Explore article hub
- 1Research Group Plant Defense Physiology, Max Planck Institute for Chemical Ecology, Jena, Germany
- 2Matthias Schleiden Institute of Genetics, Bioinformatics and Molecular Botany, Department of Plant Physiology, Friedrich Schiller University, Jena, Germany
A Viewpoint on the Frontiers in Science Lead Article
Enabling sustainable crop protection with induced resistance in plants
Key points
- Induced resistance (IR), as part of a plant’s innate immune response, could significantly contribute to sustainable and eco-friendly agriculture in the future.
- Effectively employing IR will require detailed knowledge about the eliciting signals and defensive compounds involved in the crop plant of interest to avoid accumulation of compounds harmful to humans.
- IR will not be sufficient for successful plant protection in isolation but is important in combination with additional specified and adapted methods.
Against the backdrop of climate change, spreading pests and pathogens, the demand to feed the world’s growing population, and the enormous environmental impact associated with the use of pesticides, the Frontiers in Science lead article by Flors et al. (1) presents the alternative and innovative idea of using plants’ intrinsic resilience capacity in an environmentally friendly and efficient way to tackle these challenges. This timely article highlights the phenomenon of induced resistance (IR) as part of the plant immune response against pathogenic and/or herbivorous attacks. A major goal for researchers currently is to reduce or even replace the use of synthetic chemical pesticides in order to protect biodiversity in a sustainable, ecological, and economically viable way and minimize harmful effects on soil and groundwater. Flors et al. (1) propose that endogenous, plant-based defense mechanisms are generally more environmentally friendly, efficient, and targeted than, for example, the use of pesticides—thereby providing an impetus toward a future for agriculture that relies less on pesticides. We support the authors’ idea and offer our perspective and a few critical considerations that we hope will help drive the process forward.
IR influences plant development and stress-mediated reactions
The induction of appropriate reactions in plants against abiotic or biotic stress factors, in particular against attacking pests and pathogens, is part of the plant immune response and is known as IR (2). IR is characterized by drastic changes in gene regulation and in the entire primary and secondary metabolism, with the aim of synthesizing and accumulating defense-related compounds that are directed against the aggressor and that protect the plant both locally at the site of attack and systemically (3). These mechanisms and compounds, which have evolved over millions of years, are efficient and effective in the defense of individual plants. However, they have limitations that mean modern, yield-oriented agriculture with many monocultures need to use large quantities of additional pesticides. For example, the prolonged establishment of natural intrinsic defenses might be too slow from an agricultural point of view. Initiating IR without the presence of pathogens or pests will avoid this time-handicap. However, IR is usually accompanied by yield losses, in part because the metabolic switch from growth to defense causes a “trade-off” within the plant: energy and nutrients normally invested in growth are redirected to defensive purposes. Growth-promoting agents could compensate for this, as Flors et al. (1) discuss. Nevertheless, the search for signaling substances that do not trigger IR but rather “prime” the plants might represent a better alternative. As Mauch-Mani et al. (4) have described in detail, priming can be seen as an adaptive part of IR. The plant is prepared for a possible stress event without switching its metabolism; it can then react quickly in an emergency.
IR involves an energy trade-off that can reduce plant growth, owing to the inhibition of one resistance pathway by the other. Indeed, IR can reduce crop yields by up to 18% (5). The reasons for the reduced growth can vary, ranging from altered metabolism (as described in the review) to influences on the natural microbiome. The surface of plants is a habitat for a myriad of microorganisms that ensure its survival in a well-balanced way by influencing fitness, growth, and multi-disease resistance (6) with a stable resistance gene polymorphism maintained through complex and diffuse community-wide interactions (7). Pathogen immunity and non-pathogen recruitment function in parallel (8). By altering plant-microbe interactions, IR-related compounds risk disrupting the microbiome balance, leading to dysbiosis and, potentially, devastating plant diseases and loss of productivity. Moreover, a particular IR-related compound may increase a plant’s susceptibility to other environmental stressors by creating an imbalance in a well-established microbiome. Focusing on priming as part of IR could be the better solution, as it is activated on demand and could be more specific to the stressor involved.
Activation of secondary metabolites can be harmful to humans
IR activating biosynthetic pathways leads to the accumulation of secondary metabolites. Some of these promote human health, such as many flavonoids, but others without nutritional/nutraceutical value are undesirable in this context, e.g., glucosinolates or other bitter compounds, cyanogenic glucosides, saponins, tannins, and alkaloids. Thus, high concentrations of glucosinolates would make crops more resistant to certain pathogens but also unpalatable to humans or livestock. While cyanogenic glucosides and glucosinolates themselves are not harmful, their deglycosylation releases the highly toxic aglycon hydrocyanic acid and the irritant isothiocyanates, respectively. Isothiocyanates, specifically, are responsible for a pungent taste. Saponins have detergent properties that can influence the permeability of the membrane, while tannins can hinder resorption in the human body. Some alkaloids, such as strychnine or solanine, can even be life-threatening. These properties are all undesirable in agricultural crops but may be desirable in medicinal plants. Alkaloids, for example, include various medicinal substances such as morphine and codeine, together with stimulants such as caffeine and nicotine. This necessitates a conscientious approach to the application of particular IR-related compounds and the appropriate legal basis and controls.
Combinatorial IR application has advantages and disadvantages
Plants are exposed to many different pathogens and pests in their environment. Their innate and induced immunity arises from various mechanisms, depending on the attacker. If the plant cannot defend itself (e.g., if the pathogen is not recognized) IR-inducing chemicals can be used to activate the relevant immune pathways. However, each chemical can typically activate only one defense pathway, unavoidably necessitating the combination of different plant protection strategies (Figure 1) and causing new challenges. Here, the use of synthetic chemical plant protection products must be replaced or reduced to protect biodiversity and minimize the impact on soil and groundwater. One approach that could be combined with IR is resistance induction through intercropping. Chadfield et al. (9) found that intercropping reduced nematode-mediated crop damage by 40% and disease incidence by 55%. These results show that intercropping can be ideally used in combination with, for example, the IR-related compound benzo-(1,2,3)-thiadiazole-7-carbothioic acid S-methyl ester, which reduces fungal and bacterial symptoms by 30–90% (5). Another approach addresses the application of selective RNA interference (RNAi)-based crop protection agents with a sequence-based mode of action. RNAi agents utilize the naturally occurring principle of RNAi-mediated gene silencing in cells. Their application, as so-called RNAi sprays, promises to be free of genetically modified organisms (GMOs), environmentally friendly, and specific.
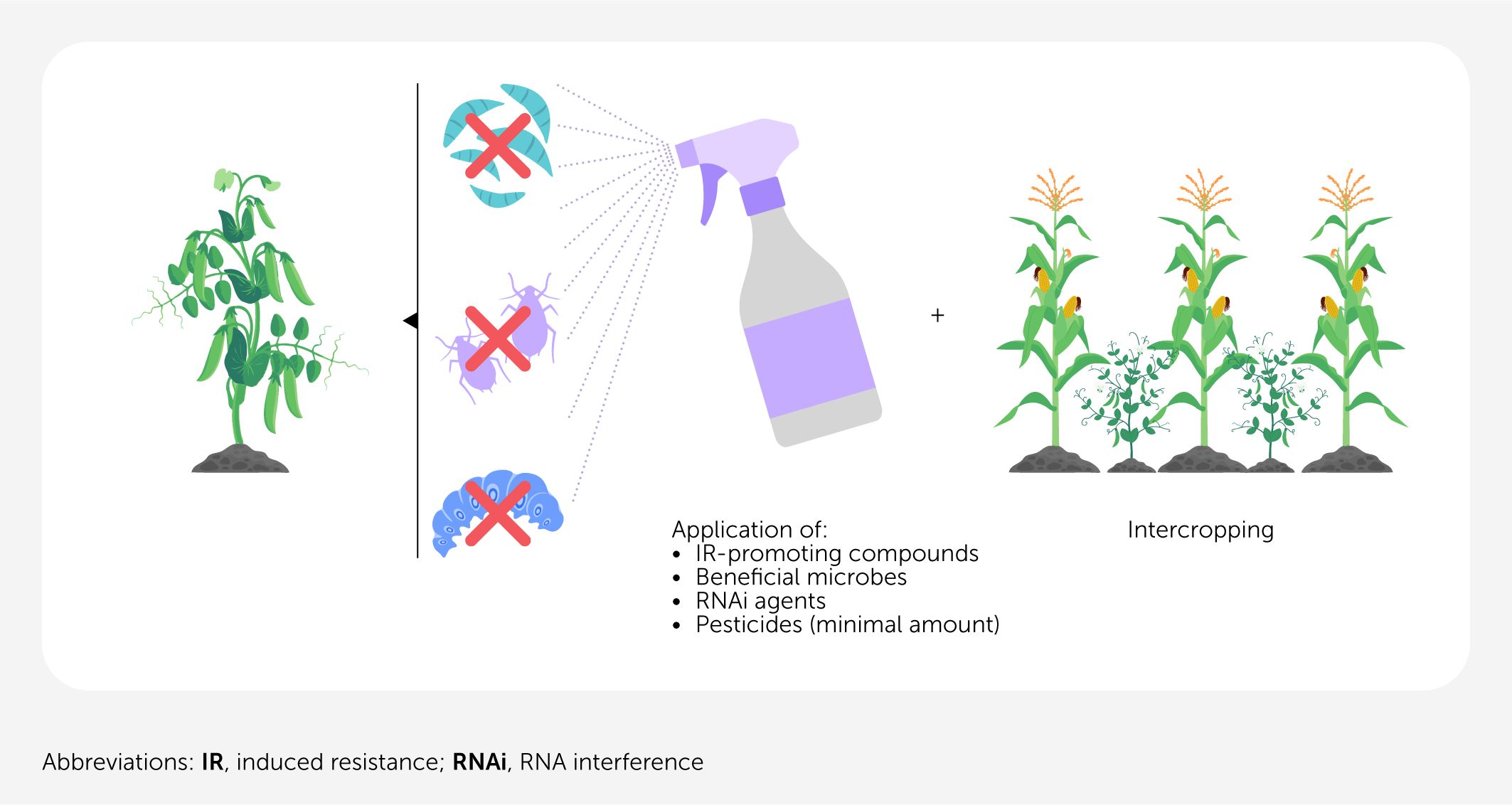
Figure 1. Scheme for an induced-resistance (IR)-based plant protection approach. IR works best when applied in combination with other pest management practices, such as the application of small amounts of pesticide, RNA interference (RNAi)-based pest control, or intercropping to enhance plant resistance against nematodes or herbivores. In the best-case scenario, the application targets all pathogens/pests at the right time and avoids negative growth effects and crop losses.
Applying the combinatorial approach in the field requires a monitoring system to determine the optimal timing, quantity, and type of crop protection product to avoid opposing or overly amplifying effects. It is also critical to define the best means of field application (spray, pour, or electrospinning) and the product formulation (e.g., powder, granules, emulsion, gas, nanoparticles, or nanofibers). These are tasks for industry; research should focus on potential combinations and their ecological effects.
Flors et al. (1) overlook one potentially important ecological risk from IR: microorganisms introduced to induce resistance could inadvertently become invasive species in areas where they do not occur naturally. The literature is full of examples of isolated microbes worldwide that can benefit their host plants when inoculated together, for example by increasing their resistance to salt, drought stress, or pathogens (10)—in principle supporting their use elsewhere for agriculture. However, when introduced into a new environment, such microbes might behave as invasive species. For example, they could displace important microbial species in ecological niches, affect biocenosis in the field, or, even worse, affect the whole biotope. To our knowledge, there are no comprehensive studies investigating the consequences of such introduced microbes on the environment, which is a topic worthy of further study.
In conclusion, Flors et al. (1) clearly show that IR could be an excellent and promising approach for future agriculture, even if it will most likely only work in combination with other complementary approaches. Further research should focus on understanding the underlying mechanisms of IR in different species to achieve additive or synergistic effects on plant defense as well as on the development of optimal applications for complementary strategies.
Statements
Author contributions
AM: Conceptualization, Writing – original draft, Writing – review & editing. AF: Conceptualization, Writing – original draft, Writing – review & editing.
Funding
The author(s) declare that no financial support was received for the research, authorship, and/or publication of this article.
Conflict of interest
The authors declare that the research was conducted in the absence of financial relationships that could be construed as a potential conflict of interest.
The author AM declared that they were an editorial board member of Frontiers, at the time of submission. This had no impact on the peer review process and the final decision.
Publisher’s note
All claims expressed in this article are solely those of the authors and do not necessarily represent those of their affiliated organizations, or those of the publisher, the editors and the reviewers. Any product that may be evaluated in this article, or claim that may be made by its manufacturer, is not guaranteed or endorsed by the publisher.
References
1. Flors V, Kyndt T, Mauch-Mani B, Pozo MJ, Ryu C-M, Ton J. Enabling sustainable crop protection with induced resistance in plants. Front Sci (2024) 2:1407410. doi: 10.3389/fsci.2024.1407410
2. Walters D, Heil M. Costs and trade-offs associated with induced resistance. Physiol Mol Plant Pathol (2007) 71:3–17. doi: 10.1016/j.pmpp.2007.09.008
3. Pieterse CMJ, Zamioudis C, Berendsen RL, Weller DM, Van Wees SCM, Bakker PAHM. Induced systemic resistance by beneficial microbes. Annu Rev Phytopathol (2014) 52:347–75. doi: 10.1146/annurev-phyto-082712-102340
4. Mauch-Mani B, Baccelli I, Luna E, Flors V. Defense priming: an adaptive part of induced resistance. Annu Rev Plant Biol (2017) 68:485–512. doi: 10.1146/annurev-arplant-042916-041132
5. Vallad GE, Goodman RM. Systemic acquired resistance and induced systemic resistance in conventional agriculture. Crop Sci (2004) 44(6):1920–34. doi: 10.2135/cropsci2004.1920
6. Agler MT, Ruhe J, Kroll S, Morhenn C, Kim ST, Weigel D, et al. Microbial hub taxa link host and abiotic factors to plant microbiome variation. PloS Biol (2016) 14:e1002352. doi: 10.1371/journal.pbio.1002352
7. Karasov TL, Kniskern JM, Gao L, DeYoung BJ, Ding J, Dubiella U, et al. The long-term maintenance of a resistance polymorphism through diffuse interactions. Nature (2014) 512:436–40. doi: 10.1038/nature13439
8. Jose J, Teutloff E, Naseem S, Barth ET, Halitschke R, Marz M, et al. Immunity and bacterial recruitment in plant leaves are parallel processes whose link shapes sensitivity to temperature stress. bioRxiv [preprint] (2024). doi: 10.1101/2024.06.10.598336
9. Chadfield VG, Hartley SE, Redeker KR. Associational resistance through intercropping reduces yield losses to soil-borne pests and diseases. New Phytol (2022) 235(6):2393–405. doi: 10.1111/nph.18302
Keywords: priming, energy trade-off, secondary metabolites, combinatorial IR application, ecological microbiome implications, invasive microbial species
Citation: Mithöfer A and Furch ACU. Induced resistance: making the most of plants’ innate immune response. Front Sci (2024) 2:1483191. doi: 10.3389/fsci.2024.1483191
Received: 19 August 2024; Accepted: 18 September 2024;
Published: 15 October 2024.
Edited and Reviewed by:
Ian A. Dubery, University of Johannesburg, South AfricaCopyright © 2024 Mithöfer and Furch. This is an open-access article distributed under the terms of the Creative Commons Attribution License (CC BY). The use, distribution or reproduction in other forums is permitted, provided the original author(s) and the copyright owner(s) are credited and that the original publication in this journal is cited, in accordance with accepted academic practice. No use, distribution or reproduction is permitted which does not comply with these terms.
*Correspondence: Axel Mithöfer, YW1pdGhvZWZlckBpY2UubXBnLmRl
†ORCID: Axel Mithöfer, orcid.org/0000-0001-5229-6913
Alexandra C. U. Furch, orcid.org/0000-0002-9968-753x