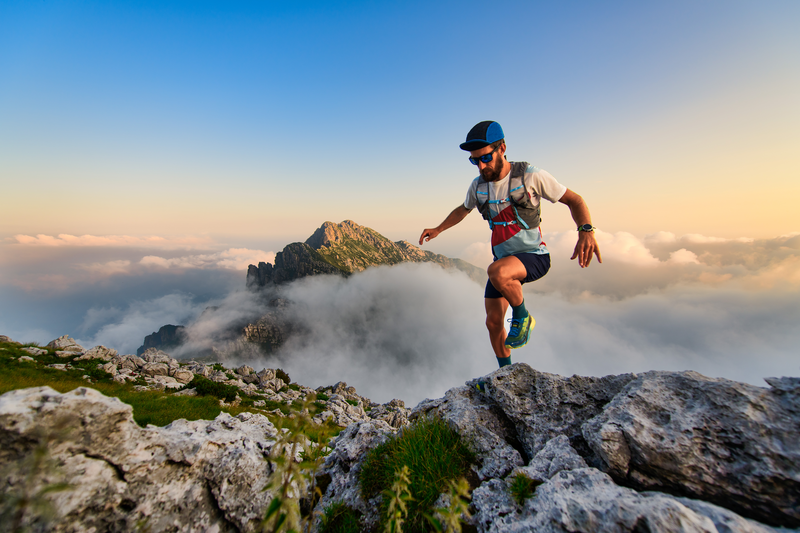
94% of researchers rate our articles as excellent or good
Learn more about the work of our research integrity team to safeguard the quality of each article we publish.
Find out more
ORIGINAL RESEARCH article
Front. Psychol.
Sec. Movement Science
Volume 16 - 2025 | doi: 10.3389/fpsyg.2025.1547493
This article is part of the Research Topic Football training and competition View all articles
The final, formatted version of the article will be published soon.
You have multiple emails registered with Frontiers:
Please enter your email address:
If you already have an account, please login
You don't have a Frontiers account ? You can register here
The aim of this study was to assess the effectiveness of a video-based perceptual training programme designed to enhance anticipation skills in professional female football players. The video reference task was used to test participants in pre-and post-tests, where they predicted the ball's arrival location during corners. Participants were evenly divided into an experimental group, which received training in the task between the pre-and post-tests using a progressive temporal occlusion method, and a control group, which received no training. The rate of correct responses, response time, and confidence scores were analysed in the reference task, as well as performance on corners in real matches, to assess the expected transfer of learning to the field.The results revealed that the experimental group, which underwent training, significantly improved their precision in predicting the ball's landing zone after the intervention, with their accuracy score increasing from 54% to 68% (p < 0.05, ƞ² = 0.60). Additionally, their response time decreased from 3.2 seconds to 2.4 seconds (p < .05, ƞ² = 0.48), and their confidence score improved from 3 to 3.8 (p < .05; ƞ² = 0.76). This effect slightly diminished after a 6-month retention interval but remained significantly higher than at the pre-test. Furthermore, we observed that the performance of professional football players during corners in actual matches improved, suggesting a positive effect of the video-based perceptual-cognitive training.
Keywords: Corner kicks, perceptual training, Football, Expertise, Temporal occultation
Received: 18 Dec 2024; Accepted: 11 Feb 2025.
Copyright: © 2025 Libreau and BENGUIGUI. This is an open-access article distributed under the terms of the Creative Commons Attribution License (CC BY). The use, distribution or reproduction in other forums is permitted, provided the original author(s) or licensor are credited and that the original publication in this journal is cited, in accordance with accepted academic practice. No use, distribution or reproduction is permitted which does not comply with these terms.
* Correspondence:
Clement Libreau, Université de Caen Normandie, Caen, France
Disclaimer: All claims expressed in this article are solely those of the authors and do not necessarily represent those of their affiliated organizations, or those of the publisher, the editors and the reviewers. Any product that may be evaluated in this article or claim that may be made by its manufacturer is not guaranteed or endorsed by the publisher.
Research integrity at Frontiers
Learn more about the work of our research integrity team to safeguard the quality of each article we publish.