- 1National Engineering Research Center of Ophthalmology and Optometry, Eye Hospital, Wenzhou Medical University, Wenzhou, China
- 2State Key Laboratory of Ophthalmology, Optometry and Visual Science, Eye Hospital, Wenzhou Medical University, Wenzhou, China
- 3Shaanxi Eye Hospital, Xi'an People’s Hospital (Xi'an Fourth Hospital), Affiliated People's Hospital of Northwest University, Xi'an, China
- 4Department of Medical Information Management, First Affiliated Hospital of Xi'an Jiaotong University, Xi'an, Shaanxi, China
- 5McGill Vision Research, Department of Ophthalmology and Visual Sciences, McGill University, Montreal, QC, Canada
Purpose: The study aimed to explore potential discrepancies in contrast sensitivity in the ON and OFF visual pathways among individuals with amblyopia compared to controls.
Methods: Eleven adult amblyopes (26.2 ± 4.4 [SD] years old) and 10 controls (24.6 ± 0.8 years old) with normal or corrected to normal visual acuity (logMAR VA ≤ 0) participated in this study. Using the quick contrast sensitivity function (qCSF) algorithm, we measured balanced CSF which would stimulate the ON and OFF pathways unselectively, and CSFs for increments and decrements that would selectively stimulate the ON and OFF visual pathways. Contrast sensitivity and area under log contrast sensitivity function were extracted for statistical analysis.
Results: For the balanced CSF, we found significant interocular differences in sensitivity and area under log contrast sensitivity function in both amblyopes [F(1,10) = 74.992, p < 0.001] and controls [F(1,9) = 35.6, p < 0.001], while such differences were more pronounced in amblyopes than in controls. For increment and decrement CSFs, we found that the increment sensitivity (p = 0.038) and area under log contrast sensitivity function (p = 0.001) were significantly lower than the decrement in the amblyopic eye. Such differences between increment and decrement CSFs were not observed in the fellow eye of the amblyopes or in the controls.
Conclusion: There is a subtle difference in the contrast sensitivity of the amblyopic eye when exposed to stimulation in the ON and OFF pathways.
Introduction
Amblyopia, caused by abnormal visual experience (such as strabismus, anisometropia, or form-deprivation) during visual development (DeSantis, 2014) affects approximately 1–5% of the general population worldwide (Webber and Wood, 2005; Xiao et al., 2015). It has been shown that amblyopes not only have reduced visual acuity but also have general deficits in several visual processes, such as contrast sensitivity (Hess and Howell, 1977; Bradley and Freeman, 1981; Sjöstrand, 1981; Pang et al., 2019), binocular combination (Ding et al., 2013; Zhou et al., 2016; Mao et al., 2020), stereo vision (Levi et al., 2015), contour integration (Hamm et al., 2014), color perception (Davis et al., 2008), and so on. Consequently, amblyopia is now recognized as a developmental visual disorder of the central nervous system.
Most of the aforementioned studies measured the visual deficits of amblyopia with stimuli modulated uniformly around a mean value, typically featuring both light and dark bars in a sinusoidal profile (Ding et al., 2013; Ding and Levi, 2014; Zhou et al., 2016; Mao et al., 2020). This approach assumes that stimuli modulated in this fashion are detected by the overall amplitude of the stimulus and from what we know of visual processing in both retina and cortex, this would involve the equal contribution of both ON and OFF visual channels (Hubel and Wiesel, 1961; Schiller, 1982; Schiller et al., 1986; Schiller, 1992; Dolan and Schiller, 1994). Recently, Pons et al. (2019) studied the ON–OFF pathways sensitivity differences in amblyopia using an acuity-based paradigm. They found that there was a significant dark–light difference in accuracy and reaction time in the amblyopic eye of amblyopes. Any dark–light difference was very subtle in the fellow eye of amblyopes and normal controls. Their results indicate that amblyopia affects the ON pathway more than the OFF at suprathreshold contrast. Their measurements involve stimuli with high contrast and a discrimination task that would be expected to target extra striate function (Ziemba et al., 2019). As visual processing in the brain is very hierarchical (Grill-Spector and Malach, 2004), our question is whether the difference they observe between ON and OFF pathways could originate earlier in the visual processing stream. Indeed, studies have shown an overrepresentation of the OFF visual responses in primary visual cortex (Jin et al., 2008; Yeh et al., 2009; Xing et al., 2010). OFF processing is faster (Komban et al., 2014); and, in particular, cortical neurons are more strongly driven by darks at low spatial frequencies (Kremkow et al., 2014). Psychophysically, the most comprehensive way to understand low-level visual function is by measuring the contrast sensitivity function (Jindra and Zemon, 1989). By measuring contrast sensitivity across various spatial frequencies, we could get insight into which early processing channels could be involved in this difference. Or if we do not observe any difference, conclude a higher-order deficit. Hence, we wanted to answer two questions: (i) could the ON-selective pattern that they observed also exist at the early pathway in processing contrast sensitivity, and (ii) if so, are ON-selective deficits broad-based or spatially tuned? In current practice, the assessment of amblyopia relies mainly on high-contrast OFF stimuli, i.e., visual acuity. However, if we find ON pathway impairments at low-level visual processing stages, this may offer a novel avenue for amblyopia treatment and evaluation. For instance, selectively enhancing the ON pathway at low-levels to improve the sensitivity.
To answer these questions, we measured the contrast sensitivity of the ON and OFF visual pathways in amblyopic and control adults. Contrast sensitivity function (CSF), which describes how contrast sensitivity changes as a function of the stimulus spatial frequency (SF), determines the quality of the visual input to higher visual areas for the processing of more global and shape properties. It has been the gold standard in amblyopia research for determining whether higher-order deficits are simply the consequence of lower-order limitations (e.g., the use of a constant suprathreshold contrast). Contrast sensitivity is not only a more sensitive measure than acuity (Xiong et al., 2020) but also it provides essential information about spatial processing for lower spatial scales. In this study, we used unipolar stimuli of positive and negative polarity to explore the difference in contrast sensitivity of the ON and OFF pathways. We used the method of the quick Contrast Sensitivity Function (qCSF) (Hou et al., 2010; Lesmes et al., 2010) to measure the contrast sensitivity over different spatial frequencies.
Materials and methods
Participants
Eleven anisometropic amblyopes (mean age: 26.2 ± 4.4 years old; mean ± standard deviation [SD]; eight males) and 10 controls (mean age: 24.6 ± 0.8 years old; six males, including one author) with normal or corrected to normal visual acuity (0 logMAR or better) participated in this study. None of the amblyopes had any detectable ocular diseases or structural anomalies, clinical details are provided in Table 1. Amblyopia in this study was defined according to the PPP [American Academy of Ophthalmology, Preferred Practice Patterns] (Wallace et al., 2018), i.e., interocular acuity difference of more than 2 lines (0.2 logMAR) with an obvious cause like anisometropia, strabismus, or deprivation. The dominant eyes of controls were defined by the card-in-the-hole test (Dane and Dane, 2004). All subjects except one (being an author) were naive to the purpose of the experiment. Written informed consent was obtained from all participants after an explanation of the nature and possible consequences of the study. Our study has been approved by the Ethical Committee of the affiliated eye hospital of Wenzhou Medical University (ethics approval number: 2019-095-K-89) and conformed to the Declaration of Helsinki.
Apparatus
A PC running Matlab R2016a (Mathworks, Natick, MA, United States) with Psychtoolbox 3.0.14 (Brainard, 1997; Pelli, 1997; Kleiner et al., 2007) generated and controlled the stimuli. The stimuli were presented on a gamma-corrected cathode-ray-tube (CRT) monitor (CPD-G520, 2001, SONY Ichinomiya Corp., Japan), with a display area of 40.5 × 30.7 cm, 1,280 × 1024 pixels resolution, a refresh rate of 85 Hz, and mean background luminance of 54.5 cd/m2. Bits# Stimulus Processor (Cambridge Research Systems Ltd., United Kingdom) was used to generate 14-bit contrast resolution. All tests were carried out in a dark room. Before starting the test, all participants underwent dark adaptation for 5 min in the darkroom. Participants viewed the screen monocularly with a black fabric patch occluding the untested eye. A chin rest was used to minimize participants’ head movement and to ensure the viewing distance.
Design
We measured the participant’s monocular contrast sensitivity function (CSF) using the quick contrast sensitivity function (qCSF) algorithm (Hou et al., 2010; Lesmes et al., 2010). It is a Bayesian adaptive procedure that estimates the contrast sensitivity function with a truncated log-parabola model, which is described by four parameters: gain, peak SF, bandwidth, and truncation at low spatial frequencies. This methodology has demonstrated clinical accuracy and efficiency in measuring contrast sensitivity within control and amblyopic populations (Hou et al., 2010; Gao et al., 2015). For detailed equations and descriptions, please refer to Equation 1 in the Supplementary material.
For each participant, the monocular CSFs were measured with three types of stimuli: balanced contrast, increment, and decrement patterns. These three types of stimuli were tested in a randomized order to eliminate the possible learning effect. The 6 CSFs (3 stimuli types × 2 eyes) were tested twice in the same day, and the results were averaged based on the two repetitions. Each CSF was measured in 100 trials, preceded by 5 practice trials with full-contrast (100%) stimuli. It took about 5 min for each participant to complete one CSF measure. After finishing two CSF measures (i.e., ~10 min) with one eye, the participant was asked to take a 2-min rest before proceeding to test the other eye with two additional CSF measures.
Stimuli
Figure 1A illustrates the three types of stimuli we generated for the CSF tests. The stimuli consisted of horizontal or vertical bandpass filtered noise in a Gaussian window. They were generated by filtering white noise in the spatial domain with a Gabor filter at the desired spatial frequency (bandwidth of 1.84 octaves). The three stimuli types included: (1) Balanced contrast pattern (as shown in the black box in Figure 1A) aimed to simultaneously stimulate both ON and OFF pathways in the CSF measurement (Kim et al., 2017). (2) Increment pattern (illustrated in the red box in Figure 1A) presented positive contrast (brighter filtered noise than the background) to selectively stimulate the ON pathway. (3) Decrement pattern (displayed in the blue box in Figure 1A) provided negative contrast (darker filtered noise than the background) to selectively stimulate the OFF pathway. Amblyopic individuals viewed stimuli in a Gaussian window with a sigma of 10°, while control subjects viewed stimuli in a Gaussian window with a sigma of 4°. Amblyopes have impaired contrast sensitivity in high SF compared to normal individuals (Hess and Howell, 1977; Sjöstrand, 1981). This implies that these two groups would present different cutoff spatial frequencies (Volkers et al., 1987; Majander et al., 2017). Based on these previous reports, we optimized the testing range to [0.31–32.31] c/d for the control group and [0.31–11.77] c/d for the amblyopic group. Spatial frequency (SF) and contrast levels were varied by the qCSF approach (Lesmes et al., 2010) in different trials. Moreover, to maintain comparable computational times for processing stimuli (in terms of pixels), different viewing distances (57 cm for amblyopes and 140 cm for normal controls) were employed. This variation in viewing distance had minimal impact on their light adaptation levels, given the negligible pupillary changes within this range (Lin et al., 2022).
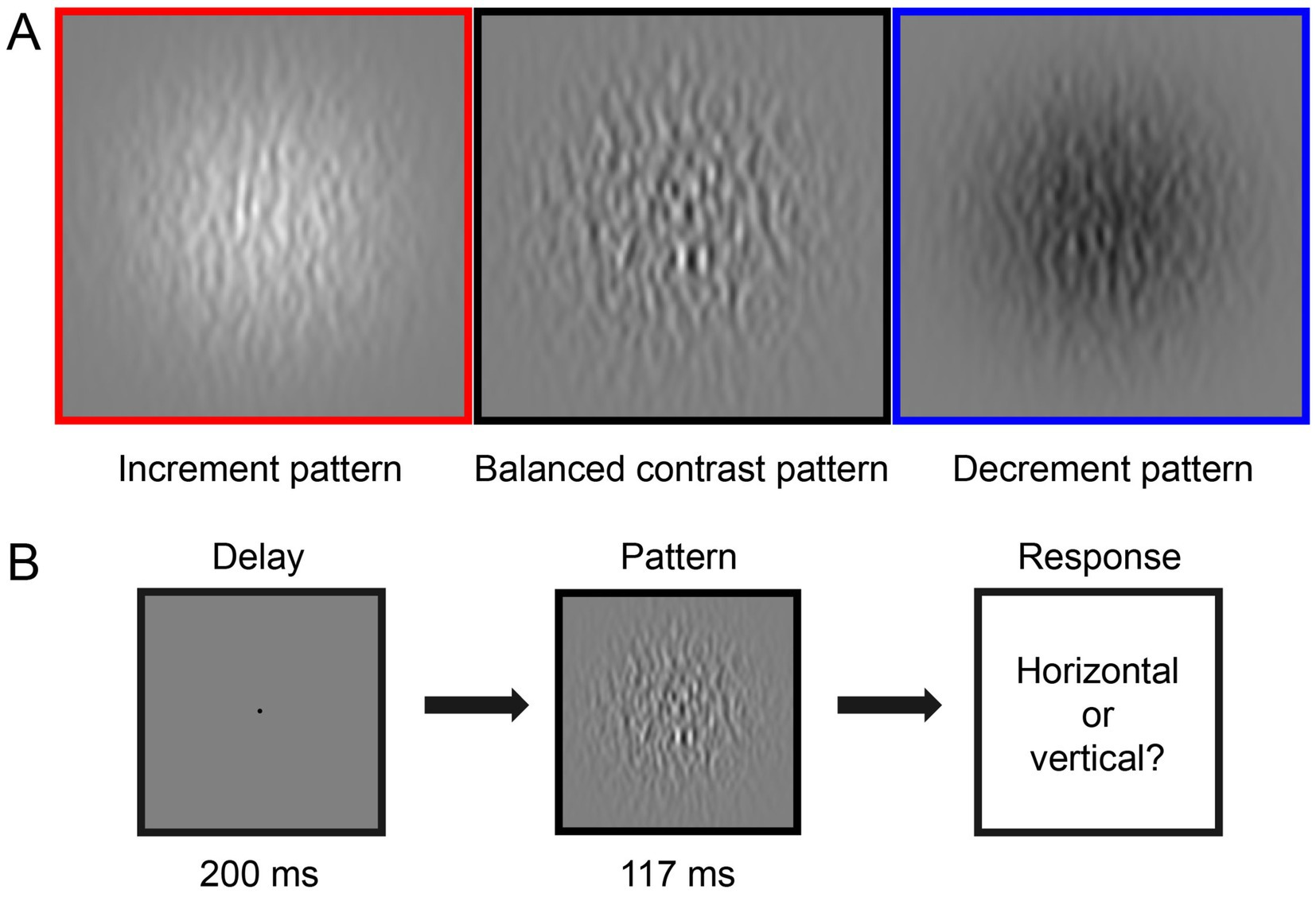
Figure 1. Illustration of the experiment design. (A) Three types of visual stimuli in this study: increment pattern (red box, positive-contrast stimuli), balanced contrast pattern (black box), and decrement pattern (blue box, negative-contrast stimuli). (B) Each trial started with a 200-ms presentation of the black fixation point in the center of the gray background, followed by a 117-ms presentation of the test stimuli. Observers were asked to report the orientation of the stimulus in each trial, i.e., horizontal or vertical.
Procedure
As shown in Figure 1B, in one CSF trial, a black fixation point (radius 0.15°), signaled by a brief tone to begin, was presented in the center of the screen for 200 milliseconds (ms). This was followed by a 117 ms presentation of a horizontal or vertical (randomized in each trial) test stimulus. Participants were asked to identify whether the orientation of the stimulus was horizontal or vertical by pressing the ‘left’ or ‘up’ key on the keyboard. The next trial started immediately after the response.
Statistical analysis
We extracted various parameters for statistical analysis, including contrast sensitivities at chosen spatial frequencies (SF), the area under log contrast sensitivity function (AULCSF; the integration of the log-parabola over the entire SF range of the measurements), cut-off SF corresponding to a contrast threshold of 0.5, and the four qCSF parameters (gain, peak SF, bandwidth, and truncation at low SF). Repeated-measures analysis of variances (ANOVAs), Bonferroni-corrected Pairwise Comparisons, t-tests, and Pearson correlation tests were used to compare the difference and correlation of contrast sensitivity, AULCSF, cut-off SF, qCSF parameters and the interocular difference between the three testing conditions (i.e., balanced contrast, decrement and increment patterns). Wilcoxon signed-rank tests were used to compare the differences between the ratio of increment/decrement contrast sensitivity and 1. The analysis of the cut-off SF and qCSF parameters are presented in Supplementary Figures S1, S2. Statistical analyses were performed using IBM-SPSS 23.0 (IBM Inc., Armonk, NY, United States).
Results
The balanced contrast sensitivity
Figure 2A shows the average CSFs for 11 amblyopes (left) and 10 controls (right) under the balanced contrast test condition. As expected, the amblyopic group exhibited a large extent of interocular difference in balanced contrast sensitivity across spatial frequencies, whereas the control group displayed minimal interocular differences. A two-way repeated-measures analysis of variance (ANOVA) revealed that the contrast sensitivity was significantly different between eyes in both amblyopes [F(1,10) = 74.992, p < 0.001, partial = 0.882] and controls [F(1,9) = 35.561, p < 0.001, partial = 0.789]. In Figure 2B, we plotted individuals’ area under the log contrast sensitivity function (AULCSF) for the amblyopic eye (or non-dominant eye) as a function of the fellow eye (or dominant eye). Notably, the datapoints of controls (triangles) cluster around the identity line, the average ratio of the area under the log contrast sensitivity function for the non-dominant eye to the dominant eye was 0.91. In contrast, the majority of amblyopic individuals’ data points (circles) fall below the identity line, the average ratio of the area under the log contrast sensitivity function for the amblyopic eye to the fellow eye was 0.67. Paired-samples t-tests confirmed the statistical significance of interocular differences in balanced area under the log contrast sensitivity function for both amblyopes (t = 6.677, p < 0.001, Cohen’s d = 2.986) and controls (t = 6.272, p < 0.001, Cohen’s d = 2.957). Additionally, in Supplementary Figure S4, we provide evidence of a linear predictive relationship between the balanced CSF and the decrement and increment CSF. We did not directly compare results in the amblyopic and control groups because of the different stimulus sizes and testing distances employed.
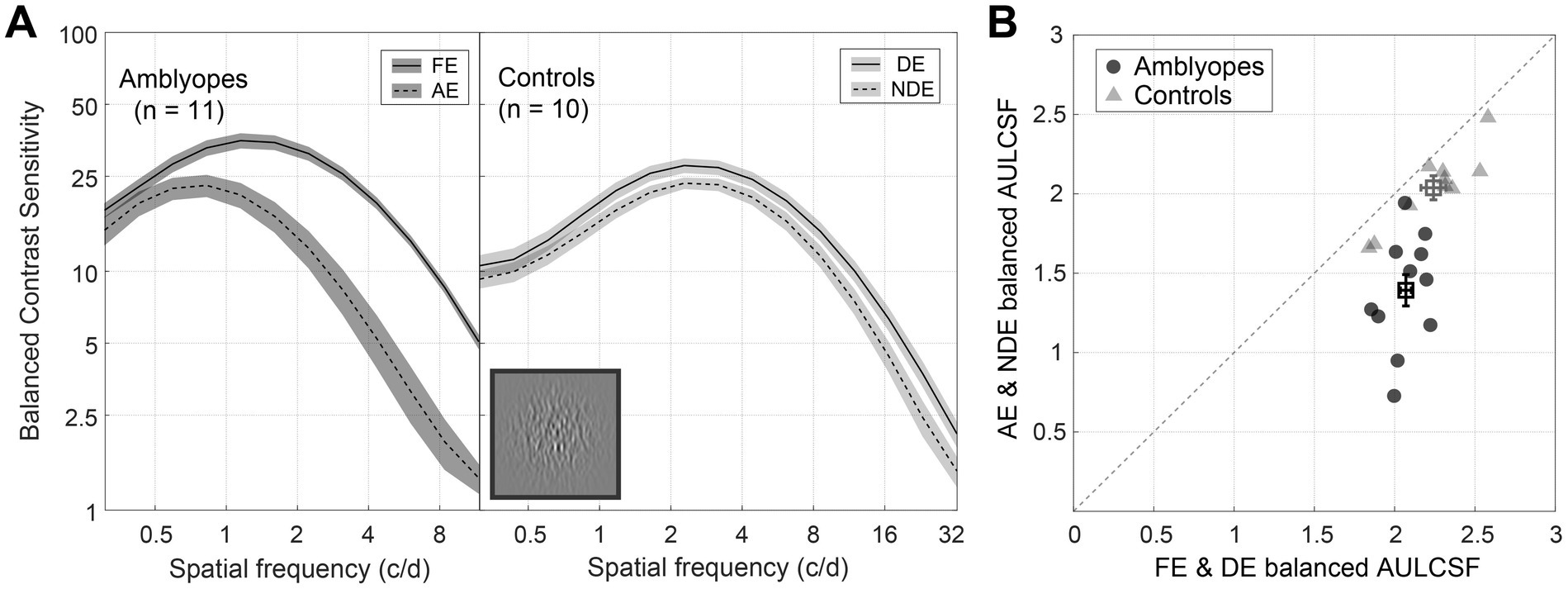
Figure 2. Balanced contrast sensitivity of amblyopes and controls. (A) Average balanced CSF of amblyopes (left) and controls (right). The solid lines represent the fellow eye (FE) and dominant eye (DE), and the dashed lines represent the amblyopic eye (AE) and non-dominant eye (NDE). The shaded regions represent ± standard error [SE]. (B) Average balanced the area under the log contrast sensitivity function (AULCSF) of amblyopes (circles) and controls (triangles), X-axis represents FE and DE, and Y-axis represents AE and NDE. Each symbol represents one subject. The average results were plotted with square symbols. The dashed line represents the identity. Error bars represent SE. A direct comparison between amblyopes and controls is not possible due to the difference in experimental design.
The difference between decrement and increment contrast sensitivity
In Figure 3A, we have depicted the average decrement and increment CSFs for both amblyopes and controls. As anticipated, both decrement and increment CSFs were significantly lower than the balanced CSF (p < 0.001, for all). For amblyopes (Figure 3A, left), contrast sensitivities of the amblyopic eye were lower in both the decrement (blue curves) and increment (red curves) conditions compared to the fellow eye. However, no such interocular difference was found in controls (Figure 3A, right). Indeed, the area under the log contrast sensitivity function for the AE were significantly lower in both the two conditions for amblyopes (p < 0.001, for all; Figure 3B), while no interocular area under the log contrast sensitivity function differences were observed in controls.
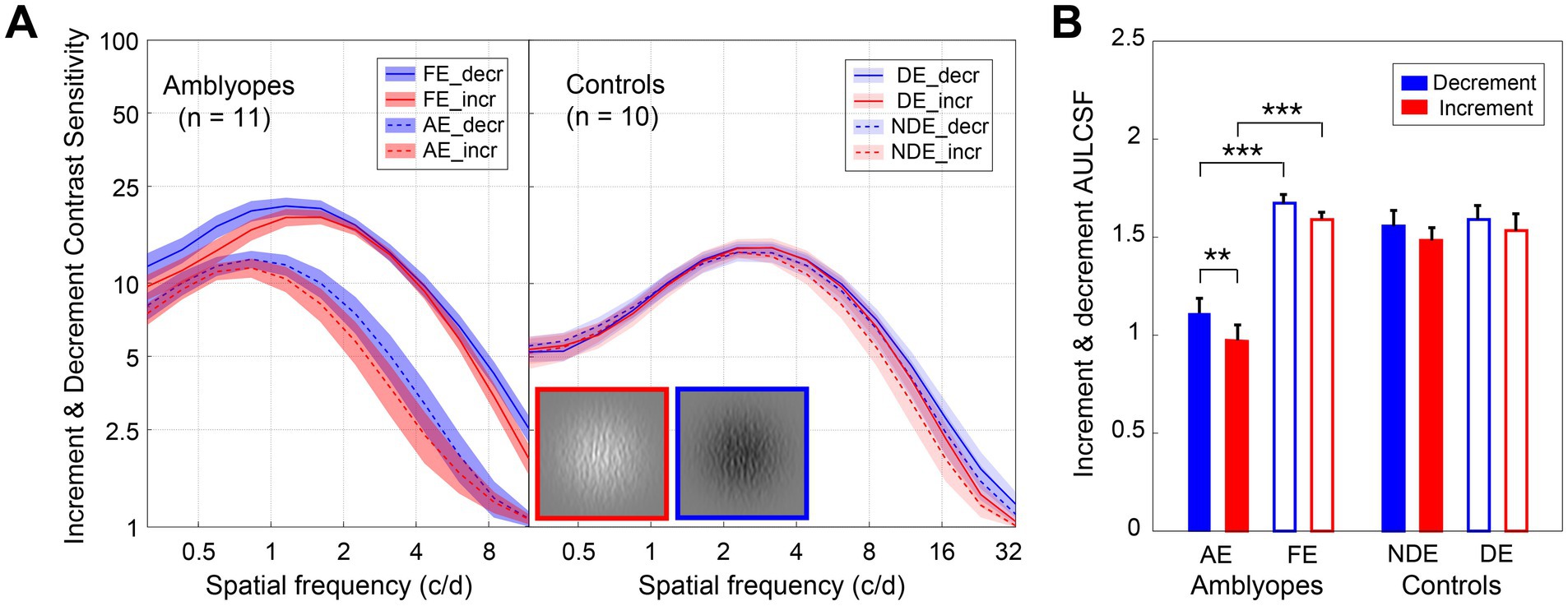
Figure 3. Decrement and increment contrast sensitivities of amblyopes and controls. (A) Average decrement (blue) and increment (red) CSF of amblyopes (left) and controls (right). The solid lines represent the FE (fellow eye) and NDE (non-dominant eye), the dashed lines represent the AE (amblyopic eye) and DE (dominant eye). The shaded areas represent ± SE. (B) The average area under the log contrast sensitivity function for decrement (blue) and increment (red) of amblyopes (left) and controls (right). The solid bars represent AE and NDE, the hollow bars represent FE and DE. Error bars represent SE. Results of the repeated measures ANOVA are shown: *p < 0.05, **p < 0.01, ***p < 0.001. A direct comparison between amblyopes and controls is not possible due to the difference in experimental design.
Interestingly, we found that the increment CSF was slightly lower than that for the decrement in the amblyopic eye of amblyopes (Figure 3A). Two three-way repeated-measures ANOVAs, with test condition (2 levels: decrement and increment), eye (2 levels), and spatial frequency (12 levels) as within-subject factors were conducted for the two groups, respectively. In amblyopes, we identified significant main effects for eye [F(1,10) = 56.059, p < 0.001, partial = 0.849], condition [F(1,10) = 4.244, p = 0.066, partial = 0.298], and spatial frequency [F(1.7,17.1) = 71.864, p < 0.001, partial = 0.878]. We also observed a significant interaction between eye and spatial frequency [F(1.7,16.7) = 13.383, p = 0.001, partial = 0.572]. However, we found no significant interactions between eye and condition [F(1,10) = 0.715, p = 0.418, partial = 0.067], condition and spatial frequency [F(1.6,16.0) = 2.484, p = 0.123, partial = 0.199], or the three-way interaction between eye, condition and spatial frequency [F(1.8,18.0) = 1.697, p = 0.212, partial = 0.145]. Subsequent Bonferroni-corrected pairwise comparisons revealed a significant difference in contrast sensitivity between decrement and increment in the amblyopic eye (p = 0.038), while such a difference was not significant in the fellow eye (p = 0.133). Conversely, in the control group, we found a significant main effect for spatial frequency [F(1.7,19.0) = 114.359, p < 0.001, partial = 0.927], but not for eye [F(1,9) = 1.513, p = 0.25, partial = 0.144] or condition [F(1,9) = 3.702, p = 0.087, partial = 0.291]. Furthermore, we found no significant interaction between eye and condition [F(1,11) = 0.116, p = 0.741, partial = 0.013], eye and spatial frequency [F(1.7,18.5) = 1.599, p = 0.088, partial = 0.151], or eye and condition and spatial frequency [F(1.8,20.0) = 0.318, p = 0.991, partial = 0.034]. Further Bonferroni-corrected pairwise comparisons indicated little difference in contrast sensitivity between decrement and increment in non-dominant eye (p = 0.272) or dominant eye (p = 0.668).
We further compared the area under the log contrast sensitivity function between the two test conditions (Figure 3B) by conducting two-way repeated-measures ANOVAs, with test condition (2 levels: decrement and increment) and eye (2 levels) as the within-subject factors for the two groups. In amblyopes, we observed significant main effects for eye [F(1,10) = 59.603, p < 0.001, partial = 0.856] and test condition [F(1,10) = 14.828, p = 0.003, partial = 0.597]. Bonferroni-corrected pairwise comparisons disclosed a significant difference between decrement and increment conditions in amblyopic eye (p = 0.001), but not in fellow eye (p = 0.107), with the disparity characterized by higher cut-off spatial frequencies in the decrement CSF (Supplementary Figure S2A). Among the control group, a significant main effect was observed for the test condition [F(1,9) = 8.783, p = 0.016, partial = 0.494], yet Bonferroni-corrected pairwise comparisons showed no significant differences between decrement and increment conditions for either non-dominant eye (p = 0.144) or dominant eye (p = 0.333).
To illustrate the joint effect of spatial frequency and the increment and decrement test conditions on observers’ contrast sensitivities, we computed the ratio of increment/decrement contrast sensitivity, and presented this ratio as a function of spatial frequency in Figure 4. To ensure accuracy and mitigate any potential floor effects due to stimuli visibility limitations at high spatial frequencies, we focused solely on the spatial frequency range where corresponding contrast thresholds were less than 1, namely 0.31–2.25 c/d for amblyopes, and 0.31–11.96 c/d for controls. As depicted in Figure 4A, the ratio of increment/decrement contrast sensitivity fell below the unity line and decreased with increasing spatial frequency in the amblyopic eye (purple dashed line). Such pattern was not observed in the fellow eye of amblyopes (Figure 4A, blue solid line) or in the eyes of controls (Figure 4B), where the ratios remained close to 1 across all spatial frequencies. We then conducted two-way ANOVAs with eye and spatial frequency as within-subject factors for the two groups, respectively. These analyses did not reveal any significant main effects for eye or spatial frequency in either group (p > 0.5, for all). However, among amblyopic individuals, a noteworthy interaction between eye and spatial frequency emerged regarding the ratio of increment/decrement contrast sensitivity [F(1.8,17.5) = 3.735, p = 0.049, partial = 0.272]. In contrast, controls did not exhibit a significant interaction between eye and spatial frequency [F(2.0,18.4) = 0.459, p = 0.643, partial = 0.049].
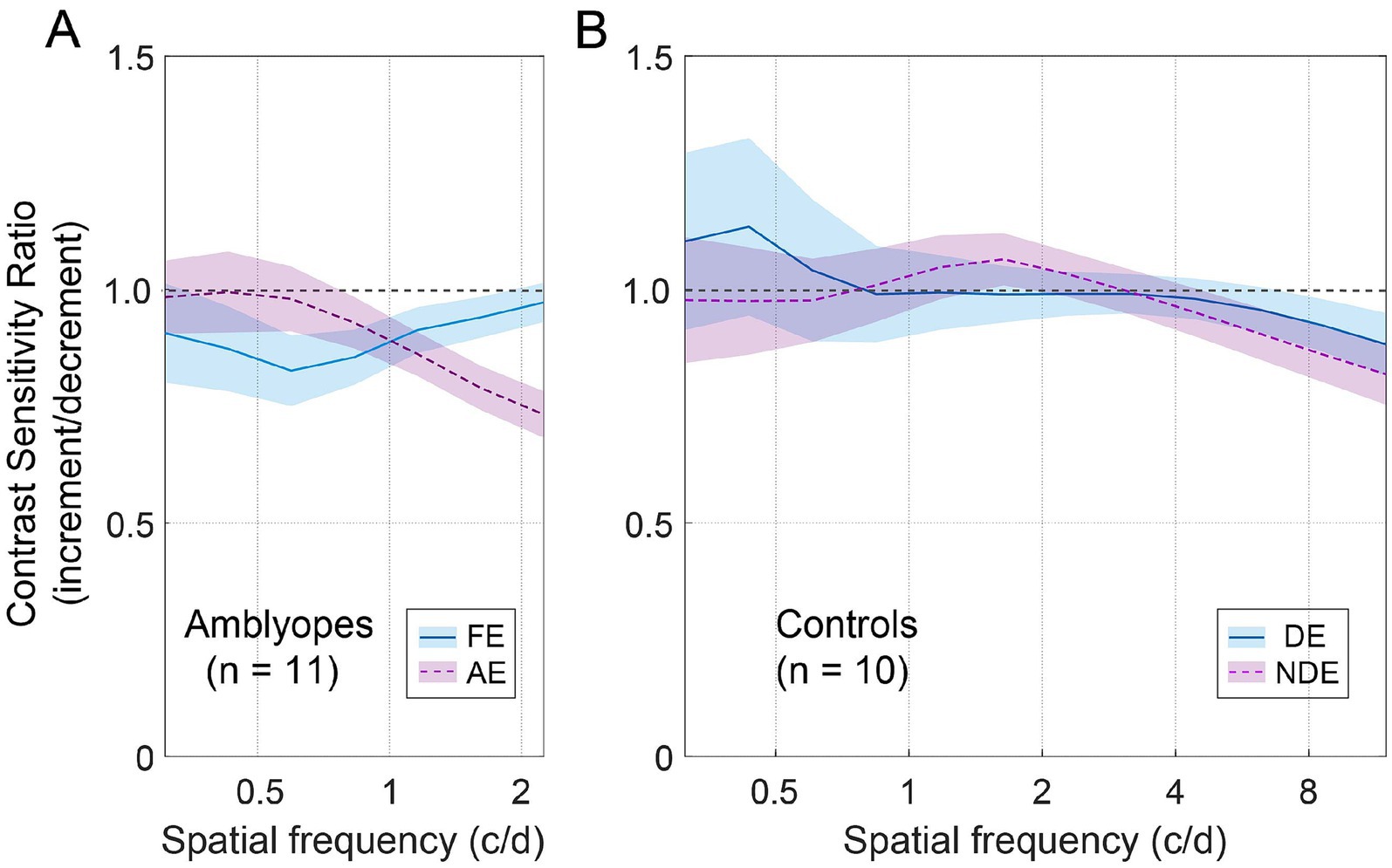
Figure 4. The average contrast sensitivity ratio of increment/decrement. (A) Average ratio for amblyopes. The blue solid line represents FE (fellow eye), and the dashed purple line represents AE (amblyopic eye). The shaded regions represent ± SE. (B) Average ratio for controls. The blue solid line represents DE (dominant eye), and the dashed purple line represents NDE (non-dominant eye).
Furthermore, we found that in the amblyopic eye, the ratio of increment/decrement contrast sensitivity was significantly smaller than 1 at spatial frequencies of 1.16 c/d (Wilcoxon signed-rank test: Z = 2.134, p = 0.033), 1.61 c/d (Z = 2.845, p = 0.004), and 2.24 c/d (Z = 2.934, p = 0.003). And in the fellow eye, the ratio was significantly smaller than 1 at a spatial frequency of 0.83 c/d (Z = 2.04, p = 0.041). For controls, the ratio was significantly smaller than 1 at spatial frequencies of 8.58 c/d (Z = 2.293, p = 0.022) and 11.96 c/d (Z = 2.395, p = 0.017).
The relationship between the decrement and increment contrast sensitivities
To further illustrate the relationship between the decrement and increment contrast sensitivities, we plotted the individual area under the log contrast sensitivity function of the decrement condition as a function of the increment condition in Figure 5. Pearson correlation analysis indicated a significant positive correlation between the area under the log contrast sensitivity function of the two conditions. This correlation was observed to be significant in the amblyopic eye (r = 0.924, p < 0.001) and in both two eyes of controls (p < 0.005, for all), although it was not significant in the fellow eye (p = 0.116). A similar relationship was observed among all qCSF parameters (Supplementary Figure S1) and the cut-off spatial frequency (Supplementary Figure S2B).
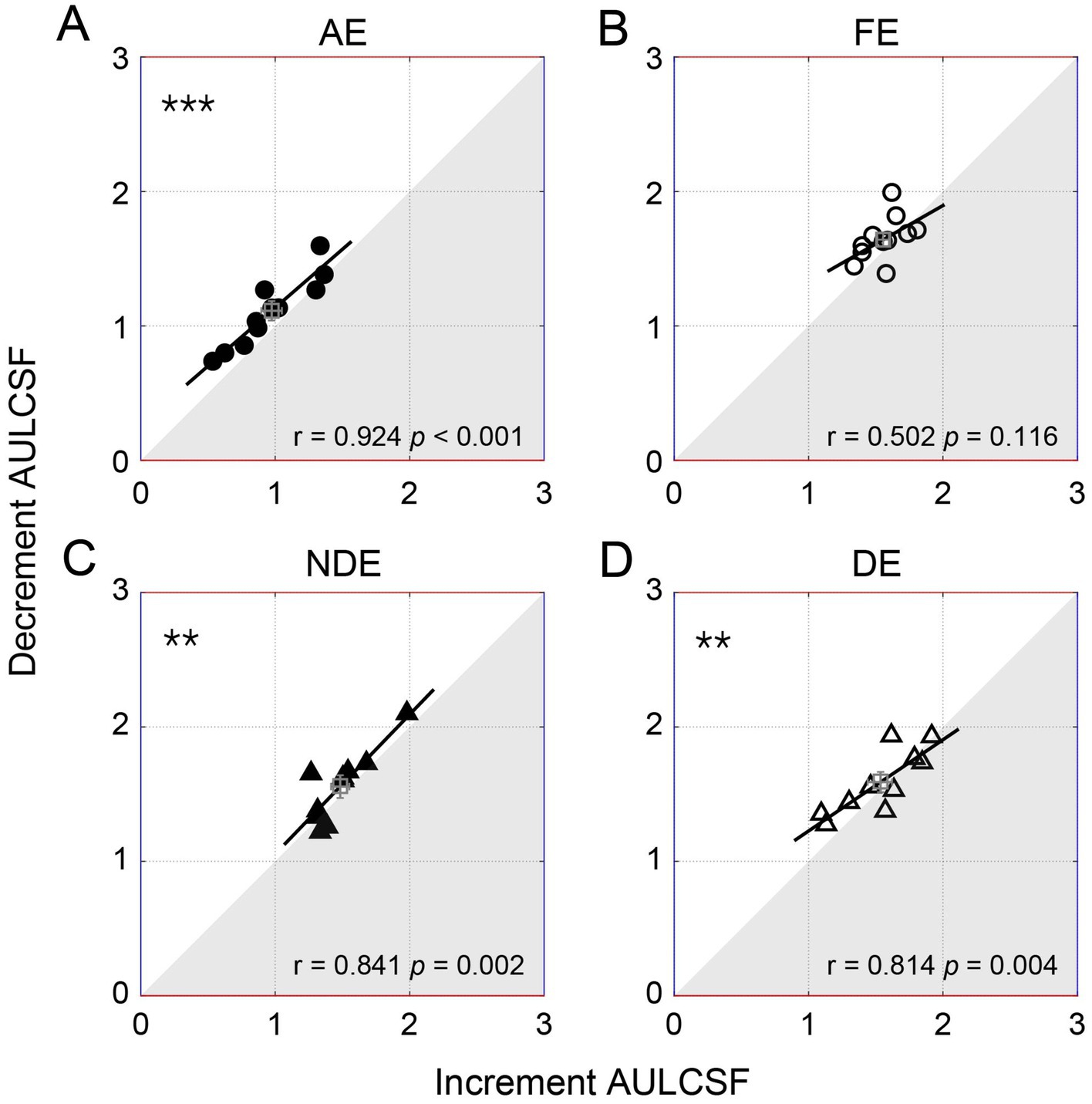
Figure 5. The individual decrement area under the log contrast sensitivity function (AULCSF) as a function of increment for amblyopes (circles, the top row) and controls (triangles, the bottom row). Each symbol represents one subject. The average results were plotted with the square symbol; error bars represent SE. The solid lines represent the best linear fits. Results of Pearson correlation test are shown: #p < 0.1, **p < 0.01, ***p < 0.001. (A) AE: amblyopic eye, (B) FE: fellow eye, (C) NDE: NON-dominant eye, (D) DE: dominant eye.
Discussion
In this study, we explored the contrast sensitivity of ON and OFF visual pathways in adult amblyopic patients and control adults, using positive-contrast stimuli that activate the ON visual pathway (increment pattern), negative-contrast stimuli activating the OFF visual pathway (decrement pattern), and stimuli activating both pathways simultaneously (balanced contrast pattern). Our findings revealed that contrast sensitivities for both increment and decrement conditions were lower than those for balanced contrast sensitivity across all eyes. Interestingly, in the amblyopic eye, the sensitivity to increment stimuli was lower than that for decrement sensitivity at high spatial frequencies. Furthermore, we observed a positive correlation between sensitivities in these two conditions. These results suggest that while amblyopes exhibit reduced sensitivity in both ON and OFF pathways, the contrast sensitivity loss in the ON visual pathway is marginally greater than that in the OFF pathway at higher spatial frequencies.
In mammals, the ON and OFF visual pathways originate from the bipolar cells of the retina (Masu et al., 1995; Vardi and Morigiwa, 1997). OFF cells, characterized by their higher number, smaller dendritic fields, and denser branching, likely reflect the more negative contrast information present in natural scenes (van Hateren et al., 2002; Ratliff et al., 2010). These two pathways transmit brightness and darkness information separately to the LGN (Hubel and Wiesel, 1961; Kaplan and Shapley, 1982), and subsequently to the primary visual cortex (McConnell and LeVay, 1984; Zahs and Stryker, 1988; Kremkow et al., 2016), where they converge on individual V1 neurons to form receptive fields (Alonso et al., 2001). The dominance of OFF cells in cortical responses is attributed to their wider thalamic input converge, stronger connections, and greater excitatory afferents compared to ON cells (Jin et al., 2008; Yeh et al., 2009; Jin et al., 2011). In addition, studies such as Luo-Li et al. (2016) have highlighted the ON–OFF difference in detecting light and dark bars, with individuals responding more accurately and faster to dark stimuli, consistent with other psychophysics studies (Bowen et al., 1989; Chubb and Nam, 2000; Buchner and Baumgartner, 2007; Komban et al., 2011; Komban et al., 2014).
Controversy exists in studies on ON–OFF perception in normal individuals. To illustrate, Barboni et al. (2013) showed that ON contrast sensitivity was lower than OFF contrast sensitivity, using sawtooth stimuli at 0.3 and 2 c/d. While, Komban et al. (2011) measured the contrast thresholds of normal subjects at 16, 24, and 32 c/d using square-wave grating, and found that the contrast perception of the ON pathway was slightly reduced compared with that of the OFF, but this difference was not significant. Our findings align more with the latter, indicating slightly larger area under the log contrast sensitivity function in the decrement condition in the non-dominant eye compared to the increment condition without statistical significance. Future research might explore the spatial frequency dependency of this comparison further.
In adults with amblyopia, we noted a slightly larger deficit in contrast sensitivity for the ON pathway stimulation, positively correlated with the OFF-pathway deficit. Amblyopes generally exhibit lower contrast sensitivity compared to controls, particularly at high spatial frequencies (Hess and Howell, 1977; Sjöstrand, 1981). This means that the stimulus contrast at threshold (and particularly at high spatial frequencies) is higher when testing the amblyopic eye compared to the fellow eye or the normal controls. While at high contrast, there is more perceptual difference between light and dark targets (Wallis et al., 2013). This is consistent with previous studies, notably Pons et al.’s study (2019), which indicated a larger deficit for ON stimulation compared to OFF at suprathreshold contrast levels. They revealed that for this high-level perceptual task, amblyopia exhibits a larger deficit for ON compared with OFF stimulation. Our study’s results at the detection threshold are in harmony with these findings, showing a slightly greater deficit for ON stimulation than OFF in the amblyopic eye and a positive correlation between the two pathways.
As for the interocular difference (i.e., the difference between amblyopic eye and fellow eye, and the difference between non-dominant eye and dominant eye), we found that the interocular differences of area under the log contrast sensitivity function in amblyopes were larger than those of controls in both ON and OFF pathways (Supplementary Figure S3B). Notably, a smaller difference in the ON pathway correlated with a reduced difference in the OFF pathway in amblyopic eyes, a correlation not seen in normal subjects (Supplementary Figures S3B,C). Our study indicates that the ON–OFF difference in amblyopia already exists in the early stages of contrast processing (Owsley, 2003). This impairment in low-level, high spatial frequency contrast detection may impact higher processing stages, potentially affecting the perception of lower spatial frequency suprathreshold stimuli e.g., (see Pons et al., 2019) due to the convergence of low-level small receptive fields at these stages.
There is a potential effect of the phantom array (Jordan and Hershberger, 1994; Hershberger et al., 1998) that has been reported mainly at the neuronal level but has been neglected in the psychophysical literature. However, we do not believe that this effect can explain the ON-selective deficit reported in Pons’ study (2019) and the pattern we show here. First, if this effect selectively affected the percept of ON stimuli, we would expect a similar ON-selective deficit in all participants. However, we found selective deficits in ON percept only in amblyopic eyes and not in fellow eyes or normal controls. Second, none of our participants reported such percepts which we believe was due to two things: first, we instructed all participants to fixate during stimulus presentation; second, the target stimulus was not strongly periodic with sharp edges, it was a filtered texture with no sharp edges. Another limitation to consider is the different experimental designs used for amblyopes and controls. While a larger viewing distance was used to test higher spatial frequencies in the control group, this difference did not allow us to directly compare the results between amblyopes and controls (Figures 2, 3). This viewing distance difference would not significantly impact participants’ light adaptation (Lin et al., 2022). And, as other testing conditions were similar, we believe that it should not unduly affect our measurements and conclusions. Additionally, it is worth noting that we used a small black fixation point (radius 0.15°) in our tests to guide subjects to the stimulus location. This fixation point disappeared once the stimulus appeared, ensuring it did not influence the experimental results.
Conclusion
Current diagnosis, treatment, or clinical trials of amblyopia mainly focus on the improvement of visual acuity. Visual acuity is measured usually by asking patients to identify high-contrast black letters on a white background, which is biased toward OFF pathway stimulation. We show that amblyopia affects both the ON and OFF visual pathways at an early level of visual processing, and that amblyopes exhibit a slight difference between the ON and OFF visual pathways. This discovery represents a significant stride toward comprehending the development of ON and OFF visual pathways, potentially contributing to refining amblyopia assessment and treatment strategies.
Data availability statement
The datasets presented in this study can be found in online repositories. The names of the repository/repositories and accession number(s) can be found: Github repository (https://github.com/junhan16/amblyopia.git).
Ethics statement
The studies involving humans were approved by the Ethical Committee of the affiliated eye hospital of Wenzhou Medical University. The studies were conducted in accordance with the local legislation and institutional requirements. The participants provided their written informed consent to participate in this study. Written informed consent was obtained from the individual(s) for the publication of any potentially identifiable images or data included in this article.
Author contributions
JW: Conceptualization, Data curation, Formal analysis, Investigation, Methodology, Software, Validation, Visualization, Writing – original draft, Writing – review & editing. ZC: Validation, Writing – review & editing. DK: Data curation, Formal analysis, Investigation, Writing – review & editing. WL: Methodology, Writing – review & editing. RH: Conceptualization, Funding acquisition, Investigation, Project administration, Resources, Supervision, Validation, Writing – review & editing. JZ: Conceptualization, Data curation, Formal analysis, Funding acquisition, Investigation, Methodology, Project administration, Resources, Software, Supervision, Validation, Visualization, Writing – review & editing. AR: Conceptualization, Investigation, Methodology, Resources, Software, Validation, Writing – review & editing.
Funding
The author(s) declare that financial support was received for the research, authorship, and/or publication of this article. This work was supported by the National Key Research and Development Program of China Grant (2023YFC3604104), the Natural Science Foundation for Distinguished Young Scholars of Zhejiang Province, China (LR22H120001), the Non-profit Central Research Institute Fund of Chinese Academy of Medical Sciences (2023-PT320-04) and the Project of State Key Laboratory of Ophthalmology, Optometry and Vision Science, Wenzhou Medical University (No. J02-20210203) to JZ. RH was supported by Canadian Institutes of Health Research (#125686) and the Natural Sciences and Engineering Research Council of Canada (#228103). The sponsor or funding organizations had no role in the design or conduct of this research.
Acknowledgments
The authors would like to thank all the subjects who participated in this study.
Conflict of interest
The authors declare that the research was conducted in the absence of any commercial or financial relationships that could be construed as a potential conflict of interest.
The author(s) declared that they were an editorial board member of Frontiers, at the time of submission. This had no impact on the peer review process and the final decision.
Publisher’s note
All claims expressed in this article are solely those of the authors and do not necessarily represent those of their affiliated organizations, or those of the publisher, the editors and the reviewers. Any product that may be evaluated in this article, or claim that may be made by its manufacturer, is not guaranteed or endorsed by the publisher.
Supplementary material
The Supplementary material for this article can be found online at: https://www.frontiersin.org/articles/10.3389/fpsyg.2024.1494964/full#supplementary-material
References
Alonso, J. M., Usrey, W. M., and Reid, R. C. (2001). Rules of connectivity between geniculate cells and simple cells in cat primary visual cortex. J. Neurosci. 21, 4002–4015. doi: 10.1523/jneurosci.21-11-04002.2001
Barboni, M. T. S., Nagy, B. V., De Araújo Moura, A. L., Damico, F. M., Da Costa, M. F., Kremers, J., et al. (2013). ON and OFF electroretinography and contrast sensitivity in Duchenne muscular dystrophy. Invest. Ophthalmol. Vis. Sci. 54, 3195–3204. doi: 10.1167/iovs.13-11700
Bowen, R. W., Pokorny, J., and Smith, V. C. (1989). Sawtooth contrast sensitivity: decrements have the edge. Vis. Res. 29, 1501–1509. doi: 10.1016/0042-6989(89)90134-X
Bradley, A., and Freeman, R. D. (1981). Contrast sensitivity in anisometropic amblyopia. Invest. Ophthalmol. Vis. Sci. 21, 467–476
Brainard, D. H. (1997). The psychophysics toolbox. Spat. Vis. 10, 433–436. doi: 10.1163/156856897X00357
Buchner, A., and Baumgartner, N. (2007). Text-background polarity affects performance irrespective of ambient illumination and colour contrast. Ergonomics 50, 1036–1063. doi: 10.1080/00140130701306413
Chubb, C., and Nam, J. H. (2000). Variance of high contrast textures is sensed using negative half-wave rectification. Vis. Res. 40, 1677–1694. doi: 10.1016/S0042-6989(00)00007-9
Dane, A., and Dane, S. (2004). Correlations among handedness, eyedness, monocular shifts from binocular focal point, and non-verbal intelligence in university mathematics students. Percept. Mot. Skills 99, 519–524. doi: 10.2466/pms.99.2.519-524
Davis, A. R., Sloper, J. J., Neveu, M. M., Hogg, C. R., Morgan, M. J., and Holder, G. E. (2008). Differential changes in color and motion-onset visual evoked potentials from both eyes in early- and late-onset strabismic amblyopia. Invest. Ophthalmol. Vis. Sci. 49, 4418–4426. doi: 10.1167/iovs.07-1437
Ding, J., Klein, S. A., and Levi, D. M. (2013). Binocular combination in abnormal binocular vision. J. Vis. 13:14. doi: 10.1167/13.2.14
Ding, J., and Levi, D. M. (2014). Rebalancing binocular vision in amblyopia. Ophthalmic Physiol. Opt. 34, 199–213. doi: 10.1111/opo.12115
Dolan, R. P., and Schiller, P. H. (1994). Effects of ON channel blockade with 2-amino-4-phosphonobutyrate (APB) on brightness and contrast perception in monkeys. Vis. Neurosci. 11, 23–32. doi: 10.1017/s095252380001107x
Gao, Y., Reynaud, A., Tang, Y., Feng, L., Zhou, Y., and Hess, R. F. (2015). The amblyopic deficit for 2nd order processing: generality and laterality. Vis. Res. 114, 111–121. doi: 10.1016/j.visres.2014.10.020
Grill-Spector, K., and Malach, R. (2004). The human visual cortex. Annu. Rev. Neurosci. 27, 649–677. doi: 10.1146/annurev.neuro.27.070203.144220
Hamm, L. M., Black, J., Dai, S., and Thompson, B. (2014). Global processing in amblyopia: a review. Front. Psychol. 5:583. doi: 10.3389/fpsyg.2014.00583
Hershberger, W. A., Jordan, J. S., and Lucas, D. R. (1998). Visualizing the perisaccadic shift of spatiotopic coordinates. Percept. Psychophys. 60, 82–88. doi: 10.3758/bf03211919
Hess, R. F., and Howell, E. R. (1977). The threshold contrast sensitivity function in strabismic amblyopia: evidence for a two type classification. Vis. Res. 17, 1049–1055. doi: 10.1016/0042-6989(77)90009-8
Hou, F., Huang, C.-B., Lesmes, L., Feng, L.-X., Tao, L., Zhou, Y.-F., et al. (2010). qCSF in clinical application: efficient characterization and classification of contrast sensitivity functions in amblyopia. Invest. Ophthalmol. Vis. Sci. 51, 5365–5377. doi: 10.1167/iovs.10-5468
Hubel, D. H., and Wiesel, T. N. (1961). Integrative action in the cat's lateral geniculate body. J. Physiol. 155, 385–398. doi: 10.1113/jphysiol.1961.sp006635
Jin, J., Wang, Y., Lashgari, R., Swadlow, H. A., and Alonso, J.-M. (2011). Faster thalamocortical processing for dark than light visual targets. J. Neurosci. 31, 17471–17479. doi: 10.1523/jneurosci.2456-11.2011
Jin, J. Z., Weng, C., Yeh, C. I., Gordon, J. A., Ruthazer, E. S., Stryker, M. P., et al. (2008). On and off domains of geniculate afferents in cat primary visual cortex. Nat. Neurosci. 11, 88–94. doi: 10.1038/nn2029
Jindra, L. F., and Zemon, V. (1989). Contrast sensitivity testing: a more complete assessment of vision. J Cataract Refract Surg 15, 141–148. doi: 10.1016/s0886-3350(89)80002-1
Jordan, J. S., and Hershberger, W. A. (1994). Timing the shift in retinal local signs that accompanies a saccadic eye movement. Percept. Psychophys. 55, 657–666. doi: 10.3758/bf03211680
Kaplan, E., and Shapley, R. M. (1982). X and Y cells in the lateral geniculate nucleus of macaque monkeys. J. Physiol. 330, 125–143. doi: 10.1113/jphysiol.1982.sp014333
Kim, Y. J., Reynaud, A., Hess, R. F., and Mullen, K. T. (2017). A normative data set for the clinical assessment of achromatic and chromatic contrast sensitivity using a qCSF approach. Invest. Ophthalmol. Vis. Sci. 58, 3628–3636. doi: 10.1167/iovs.17-21645
Kleiner, M., Brainard, D., Pelli, D., Ingling, A., Murray, R., and Broussard, C. (2007). What’s new in Psychtoolbox-3? Perception 36:1–16. doi: 10.1068/v070821
Komban, S. J., Alonso, J.-M., and Zaidi, Q. (2011). Darks are processed faster than lights. J. Neurosci. 31, 8654–8658. doi: 10.1523/jneurosci.0504-11.2011
Komban, S. J., Kremkow, J., Jin, J., Wang, Y., Lashgari, R., Li, X., et al. (2014). Neuronal and perceptual differences in the temporal processing of darks and lights. Neuron 82, 224–234. doi: 10.1016/j.neuron.2014.02.020
Kremkow, J., Jin, J., Komban, S. J., Wang, Y., Lashgari, R., Li, X., et al. (2014). Neuronal non-linearity explains greater visual spatial resolution for darks than lights. Proc. Natl. Acad. Sci. USA 111, 3170–3175. doi: 10.1073/pnas.1310442111
Kremkow, J., Jin, J., Wang, Y., and Alonso, J. M. (2016). Principles underlying sensory map topography in primary visual cortex. Nature 533, 52–57. doi: 10.1038/nature17936
Lesmes, L. A., Lu, Z.-L., Baek, J., and Albright, T. D. (2010). Bayesian adaptive estimation of the contrast sensitivity function: the quick CSF method. J. Vis. 10:17.1. doi: 10.1167/10.3.17
Levi, D. M., Knill, D. C., and Bavelier, D. (2015). Stereopsis and amblyopia: a mini-review. Vis. Res. 114, 17–30. doi: 10.1016/j.visres.2015.01.002
Lin, S. Y., Su, H. R., Lo, C. C., Yeh, S. M., Lee, C. H., Wu, R., et al. (2022). Effects of extended viewing distance on accommodative response and pupil size of myopic adults by using a double-Mirror system. Int. J. Environ. Res. Public Health 19:2942. doi: 10.3390/ijerph19052942
Luo-Li, G., Alais, D., and Freeman, A. W. (2016). Orientation discrimination requires co-activation of on- and off-dominated visual channels. J. Vis. 16:18. doi: 10.1167/16.15.18
Majander, A., João, C., Rider, A. T., Henning, G. B., Votruba, M., Moore, A. T., et al. (2017). The pattern of retinal ganglion cell loss in OPA1-related autosomal dominant optic atrophy inferred from temporal, spatial, and chromatic sensitivity losses. Invest. Ophthalmol. Vis. Sci. 58, 502–516. doi: 10.1167/iovs.16-20309
Mao, Y., Min, S. H., Chen, S., Gong, L., and Zhou, J. (2020). Binocular imbalance in amblyopia depends on spatial frequency in binocular combination. Invest. Ophthalmol. Vis. Sci. 61:7. doi: 10.1167/iovs.61.8.7
Masu, M., Iwakabe, H., Tagawa, Y., Miyoshi, T., Yamashita, M., Fukuda, Y., et al. (1995). Specific deficit of the ON response in visual transmission by targeted disruption of the mGluR6 gene. Cell 80, 757–765. doi: 10.1016/0092-8674(95)90354-2
Mcconnell, S. K., and Levay, S. (1984). Segregation of on- and off-center afferents in mink visual cortex. Proc. Natl. Acad. Sci. USA 81, 1590–1593. doi: 10.1073/pnas.81.5.1590
Owsley, C. (2003). Contrast sensitivity. Ophthalmol. Clin. N. Am. 16, 171–177. doi: 10.1016/s0896-1549(03)00003-8
Pang, Y., Allen, M., Robinson, J., and Frantz, K. A. (2019). Contrast sensitivity of amblyopic eyes in children with myopic anisometropia. Clin. Exp. Optom. 102, 57–62. doi: 10.1111/cxo.12817
Pelli, D. G. (1997). The VideoToolbox software for visual psychophysics: transforming numbers into movies. Spat. Vis. 10, 437–442. doi: 10.1163/156856897X00366
Pons, C., Jin, J. Z., Mazade, R., Dul, M., Zaidi, Q., and Alonso, J. M. (2019). Amblyopia affects the ON visual pathway more than the OFF. J. Neurosci. 39, 6276–6290. doi: 10.1523/jneurosci.3215-18.2019
Ratliff, C. P., Borghuis, B. G., Kao, Y., Sterling, P., and Balasubramanian, V. (2010). Retina is structured to process an excess of darkness in natural scenes. Proc. Natl. Acad. Sci. USA 107, 17368–17373. doi: 10.1073/pnas.1005846107
Schiller, P. H. (1982). Central connections of the retinal ON and OFF pathways. Nature 297, 580–583. doi: 10.1038/297580a0
Schiller, P. H. (1992). The ON and OFF channels of the visual system. Trends Neurosci. 15, 86–92. doi: 10.1016/0166-2236(92)90017-3
Schiller, P. H., Sandell, J. H., and Maunsell, J. H. (1986). Functions of the ON and OFF channels of the visual system. Nature 322, 824–825. doi: 10.1038/322824a0
Sjöstrand, J. (1981). Contrast sensitivity in children with strabismic and anisometropic amblyopia. A study of the effect of treatment. Acta Ophthalmol. 59, 25–34. doi: 10.1111/j.1755-3768.1981.tb06706.x
Van Hateren, J. H., Rüttiger, L., Sun, H., and Lee, B. B. (2002). Processing of natural temporal stimuli by macaque retinal ganglion cells. J. Neurosci. 22, 9945–9960. doi: 10.1523/JNEUROSCI.22-22-09945.2002
Vardi, N., and Morigiwa, K. (1997). ON cone bipolar cells in rat express the metabotropic receptor mGluR6. Vis. Neurosci. 14, 789–794. doi: 10.1017/s0952523800012736
Volkers, A. C., Hagemans, K. H., Van Der Wildt, G. J., and Schmitz, P. I. (1987). Spatial contrast sensitivity and the diagnosis of amblyopia. Br. J. Ophthalmol. 71, 58–65. doi: 10.1136/bjo.71.1.58
Wallace, D. K., Repka, M. X., Lee, K. A., Melia, M., Christiansen, S. P., Morse, C. L., et al. (2018). Amblyopia preferred practice pattern®. Ophthalmology 125, P105–P142. doi: 10.1016/j.ophtha.2017.10.008
Wallis, S. A., Baker, D. H., Meese, T. S., and Georgeson, M. A. (2013). The slope of the psychometric function and non-stationarity of thresholds in spatiotemporal contrast vision. Vis. Res. 76, 1–10. doi: 10.1016/j.visres.2012.09.019
Webber, A., and Wood, J. (2005). Amblyopia: prevalence, natural history, functional effects and treatment. Clin. Exp. Optom. 88, 365–375. doi: 10.1111/j.1444-0938.2005.tb05102.x
Xiao, O., Morgan, I. G., Ellwein, L. B., and He, M. (2015). Prevalence of amblyopia in school-aged children and variations by age, gender, and ethnicity in a multi-country refractive error study. Ophthalmology 122, 1924–1931. doi: 10.1016/j.ophtha.2015.05.034
Xing, D., Yeh, C. I., and Shapley, R. M. (2010). Generation of black-dominant responses in V1 cortex. J. Neurosci. 30, 13504–13512. doi: 10.1523/jneurosci.2473-10.2010
Xiong, Y. Z., Kwon, M. Y., Bittner, A. K., Virgili, G., and Legge, G. E. (2020). Relationship between acuity and contrast sensitivity: differences due to eye disease. Invest. Ophthalmol. Vis. Sci. 61:40. doi: 10.1167/iovs.61.6.40
Yeh, C. I., Xing, D., and Shapley, R. M. (2009). “Black” responses dominate macaque primary visual cortex v1. J. Neurosci. 29, 11753–11760. doi: 10.1523/JNEUROSCI.1991-09.2009
Zahs, K. R., and Stryker, M. P. (1988). Segregation of ON and OFF afferents to ferret visual cortex. J. Neurophysiol. 59, 1410–1429. doi: 10.1152/jn.1988.59.5.1410
Zhou, J. W., Liu, R., Feng, L. X., Zhou, Y. F., and Hess, R. F. (2016). Deficient binocular combination of second-order stimuli in amblyopia. Invest. Ophthalmol. Vis. Sci. 57, 1635–1642. doi: 10.1167/iovs.15-18253
Keywords: amblyopia, contrast sensitive function (CSF), qCSF, ON and OFF channels, contrast perception
Citation: Wei J, Cheng Z, Kong D, Lin W, Hess RF, Zhou J and Reynaud A (2024) Understanding contrast perception in amblyopia: a psychophysical analysis of the ON and OFF visual pathways. Front. Psychol. 15:1494964. doi: 10.3389/fpsyg.2024.1494964
Edited by:
Anna Maria Berardi, Université de Lorraine, FranceReviewed by:
Xuefeng Shi, Tianjin Eye Hospital, ChinaErnest Greene, University of Southern California, United States
Copyright © 2024 Wei, Cheng, Kong, Lin, Hess, Zhou and Reynaud. This is an open-access article distributed under the terms of the Creative Commons Attribution License (CC BY). The use, distribution or reproduction in other forums is permitted, provided the original author(s) and the copyright owner(s) are credited and that the original publication in this journal is cited, in accordance with accepted academic practice. No use, distribution or reproduction is permitted which does not comply with these terms.
*Correspondence: Jiawei Zhou, emhvdWp3QG1haWwuZXllLmFjLmNu