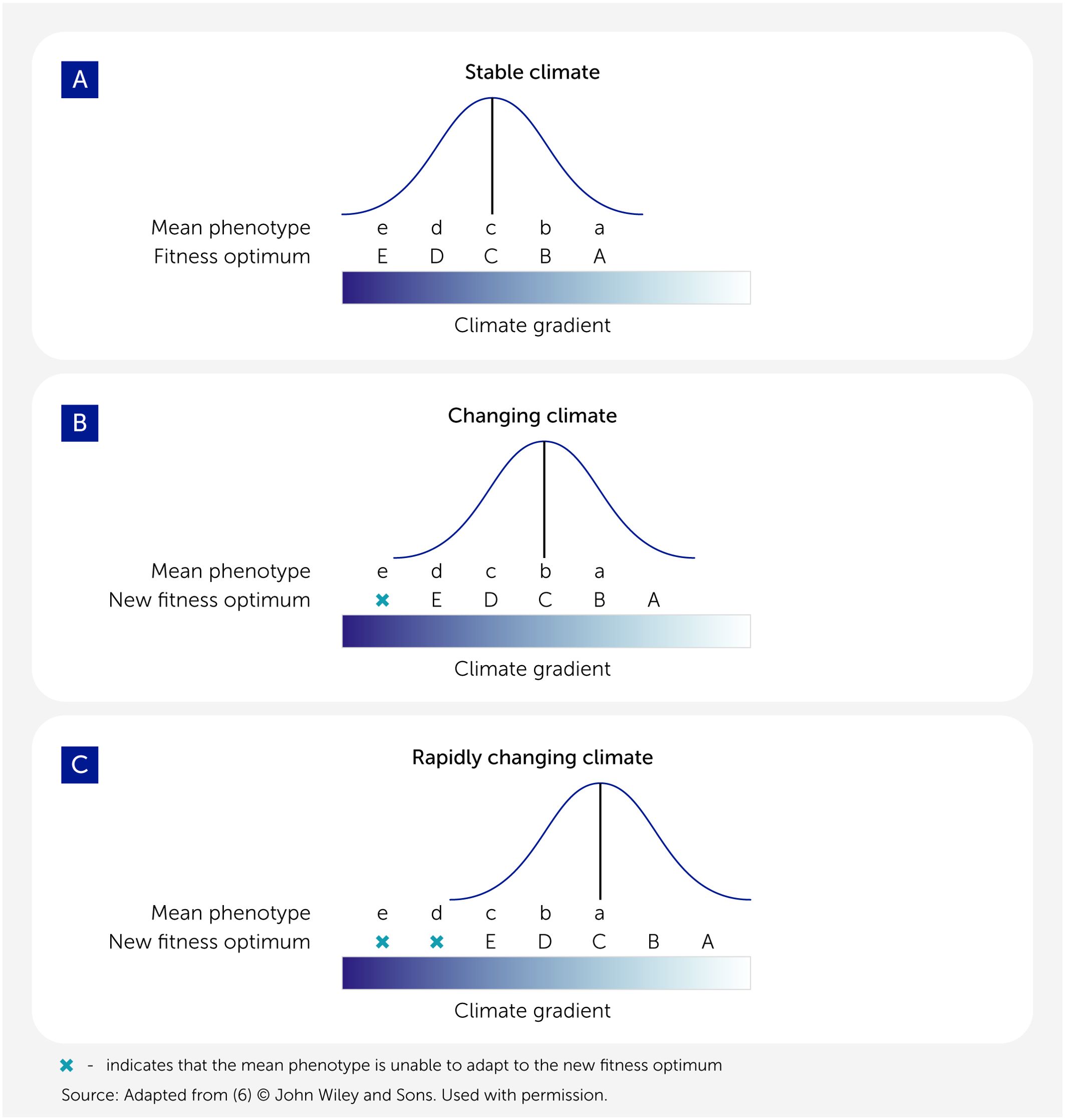
Figure 1. Changes of phenotypic means along a climate gradient. The figure shows changes in phenotypic means and optimal fitness means of populations of a plant species along a climate gradient under a (A) stable climate, (B) changing climate, and (C) rapidly changing climate. While optimal conditions follow the shift in the climate gradient, mean phenotypes are unable to follow; the more rapid the shift in climate change is, the more individual in a population will be facing conditions favoring their extinction. Adapted from (6), with permission from John Wiley and Sons.
Additionally, farming might have eroded the capacity for high phenotypic plasticity of agricultural (domesticated) plants. For example, breeding exclusively for high productivity selects for traits that enhance crop yields (e.g., shorter stems, increased fruit and seed size, or increased production). At the same time, it selects against other traits that positively influence plant survival, such as the synthesis of secondary metabolites, including phenolics, which reduce growth and/or produce antinutrients. As a result, plants lose crucial adaptation strategies, leaving them more vulnerable to the impacts of climate change, and to the loss of biodiversity.
To allow plants to adapt to climate change in an effective and timely manner may require “help” from our side. In their Frontiers in Science lead article, Palmgren and Shabala (7) discuss current knowledge about the possibility of reintroducing lost traits from wild relatives of cultivated plants (rewilding) or, alternatively, of de novo domestication of wild plants. However, adaptation to abiotic stresses by rewilding plants probably requires reintroduction of a suite of genes, as tolerance to abiotic stresses is a complex trait, entailing activation of multiple metabolic pathways. This is unlikely to result in the fast help that is required. In fact, taking on board the case of plant adaptation to salinity as highlighted in Palmgren and Shabala (7), and despite considerable progress in understanding plant responses to salt stress, breeding of salt-tolerant cultivars has progressed slowly. Even when salt-tolerance genes have been identified, we have not yet been able to produce commercially relevant salt-tolerant varieties (8).
Driving accelerated domestication could present a more promising and practical method for developing plants that can adapt better to climate change. Today, around thirty domesticated species account for a significant portion of dietary diversity, with only three principal cereal grains (rice, wheat, and maize) contributing to more than half of the caloric intake worldwide (9). Thousands of edible species have been left out in the course of plant domestication; these underutilized species, however, might hold the potential to transform our food systems toward being more nutritious, sustainable, and resilient to climate change (10).
There are numerous suitable candidates for domestication among wild relative species, especially when stress-related tolerance has already successfully evolved in nature (e.g., in halophytes). However, domestication today often requires conversion of wild plant species into crops that are not only viable but also produce high yields (11). It is unclear whether this goal will be successfully met, as any step toward setting final yields (e.g., successful seed germination or propagation, resistance to plant diseases, and a positive response to farming practices such as harvesting) needs to be tested, and this, if achievable, may require time. Even if domestication of wild relatives can be successfully carried out, will the new crops meet the expectations of farmers and consumers? Tolerance to abiotic stressors often leads to better nutritional quality of food crops (12), but are farmers ready to cultivate plants with presumably lower top yields? Are consumers willing to change their alimentary habits in favor of novel crops? Last but not least, as many developed countries are not self-sufficient in terms of agricultural production and depend on imports from developing countries, are all these countries willing to adopt policies encouraging cultivation of plants not solely based on high yields? In other words, should we expect only plants to adapt to climate change or should humans also quickly adapt their food choices?
Basic research can contribute to expediting both rewilding and de novo domestication, overcoming limitations often observed when growing crops in stressful conditions. High-throughput phenotyping, in particular, has previously been a bottleneck, slowing the selection of suitable plant material for breeding and monitoring resistance to biotic and abiotic stresses. However, significant progress has been made in plant phenotyping by taking advantage of new technologies that merge new sensors (especially optical sensors) with robotics and artificial intelligence (13). Today, plant phenotyping is the main tool scientists use to non-destructively characterize plant–environment interactions over the plant’s lifetime, and breeders use it for high-throughput selection of desirable genotypes for specific traits. However, the massive volume of plant phenotyping data needs harmonization and powerful statistical tools to be useful in the rapid selection of adapted plants.
There are other biotechnological tools that can help to increase plants’ capacity to adapt to fast-changing environments. For example, genomic selection promises to overcome problems experienced when applying marker-assisted selection for quantitative traits controlled by multiple genes, such as those involved in climate change adaptation (14). Other technologies, such as directed evolution, may speed up retrieval and insertion of resistance traits, with microbial assistance (12).
Significant progress might come from improved knowledge of plant interactions with soil and airborne microorganisms. This emerging field has benefitted from great advances in the understanding of microbial genomics, plant-microbe interactions, and the resulting changes in plant physiology and phenotypes. These developments hold great potential for enhancing plant adaptation to changing environments.
As a final point, it is important to note that developing crops without accounting for their need to cope with environmental stresses may not be the most effective strategy for future agriculture. While farming practices strive to provide conditions that minimize stress to maximize growth and yield, climate change is expected to exacerbate environmental stresses and crop yield losses, and a lack of resources, such as water and fertilizers, are increasingly limiting plant production (15). Moreover, farming marginal lands has important economic, environmental, and social consequences, e.g., fighting desertification and decreasing the migration of environmental refugees. Finally, secondary metabolites that plants need to self-defend against stresses, such as many antioxidants and pigments, have positive nutritional properties (12). Thus, breeding plants that successfully cope with stresses can also make our food safer and healthier.
Statements
Author contributions
FL: Conceptualization, Writing – original draft, Writing – review & editing. GA: Conceptualization, Writing – original draft, Writing – review & editing.
Funding
The authors declare that financial support was received for the research, authorship, and/or publication of this article. This study was supported by the European Commission Horizon Europe project “EMPHASIS-GO: Bringing EMPHASIS (European Infrastructure for multi-scale Plant Phenomics and Simulation for food security in a changing climate) to Operation”. We do also acknowledge the European Union Next-GenerationEU (Piano Nazionale di Ripresa e Resilienza -PNRR), Missione 4, Componente 2, investimento 3.1 (D.D. 130 21 June 2022, IR0000032) project Italian Integrated Environmental Research Infrastructures System (ITINERIS). The funders were not involved in the study design, collection, analysis, interpretation of data, the writing of this article or the decision to submit it for publication.
Conflict of interest
The authors declare that the research was conducted in the absence of any commercial or financial relationships that could be construed as a potential conflict of interest.
Publisher’s note
All claims expressed in this article are solely those of the authors and do not necessarily represent those of their affiliated organizations, or those of the publisher, the editors and the reviewers. Any product that may be evaluated in this article, or claim that may be made by its manufacturer, is not guaranteed or endorsed by the publisher.
References
1. Ainsworth EA, Long SP. 30 years of free-air carbon dioxide enrichment (FACE): what have we learned about future crop productivity and its potential for adaptation? Global Change Biol (2021) 27(1):27–49. doi: 10.1111/gcb.15375
2. Steffen W, Rockström J, Richardson K, Lenton TM, Folke C, Liverman D, et al. Trajectories of the Earth system in the Anthropocene. Proc Natl Acad Sci USA (2018) 115(33):8252–9. doi: 10.1073/pnas.1810141115
3. Corlett RT, Westcott DA. Will plant movements keep up with climate change? Trends Ecol Evol (2013) 28(8):482–8. doi: 10.1016/j.tree.2013.04.003
4. Loarie SR, Duffy PB, Hamilton H, Asner GP, Field CB, Ackerly DD. The velocity of climate change. Nature (2009) 462:1052–5. doi: 10.1038/nature08649
5. Oostra V, Saastamoinen M, Zwaan BJ, Wheat C. Strong phenotypic plasticity limits potential for evolutionary responses to climate change. Nat Commun (2018) 9:1005. doi: 10.1038/s41467-018-03384-9
6. Davis MB, Shaw RG, Etterson JR. Evolutionary responses to changing climate. Ecol (2005) 86(7):1704–14 doi: 10.1890/03-0788
7. Palmgren M, Shabala S. Adapting crops for climate change: regaining lost abiotic stress tolerance in crops. Front Sci (2024) 2:1416023. doi: 10.3389/fsci.2024.1416023
8. Hu Y, Schmidhalter U. Opportunity and challenges of phenotyping plant salt tolerance. Trends Plant Sci (2023) 28(5):552–66. doi: 10.1016/j.tplants.2022.12.010
9. Food and Agriculture Organization of the United Nations. The second report on the state of the world’s plant genetic resources for food and agriculture. Rome: FAO (2010). Available at: https://www.fao.org/4/i1500e/i1500e00.htm
10. Ray A, Ray R, Sreevidya E. How many wild edible plants do we eat—their diversity, use, and implications for sustainable food system: an exploratory analysis in India. Front Sustain Food Syst (2020) 4:56. doi: 10.3389/fsufs.2020.00056
11. Rozema J, Flowers T. Crops for a salinized world. Science (2008) 80(322):1478–80. doi: 10.1126/science.1168572
12. Gionfriddo M, De Gara L, Loreto F. Directed evolution of plant processes: toward a green (r)evolution? Trends Plant Sci (2019) 24(11):999–1007. doi: 10.1016/j.tplants.2019.08.004
13. Watt M, Fiorani F, Usadel B, Rascher U, Muller O, Schurr U. Phenotyping: new windows into the plant for breeders. Annu Rev Plant Biol (2020) 71:689–712. doi: 10.1146/annurev-arplant-042916-041124
14. Budhlakoti N, Kushwaha AK, Rai A, Chaturvedi KK, Kumar A, Pradhan AK, et al. Genomic selection: a tool for accelerating the efficiency of molecular breeding for development of climate-resilient crops. Front Genet (2022) 13:832153. doi: 10.3389/fgene.2022.832153
Keywords: abiotic stress tolerance, plant adaptation, domestication, global warming, plant phenotypic plasticity, plant-microbe interactions, rewilding
Citation: Loreto F and Atzori G. Climate challenges: can plants adapt in time? Front Sci (2024) 2:1522649. doi: 10.3389/fsci.2024.1522649
Received: 04 November 2024; Accepted: 27 November 2024;
Published: 05 December 2024.
Edited and reviewed by:
Francisco J. Corpas, Spanish National Research Council (CSIC), SpainCopyright © 2024 Loreto and Atzori. This is an open-access article distributed under the terms of the Creative Commons Attribution License (CC BY). The use, distribution or reproduction in other forums is permitted, provided the original author(s) and the copyright owner(s) are credited and that the original publication in this journal is cited, in accordance with accepted academic practice. No use, distribution or reproduction is permitted which does not comply with these terms.
*Correspondence: Francesco Loreto, ZnJhbmNlc2NvLmxvcmV0b0B1bmluYS5pdA==