- 1Department of Biomedical Science, College of Health Sciences, QU Health, Qatar University, Doha, Qatar
- 2Department of Human Nutrition, College of Health Sciences, QU Health, Qatar University, Doha, Qatar
Background and aims: Anorexia nervosa (AN) is a complex neuropsychiatric disorder. This systematic review synthesizes evidence from diverse studies to assess and investigate the association between gene polymorphisms and psychological and neurobiological factors in patients with AN.
Methods: A systematic search across PubMed, PsycINFO, Scopus, and Web of Science databases, along with manual searching, was conducted. The review protocol was approved by PROSPERO (CRD42023452548). Out of 1,250 articles, 11 met the inclusion criteria. The quality of eligible articles was assessed using the Newcastle-Ottawa Scale (NOS) tool. The systematic review followed the PRISMA guidelines.
Results: The serotoninergic system, particularly the 5-HTTLPR polymorphism, is consistently linked to altered connectivity in the ventral attention network, impaired inhibitory control, and increased susceptibility to AN. The 5-HTTLPR polymorphism affects reward processing, motivation, reasoning, working memory, inhibition, and outcome prediction in patients with AN. The dopaminergic system, involving genes like COMT, DRD2, DRD3, and DAT1, regulates reward, motivation, and decision-making. Genetic variations in these dopaminergic genes are associated with psychological manifestations and clinical severity in patients with AN. Across populations, the Val66Met polymorphism in the BDNF gene influences personality traits, eating behaviors, and emotional responses. Genes like OXTR, TFAP2B, and KCTD15 are linked to social cognition, emotional processing, body image concerns, and personality dimensions in patients with AN.
Conclusion: There was an association linking multiple genes to the susceptibly and/or severity of AN. This genetic factor contributes to the complexity of AN and leads to higher diversity of its clinical presentation. Therefore, conducting more extensive research to elucidate the underlying mechanisms of anorexia nervosa pathology is imperative for advancing our understanding and potentially developing targeted therapeutic interventions for the disorder.
Systematic review registration: [https://clinicaltrials.gov/], identifier [CRD42023452548].
1 Introduction
Anorexia nervosa (AN) is a complex neuropsychiatric disorder characterized by restricted caloric intake, resulting in dangerously low body weight, distorted body image, and an intense fear of weight gain (American Psychiatric Association, 2013). AN is a common eating disorder among female adolescents, with prevalence rates of 0.3–1.2% (Schwartz et al., 2008) and a female-to-male ratio of 10:1 (Smink et al., 2012). This gender disparity is most pronounced in female adolescents aged 15–19 years, where incidence rates are highest (Auger et al., 2023). The reasons for this gender imbalance are not fully understood, but they may involve a combination of biological, psychological, and sociocultural factors (Boerner et al., 2004; Crisp and Collaborators, 2006; Stanford and Lemberg, 2012). For instance, societal pressures and ideals regarding female body image, as well as differences in stress response and coping mechanisms between genders, may contribute to the higher prevalence in females (Crisp and Collaborators, 2006). Despite being less common in males, AN in men is often underdiagnosed and understudied, leading to a lack of awareness and understanding of the disorder in this population (Raevuori et al., 2014).
Additionally, AN is particularly serious due to the high rate of relapse (Klump et al., 2009), its medical complications resulting from severe malnutrition, as well as the elevated risk of suicidality (Attia, 2010). In 20–30% of cases, AN develops into a chronic illness persisting for many years and often over the lifetime of an individual, impairing social interaction and diminishing academic and professional potential. Notably, compared to age and sex-matched controls, people with AN have mortality rates that are five to ten times higher (Kirk et al., 2017). The chronic nature of the disease, coupled with a high relapse rate and significant personal, familial, and societal burdens, underscores the pressing need for increased research to understand its etiology and, therefore, improve treatment approaches.
Typically, patients with AN are assessed and diagnosed according to The Diagnostic and Statistical Manual of Mental Disorders, fifth edition (DSM-5) tool (American Psychiatric Association, 2013), which is the 2013 publication of the American Psychology Association (APA). A range of adverse outcomes are associated with AN, including medical and psychiatric comorbidities (Herzog et al., 1997; Fairburn and Harrison, 2003; Treasure et al., 2010), and is defined as the psychiatric disorder with the highest mortality rate (Arcelus et al., 2011; Zipfel et al., 2014). Anxiety and depression are prevalent comorbidities in individuals with AN that further exacerbate the severity and complexity of the disorder (Pleplé et al., 2021; Calvo-Rivera et al., 2022). In addition, individuals with AN tend to display personality traits characterized by a low impulsivity (Favaro et al., 2004; Testa et al., 2022), high harm avoidance (Frank et al., 2018), and perfectionism (Lloyd et al., 2014), suggesting a neurobiological basis for the disorder rooted in heightened inhibition and control mechanisms that govern behavior, emotion, and physiology (Ellison and Foong, 1998; Bulik et al., 2010; Wonderlich et al., 2018). Individuals with AN may have impairments in this inhibitory function, as seen in their struggles with set-shifting tasks that require redirecting attention away from previously relevant stimuli (Roberts et al., 2007). Psychological assessment tools such as semi-structured interviews and self-reporting questionnaires are pivotal in providing insights into diagnosis, disease severity, and comorbidities and understanding the various psychological traits and behavioral aspects of eating disorders like AN, hence the impact on the quality of life of those individuals (Schaefer et al., 2021; Wade and Pellizzer, 2024). While to understand the underlying structural and functional changes in the brain associated with AN, neuroimaging tools like Magnetic Resonance Imaging (MRI), functional Magnetic Resonance Imaging (fMRI), and Resting-state functional magnetic resonance imaging (rs-fMRI) were used. These tools detect changes in brain activity, blood flow, and oxygenation, revealing functional connectivity patterns and baseline brain activity in which variability in connectivity may be linked to cognitive abilities and personality traits (van den Eynde et al., 2012; Zhu et al., 2012). Positron Emission Tomography (PET) and Single Photon Emission Computed Tomography (SPECT) scans analyze metabolic and blood flow activity, aiding in the assessment of functional abnormalities (Blake et al., 2003). Brain research using these techniques has provided abundant evidence of neurobiological changes in eating disorders (ED).
The underlying mechanisms of the development and progression of AN are not yet fully understood. Present research indicates that the disease lies at an intersection of psychological characteristics and environmental and sociocultural factors, in addition to genetic and biological predispositions (Mazzeo and Bulik, 2009; Campbell and Peebles, 2014; Baker et al., 2017). Interestingly, family (Strober et al., 2000; Frisch et al., 2001) and twin (Klump et al., 2001; Bulik et al., 2010) studies strongly suggest a genetic component to the disorder by consistently showing that first-degree relatives of individuals with AN have an increased risk of developing the disorder compared with relatives of unaffected individuals (Bulik et al., 2007). This is reflected in the heritability rates of AN, which are estimated to be approximately 33–84% (Donato et al., 2022), indicating a significant genetic contribution to the disorder. Genetic polymorphisms, which are variations in DNA sequences among individuals, have emerged as potential candidates influencing susceptibility to the onset, progress, and development of AN (Paolacci et al., 2020). Recent advances in molecular genetics have enabled the identification of genetic polymorphisms that may confer susceptibility to AN. Variations in genes related to serotonergic, dopaminergic, opioid, and appetite regulation systems have gained particular attention for their involvement in mood and body weight regulation (Compan, 2021), reward and emotional behavior (Weiss et al., 1988; Hasan and Hunaid, 2011), as well as energy intake pathways (Monteleone et al., 2005; Perello and Dickson, 2015).
The intricate relationship between genetic polymorphisms and the traits found in AN is a critical area of investigation. Neurotransmitter imbalances, altered brain structure, and disrupted neural circuits contribute to the AN pathophysiology. The impact of hormones and neuroactive peptides, such as sex hormones and gut hormones, on brain responses disrupts normal food reward circuits (Monteleone and Maj, 2013). Structural brain alterations involve cerebral atrophy, enlarged ventricles, and changes in grey and white matter volumes (van den Eynde et al., 2012; Seitz et al., 2016). Studies examining gray matter volumes in AN demonstrate fluctuations across various brain regions, with some areas exhibiting smaller volumes, particularly linked to the severity of the illness (Martin Monzon et al., 2017). Similarly, research on white matter volume and integrity yields inconsistent findings, but alterations in structural connectivity patterns suggest compensatory changes during the recovery process (Frank et al., 2013). Functional and effective connectivity studies point to disruptions within networks associated with the executive function (Lee et al., 2014), reward processing (Cha et al., 2016), and perception (Fonville et al., 2014), potentially contributing to the altered eating behaviors seen in AN. Body image distortion, a hallmark of AN, implicates parietal and frontal regions in encoding motivational relevance to sensory events (Wagner et al., 2003) as well as prefrontal and cingulate brain response (Xu et al., 2017). The exploration of genetic polymorphisms and their correlation with neurobiological and psychological factors in AN represents a crucial step toward unraveling the intricate complexities of this debilitating disorder.
This research investigates how specific genetic polymorphisms might amplify individuals’ susceptibility to these psychological triggers, leading to the manifestation of AN. By examining the intricate gene–environment interplay, this study aims to provide a more nuanced understanding of the factors contributing to the disorder’s heterogeneity. This systematic review synthesizes evidence from diverse studies to assess and investigate the association of gene polymorphisms with psychological and neurobiological factors in patients with AN.
2 Materials and methods
The International Prospective Registry of Systematic Reviews (PROSPERO CRD42023452548)1 received and approved a copy of the review protocol. The guidelines of the Preferred Reporting of Systematic Reviews and Meta-Analyses (PRISMA) statement served as the basis for this systematic review (Liberati et al., 2009; Moher et al., 2009).
2.1 Search strategy
A comprehensive and systematic search was conducted across scientific databases PubMed, PsycINFO, Scopus, and Web of Science with additional hand search for relevant articles that were searched using the following search strategy: [(“anorexia nervosa” OR “eating disorders” OR “anorexia” OR “eating behavior”) AND (“genetics” OR “polymorphism” OR “genetic variation” OR “genetic factors”) AND (“brain” OR “neuroimaging” OR “neurobiology” OR “neurophysiology” OR “neurotransmitters”) AND (“psychology” OR “personality” OR “cognition” OR “emotion” OR “behavior”)]. The search was restricted to publications between 1990 and August 2023 to provide insight into the most recent developments in research within the field. Only English articles were considered for inclusion. Additionally, the reference lists of identified articles and relevant reviews were hand-searched to ensure the inclusiveness of the retrieved literature.
2.2 Eligibility criteria
As recommended in the PRISMA statement, eligibility was based on the PICOS criteria: population, interventions, comparison, outcome and study design (Moher et al., 2009). Through our systematic review, we will try to answer the following PICO question: Among individuals diagnosed with anorexia nervosa (P), does the presence of specific genetic polymorphisms (I) correlate with variations in neurobiological and psychological factors (O), influencing the onset, development, or severity of the disorder, as compared to those without these polymorphisms (C)?
2.2.1 Population
Studies that included individuals with a confirmed diagnosis of AN, based on established diagnostic criteria such as the Diagnostic and Statistical Manual of Mental Disorders, 4th or 5th edition (DSM-4/5), were included. Studies had to exclude any participant with any past or current other medical condition, especially neuropsychiatric disorder that could misinterpreted as part of AN comorbidities. As this review focused on isolating the specific influence of genetic polymorphisms on AN and its associated neurobiological and psychological factors, included participants with other medical conditions (e.g., gastrointestinal disorders, endocrine disorders, chronic pain) or neuropsychiatric disorders (e.g., mood disorders, OCD, ADHD) could introduce confounding factors. These conditions might share symptoms with AN, potentially obscuring the true association between genetic polymorphisms and AN itself. By excluding participants with comorbidities, the review aims to achieve a clearer understanding of the independent genetic effects on AN development. Moreover, studies with an exclusive focus on populations other than individuals diagnosed with AN [e.g., Bulimia nervosa (BN), binge-eating disorder (BED), or any other disorder] were excluded.
2.2.2 Intervention
In order to be included, studies had to examine the presence of specific genetic polymorphisms in the AN case group through genotyping.
2.2.3 Comparison
As a comparison group, studies with a control group in which participants to be genotyped were healthy with no current or history of any ED were included. Healthy controls had to have a BMI of 18 and higher. They had to have no past or current metabolic, endocrine, or gastrointestinal illness that could affect their weight, nor should they suffer from any neuropsychiatric disorder. Studies with within-subject comparisons, i.e., where genotyped patients with AN, BN, or BED were compared, were also included. In addition, studies that only investigated one group of AN and compared those who harbor the studied SNP and those who do not are also included.
2.2.4 Outcome
Studies were considered to be eligible if they included at least one variation in neurobiological and/or psychological factors influencing the onset, development, or severity of AN, such as brain imaging data (MRI, fMRI), neuroendocrine markers, neurotransmitter systems, neuropsychological assessments, or psychological traits and behaviors related to AN.
2.2.5 Study design
Observational studies (case–control studies, cohort studies, and cross-sectional) investigating the association between specific genetic polymorphisms and neurobiological and/or psychological factors in individuals diagnosed with AN were considered for inclusion. Review articles, meta-analyses, systematic reviews, commentary papers, and editorials were all excluded to avoid duplication of information. Animal Studies conducted solely on animal models were excluded, as the focus is on human populations. Non-peer-reviewed sources such as conference abstracts, posters, and non-peer-reviewed publications were all excluded to ensure the quality and reliability of the included studies.
2.3 Study selection
Initial screening of titles and abstracts was conducted by two independent reviewers (HA and HB), followed by full-text assessments of potentially eligible articles. Discrepancies were resolved through consensus. For data screening and filtration, Rayyan,2 an open-source website tool designed to assist researchers in systematic reviews and other knowledge synthesis initiatives, was utilized. After eliminating duplicate articles, titles and abstracts were evaluated using Rayyan, and articles were categorized as included, excluded, or maybe. Any records not meeting our inclusion criteria were excluded. During the secondary selection process, the full text of all articles was evaluated to address those initially categorized as “maybe” and finally included the eligible articles. In cases where the two reviewers disagreed on an article classified as “maybe” during the final review, discussion ensued until a consensus was reached. A PRISMA flowchart depicting the screening and selection procedure is presented in Figure 1.
2.4 Data extraction
For articles included in this systematic review, HA and HB collected the data independently and then discussed the final extraction. If any important data was not understood, researchers of the meant report were contacted. Variables extracted are displayed in Table 1. The following variables were extracted: (1) First Author and Year of publication; (2) study design (i.e., cross-sectional, case–control, or cohort); (3) characteristics of the study participants (sample size, gender distribution, and ethnicity); (4) Genetic polymorphisms studied (gene associated, variant investigated, and SNP ID) and finally, (5) summary of main findings as well as the associated p value were extracted.
2.5 Quality assessment
Two researchers (HA and HB) evaluated the quality of eligible articles for risk of bias of included cross-sectional and case–control studies using the Newcastle-Ottawa Scale (NOS) tool (Stang, 2010). When using the NOS tool, three domains of scoring criteria, totaling seven in all, were applied to the included cross-sectional and case–control studies. Study quality was determined by the studies’ selection of study groups, generalizability and verification of exposure and results, with little differences in scoring parameters when adapted to different study designs and a total of eight to nine points could be earned. For cross-sectional studies, the classification of quality was as follows: studies receiving more than seven points were categorized as very good, those earning 6–5 points were considered good quality, studies obtaining 4–3 points were deemed satisfactory, and those with 2 or fewer points were labeled as poor or unsatisfactory quality. For case–control studies, the quality categorization was as follows: studies accruing more than eight points were classified as very good, those receiving 7–6 points were termed good quality, studies earning 5–4 points were seen as satisfactory, and those with 3 or fewer points were designated as poor or unsatisfactory quality. Any instances of conflicts or disagreements arising during the evaluation process were resolved through consensus discussions between the researchers.
2.6 Ethical considerations
All included studies were scrutinized for evidence of ethical approval and participant consent. All the studies explicitly reported obtaining formal written informed consent from participants. This review is confined to the analysis of published data, and no primary data collection from human participants was conducted as part of this study.
2.7 Data synthesis
Considering the heterogeneity in study designs, the genetic polymorphisms investigated, neurobiological outcome measures, and psychological factors assessed, a narrative synthesis approach was utilized. The findings of the studies were systematically summarized and organized according to the impact of genetic polymorphisms on neurobiological and psychological factors in AN.
3 Results and discussion
3.1 Search results and study selection
An initial search through Medline, Scopus, Web of Science, and PsycArticles as of 20 August 2023 yielded 1,250 studies. Following the removal of 130 duplicates, the titles and abstracts of the remaining 1,120 articles were screened. Thirty-nine articles were identified for full paper review and were assessed for eligibility; of these, 30 articles were further excluded for not fulfilling one or more of the eligibility criteria. The reasons for exclusion were the investigation of different shared SNPs between AN and other psychiatric disorders or other EDs rather than isolated investigation on AN-associated SNPs (n = 4), studies that did not include the investigation of identified SNPs with neither neurobiological nor psychological factors, but only the genetic association and predisposition to AN (n = 9), studies focused on eating behaviors without explicitly focusing on AN (n = 6), family trio studies (n = 6) since we focused on population-based studies to enhance the generalization, a lack of enough data describing AN participants (n = 6). Further hand search, which involved reviewing the reference lists of the articles identified through our initial database search as well as relevant review articles and meta-analyses in the field of AN and genetic polymorphisms, resulted in two additional articles (Chen et al., 2015; González et al., 2021), increasing the total included to 11. The PRISMA flowchart in Figure 1 presents the detailed selection procedure flow.
3.2 Quality assessment of eligible studies
Six of the eleven articles included were cross-sectional studies (Favaro et al., 2013; Clarke et al., 2016; Collantoni et al., 2016; Gamero-Villarroel et al., 2017; Sala et al., 2018; González et al., 2021), whereas the remaining five were case–control studies (Rybakowski et al., 2007; Kim et al., 2009; Ando et al., 2012; Chen et al., 2015; Genis-Mendoza et al., 2019), all of which were assessed in terms of quality using the Newcastle-Ottawa Scale (NOS) Quality Assessment criteria (Supplementary Figure S1) (Stang, 2010). None of the included studies was below the satisfactory level; in fact, nine included articles achieved a good quality level and above after the assessment (Kim et al., 2009; Ando et al., 2012; Favaro et al., 2013; Scott-van Zeeland et al., 2014; Chen et al., 2015; Clarke et al., 2016; Collantoni et al., 2016; Gamero-Villarroel et al., 2017; Genis-Mendoza et al., 2019; González et al., 2021; Supplementary Table S1).
The final quality score for the six cross-sectional studies (Favaro et al., 2013; Clarke et al., 2016; Collantoni et al., 2016; Gamero-Villarroel et al., 2017; Sala et al., 2018; González et al., 2021) that were evaluated for selection, comparability, and outcome were mostly positive—with the majority rated as “Very good” (score of 7 out of 10) for four studies (Favaro et al., 2013; Clarke et al., 2016; Collantoni et al., 2016; Gamero-Villarroel et al., 2017). One study received a “Good” rating (score of 6 out of 10) (González et al., 2021), and another one study was considered “Satisfactory” (score of 4 out of 10) (Sala et al., 2018). At a similar rate, the final quality scores for the six case–control studies that were evaluated for selection, comparability and exposure were found to meet or exceed satisfactory standards, with two studies rated “Very good” with a score of 8 (Chen et al., 2015; Genis-Mendoza et al., 2019), two studies rated “good” with scores 6 and 7 (Kim et al., 2009; Ando et al., 2012) and one study deemed “satisfactory” with a score of 5 (Rybakowski et al., 2007).
3.3 Characteristics of the included studies
The main characteristics of the included articles are displayed in Table 1. All 11 articles were based on subjects presenting with AN (Rybakowski et al., 2007; Kim et al., 2009; Ando et al., 2012; Favaro et al., 2013; Chen et al., 2015; Clarke et al., 2016; Collantoni et al., 2016; Gamero-Villarroel et al., 2017; Sala et al., 2018; Genis-Mendoza et al., 2019; González et al., 2021). All articles included outcomes related to relationship investigation between specific studied SNPs of genes to neurobiological and/or psychological factors to AN (Rybakowski et al., 2007; Kim et al., 2009; Ando et al., 2012; Favaro et al., 2013; Chen et al., 2015; Clarke et al., 2016; Collantoni et al., 2016; Gamero-Villarroel et al., 2017; Sala et al., 2018; Genis-Mendoza et al., 2019; González et al., 2021). All the referenced studies in this review were published between 2007 and 2021 (Rybakowski et al., 2007; Kim et al., 2009; Ando et al., 2012; Favaro et al., 2013; Scott-van Zeeland et al., 2014; Chen et al., 2015; Clarke et al., 2016; Collantoni et al., 2016; Gamero-Villarroel et al., 2017; Sala et al., 2018; Genis-Mendoza et al., 2019; Boehm et al., 2020; González et al., 2021), with a concentration of publications in the latter half of the 2010s (Ando et al., 2012; Favaro et al., 2013; Chen et al., 2015; Clarke et al., 2016; Collantoni et al., 2016; Gamero-Villarroel et al., 2017; Sala et al., 2018; Genis-Mendoza et al., 2019). The participants were either healthy individuals or had the specific condition of interest (AN) typically including underweight patients who were diagnosed according to DSM criteria. The majority of the articles had the participant diagnosis based on the DSM-4 criteria (Rybakowski et al., 2007; Kim et al., 2009; Ando et al., 2012; Favaro et al., 2013; Chen et al., 2015; Collantoni et al., 2016; Sala et al., 2018), with a few re-evaluating to comply with the DSM-5 edition (Gamero-Villarroel et al., 2017; González et al., 2021), while others directly used the DSM-5 criteria for diagnosis (Clarke et al., 2016; Genis-Mendoza et al., 2019).
Those with other significant medical conditions, psychiatric comorbidities (except anxiety), and specific medication use were excluded. Healthy Controls (HC) were generally required to have no EDs with exclusions for significant medical or psychiatric conditions or substance abuse. All of the studies had all-female participants with the exception of two studies that had mixed-gender participants (Chen et al., 2015; Genis-Mendoza et al., 2019), with females comprising the majority of the group. The ethnic composition of the study participants was diverse, with six studies involving Caucasian participants (Rybakowski et al., 2007; Favaro et al., 2013; Clarke et al., 2016; Collantoni et al., 2016; Gamero-Villarroel et al., 2017; González et al., 2021), one study involving Mexican participants (Genis-Mendoza et al., 2019), one involving Japanese participants (Ando et al., 2012), one involving Korean participants (Kim et al., 2009), one involving Han Chinese participants (Chen et al., 2015), and one study not specifying the ethnicity but mentioned that the participants were recruited from Texas in the United States (Sala et al., 2018), while another was conducted in Germany without specifying the recruitment source (Boehm et al., 2020). The sample size in these studies varied widely, ranging from smaller groups of 49 AN cases to large cohorts of 689 AN cases, often paired with a comparable number of HC. The age range and mean ages of AN and HC participants across the studies spanned from early adolescence to mid-adulthood.
Notably, the results of the included studies, which involved other subgroups of different EDs, such as BN or BED, were filtered to include only results related to patients with AN in comparison to healthy controls. This helped accurately identify the possible correlation between the studied polymorphism and the neurobiological or psychological factors of AN as a unique ED.
3.4 Genetic polymorphism characteristics
The genetic polymorphisms investigated in these 11 studies focused on the polymorphisms within 1 or 2 target genes. The most common gene evaluated was the BDNF gene (Rybakowski et al., 2007; Ando et al., 2012; Clarke et al., 2016), followed by the SLC6A4 (Chen et al., 2015; Collantoni et al., 2016) and the COMT gene (Favaro et al., 2013; Collantoni et al., 2016), which were the genes of interest in 2 studies each. The rest of the genes TPH1 (Kim et al., 2009), OXTR (Sala et al., 2018), KCTD1 (Gamero-Villarroel et al., 2017), TFAP2B (Gamero-Villarroel et al., 2017), 5HTR2A (Genis-Mendoza et al., 2019), DRD2 (González et al., 2021), DRD3 (González et al., 2021) and DAT1 (González et al., 2021) were evaluated only in 1 study each. Among these 11, three studies evaluated the association of genetic polymorphism with both neurobiological factors and psychological factors (Favaro et al., 2013; Collantoni et al., 2016; Sala et al., 2018), whereas the other 8 studies evaluated the association with only psychological factors (Rybakowski et al., 2007; Kim et al., 2009; Ando et al., 2012; Chen et al., 2015; Clarke et al., 2016; Gamero-Villarroel et al., 2017; Genis-Mendoza et al., 2019; González et al., 2021). All the three studies that looked at neurobiological implications in the AN individuals employed neuroimaging techniques such as fMRI (Favaro et al., 2013; Collantoni et al., 2016; Sala et al., 2018). The correlation between the studied factors and the investigated genetic polymorphism was then reported in a separate column as studies’ findings.
3.5 Association of serotonergic system-related gene polymorphisms with psychological and neurobiological factors in patients with AN
Serotonin dysfunction has been shown to have an involvement in almost all neuropsychiatric conditions (Marazziti, 2017), including EDs (Pugsley et al., 1978; Steiger, 2004). Its role in the regulation of eating behavior has been well-confirmed (van Galen et al., 2021). Polymorphisms in the serotonin-related genes have been shown to influence the psychopathological features of EDs (Gervasini et al., 2012). Across the studies included in this review, four studies investigated the association of genetic polymorphisms in the serotonin-related genes including SLC6A4 (Chen et al., 2015; Collantoni et al., 2016), TPH1, and 5-HT2R with the psychological profiles and/or neurobiological characteristics of patients with AN. Figure 2 highlights the most important findings of the included studies which investigated the serotonergic system. The SLC6A4 gene codes for the serotonin transporter protein that carries serotonin from the synaptic cleft back into the presynaptic neuron (Mia et al., 2022). The degenerate repeat sequence in the promoter region of this gene, called the 5HTTLPR (5HT Transporter Linked Promoter Region), occurs in two common polymorphs designated as the short “S” and the long “L” alleles (Heils et al., 1996). Two of the reviewed studies investigated the polymorphism in the 5HTTLPR region of this gene (Chen et al., 2015; Collantoni et al., 2016). The TPH1 gene codes for the Tryptophan hydroxylase enzyme that catalyzes the rate-limiting step of serotonin synthesis. TPH1 is expressed in the body but not the brain (Walther et al., 2003). Nevertheless, the effect of variations in the TPH1 gene on brain-related variables, such as personality traits and neuropsychiatric disorders, has been studied (New et al., 1998; Allen et al., 2008). The 5HTR2A gene belongs to the G-protein coupled serotonin receptor family and codes for a Gq/G11-coupled receptor, which mainly plays a role in neuronal excitation in the central nervous system (Beliveau et al., 2017).
Collantoni et al. (2016) suggested that there is a correlation between the functional connectivity within the ventral attention network and reaction times measured by the stop signal task (SST), and that this relationship is mediated by the presence of the short allele (S allele) of the 5-HTTLPR polymorphism, proposing by that a potential modulatory role of the serotoninergic system in the ventral attention network. In patients with AN, positive connectivity in the ventral attention circuit correlated with response times in carriers of the LL genotype, while the opposite was true in carriers of the S allele. In other words, for patients with AN S allele carriers, negative connectivity is associated with longer response times suggesting impaired inhibitory control. This subsequently correlates with the severity of the disorder since there was a significant negative correlation between task performance and the lowest lifetime BMI. Ultimately, these correlations may reflect alterations in the mechanisms that regulate goal-oriented attention and self-referential processes in patients with AN (McFadden et al., 2014). This effect on the ventral attention network suggests that genetic variation can disrupt the processing reward, motivation, reasoning, working memory, inhibition, as well as outcome prediction (Krawczyk, 2002). In addition, the modulatory effect of the 5-HTTLPR polymorphism on the connectivity of the ventral attention network can also explain the impaired response inhibition in patients with AN as this network has been implicated in stimulus-driven attentional control (Corbetta and Shulman, 2002). Meanwhile, Chen et al. (2015) study looked into this gene polymorphism in Han Chinese patients with AN for association with psychological traits. These traits were measured using the Eating Disorders Examination (EDE) Interview as the assessment tool. They found that the frequency of the S allele of the 5-HTTLPR region of the SCL6A gene was significantly higher in patients than in controls, hence, associating with increased predisposition to AN. However, they did not find any clinical correlation or significant association between this genetic polymorphism and the severity of AN psychological symptoms. The same was reported by a meta-analysis done in 2015. Based on their results, the S allele was found to be significantly more prevalent in the AN group than in the control group (p = 0.006). Moreover, upon ethnically stratified analysis, it was observed that only Caucasians showed a correlation between the 5-HTTLPR polymorphism and AN, while no such correlation was found in Asians (Chen and Qian, 2015). Another meta-analysis of 15 studies concluded that being a carrier of the 5-HTTLPR S allele represents a risk factor for eating disorders (Solmi et al., 2016).
Historically, in 1996, it was discovered that the human serotonin transporter gene (SLC6A4; also known as 5-HTT) has a repeat length polymorphism in its promoter region, which regulates gene expression in vitro. People with one or two copies of the S allele of 5-HTTLPR have been found to exhibit elevated neuroticism, a personality trait that is linked to depression (Lesch et al., 1996). In 2002, it was reported that individuals carrying the S allele show increased amygdala reactivity to threatening stimuli, as determined by functional MRI (Hariri et al., 2002). In 2003, it was reported that S-carriers are more likely to experience depressive symptoms, have diagnosable depression, and exhibit suicidal tendencies after experiencing stressful life events and childhood maltreatment (Caspi et al., 2003). From there, a study has suggested that carriers of the S allele tend to exhibit lower expression of the serotonin transporter (SLC6A4), resulting in reduced reuptake of serotonin (5-HT) from the synapse. As a result, this reduced reuptake may lead to stronger psychopathological reactions to stressful experiences in individuals with the S allele compared to those with the L allele (Li, 2006).
In sum, the collected evidence strongly suggests that 5-HTTLPR polymorphism, particularly the S allele, is associated with altered functional connectivity in the ventral attention network, impaired inhibitory control, and increased predisposition to AN. However, a meta-analysis that included biobank data analysis concluded no significant difference in low-functioning genotype (S allele) and allele frequencies between AN group and controls, suggesting no potential association of 5-HTTLPR polymorphism with AN (Solmi et al., 2016).
Another study by Genis-Mendoza et al. (2019) in the Mexican population investigated two different SNPs (rs6311 and rs6313) in the 5HTR2A gene. They used two different psychological assessment tools, the Mini International Neuropsychiatric Interview for Children and Adolescents (MINI Kid) and Questionnaire on Eating and Weight Pattern (QEWP), to assess different comorbidities associated with AN disorder and other EDs, including major depressive episodes, suicidality, dysthymia disorder, attention-deficit/hyperactivity disorder, generalized anxiety disorder, oppositional defiant disorder, and psychotic disorder. The results were different between the two SNPs investigated. The G allele of rs6311 (−1438G/A) was much more common in the AN group and increased AN risk by almost nine-fold, as it was hypothesized that carriers of the G allele are susceptible to express the behavioral traits before the disease onset. It was also shown that rs6311 SNP is associated with an increased risk of suicide risk. No positive associations were observed for rs6313 SNP.
A study by Kim et al. (2009) looked at the TPH1 gene variation in a group of Korean AN patients. They investigated the genetic variant A218C (rs1800532) in the TPH1 gene among patients with AN and HC. Using the Korean version of Eating Disorder Examination (EDE), Temperament and Character Inventory (TCI), Yale-Brown Obsessive Compulsive Scale (Y-BOCS), and State–Trait Anxiety Inventory (STAI), they assessed the psychological factors of restraint, eating concern, weight concern, shape concern subscales, persistence and harm avoidance, obsessive-compulsive symptoms, as well as the level of depression and anxiety in patients with AN and HC. Interestingly, there were no differences in the TPH1 A218C allele/genotype frequency between the healthy controls and the individuals with AN. In addition, although the A218C variant in the TPH1 gene is reported to be related to a higher risk of suicidal behavior (Franken et al., 2005) and acute depression (Ikemoto and Panksepp, 1999), it was found by Kim et al. not to be associated with psychopathologies or obsessionally of patients with AN. Thus far, one could speculate that the SCL6A4 and the 5HTR2A may have a more direct and potent impact on serotonin reuptake. This could influence behaviors associated with AN more significantly than the TPH1 gene, which is involved in the earlier stages of serotonin synthesis and might be buffered by compensatory mechanisms.
3.6 Association of dopaminergic system-related gene polymorphisms with psychological and neurobiological factors in patients with AN
Dopamine has also long been proposed to play a central part in the pathophysiology symptoms observed in individuals with AN. Dopamine regulates the reward system (Lippa et al., 1973; Wise, 1978), specifically the mood (Ashby and Isen, 1999), feeding behavior (Szczypka et al., 1999), motivation (Blackburn et al., 1992), and decision-making (Assadi et al., 2009). Disruption of the brain reward system is a common observation in individuals with AN (Avena and Bocarsly, 2012). It has been proved that dopamine-related genes have been shown to influence the psychopathological features of EDs (Gervasini et al., 2013). Dopamine is thought to play a role in the development of AN by promoting reward and motivation (Franken et al., 2005). Higher levels of dopamine may make individuals with AN more susceptible to engaging in reward-seeking behaviors, such as restricting food intake (Ikemoto and Panksepp, 1999). A recent perspective article hypothesizes a two-stage role of dopamine in AN. In the first stage, when AN develops, dieting and exercise increase dopamine activity, which helps lock in the habits of severe dieting and weight loss. In the second stage, when these behaviors become deeply ingrained, an ongoing lack of food causes dopamine activity to drop as part of the body’s response to long-term stress (Beeler and Burghardt, 2022).
In the present systematic review, three studies investigated the association of genetic polymorphisms in the dopamine-related genes, including COMT (Favaro et al., 2013; Collantoni et al., 2016) as well as DRD2, DRD3, and DAT1 (González et al., 2021) with the psychological profiles and/or neurobiological characteristics of patients with AN. Figure 3 presents the key findings of the studies that explored the dopaminergic system. The COMT gene codes for catechol-O-methyltransferase, an enzyme that plays a role in the breakdown of catecholamine neurotransmitters such as dopamine, norepinephrine, and epinephrine (Qayyum et al., 2015), hence regulating synaptic dopamine (Malhotra et al., 2002). DRD2 and DRD3 code for 2 subtypes of Dopamine receptors, coding for D2 and D3 subtypes, respectively. They have been found to regulate the synthesis, storage, and release of dopamine (Cooper, 2011). Whereas the DAT1 gene (also known as SLC6A3) codes for dopamine transporter, an integral membrane protein that reuptakes and transports the dopamine from the synaptic cleft into the cytosol of surrounding cells (Baeuchl et al., 2019). Together, these three genes are an essential part of the dopamine pathway.
Firstly, the COMT gene was evaluated by two studies (Favaro et al., 2013; Collantoni et al., 2016) to investigate its association with the neurological factors of patients with AN. Favaro et al. (2013) studied the impact of the functional polymorphism Val158Met in the COMT gene on set-shifting abilities and prefrontal functional connectivity in patients with AN. The study provided evidence that the Val158Met COMT polymorphism has an effect on prefrontal cortex functional connectivity in patients with AN. In other words, Met-homozygous patients showed greater coactivation in the dorsolateral and ventromedial prefrontal cortex (PFC) compared to Val carriers. These brain areas relate to cognitive functions like conflict and error monitoring, reward-based learning, and decision-making (Nejati et al., 2021). Moreover, patients with an increasing number of Met alleles were positively associated with bad performance in the Wisconsin Card Sorting Test (WCST), which assesses abstract reasoning and set-shifting abilities and is considered a measure of executive functions (Kohli and Kaur, 2006). Hence, the study suggests that the studied genetic polymorphism in the COMT gene is associated with poor executive performance, in terms of inflexibility, and PFC functional connectivity in patients with AN. However, in a later study by Collantoni et al. (2016) SST psychological tool was used to assess the emotional response inhibition, behavioral inhibition, cognitive flexibility, and response monitoring in patients with AN. Interestingly, when investigating significant associations between SST reaction times and connectivity of the right inferior gyrus according to the presence of the Val158Met variant of the COMT genotype, no significant correlation was found.
Executive functions are cognitive processes that support goal-directed behavior (Luciana and Nelson, 2001). These processes are orchestrated by activity within the prefrontal cortex (Shimamura, 2000), including inhibition (behavioral and interference control), working memory, decision-making, problem-solving, and cognitive flexibility (mental flexibility or set shifting, closely associated with creativity) (Miyake et al., 2000; Lehto et al., 2003). In a recent separate study, it was confirmed that executive function impairment in ED is significantly associated with more severe symptoms and may result in a negative treatment outcome (Diaz-Marsa et al., 2023). This conclusion was reached after observing that patients with ED had significantly lower scores than HC in the performance of several executive function tests, particularly in the domains of set-shifting, interference control, and processing speed (Diaz-Marsa et al., 2023). According to the included study published by Favaro et al. (2013), patients with AN with Met homozygosity have poor set-shifting, leading to worse WCST performance than HC. The study also showed abnormal regional cortical processing in the prefrontal cortex area of the brain, which is responsible for executive functions, including cognitive flexibility and the other aforementioned processes. This can be explained by the effect of the Val158Met variant, a Val allele associated with a high-activity state of COMT, which increases the rate of dopamine degradation. This leads to lower levels of dopamine in PFC. On the other hand, the Met allele produces COMT with a low activity state, which slows the rate of degradation, resulting in higher levels of dopamine. Due to the crucial role that PFC dopamine plays in set-shifting and attention, these genetic-related differences in levels of PFC dopamine were found to be associated with different performances in executive function tasks (Esmaiel et al., 2020). Notably, elevated dopamine levels in the prefrontal cortex have been associated with impaired cognitive function mediated by this brain region since 1996 (Murphy et al., 1996). Contradictory to that conclusion, a systematic review done in 2020 suggested no association between the COMT gene and AN susceptibility. They suggested that the inconsistency observed could be due to the presence of other SNPs that are also in linkage disequilibrium or SNPs present in genes other than the COMT. These SNPs may have a controlling effect on the association of COMT Val158Met with AN patients (Abou Al Hassan et al., 2021).
Moreover, a large Spanish study by González et al. (2021) investigated genetic polymorphisms in 3 genes related to the dopaminergic system. This study evaluated the genetic polymorphisms of A2/A1 (rs1800497), Ser9Gly (rs6280) and 10R/9R (rs28363170) in the DRD2, DRD3, and DAT1 genes, respectively. Eating Disorder Inventory-2 (EDI-2) and the Symptom Checklist-90-Revised (SCL90R) were used as psychological assessment tools to investigate several AN-related symptoms, such as drive for thinness, bulimia, body dissatisfaction, ineffectiveness, perfectionism, interpersonal distrust, interoceptive awareness, maturity fears, asceticism, impulse regulation, and social insecurity, with and without the aforementioned genetic variations in DRD2, DRD3, and DAT1 genes. The study found that patients with AN who carried the Gly9Gly genotype in the dopamine D3 receptor had an overall significantly worse symptomatology than those with other genotypes. In essence, those patients had far higher EDI-2 scores than the rest of the patients did (relating to bulimia and interoceptive awareness as AN-related symptoms). Whereas for DAT1 gene, DAT1 9R/9R carriers had higher scores for both bulimia and asceticism compared with 10R/10R-10R/9R carriers. Elevated scores for perfectionism and ineffectiveness were displayed in DRD2 A1/A1 carriers. Taken together, this study indicates that these specific genetic variations in the dopaminergic DRD2, DRD3, and DAT1 genes have illustrated significant association with the predisposition with a more severe clinical picture of psychological manifestations presented in patients with AN.
In the past years, different polymorphisms of the DRD2, DRD3, and DAT1 genes appear to play a role in the vulnerability to AN (Gervasini et al., 2013). In 2005, a research paper concluded that the D2 receptor gene is a susceptibility factor in the development of AN, establishing a foundational link between genetic variations in DRD2 and EDs (Bergen et al., 2005). Beyond AN, DRD2 was also linked to an increased risk of developing pathological eating behavior (Nisoli et al., 2007). In fact, previous studies suggested that homozygous A1/A1 subjects possess a lower number of DRD2 in brain areas (Thompson et al., 1997). The A1 allele of DRD2 has been linked not only to perfectionism and ineffectiveness, but also to the dysregulation of the body’s primary stress systems, namely the hypothalamic–pituitary–adrenal (HPA) axis (Belda and Armario, 2009), which predisposes to worse AN symptoms. Furthermore, researchers have noted a specific correlation between self-oriented perfectionism and EDs, distinguishing it from correlations with depression or adaptive disorders (Castro-Fornieles et al., 2007). Regarding the DRD3 gene, our included study suggests a significant effect of the Gly9Gy genotype on worsened AN symptoms predisposition, especially for bulimia and interoceptive awareness (González et al., 2021). This can correlate to the DRD3 gene association with emotional and food motivational responses (Sokoloff et al., 1990), given that D3 receptor is expressed in brain regions thought to govern emotion and emotional responses to stress, reward motivation, and executive function (Sokoloff et al., 1990). Notably, the DRD3 gene was found to play a role in the etiology of a wide range of psychopathologies, including obsessive-compulsive personality disorder (Light et al., 2006), which was found to be a very common comorbidity of AN (Serpell et al., 2002). On the other hand, the DAT1 gene is associated with higher scores for asceticism in patients with AN (González et al., 2021). Asceticism is the practice of self-denial, often for religious or moral reasons. Individuals with AN may engage in asceticism as a way to control their weight or to express their distress. Interestingly, one paper found that as BMI decreases, religious favor of asceticism increases (Smith et al., 2004). Suggesting a potential link between asceticism and the drive for thinness, which is one of the most common motivational cues leading to EDs (Chernyak and Lowe, 2010).
3.7 Association of other gene polymorphisms with psychological and/or neurobiological factors in patients with AN
In this systematic review, five included studies investigated the association of genetic polymorphisms in several other genes, apart from these monoamine neurotransmitter genes, with the psychological profiles and/or neurobiological characteristics of patients with AN. Out of these 3 studies investigated the BDNF gene polymorphism (Rybakowski et al., 2007; Ando et al., 2012; Clarke et al., 2016), one study looked into the OXTR gene (Sala et al., 2018), and another study focused on the polymorphisms within two genes, KCTD1 and TFAP2B (Gamero-Villarroel et al., 2017). Figure 4 illustrates the main findings of this section.
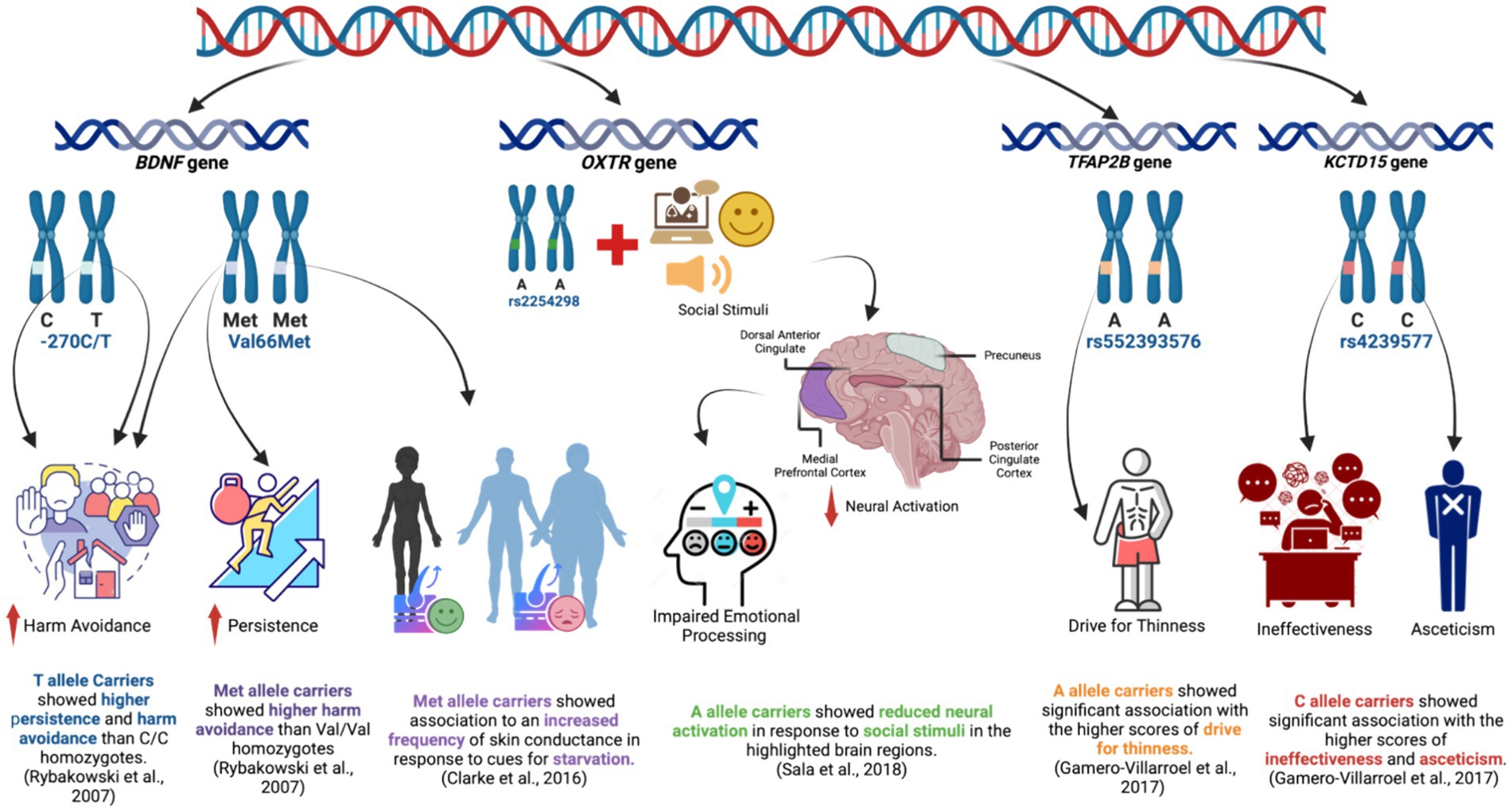
Figure 4. Key findings on the association of BNDF, OXTR, TFAP2B, and KCTD15 gene polymorphisms with psychological and/or neurobiological factors in patients with AN.
BDNF gene plays a crucial role in regulating neurotransmitter systems and has been of interest in the field of EDs (Hashimoto et al., 2005; Nakazato et al., 2012). The BDNF gene codes for the brain-derived neurotrophic factor (BDNF), a significant growth factor belonging to the neurotrophin family (Nagahara and Tuszynski, 2011). It greatly impacts the growth and differentiation of cells and also influences the plasticity of synapses and the survival of neurons (Bathina and Das, 2015). BDNF is released in varying amounts from neurons in different regions of the brain, such as the hippocampus and ventral tegmental area (Kowiański et al., 2018). These regions are known to play a vital role in learning and reward processes (Homan et al., 2014). In individuals with AN, these processes are believed to be affected (Wierenga et al., 2022). BDNF neurons in the anterior paraventricular nucleus of the hypothalamus suppress hunger and food intake while promoting physical activity (An et al., 2015). Therefore, there is substantial evidence that supports the interaction between BDNF and eating behavior, as well as the regulation of body weight. Interestingly, The Val66Met rs6265 in the BDNF gene is a widely researched polymorphism in brain function, mental health, and a plethora of mental disorders (Shen et al., 2018; Wang et al., 2023).
Included in our systematic review is one of the earliest studies by Rybakowski et al. (2007), where they analyzed two polymorphisms in the BDNF gene, the Val66Met and-270C/T using the Temperament and Character Inventory (TCI) tool to assess different personality dimensions including novelty-seeking, harm avoidance, reward dependence, persistence, self-directedness, cooperativeness, self-transcendence in AN and HC (Aluja et al., 2010). They found that the Val166Met was associated with harm avoidance observed in patients with AN while the-270C/T was associated with both higher persistence and harm avoidance. However, both were not directly associated with AN diagnosis from the psychological point of view; rather, both were found to predispose to higher risk affecting specific personality traits, including higher persistence and harm avoidance. Harm avoidance is characterized by excessive worrying, pessimism, shyness, and being fearful, suspicious, and easily fatigued (Karppanen et al., 2023), while persistence is the tendency to continue working on something notwithstanding fatigue or frustration (Constantin et al., 2012), which are both common observations patients with AN.
The variant Val66Met of the BDNF gene was also investigated by Ando et al. (2012) using the same psychological tool used by Rybakowski et al. (2007), TCI, with some modifications that would suit the Japanese population. Their findings were contrary to Rybakowski’s in terms of having lower harm avoidance scores among Met66 carriers with no other association with reward-seeking, reward dependence, and persistence between Met66 carriers and non-carriers patients with AN. This was supported by a family trio study, which suggested that BDNF Val66Met polymorphism may not have shown a significant association with AN (Dardennes et al., 2007).
Most recently, Clarke et al. (2016) investigated the correlation between a specific SNP and psychological traits in individuals with AN. The study utilized multiple psychological assessment tools, including the skin conductance response, which is a psychophysiological measure related to the autonomic nervous system (ANS) that is responsible for various involuntary bodily functions related to stress and arousal (Bradley et al., 2001). The results showed that individuals with AN had a higher frequency of skin conductance response when exposed to images of underweight bodies. This suggests that underweight body images had a more pronounced emotional impact on individuals with AN. The study also explored the association between the Val66Met polymorphism and the frequency of skin conductance response during the processing of underweight stimuli. The findings revealed a significant association between skin conductance response frequency and the presence of the BDNF Met genotype. In addition, the Body Shape Questionnaire (BSQ) was also used, which is a self-report tool that assesses body image concerns and dissatisfaction (Yurtsever et al., 2022). According to the study, individuals with AN experienced more positive feelings when processing images of underweight bodies (Clarke et al., 2016), suggesting that underweight stimuli evoked a distinct emotional reaction in patients with AN and could be related to their body image perception. On the other hand, patients with AN experienced more negative feelings when processing images of normal-weight and overweight bodies, indicating that individuals with AN may have heightened negative emotional responses to images of bodies within the normal or higher weight range (Clarke et al., 2016). These findings strongly support the distorted body image hallmark of patients with AN and suggest that the Val66Met polymorphism might play a role in mediating the heightened reward value associated with starvation imagery in AN.
In conclusion, these three aforementioned studies demonstrate that the BDNF gene, especially the Val66Met polymorphism, appears to be implicated in the personality traits, eating behaviors, and emotional responses associated with AN in different populations. Consistent with those findings, in a recent study conducted by Abou Al Hassan et al. (2021), a meta-analysis was performed to examine the influence of the BDNF gene on the progression of AN. Among various factors analyzed, the researchers found evidence suggesting a connection between the restrictive subtype of AN and the phenotypic distribution of the Val66Met gene polymorphism within the BDNF gene. Additionally, the presence of the Met66-allele was linked to typical clinical characteristics observed in individuals with AN (Abou Al Hassan et al., 2021). Animal studies on the same variant change were also shown to promote anorectic behavior in mice, when food restriction was paired with stress such as social isolation, but only in the peri-pubertal period (Madra and Zeltser, 2016).
Another gene studied in relation to AN is the OXTR gene, which codes for the oxytocin receptor protein. The role of the neuropeptide oxytocin (OT) in facilitating a range of social processes is well established (MacDonald and MacDonald, 2010). It was reported that OXTR polymorphisms are associated with social/emotional/behavioral functioning in children and adolescents (Kohlhoff et al., 2022). Sala et al. (2018), an included study in our systematic review, investigated two different SNPs in the OXTR gene, rs2254298 (G/A) and rs53576 (G/A), in patients with AN. They assessed the neural response to social stimuli using functional MRI in different brain regions. In contrast to previously reported data, no significant differences were found in the examined brain regions based on the rs53576 genotype. At the same time, carriers of the A allele for OXTR rs2254298 showed reduced neural activation in response to social stimuli in the medial prefrontal cortex, dorsal anterior cingulate, posterior cingulate cortex, and precuneus. Interestingly, the medial prefrontal cortex, dorsal anterior cingulate, posterior cingulate cortex, and precuneus are all areas associated with social cognition, emotional processing, and self-referential thinking (McAdams and Krawczyk, 2011, 2014; Schulte-Rüther et al., 2012; McAdams et al., 2015, 2016). Those processes are usually impaired in patients with AN (Oldershaw et al., 2011; Tchanturia et al., 2011; Lang et al., 2016). Consistent with this, another study showed that the A allele carriers of rs2254298 exhibited greater global social impairments (Parker et al., 2014). The study by Sala et al. (2018) further assessed its preliminary findings by the eating attitude test (EAT-26) as a psychological tool to assess the ED behaviors and ED symptomatology related to shape and weight concerns for significantly higher measures to be found for rs2254298A carriers compared to rs2244298GG carriers (Sala et al., 2018). That would suggest a potentially significant association between this genetic variation and disturbed body image and distorted perception of weight, which are central aspects of AN.
In a review of EDs and oxytocin receptor polymorphisms (Burmester et al., 2021), they observed that research on the OXTR SNPs rs53576 and rs2254298 has revealed distinct associations between genetic variations and eating behaviors. Specifically, the A allele of both SNPs is independently linked to restrictive eating behaviors, while the G allele of rs53576 is associated with binging behaviors, a correlation reflected in neuroanatomical findings (Micali et al., 2017). Interestingly, one study discovered that the A allele of OXTR SNPs poses a risk for more severe symptoms related to ED, whereas the G allele provides some protective effects (Acevedo et al., 2015). Despite these associations, individual OXTR SNPs alone are unlikely to fully explain the complexity of EDs, suggesting that they may influence the expression and/or effectiveness of the OXTR.
TFAP2B and KCTD1 are two other genes investigated in an included study by Gamero-Villarroel et al. (2017). Transcription Factor AP-2 Beta (TFAP2B) and the Potassium Channel Tetramerization Domain Containing 15 (KCTD15) are two obesity-related genes that interact to regulate feeding behavior (Williams et al., 2014). TFAP2B is a crucial regulator of monoaminergic genes (Damberg, 2005), while KCTD15 is reported to be associated with putative regulation of body mass index (BMI) (Mei et al., 2012). In the prespective included cross-sectional study, ten clinically relevant and tag single-nucleotide polymorphisms (SNPs) in KCTD15 and TFAP2B were screened in the included participants in both AN and HC. The EDI-2 and the anxiety subscale of the Symptom Checklist 90 Revised (SCL90R) were utilized as psychological assessment tools to assess all of the drive for thinness, bulimia, body dissatisfaction, ineffectiveness, perfectionism, interpersonal distrust, interoceptive awareness, maturity fears, asceticism, impulse regulation, and social insecurity. The results showed that the effect of the gene–gene interaction was more profound in scales such as perfectionism, maturity fears, or social insecurity for patients with AN. Moreover, in terms of the correlation with the studied SNPs, rs552393576 and rs2817420 in TFAP2B and rs4805059 and rs4239577 in KCTD15 showed significant relevant associations with the scores of several dimensions, including drive for thinness, ineffectiveness, and asceticism. In other words, the study suggests that genetic variability in TFAP2B and KCTD15 genes may influence personality dimensions related to AN, suggesting that the interaction of genetic variability in these loci could influence the risk for ED and/or psychological parameters.
Interestingly, both TFAP2B and KCTD15 genes were reported by multiple studies to be related to higher obesity risk (Bauer et al., 2009; Willer et al., 2009; Albuquerque et al., 2014; Lv et al., 2015) and there are many shared personality dimensions and risk factors between obesity and ED. These include body dissatisfaction, low self-esteem, anxiety, depression, substance abuse, dieting, binge-eating, and a history of sexual/physical abuse (Day et al., 2009). With that being said and given that both genes were found to alter personality dimensions, it is possible that genetic variations of both genes would not specifically predispose to more severe psychological manifestations of AN specifically, but rather in any eating disorder.
4 Conclusion
In conclusion, this comprehensive review highlights the intricate relationship between genetic polymorphisms and the psychological and neurobiological factors associated with AN. The serotoninergic system, particularly the 5-HTTLPR polymorphism, has been consistently implicated in altered functional connectivity within the ventral attention network, impaired inhibitory control, and an increased predisposition to AN. The findings emphasize the impact of the 5-HTTLPR polymorphism on the ventral attention network, shedding light on the potential mechanisms underlying altered reward processing, motivation, reasoning, working memory, inhibition, and outcome prediction in patients with AN. The dopaminergic system, encompassing genes such as COMT, DRD2, DRD3, and DAT1, plays a crucial role in regulating reward, motivation, and decision-making processes. Genetic variations in these dopaminergic genes have been linked to diverse psychological manifestations and clinical severity in AN patients.
The BDNF gene, specifically the Val66Met polymorphism, emerges as a significant player in influencing personality traits, eating behaviors, and emotional responses associated with AN across diverse populations. The OXTR gene, responsible for oxytocin receptor protein, and other genes like TFAP2B and KCTD15, reveal intriguing associations with social cognition, emotional processing, body image concerns, and personality dimensions in patients with AN. It is evident that genetic variations contribute to the complexity of AN, influencing not only the susceptibility to the disorder but also shaping its diverse clinical presentations. Future research should continue exploring the interplay between genetic factors and environmental influences to provide a more comprehensive understanding of the etiology and pathophysiology of AN. There is a need for further investigation to extend these findings to eating pathology. The use of Genome-Wide Association Studies (GWAS) could significantly contribute to expanding our knowledge in this area, shedding light on the intricate interplay between genetics and environmental factors in the development of EDs. This knowledge could pave the way for personalized therapeutic interventions that target specific genetic vulnerabilities, ultimately improving outcomes for individuals affected by this challenging and potentially life-threatening condition.
5 Strengths and limitations
This is the first systematic review to evaluate the genetic polymorphisms and their association with neurobiological and psychological factors in anorexia nervosa status and summarize almost all the available evidence regarding this association. In addition, a rigorous systematic approach was followed to develop this review, such as the PRISMA guidelines and the Rayyan application, which was used to perform initial screening and enabled the research team to conduct a blind review that would enhance the credibility of the findings. Additionally, the studies included were conducted on a large number of participants, who were relatively homogenous for their age (mainly adolescents) and health status (all cases were clinically diagnosed according to internationally established criteria). Additionally, the studies included in this systematic review were conducted in various countries, which allows us to see the influence of different genetic polymorphisms on neurobiological and psychological factors in individuals with various genetic backgrounds. Furthermore, the quality of the included studies was mainly high, and only two out of eleven studies were of stationary quality, which indicates that the evidence drawn from these studies is unlikely to be biased or the result of other uncontrolled confounding factors.
Despite the valuable insights provided by the reviewed studies on the association between genetic polymorphisms and psychological/neurobiological factors in AN, several limitations should be considered. Many studies exhibit small sample sizes, potentially compromising the generalizability of findings. Using cross-sectional designs might limit the ability to establish causation or determine the direction of relationships. Publication bias may skew the overall understanding, as positive results are more likely to be published. Multiple testing and the lack of replication studies pose risks of false-positive results. Psychological assessment tools vary across studies, impacting the comparability of results. The complexity of gene–gene interactions and the functional implications of genetic variations require further exploration. Addressing these limitations in future research endeavors will contribute to a more nuanced understanding of the genetic factors influencing AN.
Data availability statement
The original contributions presented in the study are included in the article/
Author contributions
HA: Writing – original draft, Visualization, Methodology, Investigation, Data curation, Conceptualization. HB: Writing – review & editing, Validation, Supervision.
Funding
The author(s) declare that financial support was received for the research, authorship, and/or publication of this article. The APC fund was provided by Qatar University with the Qatar University grant: QUST-1-CHS-2024-1672.
Acknowledgments
We would like to thank Qatar University as the APC fund was provided with the Qatar University grant: QUST-1-CHS-2024-1672.
Conflict of interest
The authors declare that the research was conducted in the absence of any commercial or financial relationships that could be construed as a potential conflict of interest.
Publisher’s note
All claims expressed in this article are solely those of the authors and do not necessarily represent those of their affiliated organizations, or those of the publisher, the editors and the reviewers. Any product that may be evaluated in this article, or claim that may be made by its manufacturer, is not guaranteed or endorsed by the publisher.
Supplementary material
The Supplementary material for this article can be found online at: https://www.frontiersin.org/articles/10.3389/fpsyg.2024.1386233/full#supplementary-material
Footnotes
References
Abou Al Hassan, S., Cutinha, D., and Mattar, L. (2021). The impact of COMT, BDNF and 5-HTT brain-genes on the development of anorexia nervosa: a systematic review. Eat. Weight Disord. 26, 1323–1344. doi: 10.1007/s40519-020-00978-5
Acevedo, S. F., Valencia, C., Lutter, M., and McAdams, C. J. (2015). Severity of eating disorder symptoms related to oxytocin receptor polymorphisms in anorexia nervosa. Psychiatry Res. 228, 641–648. doi: 10.1016/j.psychres.2015.05.040
Albuquerque, D., Nóbrega, C., Rodríguez-López, R., and Manco, L. (2014). Association study of common polymorphisms in MSRA, TFAP2B, MC4R, NRXN3, PPARGC1A, TMEM18, SEC16B, HOXB5 and OLFM4 genes with obesity-related traits among Portuguese children. J. Hum. Genet. 59, 307–313. doi: 10.1038/jhg.2014.23
Allen, N. C., Bagade, S., McQueen, M. B., Ioannidis, J. P. A., Kavvoura, F. K., Khoury, M. J., et al. (2008). Systematic meta-analyses and field synopsis of genetic association studies in schizophrenia: the SzGene database. Nat. Genet. 40, 827–834. doi: 10.1038/ng.171
Aluja, A., Blanch, A., Gallart, S., and Dolcet, J.-M. (2010). The temperament and character inventory revised (TCI-R): descriptive and factor structure in different age levels. Psicol. Conduct. 18:385,
An, J. J., Liao, G. Y., Kinney, C. E., Sahibzada, N., and Xu, B. (2015). Discrete BDNF neurons in the paraventricular hypothalamus control feeding and energy expenditure. Cell Metab. 22, 175–188. doi: 10.1016/j.cmet.2015.05.008
Ando, T., Ishikawa, T., Hotta, M., Naruo, T., Okabe, K., Nakahara, T., et al. (2012). No association of brain-derived neurotrophic factor Val66Met polymorphism with anorexia nervosa in Japanese. Am. J. Med. Genet. B Neuropsychiatr. Genet. 159B, 48–52. doi: 10.1002/ajmg.b.32000
Arcelus, J., Mitchell, A. J., Wales, J., and Nielsen, S. (2011). Mortality rates in patients with anorexia nervosa and other eating disorders: a meta-analysis of 36 studies. Arch. Gen. Psychiatry 68, 724–731. doi: 10.1001/archgenpsychiatry.2011.74
Ashby, F. G., and Isen, A. M. (1999). A neuropsychological theory of positive affect and its influence on cognition. Psychol. Rev. 106, 529–550. doi: 10.1037/0033-295X.106.3.529
Assadi, S. M., Yücel, M., and Pantelis, C. (2009). Dopamine modulates neural networks involved in effort-based decision-making. Neurosci. Biobehav. Rev. 33, 383–393. doi: 10.1016/j.neubiorev.2008.10.010
Attia, E. (2010). Anorexia nervosa: current status and future directions. Annu. Rev. Med. 61, 425–435. doi: 10.1146/annurev.med.050208.200745
Auger, N., Steiger, H., Luu, T. M., Chadi, N., Low, N., Bilodeau-Bertrand, M., et al. (2023). Shifting age of child eating disorder hospitalizations during the Covid-19 pandemic. J. Child Psychol. Psychiatry 64, 1176–1184. doi: 10.1111/jcpp.13800
Avena, N. M., and Bocarsly, M. E. (2012). Dysregulation of brain reward systems in eating disorders: neurochemical information from animal models of binge eating, bulimia nervosa, and anorexia nervosa. Neuropharmacology 63, 87–96. doi: 10.1016/j.neuropharm.2011.11.010
Baeuchl, C., Chen, H. Y., Su, Y. S., Hämmerer, D., Klados, M. A., and Li, S. C. (2019). Interactive effects of dopamine transporter genotype and aging on resting-state functional networks. PLoS One 14:e0215849. doi: 10.1371/journal.pone.0215849
Baker, J. H., Schaumberg, K., and Munn-Chernoff, M. A. (2017). Genetics of anorexia nervosa. Curr. Psychiatry Rep. 19:84. doi: 10.1007/s11920-017-0842-2
Bathina, S., and Das, U. N. (2015). Brain-derived neurotrophic factor and its clinical implications. Arch. Med. Sci. 11, 1164–1178. doi: 10.5114/aoms.2015.56342
Bauer, F., Elbers, C. C., Adan, R. A. H., Loos, R. J. F., Onland-Moret, N. C., Grobbee, D. E., et al. (2009). Obesity genes identified in genome-wide association studies are associated with adiposity measures and potentially with nutrient-specific food preference. Am. J. Clin. Nutr. 90, 951–959. doi: 10.3945/ajcn.2009.27781
Beeler, J. A., and Burghardt, N. S. (2022). The rise and fall of dopamine: a two-stage model of the development and entrenchment of anorexia nervosa. Front. Psych. 12:799548. doi: 10.3389/fpsyt.2021.799548
Belda, X., and Armario, A. (2009). Dopamine D1 and D2 dopamine receptors regulate immobilization stress-induced activation of the hypothalamus-pituitary-adrenal axis. Psychopharmacology 206, 355–365. doi: 10.1007/s00213-009-1613-5
Beliveau, V., Ganz, M., Feng, L., Ozenne, B., Højgaard, L., Fisher, P. M., et al. (2017). A high-resolution in vivo atlas of the human Brain's serotonin system. J. Neurosci. 37, 120–128. doi: 10.1523/JNEUROSCI.2830-16.2016
Bergen, A. W., Yeager, M., Welch, R. A., Haque, K., Ganjei, J. K., van den Bree, M. B. M., et al. (2005). Association of multiple DRD2 polymorphisms with anorexia nervosa. Neuropsychopharmacology 30, 1703–1710. doi: 10.1038/sj.npp.1300719
Blackburn, J. R., Pfaus, J. G., and Phillips, A. G. (1992). Dopamine functions in appetitive and defensive behaviours. Prog. Neurobiol. 39, 247–279. doi: 10.1016/0301-0082(92)90018-A
Blake, P., Johnson, B., and VanMeter, J. W. (2003). Positron emission tomography (PET) and single photon emission computed tomography (SPECT): clinical applications. J. Neuroophthalmol. 23, 34–41. doi: 10.1097/00041327-200303000-00009
Boehm, I., Walton, E., Alexander, N., Batury, V. L., Seidel, M., Geisler, D., et al. (2020). Peripheral serotonin transporter DNA methylation is linked to increased salience network connectivity in females with anorexia nervosa. J. Psychiatry Neurosci. 45, 206–213. doi: 10.1503/jpn.190016
Boerner, L. M., Spillane, N. S., Anderson, K. G., and Smith, G. T. (2004). Similarities and differences between women and men on eating disorder risk factors and symptom measures. Eat. Behav. 5, 209–222. doi: 10.1016/j.eatbeh.2004.01.011
Bradley, M. M., Codispoti, M., Cuthbert, B. N., and Lang, P. J. (2001). Emotion and motivation I: defensive and appetitive reactions in picture processing. Emotion 1, 276–298. doi: 10.1037/1528-3542.1.3.276
Bulik, C. M., Hebebrand, J., Keski-Rahkonen, A., Klump, K. L., Reichborn-Kjennerud, T., Mazzeo, S. E., et al. (2007). Genetic epidemiology, endophenotypes, and eating disorder classification. Int. J. Eat. Disord. 40, S52–S60. doi: 10.1002/eat.20398
Bulik, C. M., Thornton, L. M., Root, T. L., Pisetsky, E. M., Lichtenstein, P., and Pedersen, N. L. (2010). Understanding the relation between anorexia nervosa and bulimia nervosa in a Swedish national twin sample. Biol. Psychiatry 67, 71–77. doi: 10.1016/j.biopsych.2009.08.010
Burmester, V., Nicholls, D., Buckle, A., Stanojevic, B., and Crous-Bou, M. (2021). Review of eating disorders and oxytocin receptor polymorphisms. Eat. Disord. 9:85. doi: 10.1186/s40337-021-00438-0
Calvo-Rivera, M. P., Navarrete-Páez, M. I., Bodoano, I., and Gutiérrez-Rojas, L. (2022). Comorbidity between anorexia nervosa and depressive disorder: a narrative review. Psychiatry Investig. 19, 155–163. doi: 10.30773/pi.2021.0188
Campbell, K., and Peebles, R. (2014). Eating disorders in children and adolescents: state of the art review. Pediatrics 134, 582–592. doi: 10.1542/peds.2014-0194
Caspi, A., Sugden, K., Moffitt, T. E., Taylor, A., Craig, I. W., Harrington, H. L., et al. (2003). Influence of life stress on depression: moderation by a polymorphism in the 5-HTT gene. Science 301, 386–389. doi: 10.1126/science.1083968
Castro-Fornieles, J., Gual, P., Lahortiga, F., Gila, A., Casulà, V., Fuhrmann, C., et al. (2007). Self-oriented perfectionism in eating disorders. Int. J. Eat. Disord. 40, 562–568. doi: 10.1002/eat.20393
Cha, J., Ide, J. S., Bowman, F. D., Simpson, H. B., Posner, J., and Steinglass, J. E. (2016). Abnormal reward circuitry in anorexia nervosa: a longitudinal, multimodal MRI study. Hum. Brain Mapp. 37, 3835–3846. doi: 10.1002/hbm.23279
Chen, J., Kang, Q., Jiang, W., Fan, J., Zhang, M., Yu, S., et al. (2015). The 5-HTTLPR confers susceptibility to anorexia nervosa in Han Chinese: evidence from a case-control and family-based study. PLoS One 10:e0119378. doi: 10.1371/journal.pone.0119378
Chen, W., and Qian, J. (2015). The association of 5-HTTLPR gene polymorphisms and eating disorder: a meta-analysis. J. Psychol. Psychother 5, 2161–0487.1000214. doi: 10.4172/2161-0487.1000214
Chernyak, Y., and Lowe, M. R. (2010). Motivations for dieting: drive for thinness is different from drive for objective thinness. J. Abnorm. Psychol. 119, 276–281. doi: 10.1037/a0018398
Clarke, J., Ramoz, N., Fladung, A. K., and Gorwood, P. (2016). Higher reward value of starvation imagery in anorexia nervosa and association with the Val66Met BDNF polymorphism. Transl. Psychiatry 6:e829. doi: 10.1038/tp.2016.98
Collantoni, E., Michelon, S., Tenconi, E., Degortes, D., Titton, F., Manara, R., et al. (2016). Functional connectivity correlates of response inhibition impairment in anorexia nervosa. Psychiatry Res. Neuroimaging 247, 9–16. doi: 10.1016/j.pscychresns.2015.11.008
Compan, V. (2021). Serotonin 4 receptors: a cornerstone in adaptive feeding response to stress? Appetite 157:104968. doi: 10.1016/j.appet.2020.104968
Constantin, T., Holman, A. C., and Hojbotă, M. A. (2012). Development and validation of a motivational persistence scale. Psihologija 45, 99–120. doi: 10.2298/PSI1202099C
Cooper, D. C. , Introduction to neuroscience. (2011). Available at: https://www.cambridge.org/core/books/abs/cambridge-handbook-of-clinical-assessment-and-diagnosis/assessment-of-eating-disorders/D2106C344BC89CDE8090C965CE52ECE1
Corbetta, M., and Shulman, G. L. (2002). Control of goal-directed and stimulus-driven attention in the brain. Nat. Rev. Neurosci. 3, 201–215. doi: 10.1038/nrn755
Crisp, A.Collaborators (2006). Anorexia nervosa in males: similarities and differences to anorexia nervosa in females. Eur. Eat. Disord. Rev. 14, 163–167. doi: 10.1002/erv.703
Damberg, M. (2005). Transcription factor AP-2 and monoaminergic functions in the central nervous system. J. Neural Transm. 112, 1281–1296. doi: 10.1007/s00702-005-0325-1
Dardennes, R. M., Zizzari, P., Tolle, V., Foulon, C., Kipman, A., Romo, L., et al. (2007). Family trios analysis of common polymorphisms in the obestatin/ghrelin, BDNF and AGRP genes in patients with anorexia nervosa: association with subtype, body-mass index, severity and age of onset. Psychoneuroendocrinology 32, 106–113. doi: 10.1016/j.psyneuen.2006.11.003
Day, J., Ternouth, A., and Collier, D. A. (2009). Eating disorders and obesity: two sides of the same coin? Epidemiol. Psichiatr. Soc. 18, 96–100. doi: 10.1017/S1121189X00000956
Diaz-Marsa, M., Pemau, A., de la Torre-Luque, A., Vaz-Leal, F., Rojo-Moreno, L., Beato-Fernandez, L., et al. (2023). Executive dysfunction in eating disorders: relationship with clinical features. Prog. Neuro-Psychopharmacol. Biol. Psychiatry 120:110649. doi: 10.1016/j.pnpbp.2022.110649
Donato, K., Ceccarini, M. R., Dhuli, K., Bonetti, G., Medori, M. C., Marceddu, G., et al. (2022). Gene variants in eating disorders. Focus on anorexia nervosa, bulimia nervosa, and binge-eating disorder. J. Prev. Med. Hyg. 63, E297–e305. doi: 10.15167/2421-4248/jpmh2022.63.2S3.2772
Esmaiel, N. N., Ashaat, E. A., Mosaad, R., Fayez, A., Ibrahim, M., Abdallah, Z. Y., et al. (2020). The potential impact of COMT gene variants on dopamine regulation and phenotypic traits of ASD patients. Behav. Brain Res. 378:112272. doi: 10.1016/j.bbr.2019.112272
Fairburn, C. G., and Harrison, P. J. (2003). Risk factors for anorexia nervosa. Lancet 361:1914. doi: 10.1016/S0140-6736(03)13529-5
Favaro, A., Clementi, M., Manara, R., Bosello, R., Forzan, M., Bruson, A., et al. (2013). Catechol-O-methyltransferase genotype modifies executive functioning and prefrontal functional connectivity in women with anorexia nervosa. J. Psychiatry Neurosci. 38, 241–248. doi: 10.1503/jpn.120068
Favaro, A., Zanetti, T., Tenconi, E., Degortes, D., Ronzan, A., Veronese, A., et al. (2004). The relationship between temperament and impulsive behaviors in eating disordered subjects. Eat. Disord. 13, 61–70. doi: 10.1080/10640260590893647
Fonville, L., Giampietro, V., Surguladze, S., Williams, S., and Tchanturia, K. (2014). Increased BOLD signal in the fusiform gyrus during implicit emotion processing in anorexia nervosa. Neuroimage 4, 266–273. doi: 10.1016/j.nicl.2013.12.002
Frank, G. K., DeGuzman, M. C., Shott, M. E., Laudenslager, M. L., Rossi, B., and Pryor, T. (2018). Association of brain reward learning response with harm avoidance, weight gain, and hypothalamic effective connectivity in adolescent anorexia nervosa. JAMA Psychiatry 75, 1071–1080. doi: 10.1001/jamapsychiatry.2018.2151
Frank, G. K., Shott, M. E., Hagman, J. O., and Yang, T. T. (2013). Localized brain volume and white matter integrity alterations in adolescent anorexia nervosa. J. Am. Acad. Child Adolesc. Psychiatry 52, 1066–1075.e5. e5. doi: 10.1016/j.jaac.2013.07.007
Franken, I. H. A., Booij, J., and van den Brink, W. (2005). The role of dopamine in human addiction: from reward to motivated attention. Eur. J. Pharmacol. 526, 199–206. doi: 10.1016/j.ejphar.2005.09.025
Frisch, A., Laufer, N., Danziger, Y., Michaelovsky, E., Leor, S., Carel, C., et al. (2001). Association of anorexia nervosa with the high activity allele of the COMT gene: a family-based study in Israeli patients. Mol. Psychiatry 6, 243–245. doi: 10.1038/sj.mp.4000830
Gamero-Villarroel, C., González, L. M., Rodríguez-López, R., Albuquerque, D., Carrillo, J. A., García-Herráiz, A., et al. (2017). Influence of TFAP2B and KCTD15 genetic variability on personality dimensions in anorexia and bulimia nervosa. Brain Behav. 7:e00784. doi: 10.1002/brb3.784
Genis-Mendoza, A. D., Ruiz-Ramos, D., López-Narvaez, M. L., Tovilla-Zárate, C. A., Rosa García, A., Cortes Meda, G., et al. (2019). Genetic association analysis of 5-HTR2A gene variants in eating disorders in a Mexican population. Brain Behav. 9:e01286. doi: 10.1002/brb3.1286
Gervasini, G., Gordillo, I., Garcia-Herraiz, A., Flores, I., Jiménez, M., Monge, M., et al. (2012). Polymorphisms in serotonergic genes and psychopathological traits in eating disorders. J. Clin. Psychopharmacol. 32, 426–428. doi: 10.1097/JCP.0b013e3182539f2b
Gervasini, G., Gordillo, I., García-Herráiz, A., Flores, I., Jiménez, M., Monge, M., et al. (2013). Influence of dopamine polymorphisms on the risk for anorexia nervosa and associated psychopathological features. J. Clin. Psychopharmacol. 33, 551–555. doi: 10.1097/JCP.0b013e3182970469
González, L. M., Mota-Zamorano, S., García-Herráiz, A., López-Nevado, E., and Gervasini, G. (2021). Genetic variants in dopamine pathways affect personality dimensions displayed by patients with eating disorders. Eat. Weight Disord. 26, 93–101. doi: 10.1007/s40519-019-00820-7
Hariri, A. R., Mattay, V. S., Tessitore, A., Kolachana, B., Fera, F., Goldman, D., et al. (2002). Serotonin transporter genetic variation and the response of the human amygdala. Science 297, 400–403. doi: 10.1126/science.1071829
Hasan, T. F., and Hunaid, H. (2011). Anorexia nervosa: a unified neurological perspective. Int. J. Med. Sci. 8, 679–703. doi: 10.7150/ijms.8.679
Hashimoto, K., Koizumi, H., Nakazato, M., Shimizu, E., and Iyo, M. (2005). Role of brain-derived neurotrophic factor in eating disorders: recent findings and its pathophysiological implications. Prog. Neuro-Psychopharmacol. Biol. Psychiatry 29, 499–504. doi: 10.1016/j.pnpbp.2005.01.007
Heils, A., Teufel, A., Petri, S., Stöber, G., Riederer, P., Bengel, D., et al. (1996). Allelic variation of human serotonin transporter gene expression. J. Neurochem. 66, 2621–2624. doi: 10.1046/j.1471-4159.1996.66062621.x
Herzog, W., Deter, H. C., Fiehn, W., and Petzold, E. (1997). Medical findings and predictors of long-term physical outcome in anorexia nervosa: a prospective, 12-year follow-up study. Psychol. Med. 27, 269–279. doi: 10.1017/S0033291796004394
Homan, P., Grob, S., Milos, G., Schnyder, U., Eckert, A., Lang, U., et al. (2014). The role of BDNF, leptin, and catecholamines in reward learning in bulimia nervosa. Int. J. Neuropsychopharmacol. 18:pyu092. doi: 10.1093/ijnp/pyu092
Ikemoto, S., and Panksepp, J. (1999). The role of nucleus accumbens dopamine in motivated behavior: a unifying interpretation with special reference to reward-seeking. Brain Res. Rev. 31, 6–41. doi: 10.1016/S0165-0173(99)00023-5
Karppanen, A.-K., Miettunen, J., Hurtig, T., Nordström, T., Tammelin, T., and Korpelainen, R. (2023). Temperament and longitudinal changes in physical activity – the northern Finland birth cohort 1966 study. BMC Public Health 23:426. doi: 10.1186/s12889-023-15303-9
Kim, Y. R., Woo, J. M., Heo, S. Y., Kim, J. H., Lim, S. J., and Yu, B. H. (2009). An association study of the A218C polymorphism of the tryptiophan hydroxylase I gene with eating disorders in a Korean population: a pilot study. Psychiatry Investig. 6, 44–49. doi: 10.4306/pi.2009.6.1.44
Kirk, K. M., Martin, F. C., Mao, A., Parker, R., Maguire, S., Thornton, L. M., et al. (2017). The anorexia nervosa genetics initiative: study description and sample characteristics of the Australian and New Zealand arm. Aust. N. Z. J. Psychiatry 51, 583–594. doi: 10.1177/0004867417700731
Klump, K. L., Bulik, C. M., Kaye, W. H., Treasure, J., and Tyson, E. (2009). Academy for eating disorders position paper: eating disorders are serious mental illnesses. Int. J. Eat. Disord. 42, 97–103. doi: 10.1002/eat.20589
Klump, K. L., Miller, K. B., Keel, P. K., McGue, M., and Iacono, W. G. (2001). Genetic and environmental influences on anorexia nervosa syndromes in a population–based twin sample. Psychol. Med. 31, 737–740. doi: 10.1017/S0033291701003725
Kohlhoff, J., Cibralic, S., Hawes, D. J., and Eapen, V. (2022). Oxytocin receptor gene (OXTR) polymorphisms and social, emotional and behavioral functioning in children and adolescents: a systematic narrative review. Neurosci. Biobehav. Rev. 135:104573. doi: 10.1016/j.neubiorev.2022.104573
Kohli, A., and Kaur, M. (2006). Wisconsin card sorting test: normative data and experience. Indian J. Psychiatry 48, 181–184. doi: 10.4103/0019-5545.31582
Kowiański, P., Lietzau, G., Czuba, E., Waśkow, M., Steliga, A., and Moryś, J. (2018). BDNF: a key factor with multipotent impact on brain signaling and synaptic plasticity. Cell. Mol. Neurobiol. 38, 579–593. doi: 10.1007/s10571-017-0510-4
Krawczyk, D. C. (2002). Contributions of the prefrontal cortex to the neural basis of human decision making. Neurosci. Biobehav. Rev. 26, 631–664. doi: 10.1016/S0149-7634(02)00021-0
Lang, K., Larsson, E. E. C., Mavromara, L., Simic, M., Treasure, J., and Tchanturia, K. (2016). Diminished facial emotion expression and associated clinical characteristics in anorexia nervosa. Psychiatry Res. 236, 165–172. doi: 10.1016/j.psychres.2015.12.004
Lee, S., Ran Kim, K., Ku, J., Lee, J. H., Namkoong, K., and Jung, Y. C. (2014). Resting-state synchrony between anterior cingulate cortex and precuneus relates to body shape concern in anorexia nervosa and bulimia nervosa. Psychiatry Res. 221, 43–48. doi: 10.1016/j.pscychresns.2013.11.004
Lehto, J. E., Juujärvi, P., Kooistra, L., and Pulkkinen, L. (2003). Dimensions of executive functioning: evidence from children. Br. J. Dev. Psychol. 21, 59–80. doi: 10.1348/026151003321164627
Lesch, K.-P., Bengel, D., Heils, A., Sabol, S. Z., Greenberg, B. D., Petri, S., et al. (1996). Association of anxiety-related traits with a polymorphism in the serotonin transporter gene regulatory region. Science 274, 1527–1531. doi: 10.1126/science.274.5292.1527
Li, Q. (2006). Cellular and molecular alterations in mice with deficient and reduced serotonin transporters. Mol. Neurobiol. 34, 51–66. doi: 10.1385/MN:34:1:51
Liberati, A., Altman, D. G., Tetzlaff, J., Mulrow, C., Gøtzsche, P. C., Ioannidis, J. P. A., et al. (2009). The PRISMA statement for reporting systematic reviews and meta-analyses of studies that evaluate health care interventions: explanation and elaboration. J. Clin. Epidemiol. 62, e1–e34. doi: 10.1016/j.jclinepi.2009.06.006
Light, K. J., Joyce, P. R., Luty, S. E., Mulder, R. T., Frampton, C. M. A., Joyce, L. R. M., et al. (2006). Preliminary evidence for an association between a dopamine D3 receptor gene variant and obsessive-compulsive personality disorder in patients with major depression. Am. J. Med. Genet. B Neuropsychiatr. Genet. 141B, 409–413. doi: 10.1002/ajmg.b.30308
Lippa, A. S., Antelman, S. M., Fisher, A. E., and Canfield, D. R. (1973). Neurochemical mediation of reward: a significant role for dopamine? Pharmacol. Biochem. Behav. 1, 23–28. doi: 10.1016/0091-3057(73)90050-6
Lloyd, S., Yiend, J., Schmidt, U., and Tchanturia, K. (2014). Perfectionism in anorexia nervosa: novel performance based evidence. PLoS One 9:e111697. doi: 10.1371/journal.pone.0111697
Lv, D., Zhang, D. D., Wang, H., Zhang, Y., Liang, L., Fu, J. F., et al. (2015). Genetic variations in SEC16B, MC4R, MAP2K5 and KCTD15 were associated with childhood obesity and interacted with dietary behaviors in Chinese school-age population. Gene 560, 149–155. doi: 10.1016/j.gene.2015.01.054
MacDonald, K., and MacDonald, T. M. (2010). The peptide that binds. Harv. Rev. Psychiatry 18, 1–21. doi: 10.3109/10673220903523615
Madra, M., and Zeltser, L. M. (2016). BDNF-Val66Met variant and adolescent stress interact to promote susceptibility to anorexic behavior in mice. Transl. Psychiatry 6:e776. doi: 10.1038/tp.2016.35
Malhotra, A. K., Kestler, L. J., Mazzanti, C., Bates, J. A., Goldberg, T., and Goldman, D. (2002). A functional polymorphism in the COMT gene and performance on a test of prefrontal cognition. Am. J. Psychiatry 159, 652–654. doi: 10.1176/appi.ajp.159.4.652
Marazziti, D. (2017). Understanding the role of serotonin in psychiatric diseases. F1000Res 6:180. doi: 10.12688/f1000research.10094.1
Martin Monzon, B., Henderson, L. A., Madden, S., Macefield, V. G., Touyz, S., Kohn, M. R., et al. (2017). Grey matter volume in adolescents with anorexia nervosa and associated eating disorder symptoms. Eur. J. Neurosci. 46, 2297–2307. doi: 10.1111/ejn.13659
Mazzeo, S. E., and Bulik, C. M. (2009). Environmental and genetic risk factors for eating disorders: what the clinician needs to know. Child Adolesc. Psychiatr. Clin. N. Am. 18, 67–82. doi: 10.1016/j.chc.2008.07.003
McAdams, C. J., Jeon-Slaughter, H., Evans, S., Lohrenz, T., Montague, P. R., and Krawczyk, D. C. (2016). Neural differences in self-perception during illness and after weight-recovery in anorexia nervosa. Soc. Cogn. Affect. Neurosci. 11, 1823–1831. doi: 10.1093/scan/nsw092
McAdams, C. J., and Krawczyk, D. C. (2011). Impaired neural processing of social attribution in anorexia nervosa. Psychiatry Res. 194, 54–63. doi: 10.1016/j.pscychresns.2011.06.016
McAdams, C. J., and Krawczyk, D. C. (2014). Who am I? How do I look? Neural differences in self-identity in anorexia nervosa. Soc. Cogn. Affect. Neurosci. 9, 12–21. doi: 10.1093/scan/nss093
McAdams, C. J., Lohrenz, T., and Montague, P. R. (2015). Neural responses to kindness and malevolence differ in illness and recovery in women with anorexia nervosa. Hum. Brain Mapp. 36, 5207–5219. doi: 10.1002/hbm.23005
McFadden, K. L., Tregellas, J., Shott, M., and Frank, G. (2014). Reduced salience and default mode network activity in women with anorexia nervosa. J. Psychiatry Neurosci. 39, 178–188. doi: 10.1503/jpn.130046
Mei, H., Chen, W., Jiang, F., He, J., Srinivasan, S., Smith, E. N., et al. (2012). Longitudinal replication studies of GWAS risk SNPs influencing body mass index over the course of childhood and adulthood. PLoS One 7:e31470. doi: 10.1371/journal.pone.0031470
Mia, M. A., Uddin, M. N., Akter, Y., Jesmin,, and Marzan, L. W. (2022). Exploring the structural and functional effects of nonsynonymous SNPs in the human serotonin transporter gene through in silico approaches. Bioinform. Biol. Insights 16:11779322221104308. doi: 10.1177/11779322221104308
Micali, N., Crous-Bou, M., Treasure, J., and Lawson, E. A. (2017). Association between oxytocin receptor genotype, maternal care, and eating disorder behaviours in a community sample of women. Eur. Eat. Disord. Rev. 25, 19–25. doi: 10.1002/erv.2486
Miyake, A., Friedman, N. P., Emerson, M. J., Witzki, A. H., Howerter, A., and Wager, T. D. (2000). The unity and diversity of executive functions and their contributions to complex "frontal lobe" tasks: a latent variable analysis. Cogn. Psychol. 41, 49–100. doi: 10.1006/cogp.1999.0734
Moher, D., Liberati, A., Tetzlaff, J., and Altman, D. G.The PRISMA Group (2009). Preferred reporting items for systematic reviews and meta-analyses: the PRISMA statement. PLoS Med. 6:e1000097. doi: 10.1371/journal.pmed.1000097
Monteleone, P., and Maj, M. (2013). Dysfunctions of leptin, ghrelin, BDNF and endocannabinoids in eating disorders: beyond the homeostatic control of food intake. Psychoneuroendocrinology 38, 312–330. doi: 10.1016/j.psyneuen.2012.10.021
Monteleone, P., Matias, I., Martiadis, V., de Petrocellis, L., Maj, M., and di Marzo, V. (2005). Blood levels of the endocannabinoid anandamide are increased in anorexia nervosa and in binge-eating disorder, but not in bulimia nervosa. Neuropsychopharmacology 30, 1216–1221. doi: 10.1038/sj.npp.1300695
Murphy, B. L., Arnsten, A. F., Goldman-Rakic, P. S., and Roth, R. H. (1996). Increased dopamine turnover in the prefrontal cortex impairs spatial working memory performance in rats and monkeys. Proc. Natl. Acad. Sci. USA 93, 1325–1329. doi: 10.1073/pnas.93.3.1325
Nagahara, A. H., and Tuszynski, M. H. (2011). Potential therapeutic uses of BDNF in neurological and psychiatric disorders. Nat. Rev. Drug Discov. 10, 209–219. doi: 10.1038/nrd3366
Nakazato, M., Hashimoto, K., Shimizu, E., Niitsu, T., and Iyo, M. (2012). Possible involvement of brain-derived neurotrophic factor in eating disorders. IUBMB Life 64, 355–361. doi: 10.1002/iub.1012
Nejati, V., Majdi, R., Salehinejad, M. A., and Nitsche, M. A. (2021). The role of dorsolateral and ventromedial prefrontal cortex in the processing of emotional dimensions. Sci. Rep. 11:1971. doi: 10.1038/s41598-021-81454-7
New, A. S., Gelernter, J., Yovell, Y., Trestman, R. L., Nielsen, D. A., Silverman, J., et al. (1998). Tryptophan hydroxylase genotype is associated with impulsive-aggression measures: a preliminary study. Am. J. Med. Genet. 81, 13–17. doi: 10.1002/(SICI)1096-8628(19980207)81:1<13::AID-AJMG3>3.0.CO;2-O
Nisoli, E., Brunani, A., Borgomainerio, E., Tonello, C., Dioni, L., Briscini, L., et al. (2007). D2 dopamine receptor (DRD2) gene Taq1A polymorphism and the eatingrelated psychological traits in eating disorders (anorexia nervosa and bulimia) and obesity. Eat. Weight Disord. 12, 91–96. doi: 10.1007/BF03327583
Oldershaw, A., Hambrook, D., Stahl, D., Tchanturia, K., Treasure, J., and Schmidt, U. (2011). The socio-emotional processing stream in anorexia nervosa. Neurosci. Biobehav. Rev. 35, 970–988. doi: 10.1016/j.neubiorev.2010.11.001
Paolacci, S., Kiani, A. K., Manara, E., Beccari, T., Ceccarini, M. R., Stuppia, L., et al. (2020). Genetic contributions to the etiology of anorexia nervosa: New perspectives in molecular diagnosis and treatment. Mol. Genet. Genomic Med. 8:e1244. doi: 10.1002/mgg3.1244
Parker, K. J., Garner, J. P., Libove, R. A., Hyde, S. A., Hornbeak, K. B., Carson, D. S., et al. (2014). Plasma oxytocin concentrations and OXTR polymorphisms predict social impairments in children with and without autism spectrum disorder. Proc. Natl. Acad. Sci. USA 111, 12258–12263. doi: 10.1073/pnas.1402236111
Perello, M., and Dickson, S. (2015). Ghrelin signalling on food reward: a salient link between the gut and the mesolimbic system. J. Neuroendocrinol. 27, 424–434. doi: 10.1111/jne.12236
Pleplé, A., Lalanne, C., Huas, C., Mattar, L., Hanachi, M., Flament, M. F., et al. (2021). Nutritional status and anxious and depressive symptoms in anorexia nervosa: a prospective study. Sci. Rep. 11:771. doi: 10.1038/s41598-020-79410-y
Pugsley, C. A., Forbes, I. J., and Morley, A. A. (1978). Immunologic abnormalities in an animal model of chronic hypoplastic marrow failure induced by busulfan. Blood 51, 601–610. doi: 10.1182/blood.V51.4.601.601
Qayyum, A., Zai, C., Hirata, Y., Tiwari, A., Cheema, S., Nowrouzi, B., et al. (2015). The role of the catechol-o-methyltransferase (COMT) GeneVal158Met in aggressive behavior, a review of genetic studies. Curr. Neuropharmacol. 13, 802–814. doi: 10.2174/1570159X13666150612225836
Raevuori, A., Keski-Rahkonen, A., and Hoek, H. W. (2014). A review of eating disorders in males. Curr. Opin. Psychiatry 27, 426–430. doi: 10.1097/YCO.0000000000000113
ROBERTS, M. E., TCHANTURIA, K., STAHL, D., SOUTHGATE, L., and TREASURE, J. (2007). A systematic review and meta-analysis of set-shifting ability in eating disorders. Psychol. Med. 37, 1075–1084. doi: 10.1017/S0033291707009877
Rybakowski, F., Dmitrzak-Weglarz, M., Szczepankiewicz, A., Skibinska, M., Slopien, A., Rajewski, A., et al. (2007). Brain derived neurotrophic factor gene Val66Met and-270C/T polymorphisms and personality traits predisposing to anorexia nervosa. Neuro Endocrinol. Lett. 28, 153–158
Sala, M., Han, K., Acevedo, S., Krawczyk, D. C., and McAdams, C. J. (2018). Oxytocin receptor polymorphism decreases midline neural activations to social stimuli in anorexia nervosa. Front. Psychol. 9:2183. doi: 10.3389/fpsyg.2018.02183
Schaefer, L. M., Crosby, R. D., and Machado, P. P. (2021). A systematic review of instruments for the assessment of eating disorders among adults. Curr. Opin. Psychiatry 34, 543–562. doi: 10.1097/YCO.0000000000000746
Schulte-Rüther, M., Mainz, V., Fink, G. R., Herpertz-Dahlmann, B., and Konrad, K. (2012). Theory of mind and the brain in anorexia nervosa: relation to treatment outcome. J. Am. Acad. Child Adolesc. Psychiatry 51, 832–841.e11. doi: 10.1016/j.jaac.2012.06.007
Schwartz, B. I., Mansbach, J. M., Marion, J. G., Katzman, D. K., and Forman, S. F. (2008). Variations in admission practices for adolescents with anorexia nervosa: a north American sample. J. Adolesc. Health 43, 425–431. doi: 10.1016/j.jadohealth.2008.04.010
Scott-van Zeeland, A. A., Bloss, C. S., Tewhey, R., Bansal, V., Torkamani, A., Libiger, O., et al. (2014). Evidence for the role of EPHX2 gene variants in anorexia nervosa. Mol. Psychiatry 19, 724–732. doi: 10.1038/mp.2013.91
Seitz, J., Herpertz-Dahlmann, B., and Konrad, K. (2016). Brain morphological changes in adolescent and adult patients with anorexia nervosa. J. Neural Transm. (Vienna) 123, 949–959. doi: 10.1007/s00702-016-1567-9
Serpell, L., Livingstone, A., Neiderman, M., and Lask, B. (2002). Anorexia nervosa: obsessive-compulsive disorder, obsessive-compulsive personality disorder, or neither? Clin. Psychol. Rev. 22, 647–669. doi: 10.1016/S0272-7358(01)00112-X
Shen, T., You, Y., Joseph, C., Mirzaei, M., Klistorner, A., Graham, S. L., et al. (2018). BDNF polymorphism: a review of its diagnostic and clinical relevance in neurodegenerative disorders. Aging Dis. 9, 523–536. doi: 10.14336/AD.2017.0717
Shimamura, A. P. (2000). The role of the prefrontal cortex in dynamic filtering. Psychobiology 28, 207–218. doi: 10.3758/BF03331979
Smink, F. R., Van Hoeken, D., and Hoek, H. W. (2012). Epidemiology of eating disorders: incidence, prevalence and mortality rates. Curr. Psychiatry Rep. 14, 406–414. doi: 10.1007/s11920-012-0282-y
Smith, M. H., Richards, P. S., and Maglio, C. J. (2004). Examining the relationship between religious orientation and eating disturbances. Eat. Behav. 5, 171–180. doi: 10.1016/S1471-0153(03)00064-3
Sokoloff, P., Giros, B., Martres, M. P., Bouthenet, M. L., and Schwartz, J. C. (1990). Molecular cloning and characterization of a novel dopamine receptor (D3) as a target for neuroleptics. Nature 347, 146–151. doi: 10.1038/347146a0
Solmi, M., Gallicchio, D., Collantoni, E., Correll, C. U., Clementi, M., Pinato, C., et al. (2016). Serotonin transporter gene polymorphism in eating disorders: data from a new biobank and META-analysis of previous studies. World J. Biol. Psychiatry 17, 244–257. doi: 10.3109/15622975.2015.1126675
Stanford, S. C., and Lemberg, R. (2012). A clinical comparison of men and women on the eating disorder inventory-3 (EDI-3) and the eating disorder assessment for men (EDAM). Eat. Disord. 20, 379–394. doi: 10.1080/10640266.2012.715516
Stang, A. (2010). Critical evaluation of the Newcastle-Ottawa scale for the assessment of the quality of nonrandomized studies in meta-analyses. Eur. J. Epidemiol. 25, 603–605. doi: 10.1007/s10654-010-9491-z
Steiger, H. (2004). Eating disorders and the serotonin connection: state, trait and developmental effects. J. Psychiatry Neurosci. 29, 20–29,
Strober, M., Freeman, R., Lampert, C., Diamond, J., and Kaye, W. (2000). Controlled family study of anorexia nervosa and bulimia nervosa: evidence of shared liability and transmission of partial syndromes. Am. J. Psychiatry 157, 393–401. doi: 10.1176/appi.ajp.157.3.393
Szczypka, M. S., Rainey, M. A., Kim, D. S., Alaynick, W. A., Marck, B. T., Matsumoto, A. M., et al. (1999). Feeding behavior in dopamine-deficient mice. Proc. Natl. Acad. Sci. USA 96, 12138–12143. doi: 10.1073/pnas.96.21.12138
Tchanturia, K., Harrison, A., Davies, H., Roberts, M., Oldershaw, A., Nakazato, M., et al. (2011). Cognitive flexibility and clinical severity in eating disorders. PLoS One 6:e20462. doi: 10.1371/journal.pone.0020462
Testa, G., Granero, R., Misiolek, A., Vintró-Alcaraz, C., Mallorqui-Bagué, N., Lozano-Madrid, M., et al. (2022). Impact of impulsivity and therapy response in eating disorders from a neurophysiological, personality and cognitive perspective. Nutrients 14:5011. doi: 10.3390/nu14235011
Thompson, J., Thomas, N., Singleton, A., Piggot, M., Lloyd, S., Perry, E. K., et al. (1997). D2 dopamine receptor gene (DRD2) Taq1 a polymorphism: reduced dopamine D2 receptor binding in the human striatum associated with the A1 allele. Pharmacogenetics 7, 479–484. doi: 10.1097/00008571-199712000-00006
Treasure, J., Claudino, A. M., and Zucker, N. (2010). Eating disorders. Lancet 375, 583–593. doi: 10.1016/S0140-6736(09)61748-7
van den Eynde, F., Suda, M., Broadbent, H., Guillaume, S., van den Eynde, M., Steiger, H., et al. (2012). Structural magnetic resonance imaging in eating disorders: a systematic review of voxel-based morphometry studies. Eur. Eat. Disord. Rev. 20, 94–105. doi: 10.1002/erv.1163
van Galen, K. A., Ter Horst, K. W., and Serlie, M. J. (2021). Serotonin, food intake, and obesity. Obes. Rev. 22:e13210. doi: 10.1111/obr.13210
Wade, T., and Pellizzer, M.. (2024). 27 assessment of eating disorders. Available at: https://www.cambridge.org/core/books/abs/cambridge-handbook-of-clinical-assessment-and-diagnosis/assessment-of-eating-disorders/D2106C344BC89CDE8090C965CE52ECE1
Wagner, A., Ruf, M., Braus, D. F., and Schmidt, M. H. (2003). Neuronal activity changes and body image distortion in anorexia nervosa. Neuroreport 14, 2193–2197. doi: 10.1097/00001756-200312020-00012
Walther, D. J., Peter, J. U., Bashammakh, S., Hörtnagl, H., Voits, M., Fink, H., et al. (2003). Synthesis of serotonin by a second tryptophan hydroxylase isoform. Science 299:76. doi: 10.1126/science.1078197
Wang, Y., Li, O., Li, N., Sha, Z., Zhao, Z., and Xu, J. (2023). Association between the BDNF Val66Met polymorphism and major depressive disorder: a systematic review and meta-analysis. Front. Psych. 14:1143833. doi: 10.3389/fpsyt.2023.1143833
Weiss, M. J., Cole, D. E., Ray, K., Whyte, M. P., Lafferty, M. A., Mulivor, R. A., et al. (1988). A missense mutation in the human liver/bone/kidney alkaline phosphatase gene causing a lethal form of hypophosphatasia. Proc. Natl. Acad. Sci. USA 85, 7666–7669. doi: 10.1073/pnas.85.20.7666
Wierenga, C. E., Reilly, E., Bischoff-Grethe, A., Kaye, W. H., and Brown, G. G. (2022). Altered reinforcement learning from reward and punishment in anorexia nervosa: evidence from computational modeling. J. Int. Neuropsychol. Soc. 28, 1003–1015. doi: 10.1017/S1355617721001326
Willer, C. J., Speliotes, E. K., Loos, R. J., Li, S., Lindgren, C. M., Heid, I. M., et al. (2009). Six new loci associated with body mass index highlight a neuronal influence on body weight regulation. Nat. Genet. 41, 25–34. doi: 10.1038/ng.287
Williams, M. J., Goergen, P., Rajendran, J., Zheleznyakova, G., Hägglund, M. G., Perland, E., et al. (2014). Obesity-linked homologues TfAP-2 and Twz establish meal frequency in Drosophila melanogaster. PLoS Genet. 10:e1004499. doi: 10.1371/journal.pgen.1004499
Wise, R. A. (1978). Catecholamine theories of reward: a critical review. Brain Res. 152, 215–247. doi: 10.1016/0006-8993(78)90253-6
Wonderlich, S., Mitchell, J., Boath, L., Steiger, H., Crow, S., Kaye, W. H., et al. (2018). Review of brain imaging in anorexia and bulimia nervosa. Annu. Rev. Eat. Disord., 113–130. doi: 10.4324/9781315384924-7
Xu, J., Harper, J. A., van Enkevort, E. A., Latimer, K., Kelley, U., and McAdams, C. J. (2017). Neural activations are related to body-shape, anxiety, and outcomes in adolescent anorexia nervosa. J. Psychiatr. Res. 87, 1–7. doi: 10.1016/j.jpsychires.2016.12.005
Yurtsever, I., Matusiak, Ł., Szepietowska, M., Evans, C., and Szepietowski, J. C. (2022). Body shape Questionnaire-34 (BSQ) and functionality appreciation scale (FAS) - pertinent body image screening tools: creation and validation of polish language versions. Sci. Prog. 105:368504221117068. doi: 10.1177/00368504221117068
Zhu, Y., Hu, X., Wang, J., Chen, J., Guo, Q., Li, C., et al. (2012). Processing of food, body and emotional stimuli in anorexia nervosa: a systematic review and meta-analysis of functional magnetic resonance imaging studies. Eur. Eat. Disord. Rev. 20, 439–450. doi: 10.1002/erv.2197
Zipfel, S., Wild, B., Groß, G., Friederich, H. C., Teufel, M., Schellberg, D., et al. (2014). Focal psychodynamic therapy, cognitive behaviour therapy, and optimised treatment as usual in outpatients with anorexia nervosa (ANTOP study): randomised controlled trial. Lancet 383, 127–137. doi: 10.1016/S0140-6736(13)61746-8
Keywords: anorexia nervosa, eating disorder, genetic polymorphism, genetic susceptibility, neurobiological factors, psychological factors
Citation: Almaghrbi H and Bawadi H (2024) Genetic polymorphisms and their association with neurobiological and psychological factors in anorexia nervosa: a systematic review. Front. Psychol. 15:1386233. doi: 10.3389/fpsyg.2024.1386233
Edited by:
Marianna Rania, University Hospital Renato Dulbecco, ItalyReviewed by:
Enrico Collantoni, University of Padua, ItalyElvira Anna Carbone, University Magna Graecia of Catanzaro, Italy
Livio Tarchi, University of Florence, Italy
Copyright © 2024 Almaghrbi and Bawadi. This is an open-access article distributed under the terms of the Creative Commons Attribution License (CC BY). The use, distribution or reproduction in other forums is permitted, provided the original author(s) and the copyright owner(s) are credited and that the original publication in this journal is cited, in accordance with accepted academic practice. No use, distribution or reproduction is permitted which does not comply with these terms.
*Correspondence: Hiba Bawadi, aGJhd2FkaUBxdS5lZHUucWE=