Explainer
Powering up the next generation of biocomputers with brain organoids
Artificial intelligence (AI) boasts impressive achievements – from diagnosing medical conditions to composing poetry. Still, the brain continues to outperform machines in many ways. For example, brains can perform several tasks simultaneously and are generally better at processing complex information such as images. In addition, brains are significantly more energy efficient than AI and machine learning algorithms. In fact, silicon-based computing is quickly reaching its processing capacity limits because its energy demands are becoming unsustainable.
To overcome these limitations, we are working to create biocomputers based on brain cell cultures called brain organoids. In our vision, this approach will allow us to harness the remarkable computational capacity of the brain to power revolutionary ‘biological’ computers.
We call this new field organoid intelligence (OI), and define the following broad areas of work to realize its full potential:
Scaling up current brain organoids into more complex and durable structures that are enriched with cells and genes associated with learning
Connecting brain organoids to next-generation input and output devices and AI/machine learning systems
Developing new models, algorithms, and interface technologies to communicate with brain organoids, understand how they learn and compute, and process and store the massive amounts of data they will generate
Taking an ‘embedded ethics’ approach to ensure OI develops in an ethically and socially responsive manner.
What is organoid intelligence?
Organoid intelligence is an emerging interdisciplinary scientific field aiming to establish a new type of biological computing system. These next-generation biocomputers will harness the computational power of the brain by using lab-grown brain organoids as ‘biological hardware’. Custom-built devices will record neural activity from the organoid and transmit instructive information to it. These interactions will be supported by new learning models and AI algorithms.
This technology promises unprecedented advances in computing speed, processing power, data efficiency, and storage capabilities – all with lower energy needs. OI could also improve our understanding of brain development, learning, and memory, which may inspire new treatments for neurological and neurodevelopmental disorders.
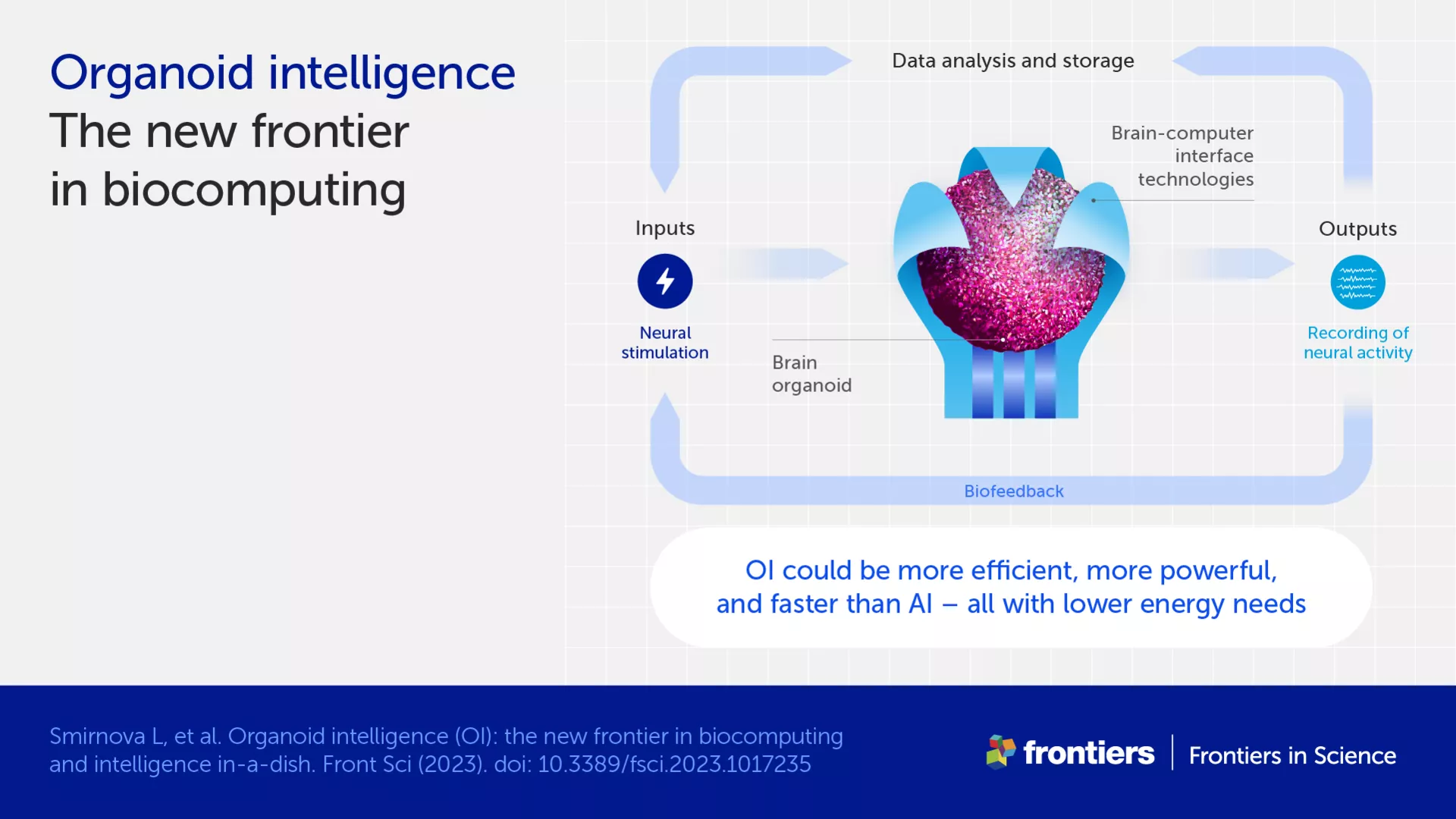
What are brain organoids?
Brain organoids are three-dimensional cultures of brain cells grown in the lab. They are generated from stem cells: unique cells that can become any type of cell under the right conditions. Specifically, we develop brain organoids from skin cells using a technology that first turns them into stem cells, and then into brain cells. These organoids measure about 500 µm and contain less than 100,000 cells, about one three-millionth the size of the human brain.
What advantages would organoid-based biocomputers offer?
While silicon-based computers are much better than brains in dealing with numbers, brains are far superior in performing complex computations. For instance, humans can learn to distinguish two types of objects (such as a dog and a cat) using around ten training samples, while AI algorithms need many millions.
The AlphaGo system – the AI that beat the world champion in Go in 2016 – demonstrates the difference between the way humans and computers learn. AlphaGo was trained on data from 160,000 games to reach this level of performance. To experience these many games, a person would have to play for five hours each day, for over 175 years.
Brains are not only better at processing data than existing computers, they are also more energy efficient. For example, the training of AlphaGo required 4x10¹⁰ joules of energy, which is more than is needed to sustain an active adult human for a decade. These high energy requirements will only increase as machine-learning algorithms become more powerful. For instance, in 2016 the power demands of US-based data centers already took the equivalent of 34 coal-powered plants, each generating 500 megawatts. Continuously raising energy consumption to match our growing computational needs is unsustainable, especially if technology companies are to meet their commitments to become carbon negative by 2030.
Another aspect in which brains outdo silicon-based computers is data storage. The brain boasts an impressive storage capacity: based on its 86-100 billion neurons linked through over 10¹⁵ connection points, it is estimated at 2,500 TB. Being significantly more efficient than current computers, brain-based biocomputers could theoretically meet the US data storage capacity using only 1600 kilowatts of energy.
Even though brain organoids aren‘t ‘mini brains’, they may offer similar computational power since they share key aspects of brain function and structure. A recently published study provides strong proof of concept, demonstrating that a group of neurons in a dish can learn to play the game Pong. Still, for organoid intelligence, brain organoids will need to become even more brain-like.
What advances in brain organoid technology are needed for organoid intelligence?
A crucial first step for developing organoid intelligence is to increase the organoids‘ size. Current brain organoids contain tens of thousands of cells, but to support sophisticated computations we aim to raise this number to 10 million.
Another important step is to increase organoids‘ cell diversity. The brain contains many types of cells besides neurons, such as oligodendrocytes and astrocytes. These cells also play key roles in learning and memory and have already been incorporated into brain organoids for OI. Finally, we also need to optimize organoid culture conditions to allow the expression of genes that boost learning and memory.
Nourishing these organoids is another interesting challenge. In the brain, blood vessels supply nutrients and remove waste. However, organoids don’t contain vessels and rely on passive diffusion instead. Since this approach won’t work with larger organoids, we must develop artificial blood vessels to keep the organoids alive and well. This can be achieved using microfluidic systems, a new technology that allows fluids to be manipulated at very small scales. These systems would not only maintain the health of the organoid, but also allow us to communicate with them via chemical signals.
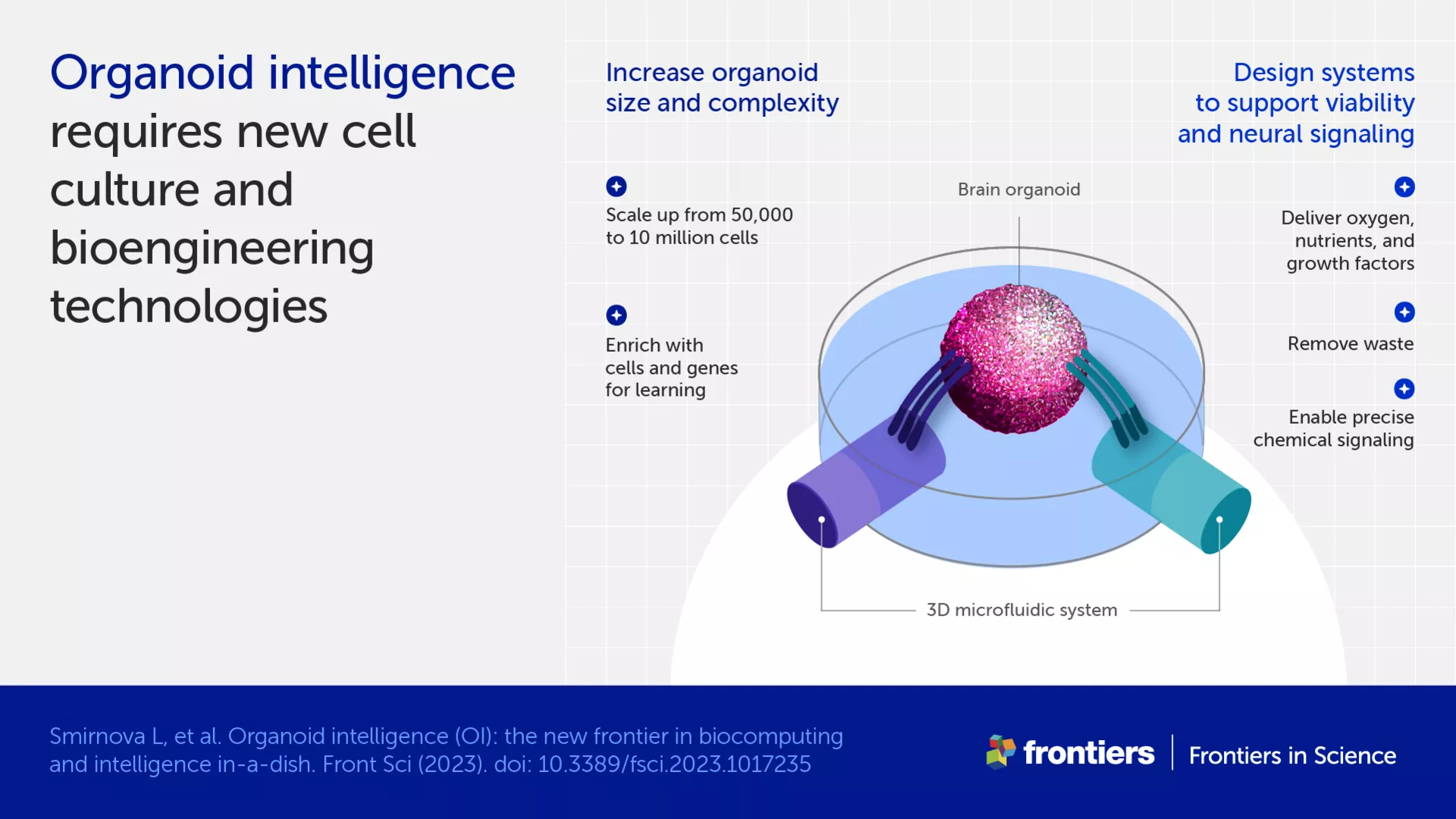
What devices are needed for communicating with organoid intelligence?
To teach organoid intelligence new things and know what it’s ‘thinking‘, we need to transmit information to, and record information from, the organoid. This can be achieved using brain-machine interface technology. A familiar example is the EEG cap, where a metal electrode array picks up brain activity and relays it to computers that then analyze the signals and translate them into actions, such as moving a cursor across the screen.
A new generation of 3D microelectrode arrays is crucial for OI. To ensure contact between the tissue and the device, we propose growing brain organoids inside flexible shells that are densely covered with tiny electrodes, similar in structure to EEG caps. These 3D microelectrode arrays will be used to record activity and send electric signals across the entire surface of the organoid.
Other complementary recording methods can provide access to cells inside the organoid. For example, optogenetics is a method commonly used in neuroscience to monitor and control neural activity using light. Another possible way to reach interior cells is to insert elongated electrodes into the organoid. These types of probes have been used in neuroscience research for decades, consistently providing high quality recordings.
In addition to sophisticated recording methods, we will also need new types of input devices for real-time control of OI. These may include chemical signaling, optogenetic stimulation, and direct connections to sensory organs, such as a retinal organoids. Eventually, OI could be scaled up to integrate a wide range of stimulation and recording tools within interconnected networks of organoids that implement more complex computations.
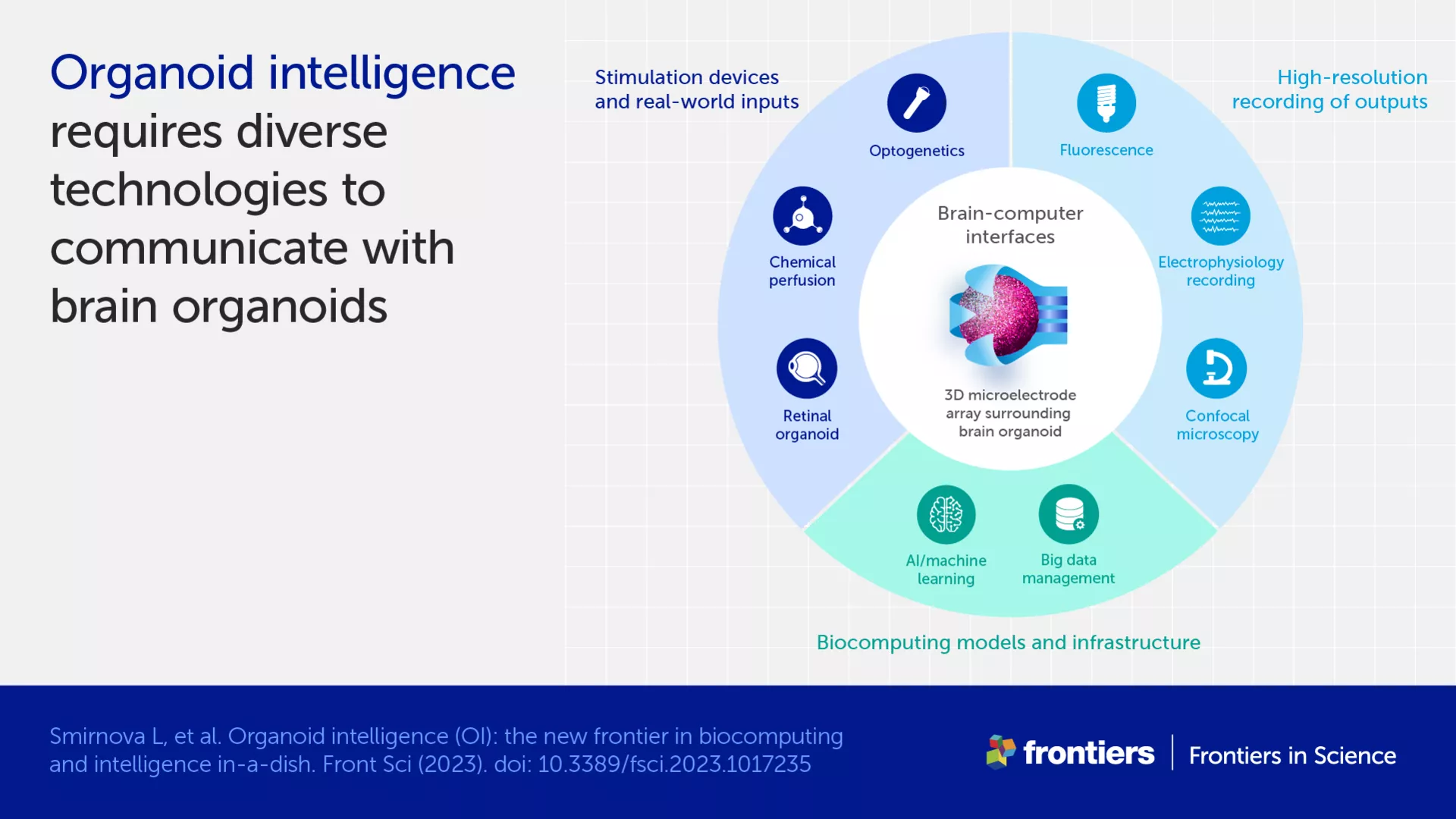
How will we teach organoid intelligence – and how will we know if it actually learns?
Realizing the potential of OI for biocomputers requires more than just recording and stimulating neural activity. These advanced technologies will generate massive datasets that will require new big data infrastructure, as well as software tools customized to handle this form of modern biological data.
Here we face two fundamental challenges: how to relate inputs to changes in the organoid’s architecture and/or functionality, and how to relate these changes to certain outputs (e.g., an improvement in playing Pong). Answering these questions will require interdisciplinary tools integrating machine learning, statistics, signal processing, information theory, and optimization.
Fortunately, the design of algorithms that can perform these operations is facilitated by the theoretical neuroscience literature. This broad body of work provides a framework for assessing and guiding learning in brains, which can be extended to OI.
Could organoid intelligence drive progress in neuroscience?
Fully developed OI would provide unprecedented understanding of the human brain in health and disease. For example, we could study neurodegenerative and neurodevelopmental conditions by comparing brain organoids developed from skin samples of patients and healthy individuals. This will help identify risk factors as well as provide a model for screening new drugs for toxicity and monitoring their long-term effects on cognitive function. This approach holds great promise for conditions such as Parkinson’s and Alzheimer’s disease, which afflict millions of people globally and remain incurable despite intense research efforts.
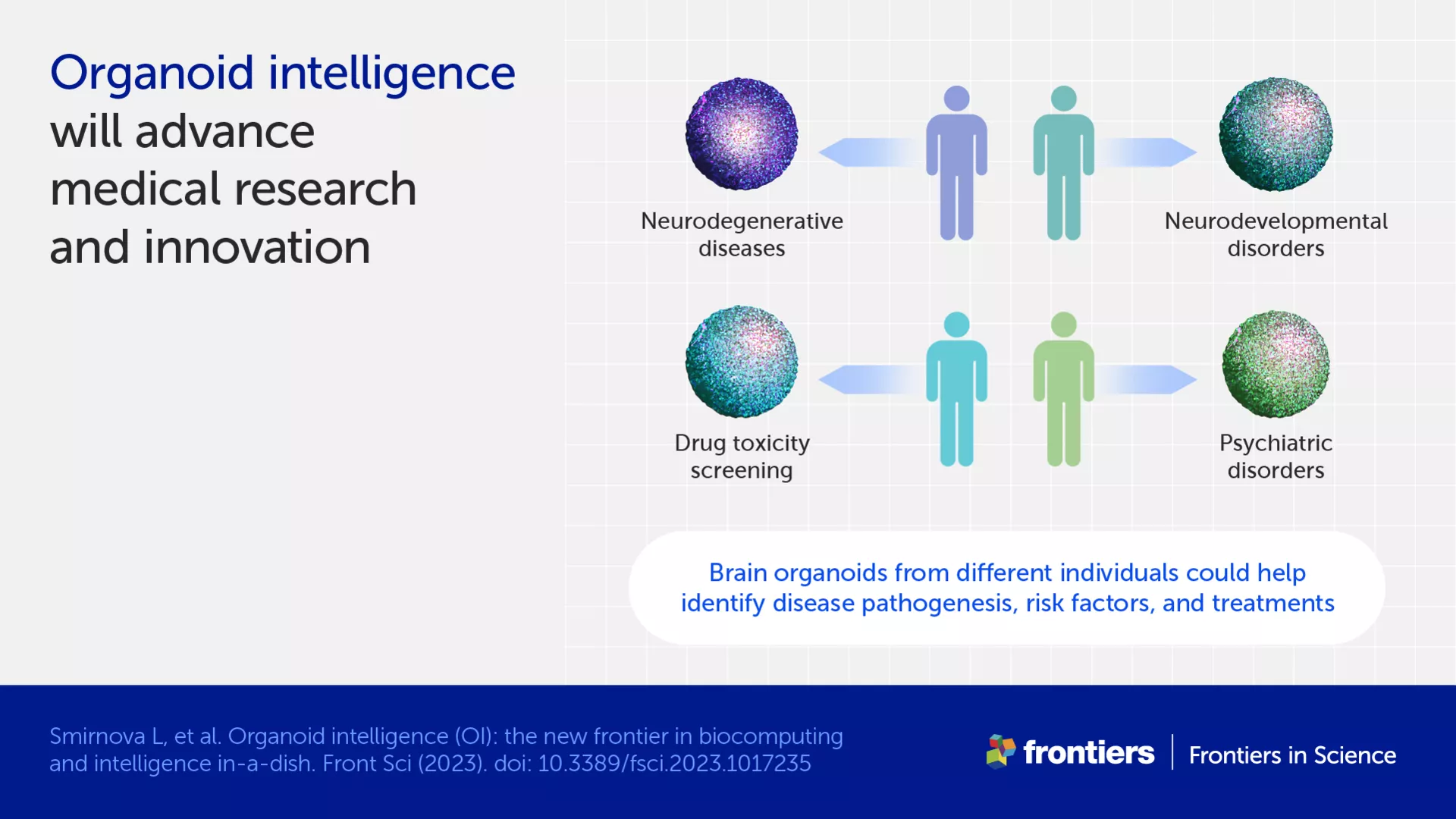
How should the ethical implications of organoid intelligence be handled?
Creating human brain organoids that can learn, remember, and interact with their environment raises complex ethical questions. Could they develop consciousness, even in a rudimentary form? Could they experience pain or suffering? And what rights would people have with respect to brain organoids made from their cells?
A key part of our vision is to ensure that OI develops in an ethically and socially responsible manner. We promote an ‘embedded ethics‘ approach, where teams of ethicists, researchers, and members of the public collectively identify, discuss, and address ethical issues in a continuous process as the research evolves. This process will also include incorporating observations from related fields, such as insights regarding the neurobiological basis of consciousness. As a whole, this approach will keep societal stakeholders informed about specific organoid intelligence applications and integrate their input as the technology evolves.
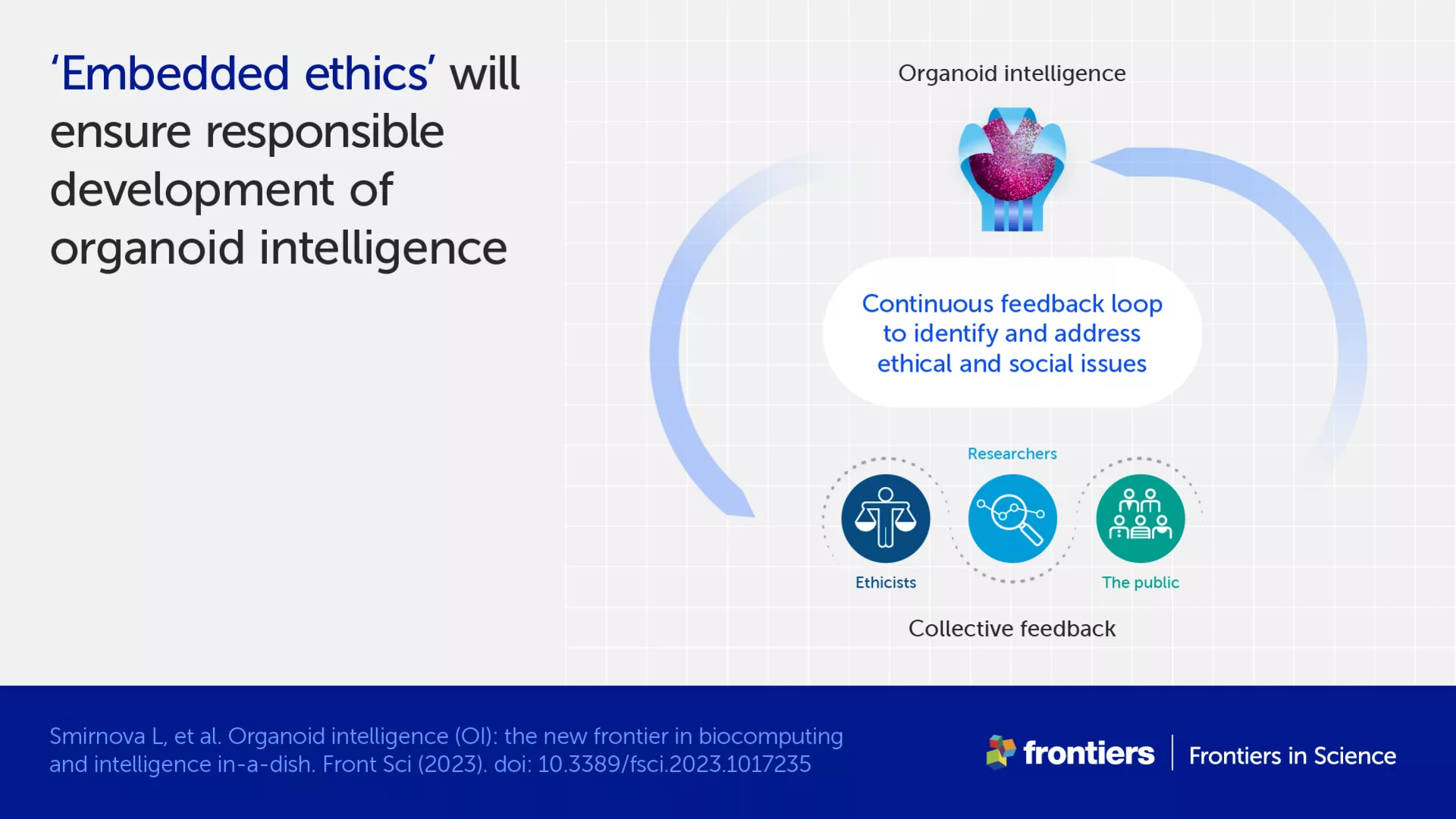