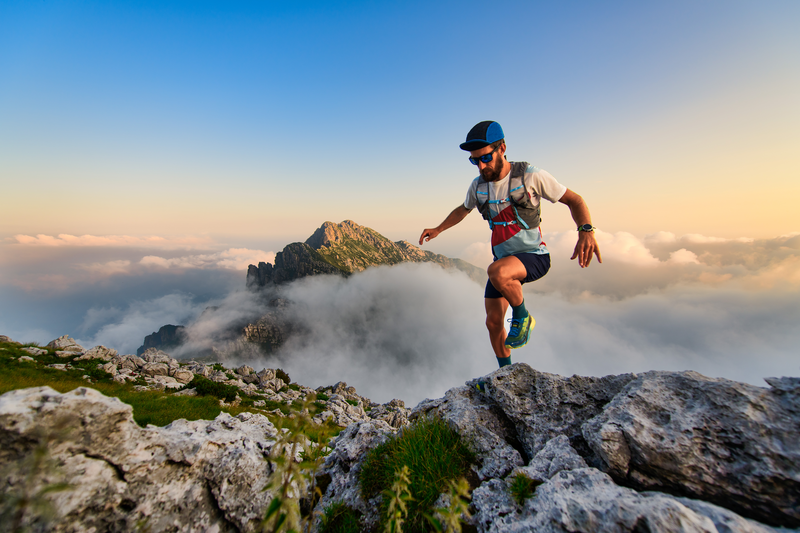
94% of researchers rate our articles as excellent or good
Learn more about the work of our research integrity team to safeguard the quality of each article we publish.
Find out more
ORIGINAL RESEARCH article
Front. Public Health , 08 December 2023
Sec. Infectious Diseases: Epidemiology and Prevention
Volume 11 - 2023 | https://doi.org/10.3389/fpubh.2023.1255969
This article is part of the Research Topic Characteristics and Prevention of the Emerging Avian Influenza A Viruses in Birds and Mammals View all 8 articles
Introduction: The continued emergence of human infections of H9N2 avian influenza virus (AIV) poses a serious threat to public health. The prevalent Y280/G9 lineage of H9N2 AIV in Chinese poultry can directly bind to human receptors, increasing the risk of spillover infections to humans. Since 2013, the number of human cases of H9N2 avian influenza has been increasing continuously, and in 2021, China reported the highest number of human cases, at 25.
Methods: In this study, we analyzed the age, geographic, temporal, and sex distributions of humans with H9N2 avian influenza in 2021 using data from the National Influenza Center (Beijing, China). We also conducted evolutionary, gene homology, and molecular characterization analyses of the H9N2 AIVs infecting humans.
Results: Our findings show that children under the age of 12 accounted for 80% of human cases in 2021, and females were more frequently affected than males. More cases occurred in winter than in summer, and most cases were concentrated in southern China. Human-infecting H9N2 viruses showed a high level of genetic homology and belonged to the prevalent G57 genotype. Several additional α2,6-SA-binding sites and sites of mammalian adaptation were also identified in the genomes of human-infecting H9N2 viruses.
Discussion: Therefore, continuous monitoring of H9N2 AIV and the implementation of further measures to control the H9N2 virus in poultry are essential to reduce the interspecies transmission of the virus.
Since the 1990s, the H9N2 avian influenza virus (AIV) has been detected in poultry and mammalian species, including chickens, ducks, smaller poultry, and pigs, in China (1–3). The virus has continuously evolved, resulting in multiple lineages, of which the G1, G9/Y280, and Y439 lineages continue to circulate in poultry (4, 5). The G9/Y280 lineage of H9N2 AIV has predominated in China in recent years. Since the emergence of the G57 genotype, which is better adapted to chickens, it has become predominant and has caused widespread outbreaks (1, 6).
Ongoing epidemics of H9N2 AIV in poultry increase the risk of spillover infections in humans. The first case of human H9N2 AIV infection was detected in Hong Kong SAR, China, in 1998. Since 2013, there have been increasing reports of human infections of H9N2 AIV (7). Several studies have shown that the majority of recent avian-origin H9N2 AIVs have a strong binding affinity for a human respiratory receptor (7, 8), and serological surveys have shown higher positivity among poultry-associated workers (9, 10). Therefore, assessing the pandemic risk of current H9N2 AIV genotypes is important and urgent.
In this study, we collected and analyzed data on human H9N2 AIV infections in China in 2021. We examined the epidemiological and genetic characteristics of the virus to extend our understanding of human infections and the risks they pose.
In China, all laboratory-diagnosed cases of H9N2 influenza in 2021 were reported through the national surveillance system. Patients whose respiratory specimens tested positive for H9 and N2 with real-time reverse transcription (RT)–PCR were confirmed as infected with H9N2. Demographic and epidemiological data on these cases of H9N2 influenza were collected with standard forms, including information on age, sex, and place of residence.
The National Health Commission of the People’s Republic of China has deemed that collecting data on each H9N2-infected patient is part of ongoing public health investigations into emerging infectious diseases. Therefore, our Institutional Review Board waived the requirement for formal ethical approval.
Original samples from human patients were collected for an H9N2 subtype analysis with real-time RT–PCR by the local CDCs (Ct less than 38 were considered positive, while those with Ct values between 38 and 40 were subjected to repeat experiments due to the requirement for confirmation. Samples with Ct values greater than 40 were regarded as negative). The H9N2 virus was isolated from positive samples in a biosafety level 2 laboratory. Nine-day-old specific-pathogen-free embryonated chicken eggs were inoculated with an aliquot (0.2 mL) of the original sample. After incubation at 37°C for 48 h, we harvested the egg allantoic fluid, extracted the RNA from it, and sequenced the genome original samples from human patients and viral samples (11).
We used the Illumina next-generation sequencing (NGS) technology on the MiSeq platform (Illumina, Inc., San Diego, CA, United States) to sequence the human H9N2 influenza virus. Following the acquisition of the viral sequences, we conducted a series of analysis steps. First, we checked the quality of the sequences, excluding any potential sequencing errors or contamination. We then assembled high-quality reads using bioinformatic tools like Velvet (version 1.2.10) and Newbler (version 2.5) to align the viral sequences with known reference sequences of H9N2 viruses in the Global Initiative on Sharing All Influenza Data (GISAID).1 This involved detecting single-nucleotide variations (SNVs), insertion/deletion of variants, and identifying variable sites that could potentially lead to changes in protein sequences. Bowtie 2 (version 2.1.0) was used to map the reads using sequences with the maximum similarity.
We obtained sequences from 16 human isolates and downloaded H9N2 AIV reference sequences from GISAID: 37 sequences of polymerase basic protein 1 (PB1), 34 of polymerase basic protein 2 (PB2), 34 of neuraminidase (NA), 55 of hemagglutinin (HA), 35 of matrix protein (M), 37 of nucleoprotein (NP), 38 of non-structural protein (NS), and 35 of polymerase (PA). We constructed maximum likelihood phylogenetic trees for each gene segment of the selected influenza viruses with the GTR model in MEGA 7.0, with 1,000 bootstrap replicates.
The first reported case of human infection with H9N2 AIV was in China in 1998 (12), and since then, continuously increasing numbers of human infections have been recorded. In 2021, China reported a record number of 25 cases of human H9N2 AIV infection. All reported patients presented with flu-like symptoms, except patients who died, in whom the infection was associated with underlying health conditions. A seasonal pattern was observed, with more cases occurring in winter than in summer. Specifically, 16 of 25 cases (64%) occurred in January, February, November, and December 2021 (Figure 1A).
Figure 1. Distribution of cumulative reported cases of human H9N2 avian influenza virus infection in China from January to December 2021 (A). Age and sex distributions of cases of human H9N2 avian influenza in 2021. Black, female; Red, male (B). Regional distribution of human cases of H9N2 avian influenza virus in China in 2021. The darker the color, the greater the number of infections (C).
The 25 infections were predominantly in children. The median patient age was 5 years (interquartile range: 2.5–9.5). The subdivided age groups showed that among the infected individuals, six (24%) were infants aged 1–2 years, eight (32%) were children aged 3–6 years, six (24%) were children aged 7–12 years, one (4%) was a teenager aged 13–18 years, three (12%) were adults, and one (4%) was an older adult (Figure 1B). These data indicate that the majority of H9N2 AIV infections occurred in children under 12 years old, who accounted for 80% (20/25) of cases. Of all the patients, 8 were male and 17 female subjects, resulting in a male-to-female ratio of 1:2.125.
These patients came from 11 provinces, municipalities, or autonomous regions (Guangdong, Hubei, Jiangsu, Guangxi, Guizhou, Fujian, Sichuan, Anhui, Jiangxi, Hunan, and Shanxi), and most were from Southern China (96%, 24/25 patients). Guangdong Province had the highest number of cases, with six patients (Figure 1C).
A total of 16 viruses were isolated from 25 patients. Phylogenetic analysis of the HA gene showed that they all belonged to the G9/Y280 lineage. The NA and internal genes of the human isolates were located in the same clades as those of the representative strain of the G57 genotype, A/chicken/Zhejiang/HJ/2007 (Figure 2; Supplementary Figures S1–S6). These human isolates showed high genetic diversity and shared 91.8–99.2% nucleotide homology in HA, 92.8–99.8% in NA, 93.4–99.7% in PB2, 92.1–99.8% in PB1, 93.9–99.5% in PA, 94.8–99.5% in NP, 95.1–99.8% in MP, and 93.9–99.5% in NS. We also categorized the 16 human H9N2 strains sequences into six subgenotypes (Figure 3). The eight gene segments of the H9N2 viruses, represented by horizontal bars are, from top to bottom, PB2, PB1, PA, HA, NP, NA, M, and NS. Each color represents a distinct origin.
Figure 2. Maximum likelihood phylogenetic tree of the HA gene (A) and NA gene (B). The H9N2 virus infections in Chinese people in 2021 analyzed in this study are marked in red.
Figure 3. The 16 human H9N2 viruses. The eight gene segments of the H9N2 viruses, represented by horizontal bars are, from top to bottom, PB2, PB1, PA, HA, NP, NA, M, and NS. Each different color represents a distinct branch.
All 16 human viruses had leucine at position 226 (H3 numbering) of HA, which may increase the virus’s binding affinity for α-2,6 sialic acid (α2,6-SA), the human AIV receptor. Other mutations, such as 155 T and 190 T/V (13), which may also increase viral binding to the human receptor, were also found in some of the isolates (Table 1; Supplementary Figures S7, S8).
Table 1. Inventory of major molecular markers affecting biological characteristics of avian influenza A viruses.
Residues previously identified as enhancing the polymerase activity, virulence, and transmission of AIVs were examined. One virus had PB2 627 K/E, whereas five had 627 V, which may confer a virulent phenotype on H9N2 AIV in mice (36). Eleven viruses had PB2 292 V and all 16 had PB2 588 V, which are markers of mammalian adaptation and enhance the viral polymerase activity, replication, and virulence in mammals (14, 15). Nine viruses in this study had PB2 702R, which is a species-specific residue of human influenza viruses (37).
We examined mutation sites associated with reduced drug susceptibility and identified M2-S31N in 16 viral strains, which confers resistance to adamantanes (38). No drug resistance mutations were found for NA inhibitors or PA inhibitors, such as NA-136 K, NA-292 K, PA-38 M/T, or PA-37 T.
In this study, we analyzed the human H9N2 AIV infections reported in China in 2021, the year in which the highest number of H9N2 cases was recorded to date. We examined the epidemiological and genetic characteristics of the patients and isolates. H9N2 infections occurred most frequently in winter in Southern China and mainly occurred in children under 12 years old, who accounted for 80% of all cases. More female subjects were infected than male subjects. Therefore, the prevalence of H9N2 AIVs must be closely monitored to prevent cross-species transmission.
Host barriers largely restrict the cross-species transmission of AIVs. In recent years, the α2,6-SA-binding ability of H9N2 has increased continuously, and the predominant strains that have emerged show dual receptor binding or preferentially bind α2,6-SA (8, 39). HA Q226 L plays a critical role in receptor binding and was detected in all 16 viruses in this study. Moreover, increased α2,6-SA-binding sites, such as 155 T, 183 N, and 190 V, were also found in the H9N2 viruses that infected humans. Although most H9N2 viruses do not have well-known mammalian adaptation markers, such as PB2 627 K and 701 N, several amino acid residues that facilitate mammalian adaptation were detected, including PB2-588 V, PB1-368 V, PA-356R, and PA-409 N.
Throughout its evolution, the genetic diversity of H9N2 AIV has increased, with mutations that adapt the virus to mammalian hosts and may increase the risk of human infection. High levels of H9N2 AIV among birds, especially poultry, may also increase the opportunities for virus spillover and human infections. Serious efforts are required to constrain the virus in poultry. Continued monitoring of H9N2 AIV, including its close genetic surveillance and phenotypic characterization in animal models, should be incorporated into risk assessment strategies.
Since 2013, the number of human cases of H9N2 avian influenza has been steadily increasing, and in 2021, China reported the highest number of human cases, at 25. Children under the age of 12 accounted for 80% of human cases in 2021, and female subjects were more frequently affected than male subjects. More cases occurred in winter than in summer, and most cases were concentrated in Southern China. Human-infecting H9N2 viruses showed a high level of genetic homology and belonged to the prevalent G57 genotype. Continuous monitoring of H9N2 avian influenza viruses and further measures to control the H9N2 virus in poultry are essential to reduce the interspecies transmission of the virus.
The datasets presented in this study can be found in online repositories. The names of the repository/repositories and accession number(s) can be found in the article/Supplementary material.
MT: Data curation, Visualization, Writing – original draft, Methodology. XZ: Data curation, Methodology, Writing – review & editing. YX: Investigation, Writing – review & editing. XL: Data curation, Methodology, Writing – review & editing. JL: Methodology, Writing – review & editing. JY: Methodology, Writing – review & editing. LY: Validation, Writing – review & editing. DW: Funding acquisition, Writing – review & editing, Supervision.
The author(s) declare financial support was received for the research, authorship, and/or publication of this article. The study was supported by the National Key Research and Development Program of China (2022YFC2303800) and the National Nature Science Foundation of China (81971941 and 81961128002).
The authors sincerely thank the laboratories that shared the H9 AIV sequences from the GISAID and GenBank databases. This article is solely the responsibility of the authors and does not represent the views of the China CDC or other organizations.
The authors declare that the research was conducted in the absence of any commercial or financial relationships that could be construed as a potential conflict of interest.
All claims expressed in this article are solely those of the authors and do not necessarily represent those of their affiliated organizations, or those of the publisher, the editors and the reviewers. Any product that may be evaluated in this article, or claim that may be made by its manufacturer, is not guaranteed or endorsed by the publisher.
The Supplementary material for this article can be found online at: https://www.frontiersin.org/articles/10.3389/fpubh.2023.1255969/full#supplementary-material
1. Li, C, Wang, S, Bing, G, Carter, RA, Wang, Z, Wang, J, et al. Genetic evolution of influenza H9N2 viruses isolated from various hosts in China from 1994 to 2013. Emerg Microbes Infect. (2017) 6:e106:1–11. doi: 10.1038/emi.2017.94
2. Homme, PJ, and Easterday, BC. Avian influenza virus infections. I. Characteristics of influenza A-Turkey-Wisconsin-1966 virus. Avian Dis. (1970) 14:66–74. doi: 10.2307/1588557
3. Li, C, Yu, K, Tian, G, Yu, D, Liu, L, Jing, B, et al. Evolution of H9N2 influenza viruses from domestic poultry in mainland China. Virology. (2005) 340:70–83. doi: 10.1016/j.virol.2005.06.025
4. Liu, Q, Zhao, L, Guo, Y, Zhao, Y, Li, Y, Chen, N, et al. Antigenic evolution characteristics and immunological evaluation of H9N2 avian influenza viruses from 1994–2019 in China. Viruses. (2022) 14:14. doi: 10.3390/v14040726
5. Gu, M, Xu, L, Wang, X, and Liu, X. Current situation of H9N2 subtype avian influenza in China. Vet Res. (2017) 48:49. doi: 10.1186/s13567-017-0453-2
6. Zou, S, Zhang, Y, Li, X, Bo, H, Wei, H, Dong, L, et al. Molecular characterization and receptor binding specificity of H9N2 avian influenza viruses based on poultry-related environmental surveillance in China between 2013 and 2016. Virology. (2019) 529:135–43. doi: 10.1016/j.virol.2019.01.002
7. Song, W, and Qin, K. Human-infecting influenza a (H9N2) virus: a forgotten potential pandemic strain? Zoonoses Public Health. (2020) 67:203–12. doi: 10.1111/zph.12685
8. Li, X, Shi, J, Guo, J, Deng, G, Zhang, Q, Wang, J, et al. Genetics, receptor binding property, and transmissibility in mammals of naturally isolated H9N2 avian influenza viruses. PLoS Pathog. (2014) 10:e1004508. doi: 10.1371/journal.ppat.1004508
9. Ma, C, Cui, S, Sun, Y, Zhao, J, Zhang, D, Zhang, L, et al. Avian influenza a (H9N2) virus infections among poultry workers, swine workers, and the general population in Beijing, China, 2013–2016: a serological cohort study. Influenza Other Respir Viruses. (2019) 13:415–25. doi: 10.1111/irv.12641
10. Ma, MJ, Zhao, T, Chen, SH, Xia, X, Yang, XX, Wang, GL, et al. Avian influenza a virus infection among workers at Live Poultry Markets, China, 2013–2016. Emerg Infect Dis. (2018) 24:1246–56. doi: 10.3201/eid2407.172059
11. Zhu, W, Li, X, Dong, J, Bo, H, Liu, J, Yang, J, et al. Epidemiologic, clinical, and genetic characteristics of human infections with influenza a(H5N6) viruses, China. Emerg Infect Dis. (2022) 28:1332–44. doi: 10.3201/eid2807.212482
12. Peacock, THP, James, J, Sealy, JE, and Iqbal, M. A global perspective on H9N2 avian influenza virus. Viruses. (2019) 11:11. doi: 10.3390/v11070620
13. Teng, Q, Xu, D, Shen, W, Liu, Q, Rong, G, Li, X, et al. A single mutation at position 190 in hemagglutinin enhances binding affinity for human type sialic acid receptor and replication of H9N2 avian influenza virus in mice. J Virol. (2016) 90:9806–25. doi: 10.1128/jvi.01141-16
14. Gao, W, Zu, Z, Liu, J, Song, J, Wang, X, Wang, C, et al. Prevailing I292V PB2 mutation in avian influenza H9N2 virus increases viral polymerase function and attenuates IFN-β induction in human cells. J Gen Virol. (2019) 100:1273–81. doi: 10.1099/jgv.0.001294
15. Xiao, C, Ma, W, Sun, N, Huang, L, Li, Y, Zeng, Z, et al. PB2-588 V promotes the mammalian adaptation of H10N8, H7N9 and H9N2 avian influenza viruses. Sci Rep. (2016) 6:19474. doi: 10.1038/srep19474
16. Liu, K, Guo, Y, Zheng, H, Ji, Z, Cai, M, Gao, R, et al. Enhanced pathogenicity and transmissibility of H9N2 avian influenza virus in mammals by hemagglutinin mutations combined with PB2-627K. Virol Sin. (2023) 38:47–55. doi: 10.1016/j.virs.2022.09.006
17. Czudai-Matwich, V, Otte, A, Matrosovich, M, Gabriel, G, and Klenk, HD. PB2 mutations D701N and S714R promote adaptation of an influenza H5N1 virus to a mammalian host. J Virol. (2014) 88:8735–42. doi: 10.1128/jvi.00422-14
18. Tombari, W, Nsiri, J, Larbi, I, Guerin, JL, and Ghram, A. Genetic evolution of low pathogenecity H9N2 avian influenza viruses in Tunisia: acquisition of new mutations. Virol J. (2011) 8:467. doi: 10.1186/1743-422x-8-467
19. Song, W, Wang, P, Mok, BW, Lau, SY, Huang, X, Wu, WL, et al. The K526R substitution in viral protein PB2 enhances the effects of E627K on influenza virus replication. Nat Commun. (2014) 5:5509. doi: 10.1038/ncomms6509
20. Liu, Q, Qiao, C, Marjuki, H, Bawa, B, Ma, J, Guillossou, S, et al. Combination of PB2 271A and SR polymorphism at positions 590/591 is critical for viral replication and virulence of swine influenza virus in cultured cells and in vivo. J Virol. (2012) 86:1233–7. doi: 10.1128/jvi.05699-11
21. Ren, X, Yang, F, Hu, Y, Zhang, T, Liu, L, Dong, J, et al. Full genome of influenza a (H7N9) virus derived by direct sequencing without culture. Emerg Infect Dis. (2013) 19:1881–4. doi: 10.3201/eid1911.130664
22. Li, C, Hatta, M, Watanabe, S, Neumann, G, and Kawaoka, Y. Compatibility among polymerase subunit proteins is a restricting factor in reassortment between equine H7N7 and human H3N2 influenza viruses. J Virol. (2008) 82:11880–8. doi: 10.1128/jvi.01445-08
23. Waters, K, Wan, HJ, Han, L, Xue, J, Ykema, M, Tao, YJ, et al. Variations outside the conserved motifs of PB1 catalytic active site may affect replication efficiency of the RNP complex of influenza a virus. Virology. (2021) 559:145–55. doi: 10.1016/j.virol.2021.04.001
24. Marjuki, H, Scholtissek, C, Franks, J, Negovetich, NJ, Aldridge, JR, Salomon, R, et al. Three amino acid changes in PB1-F2 of highly pathogenic H5N1 avian influenza virus affect pathogenicity in mallard ducks. Arch Virol. (2010) 155:925–34. doi: 10.1007/s00705-010-0666-4
25. Xu, G, Zhang, X, Gao, W, Wang, C, Wang, J, Sun, H, et al. Prevailing PA mutation K356R in avian influenza H9N2 virus increases mammalian replication and pathogenicity. J Virol. (2016) 90:8105–14. doi: 10.1128/jvi.00883-16
26. Yamayoshi, S, Yamada, S, Fukuyama, S, Murakami, S, Zhao, D, Uraki, R, et al. Virulence-affecting amino acid changes in the PA protein of H7N9 influenza a viruses. J Virol. (2014) 88:3127–34. doi: 10.1128/jvi.03155-13
27. Clements, AL, Sealy, JE, Peacock, TP, Sadeyen, JR, Hussain, S, Lycett, SJ, et al. Contribution of segment 3 to the acquisition of virulence in contemporary H9N2 avian influenza viruses. J Virol. (2020) 94:94. doi: 10.1128/jvi.01173-20
28. Timofeeva, TA, Sadykova, GK, Lomakina, NF, Gambaryan, AS, Rudneva, IA, Timofeeva, EB, et al. The effect of I155T, K156Q, K156E and N186K mutations in hemagglutinin on the virulence and reproduction of influenza a/H5N1 viruses. Mol Biol. (2020) 54:861–9. doi: 10.1134/s0026893320060126
29. Wan, H, and Perez, DR. Amino acid 226 in the hemagglutinin of H9N2 influenza viruses determines cell tropism and replication in human airway epithelial cells. J Virol. (2007) 81:5181–91. doi: 10.1128/jvi.02827-06
30. Xiong, X, Martin, SR, Haire, LF, Wharton, SA, Daniels, RS, Bennett, MS, et al. Receptor binding by an H7N9 influenza virus from humans. Nature. (2013) 499:496–9. doi: 10.1038/nature12372
31. Obadan, AO, Santos, J, Ferreri, L, Thompson, AJ, Carnaccini, S, Geiger, G, et al. Flexibility in vitro of amino acid 226 in the receptor-binding site of an H9 subtype influenza a virus and its effect in vivo on virus replication, tropism, and transmission. J Virol. (2019) 93:e02011-18. doi: 10.1128/jvi.02011-18
32. Obadan, AO, Kimble, BJ, Rajao, D, Lager, K, Santos, JJS, Vincent, A, et al. Replication and transmission of mammalian-adapted H9 subtype influenza virus in pigs and quail. J Gen Virol. (2015) 96:2511–21. doi: 10.1099/vir.0.000190
33. Kimble, JB, Sorrell, E, Shao, H, Martin, PL, and Perez, DR. Compatibility of H9N2 avian influenza surface genes and 2009 pandemic H1N1 internal genes for transmission in the ferret model. Proc Natl Acad Sci U S A. (2011) 108:12084–8. doi: 10.1073/pnas.1108058108
34. Sorrell, EM, Wan, H, Araya, Y, Song, H, and Perez, DR. Minimal molecular constraints for respiratory droplet transmission of an avian-human H9N2 influenza a virus. Proc Natl Acad Sci U S A. (2009) 106:7565–70. doi: 10.1073/pnas.0900877106
35. Pielak, RM, Schnell, JR, and Chou, JJ. Mechanism of drug inhibition and drug resistance of influenza a M2 channel. Proc Natl Acad Sci U S A. (2009) 106:7379–84. doi: 10.1073/pnas.0902548106
36. Taft, AS, Ozawa, M, Fitch, A, Depasse, JV, Halfmann, PJ, Hill-Batorski, L, et al. Identification of mammalian-adapting mutations in the polymerase complex of an avian H5N1 influenza virus. Nat Commun. (2015) 6:7491. doi: 10.1038/ncomms8491
37. Finkelstein, DB, Mukatira, S, Mehta, PK, Obenauer, JC, Su, X, Webster, RG, et al. Persistent host markers in pandemic and H5N1 influenza viruses. J Virol. (2007) 81:10292–9. doi: 10.1128/jvi.00921-07
38. WHO. Available at: https://www.who.int/.
Keywords: H9N2, avian influenza virus, poultry, zoonotic, human infections
Citation: Tan M, Zeng X, Xie Y, Li X, Liu J, Yang J, Yang L and Wang D (2023) Reported human infections of H9N2 avian influenza virus in China in 2021. Front. Public Health. 11:1255969. doi: 10.3389/fpubh.2023.1255969
Received: 10 July 2023; Accepted: 15 November 2023;
Published: 08 December 2023.
Edited by:
Daniel Roberto Perez, University of Georgia, United StatesReviewed by:
Victor C. Huber, University of South Dakota, United StatesCopyright © 2023 Tan, Zeng, Xie, Li, Liu, Yang, Yang and Wang. This is an open-access article distributed under the terms of the Creative Commons Attribution License (CC BY). The use, distribution or reproduction in other forums is permitted, provided the original author(s) and the copyright owner(s) are credited and that the original publication in this journal is cited, in accordance with accepted academic practice. No use, distribution or reproduction is permitted which does not comply with these terms.
*Correspondence: Dayan Wang, d2FuZ2RheWFuQGl2ZGMuY2hpbmFjZGMuY24=
Disclaimer: All claims expressed in this article are solely those of the authors and do not necessarily represent those of their affiliated organizations, or those of the publisher, the editors and the reviewers. Any product that may be evaluated in this article or claim that may be made by its manufacturer is not guaranteed or endorsed by the publisher.
Research integrity at Frontiers
Learn more about the work of our research integrity team to safeguard the quality of each article we publish.