- ToNIC, Toulouse NeuroImaging Center, Université de Toulouse, Inserm, UPS, Toulouse, France
Intentionally or not, humans produce rhythmic behaviors (e.g., walking, speaking, and clapping). In 1974, Paul Fraisse defined rhythmic behavior as a periodic movement that obeys a temporal program specific to the subject and that depends less on the conditions of the action (p. 47). Among spontaneous rhythms, the spontaneous motor tempo (SMT) corresponds to the tempo at which someone produces movements in the absence of external stimuli, at the most regular, natural, and pleasant rhythm for him/her. However, intra- and inter-individual differences exist in the SMT values. Even if several factors have been suggested to influence the SMT (e.g., the age of participants), we do not yet know which factors actually modulate the value of the SMT. In this context, the objectives of the present systematic review are (1) to characterize the range of SMT values found in the literature in healthy human adults and (2) to identify all the factors modulating the SMT values in humans. Our results highlight that (1) the reference value of SMT is far from being a common value of 600 ms in healthy human adults, but a range of SMT values exists, and (2) many factors modulate the SMT values. We discuss our results in terms of intrinsic factors (in relation to personal characteristics) and extrinsic factors (in relation to environmental characteristics). Recommendations are proposed to assess the SMT in future research and in rehabilitative, educative, and sport interventions involving rhythmic behaviors.
1. Introduction
Rhythm is an essential human component. “Rhythm is defined as the pattern of time intervals in a stimulus sequence” (Grahn, 2012, p. 586), and the tempo is the rate of the stimuli's onset within a regular sequence (Grahn, 2012). Early in life, rhythm is present in a large number of activities of daily life, such as walking, speaking, chewing, doing leisure activities (dancing, swimming, pedaling, playing a musical instrument, singing, clapping, etc.), or school activities (writing and reading). Some activities require producing a rhythm with a spontaneous tempo (e.g., writing, reading, chewing, walking, speaking, etc.), and some others require synchronizing with a rhythm produced by an external event (e.g., playing a musical instrument, singing, clapping, dancing, etc.). Those activities can have different rhythmic components. For example, speech generally shows a non-isochronous rhythmic structure, but other language skills, such as reading, may also show beat-based patterns (i.e., isochronous patterns based on equal time intervals; see Ozernov-Palchik and Patel, 2018). Writing seems to be linked to isochronous rhythmic production (Lê et al., 2020b), even if it is not yet well-known whether writing shows more beat- or non-beat-based processing. Other activities, such as tapping or clapping, are well-known to show isochronous patterns.
Rhythmic abilities are deficient in various populations, and nowadays, rehabilitative interventions based on rhythmic synchronization are used to improve motor control. This is the case for populations with neurological diseases (e.g., Parkinson's disease, stroke, and cerebral palsy; see Braun Janzen et al., 2021), rare diseases or conditions (Launay et al., 2014; Bégel et al., 2017, 2022a; Tranchant and Peretz, 2020), or neurodevelopmental disorders (e.g., dyslexia, developmental coordination disorder, and attention deficit and hyperactivity disorder; Puyjarinet et al., 2017; Bégel et al., 2018, 2022b; Lê et al., 2020a; Blais et al., 2021; Daigmorte et al., 2022). In this context, participants are required to synchronize their movements to an external rhythm, usually with an auditory metronome, to regulate the speed of their gait or manual or verbal responses. The ability to synchronize with an external rhythm is particularly studied during sensorimotor synchronization tasks that consist of the “coordination of a rhythmic movement with an external rhythm” (Repp and Su, 2013, p. 1). The tempo and the sensory modality of the external rhythmic stimuli can modulate the performance of sensorimotor synchronization (see Repp, 2005; Repp and Su, 2013 for extensive reviews of the literature). Sensorimotor synchronization is less accurate and stable when the tempo is slower (Drewing et al., 2006; Repp and Su, 2013) and slower than the spontaneous motor tempo (SMT; Varlet et al., 2012). SMT is the rhythm at which a person produces movements in the absence of stimuli at his/her own most regular, natural, and pleasant rate. Hence, the tempo of the external rhythm has to be adapted to the actual tempo of the participants. Recent studies individualize the parameters of the intervention by adapting the tempo of the metronome to be synchronized (Benoit et al., 2014; Dalla Bella et al., 2017; Cochen De Cock et al., 2021; Frey et al., 2022). This is done by measuring the individual's SMT before an intervention. Rehabilitation is then performed with music at either ±10% of this tempo. Therefore, it seems interesting to evaluate rhythmic abilities, especially spontaneous motor tempo (SMT), to individualize learning and rehabilitation.
It is usually admitted in the pioneering work of Paul Fraisse that the most representative reference value of the spontaneous motor tempo (SMT) is 600 ms in healthy human adults (Fraisse, 1974). However, a growing body of literature about SMT suggests that this value is not universal. Fraisse himself pointed out that, even if the SMT is supposed to be relatively stable in one individual, inter-individual differences are more important and could be related to the instructions, the material of measurement, the body position, the chronological and intellectual development, and the sensory deficits (Fraisse, 1974). Even if these factors have been tested in a few studies, to our knowledge, no updated review of the literature has been made to provide complete and recent knowledge on the range of SMT values in healthy human adults and the factors influencing them. For example, recent studies suggest that age is a major factor modulating the value of SMT. The review by Provasi et al. (2014a) focuses on the spontaneous (and induced) rhythmic behaviors during the perinatal period, with a special emphasis on the spontaneous rhythm of sucking, crying, and arm movements in newborns. The authors indicate that the SMT evolves from newborns to the elderly. Fast rhythmical movements of the arms have been identified in fetuses with a tempo of 3 or 4 movements per second (250–333 ms; Kuno et al., 2001), whereas a tempo of 450 ms has been found during drumming (Drake et al., 2000) or tapping (McAuley et al., 2006) in children around 4 years old and more. The value of the SMT is relatively fixed around 400 ms between 5 and 8 years, even if the variability of the SMT tends to decrease with age (Monier and Droit-Volet, 2019). The SMT is supposed to increase to achieve 600 ms in adulthood (Fraisse, 1974) and to slow down further with age to achieve 700–800 ms in the elderly (Vanneste et al., 2001). In the case of tempo produced with the mouth, the SMT of non-nutritive sucking is around 450 ms in neonates (Bobin-Bègue et al., 2006), whereas the spontaneous crying frequency is between 1,100 and 2,400 ms in newborns (Brennan and Kirkland, 1982). All these results suggest that the relationship between SMT and age is not general and linear. The effector producing the SMT could be a potential factor affecting the relationship between SMT and age.
Some studies focus on the SMT produced with the mouth in a quasi-rhythmic pattern during speech production and in an isochronous repetitive pattern during syllable rate production. The review of Poeppel and Assaneo (2020) reports that the temporal structure of speech “is remarkably stable across languages, with a preferred range of rhythmicity of 2–8 Hz” (125–500 ms; Poeppel and Assaneo, 2020, p. 322). One could suggest that this rhythm is faster than the rhythm supposed to be found in rhythmical movements of the arms (600 ms in adulthood, Fraisse, 1974). However, in the broader context of speech production, we cannot neglect the communicative aspect of speech. The audience for the speech could also influence the SMT (Leong et al., 2017). Thus, it is possible that, in addition to the age previously mentioned, not only the effector but also the communicative goal of the activity may influence the SMT.
Moreover, environmental factors are supposed to influence SMT values. In the review of Van Wassenhove (2022), it is suggested that the manipulation of external landmarks, such as the time of day, can modulate the endogenous temporal representation of time and, as a consequence, the SMT (Van Wassenhove, 2022).
In this context, the objectives of the systematic review are (1) to characterize the range of SMT values found in the literature in healthy human adults and (2) to identify all the factors modulating the SMT values in humans.
2. Materials and methods
We conducted a systematic review according to PRISMA recommendations (Preferred Reporting Items for Systematic Reviews and Meta-Analyses; Page et al., 2021).
2.1. Information sources and search strategy
Studies were identified by searching in the PubMed, Science Direct, and Web of Science databases. These databases were selected because they represent a broad spectrum of disciplines related to motor behavior. The final search was performed on 4 July 2022. There was no restriction on the year of publication; all articles present in the databases at this time point were searched. The search was first conducted in all languages, and then only English and French studies were selected for screening. As the term “spontaneous motor tempo” is not exclusively used, we searched a broad spectrum of synonyms for this term. Filters were also used to identify relevant research depending on the database (Table 1).
2.2. Selection of studies and eligibility criteria
We only selected articles and reviews before screening by excluding congress papers, chapters, books, and theses. Reviews identified in databases were just used to find missing original articles about SMT, and they have not been included in the systematic review (reviews not included: Provasi et al., 2014a; Poeppel and Assaneo, 2020; Van Wassenhove, 2022).
For greater specificity in the selection of the studies, inclusion criteria were based on the PICO (population, intervention, comparator, and outcome) strategy (Table 2). For this, we selected studies carried out on human samples producing rhythmic tasks. A control factor or control group was identified as a comparator. Spontaneous motor tempo was identified as the Outcome. Moreover, we selected other exclusion criteria: (1) studies that did not present experimental data; (2) studies that did not present a SMT task (i.e., focusing only on sensorimotor synchronization or on perception of rhythmic stimuli); (3) studies that did not report data on SMT (a SMT task is produced by the participants, but variables studied assess, for example, brain data or relative phases); (4) studies that did not focus on intentional SMT (studies on cardiorespiratory rhythms like breath or heart rate); and (5) studies that focus on walking with displacement (locomotion). We excluded studies on locomotion because locomotion involves spatiotemporal regulation; however, we retained studies on walking on a treadmill because walking on a treadmill involves mainly temporal regulation.
All titles and abstracts were screened by one researcher (AD), and if the articles fit the review criteria, they were read in full. The full-text eligibility assessment was conducted by two independent reviewers (AD and JT). Disagreements were resolved by a discussion according to the PICO strategy with a third researcher (EM).
2.3. Data collection process
For tabulation and extraction of data referring to the selected studies, Excel® software spreadsheets were used. After screening the selected studies, we classified them into two categories, i.e., those measuring the SMT values (in general, as a prerequisite for a subsequent rhythmic sensorimotor synchronization task) and those examining the effect of factor(s) on the SMT values.
For studies measuring the SMT values, we extracted study characteristics, demographic variables, methodological variables, and outcome indicators from each study. The extracted characteristics included the authors, the year of publication, and the sample size. Demographic variables included sex, age, and laterality. Methodological variables included the instruction, the task, the effector(s), and the measurement recording. Outcome indicators included SMT values and their units. We finally convert all of the SMT values to milliseconds to be comparable and to provide a range of SMT values.
For studies about factor(s) modulating SMT values, we extracted study characteristics (first author and year of publication), methodological variables (task and effector(s)), and outcome indicators (factor(s) effects, their significance, and their direction on SMT values, i.e., on the mean or median and/or the standard deviation or coefficient of variation). Sometimes, we also extracted other information (e.g., subgroups and specific statistical analyses) to understand and interpret the results.
3. Results
A total of 3,179 studies were identified via databases. Before screening, 357 duplicates and 159 studies were removed (e.g., language, chapters and books, congress papers, or theses). According to the exclusion criteria, 2,349 studies were excluded based on the title or the abstract. After verifying the records left in full, according to the pre-established eligibility criteria, 93 studies from databases were included in the systematic review. Moreover, 14 out of 25 studies identified via citation searching were included. Finally, a total of 107 studies were included in the systematic review. Results from the process for selecting the included articles (following the recommendations of Page et al., 2021) are described in the flowchart (Figure 1).
In total, 13 studies provide a SMT value or a range of SMT values in healthy adults (Table 3). Our results reveal that the range of SMT values is from 333 to 3,160 ms. Notably, 94 studies measure the effect of the factor(s) on the SMT values (Table 4). We classified studies according to the type of factors modulating the SMT values: intrinsic factors, in relation to personal characteristics, and extrinsic factors, in relation to environmental characteristics. Concerning intrinsic factors, we have found studies investigating the effects of a pathology (N = 27), age (N = 16), the effector or the side (N = 7), the expertise or a predisposition (N = 7), and the genotype (N = 2). Concerning extrinsic factors, we have found studies investigating the effects of physical training (N = 10), external constraints (N = 7), observation training (N = 5), the time of testing (N = 4), the internal state (N = 3), the type of task (N = 5), and a dual task (N = 2).

Table 4. Summarized results of studies investigating the effects of factors on the SMT values (N = 94).
The number of studies exploring the SMT across years is presented in Figure 2.
4. Discussion
The present systematic review aimed to (1) characterize the range of SMT values found in the literature in healthy human adults and (2) identify all the factors modulating the SMT values in humans.
First, it is interesting to note that the global number of studies has grown since the early 1970's (Figure 2). The increase in studies about SMT actually started in the mid-1990's and has grown non-linearly to reach a peak in 2020. Thus, interest in SMT is old but has recently increased.
Second, our results highlight that (1) the reference value of SMT is far from being a common value of 600 ms in healthy human adults, but a range of SMT values exists and (2) many factors modulate the SMT values. We discuss these factors according to a classification as intrinsic factors, in relation to personal characteristics, and extrinsic factors, in relation to environmental characteristics. We also provide recommendations to measure, report, and use the SMT values for future studies on rhythmic production and perception.
4.1. Range of SMT values in healthy human adults
Regarding the range of SMT values, we have selected the studies that propose an SMT task as a baseline, followed by a second task that is usually a sensorimotor synchronization task, without comparison between factors or conditions (Table 3). However, no value of SMT is reported in some studies (N = 2/13). Hence, it is important to measure the SMT as a baseline before any rhythmic task and to report the SMT values in order to interpret the results with regard to this baseline.
The number of studies measuring the SMT as a baseline for a rhythmic task (to adjust the tempo of the rhythmic task) is rather low (Table 3), compared to those testing the effects of variables on the SMT values (Table 4). This may be due to the fact that the terminology used to designate the spontaneous motor tempo is heterogeneous. Although the SMT was clearly defined by Fraisse (1974) as the speed that the subject considers most natural and pleasant (p. 50), this terminology is not unanimous. Although some authors use the term “spontaneous motor tempo” (Drake et al., 2000; McPherson et al., 2018; Amrani and Golumbic, 2020), others use different terms, such as “preferred motor tempo” (Michaelis et al., 2014), “preferred rate” (McCombe Waller and Whitall, 2004), “preferred frequency” (Volman et al., 2006; Bouvet et al., 2020), “internal clock” (Yahalom et al., 2004), “spontaneous production rate” (Wright and Palmer, 2020), “motor spontaneous tempo” (Dosseville and LaRue, 2002; Moussay et al., 2002), “spontaneous movement tempo” (Avanzino et al., 2015; Bisio et al., 2015), “freely chosen cadence” (Sidhu and Lauber, 2020; Hansen et al., 2021), or “personal tempo” (Tajima and Choshi, 1999). In the same vein, the term “self-paced” is not used with a consensual definition. Sometimes, this term relates to an intentional spontaneous motor behavior without a rhythmic component, even if authors use the term “self-paced tapping” (e.g., Bichsel et al., 2018, not included in the present review), and sometimes it relates to an intentional spontaneous rhythmic motor behavior when “self-paced” is followed by “tempo” (Serrien, 2009; Hattori et al., 2015). For future studies measuring the SMT, we recommend using the terminology “spontaneous motor tempo” when the participant is invited to produce a rhythmic motor task not induced by external stimuli specifying a required tempo. The term “spontaneous motor tempo” should be preferred to the term “self-paced” to define the task. To increase the visibility of studies implying SMT, the term “spontaneous motor tempo” and its acronym “SMT” should appear in the title or keywords of the articles.
The tasks used to measure the SMT are also very heterogeneous. Even if Fraisse (1974) declared that SMT is commonly measured during a manual task (Fraisse, 1974), our results reveal that studies exploring SMT also measure other effectors apart from manual ones. Some studies use self-paced tapping with one or two effectors; others use drumming, hopping, pointing, cycling, swaying, and producing syllables; and another uses jaw opening-closing and chewing (Table 3). Regarding the SMT values, participants seem to be slower when the whole body or the jaw is required, compared to manual responses. Thus, the heterogeneity of effectors (finger, arm, leg, whole body, mouth/lips, and jaw) used to produce the SMT could explain the heterogeneity of results. This hypothesis could be in accordance with the results of Sakamoto et al. (2007), highlighting that the SMT is effector-dependent (Sakamoto et al., 2007), but we recommend to carry out further studies to test the impact of effectors on SMT.
The range of SMT values (from 333 to 3,160 ms) is far from being a common value of 600 ms, as first reported by Fraisse (1974). More specifically, it is important to note that studies reporting the slowest SMT values involve cyclical movements compared to the discrete isochronous movements of tapping or clapping. Regarding finger tapping, SMT appears to be faster (from 333 to 931 ms). Bouvet et al. (2020), who investigate the effect of accents and subdivisions in synchronization, performed a measurement of SMT during finger-tapping with a large number of taps in several trials. They also find a faster value around 650 ms. The heterogeneity of results can be explained by the heterogeneity in the paradigm applied to measure the SMT in the studies. We provide such examples in the following paragraphs.
First, the characteristics of participants are not homogeneously reported, particularly their level of musical experience. In some studies listed in Table 3, authors report that participants have no musical training. Note that some studies mix musicians and non-musicians in their samples (e.g., Michaelis et al., 2014; De Pretto et al., 2018). However, three studies reported in Table 4 show an effect of music expertise (Drake et al., 2000; Slater et al., 2018; Hammerschmidt et al., 2021). Information about musical expertise is particularly important, including the expertise of listening to music, given that it is possible that participants could present amusia or a deficit in rhythm production or perception (Stewart et al., 2006; Clark et al., 2015; Peretz, 2016; Sarasso et al., 2022). To have a better overview of the range of SMT values in healthy adults without musical expertise, we recommend reporting a general level of musical experience, that is, both the level of expertise in music/rhythm production and music/rhythm exposure.
Second, the characteristics of participants are also heterogeneous across studies in terms of age, sex, and laterality. Regarding the age, participants are from 18 to 45 years old (Table 1). Despite the fact that the age range is representative of healthy young adults, the range of SMT values varies in five studies about manual responses from 333 to 1,100 ms (Michaelis et al., 2014; De Pretto et al., 2018; McPherson et al., 2018; Zhao et al., 2020). Regarding the sex repartition, only two studies recruit an equal number of women and men (Michaelis et al., 2014; De Pretto et al., 2018); the others recruit either more women or more men. As reported in Table 4, the effect of sex on SMT has not been extensively studied, given that only one study addresses this question and reports no significant results (Suzuki and Ando, 2018). Regarding the laterality, the majority of studies do not report the laterality of participants (Table 3, N = 8/13). The other studies generally recruit right-handed participants (Table 3, N = 3/5). Some studies include one or two left-handed participants (Table 3, N = 2/5). In Table 4, no study investigates the effect of laterality on the SMT values. In the absence of clear results about laterality, we recommend specifying the laterality of the participants by means of a laterality questionnaire (e.g., Oldfield, 1971) in the case of a SMT task performed with a lateralized effector (hand or leg). More globally, to have a better overview of the range of SMT values in healthy adults, we recommend reporting the age, sex, and laterality of participants and specifying, if possible, whether the SMT differs according to these variables.
Third, how the SMT is measured is not consistent across studies (Table 3). As specified in Table 3, SMT paradigms differ according to the number of trials and their duration, as well as to the instructions provided to the participants. The number and duration of trials vary across studies. Globally, the number of trials is from 1 to 10, and the duration of each trial can be expressed as a range of time (seconds or minutes), a number of responses, or a number of inter-response intervals (Table 3). Two studies do not report any information about trials (Ruspantini et al., 2012; Malcolm et al., 2018). Regarding the instructions, it is important to note that the instructions are not reported in three out of 13 studies (Eriksson et al., 2000; Hattori et al., 2015; Malcolm et al., 2018). When reported, the instructions contain the terms “natural,” “comfortable,” “most comfortable,” “naturally comfortable,” “preferred,” “steady,” “freely chosen,” “own self-selected,” “spontaneously,” “without mental effort,” “do not require much awareness,” “without fatigue,” and “could be performed all day if necessary,” to characterize the manner to produce the SMT (Table 3). Moreover, the tempo itself is characterized as “tempo,” “pace,” “cadence,” “speed,” “rate,” and “frequency.” Even if these terms are supposed to represent the same instruction, we would like to emphasize that the semantics is not a detail. The instruction can modify the participant's behavior depending on the interpretation he/she makes of it. For example, the term “speed” can be interpreted by participants as an instruction to go fast. Thus, to have a better overview of the range of SMT values in healthy adults, we recommend reporting exactly and exhaustively the standardized instructions given to participants. More precisely, we recommend giving priority to the notions of “preferred,” “spontaneous,” and “comfortable tempo,” in the instructions given to the participant. It seems important to avoid the notion of “speed” in order not to induce the idea of performing the task as quickly as possible.
Fourth, how SMT is recorded and computed is not consistent. Regarding the measurement recordings, authors report the inter-response interval, frequency, number of movement cycles during the total duration of the trial, rate, cycle time, speed, or cadence. If reported, the values also have different units (milliseconds, seconds, beats per minute, Hertz, repetitions per minute, or kilometers per hour). Furthermore, the authors usually report the range of SMT values, the SMT mean and/or median, its standard deviation, and/or the coefficient of variation (Table 3). These discrepancies are probably due to the type of task used. Only two studies recording SMT do not report any value for SMT (LaGasse, 2013; Zhao et al., 2017). On this basis, we recommend reporting the SMT values when recorded and homogenizing the measurement recording, the variables, and their units (in milliseconds or Hz). It is, therefore, necessary to report, at least, the SMT values with the median and the range of SMT values with a box plot representing individual values to get access to the distribution of data with the minimal and maximal values. It is also important to specify the methodology to compute the SMT, in particular to report excluded data, for example, the first responses that were performed by the participants, which can be considered warm-up.
4.2. Intrinsic and extrinsic factors modulating SMT values
Table 4 summarizes the results of studies about factors that could modulate the SMT values. We classified these factors as intrinsic and extrinsic ones, i.e., factors that could explain inter- and intra-individual variability in SMT values. Figure 3 presents the repartition of studies about the factors modulating the SMT values according to the intrinsic factors (N = 59) and the extrinsic factors (N = 36).
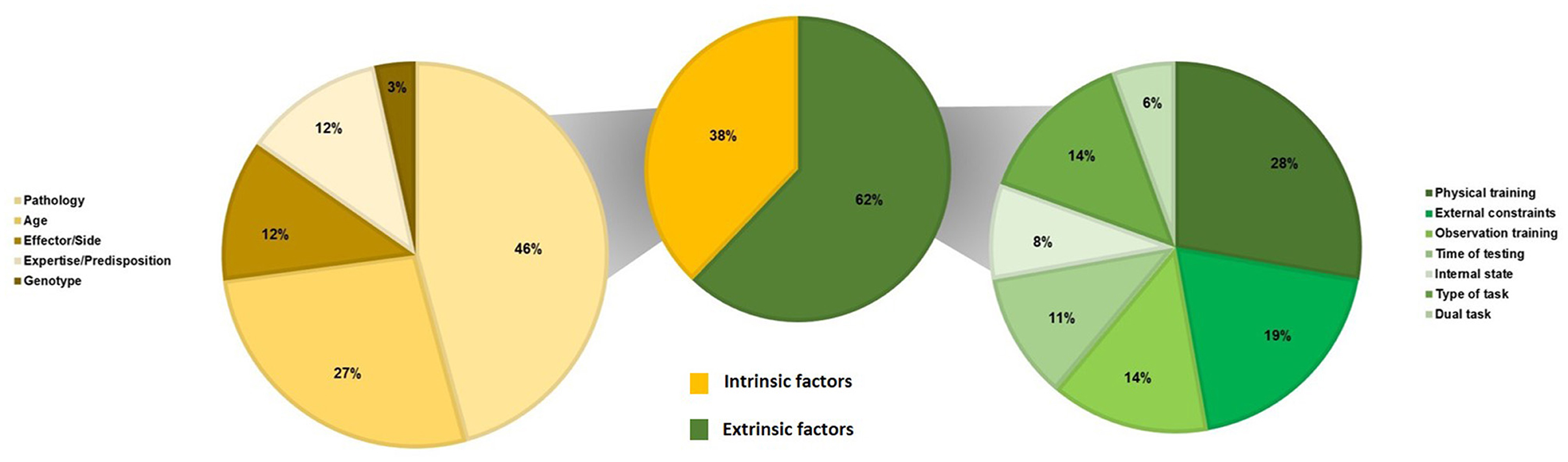
Figure 3. Repartition of studies on the factors modulating the SMT values (N = 94) according to intrinsic factors (N = 59) and extrinsic factors (N = 36).
Regarding the intrinsic factors, our results reveal that the SMT is affected by several factors such as pathology, age, effector, expertise, or genotype (see Table 4). First, our results reveal that several pathologies modify the SMT values. Studies investigate brain lesions (six on Parkinson's, four on stroke, one on Huntington disease, one on Alzheimer's disease, one on Whiplash, and two on cerebellar lesions), neurodevelopmental disorders (two on attention deficit and hyperactivity disorder, two on developmental coordination disorder, one on developmental intellectual deficit, one on stuttering, and one on minor neurological dysfunction), and mental disorders (two on schizophrenia). Two studies test the effects of a deficit in music perception (beat deafness, i.e., difficulties in tracking or moving to a beat), and only one study examines the effect of an amputation. Globally, our results show that the most studied pathologies are brain lesions. Results indicate quasi-unanimously that SMT is affected by brain lesions (Table 4, N = 12/15). Studies report that either the frequency or the stability of the SMT differs in brain-injured patients compared to controls. In brain lesions, neurodegenerative disorders are the most studied, such as Parkinson's and Huntington's diseases (both implying a lesion of the basal ganglia) or Alzheimer's disease. Studies on Parkinson's disease report quasi-consistently that SMT is significantly affected in patients compared to healthy elderly individuals (Table 4, N = 5/6), and the study on Huntington's disease reports the same effect (Martínez Pueyo et al., 2016). The only study on Alzheimer's disease does not report any difference between patients and healthy elderly individuals (Martin et al., 2017). Moreover, most of the studies report that SMT is significantly affected in patients with stroke compared to healthy adults (Table 4, N = 3/4). In contrast, results are less consistent for neurodevelopmental and mental disorders. Attention deficit and hyperactivity disorder seems to affect the SMT (Table 4, N = 2/2), as does developmental coordination disorder (Table 4, N = 2/2). Only two studies report the effects of beat deafness with no consistent results (Phillips-Silver et al., 2011; Palmer et al., 2014). Based on these results, it is interesting to note that the SMT is affected regardless of the location of the lesion (motor cortex, language areas, basal ganglia, or cerebellum) and regardless of the physiopathology (neurodegenerative vs. neurological vs. neurodevelopmental). Although it seems more likely that focal lesions affect the SMT, future studies are required to better understand if and how the SMT is affected by neurodevelopmental, mental, and sensory disorders.
A second factor modulating the SMT is age. Studies investigate mostly infants (Table 4, N = 14/16). Only three studies investigate the elderly (Vanneste et al., 2001; Baudouin et al., 2004; McAuley et al., 2006). Our results reveal that age modifies the value of the SMT in the majority of studies (Table 4, N = 11/14). In fact, only three out of 14 studies do not find an effect of age in infants or children (Droit et al., 1996; Fitzpatrick et al., 1996; Yu and Myowa, 2021). It is interesting to note that only two studies test the possible effects of age on the SMT in individuals between 18 and 60 years old (McAuley et al., 2006; Hammerschmidt et al., 2021). Anyway, our results suggest that future studies about the SMT should take into account the effect of age bands or include the age of participants as a covariate, especially if participants are infants or elderly individuals.
A third intrinsic factor modulating the SMT is the effector/side used to produce the task. Results are very contradictory, with one study revealing an effect of the effector (Sakamoto et al., 2007) and two studies failing to reveal this effect (Tomyta and Seki, 2020; Rose et al., 2021). It seems that there is no effect of the side of the hand producing the SMT (Kay et al., 1987; Byblow and Goodman, 1994; Whitall et al., 1999). Moreover, it is also possible that SMT differs when it is produced with arms and legs (Sakamoto et al., 2007). Finally, the study of Getchell et al. (2001) reveals a correlation between SMT produced by different effectors. This result suggests that individuals have a general ability to produce their own SMT regardless of the type and number of effectors used (Getchell et al., 2001). Given that only one study reports this finding, further studies are required to confirm this effect.
As previously discussed above, expertise in music seems to modify the SMT. Musicians seem to have a more stable SMT than non-musicians (Scheurich et al., 2018; Slater et al., 2018; Bégel et al., 2022c). Moreover, two studies suggest that a predisposition to high or low synchronization (i.e., good or poor synchronization skills in rhythmic synchronization tasks) alters the SMT (Tranchant et al., 2016; Assaneo et al., 2021). Even if long-lasting intensive training could modify the SMT in certain conditions, it seems that intrinsic predispositions could be important. This result is in accordance with the last intrinsic factor identified in the current literature review, namely, the genotype. Two studies focus on this factor (Wiener et al., 2011; Suzuki and Ando, 2018). The first study finds a significant correlation between the tempo level in monozygotic twins but not in dizygotic twins, thereby suggesting that the genetic code could have a role in the SMT values (Suzuki and Ando, 2018). However, no difference between women and men is found, thereby preventing the possible role of sex on the SMT values (Suzuki and Ando, 2018). The second study reveals a significant effect of a polymorphism (Wiener et al., 2011). If we consider that one polymorphism (A1+) seems implied in the regulation of the density of receptors in the striatum (see Wiener et al., 2011), this result is in accordance with the results of studies showing an effect of Parkinson's disease, which affects the striatum, on the SMT (Konczak et al., 1997; Byblow et al., 2002; Flasskamp et al., 2012; Rose et al., 2020; Horin et al., 2021). Even if further studies are required to confirm this hypothesis, there is evidence that the genotype plays a role in the SMT values.
Regarding the extrinsic factors, our results highlight that the SMT is affected by several factors such as physical training, external constraints, observation training, time of testing, type of task, or dual tasking (see Table 4).
In total, 10 studies report results about the effects of physical training on the SMT. Six studies reveal a significant effect of cycling, strength training, synchronization, or physical exercise on the SMT values measured before and after training (Table 4). This result suggests that all studies about SMT should report the activity preceding the measurement of the SMT, especially physical activity.
In the same vein, all the studies (N = 5) testing the effects of the observation of a rhythmic action on the SMT found a significant effect (see Table 4). This result indicates that observing a rhythmic action without moving or synchronizing with it induces a spontaneous change in the SMT. This result is in accordance with the results of studies about the effects of physical training with rhythmic stimuli (Byblow et al., 1994; Carson et al., 1999; Hansen et al., 2021; Rocha et al., 2021). They are also in accordance with results about the effect of external constraints that show a significant effect of producing SMT while listening to a rhythmic metronome without synchronizing (Bouvet et al., 2019). The effect of observation or listening could be related to the implication of the Mirror System that is activated during observation, listening, and action (Kohler et al., 2002; Rizzolatti and Craighero, 2004). More precisely, it is possible that observing/listening a rhythm activates the same cerebral areas (i.e., the fronto-parietal system) as synchronizing to rhythmic stimuli (Konoike and Nakamura, 2020), hence modifying the SMT values according to the observed/listened tempo.
Regarding the effect of a dual task on the SMT, only one of the two studies reports a significant difference in the SMT during a single vs. dual task (Serrien, 2009). In the other study (Aubin et al., 2021), participants were instructed to swing their legs at their preferred frequency while performing a secondary task (reaction times), but no significant effect of the dual task was found. The discrepancy of results between the two studies could be explained by the fact that the secondary task is not rhythmic in Aubin et al. (2021), whereas the secondary task implies a rhythmic component in Serrien (2009). This hypothesis is in accordance with the results of studies examining the effects of rhythmic external constraints (Bouvet et al., 2019, 2020). We could deduce that the SMT is robust to a general cognitive load but can be impacted by external rhythmic stimulation. Hence, we can recommend not to perform a rhythmic task before or during the production of a task assessing SMT because it can change the SMT values.
Regarding the external constraints, most studies (N = 5/7) report consistent results about the significant effects of external constraints, such as a noisy environment, the presence of fingertip contacts, or a varying spring constraint on the SMT values (Table 4). However, the effect of loading is not consistent (Hatsopoulos and Warren, 1996; Wagener and Colebatch, 1997; Hansen and Ohnstad, 2008).
The type of task seems to quasi-consistently modulate the SMT values in four out of five studies (Table 4). Specifically, results indicate that the SMT is affected by in-phase or anti-phase bimanual tapping, polyrhythmic or single rhythmic tapping, and by tapping, drawing, playing a melody, or reciting a sentence (Tajima and Choshi, 1999; Forrester and Whitall, 2000; Zelaznik et al., 2000; Pfordresher et al., 2021).
The internal state seems to modulate the SMT values as well (Table 4). Three out of 3 studies report an effect of the internal state, such as apnea, mental stress, and gravity on the SMT values (Murata et al., 1999; Dosseville and LaRue, 2002; Boulanger et al., 2020). Once again, these results indicate that the SMT is not robust and that intra-individual variability exists. In the same vein, the time of testing seems to have an effect on the SMT values (Table 4). More precisely, studies unanimously report an effect of the time of day on the SMT values (Oléron et al., 1970; Dosseville et al., 2002; Moussay et al., 2002; Wright and Palmer, 2020). It seems that the SMT values vary in the course of the day, being slower in the morning than in the evening (Moussay et al., 2002; Wright and Palmer, 2020). As for the effect of internal state mentioned above, this effect may be related to the circadian variations of internal physiological and psychological factors, such as hormones or fatigue. Anyway, it is important to interpret this result in relation to the results of many studies that have shown an effect of trial measurement (Collyer et al., 1994; Drake et al., 2000; Scheurich et al., 2018, 2020; Bouvet et al., 2019).
5. Conclusion and perspectives
All in all, our systematic review highlights large intra- and inter-individual variability in the SMT values. According to the internal clock model (Treisman, 1963), individuals have an internal clock that is a reference generating time information, used to perceive information, and to produce and reproduce behaviors. Each individual has his/her own internal clock, leading to strong intra-individual consistency, but individual preferences exist in the production and perception of rhythms. Moreover, the internal clock can be affected by many intrinsic and extrinsic factors. We hope that the current review will lead to a better choice of reference values for SMT. We have proposed specific recommendations and points of vigilance to assess the SMT in future research.
Our results could also be transferred to applied contexts related to rehabilitative, educative, and sport interventions involving rhythmic sensorimotor synchronization. For example, dance can be viewed as a rhythmic activity in which individuals have to learn a choreography in synchrony with rhythmic stimuli provided by music and partners. Irrespective of the context (e.g., rehabilitation, education, and sport), current studies recommend individualizing music-based rhythmic cueing to induce motor improvement (Dalla Bella et al., 2018). Given that performance in synchronization-continuation tasks is improved when the tempo of stimuli is closest to the SMT (Delevoye-Turrell et al., 2014) and that the SMT seems to predict performance in externally paced tasks such as sensorimotor synchronization (McPherson et al., 2018), the choice of the tempo of the music should be carefully determined to correspond to the SMT. However, our systematic review highlights that the SMT is not a fixed and universal value but rather a range of values, so it should be measured just before intervention to provide a reference at the time of the intervention, considering the effectors used to produce the task and the current conditions. Accordingly, the measurement of SMT should be explicitly and exhaustively described to interpret the value obtained (including the instructions provided to measure the SMT). To consider the large intra-individual variability of the SMT, we advise performing more than a single trial per participant to measure the SMT. In line with the recommendation of Amrani and Golumbic (2020), SMT consistency should be measured within a trial, within a session, and across sessions (Amrani and Golumbic, 2020). Finally, it could be interesting to conduct a similar systematic review on the preferred perceived tempo (PPT), which can be measured either as the chosen tempo among several tempi (Baruch et al., 2004; Bauer et al., 2015) or from a dynamic tempo adjustment (speed up or slow down) of a rhythmic metronome until individuals reach their preferred tempo (e.g., Amrani and Golumbic, 2020; Hine et al., 2022). Given the possible relationship between the SMT and the preferred music tempo (e.g., Hine et al., 2022), it is possible that a common tempo for motor and perceived preferences exists. In the case of a common internal clock, we could expect that similar factors affect the SMT and the PPT.
Interdisciplinary implications extend to the field of rehabilitative, educative, and sport interventions involving rhythmic sensorimotor synchronization. Indeed, studies have highlighted the strong role of rhythm in engagement, motivation, and pleasure in performing physical activities. In the context of sport performance, music—through its intrinsic qualities, such as rhythm and particularly its tempo—is known to promote engagement and involvement in a physical activity or sport (Karageorghis et al., 2021). For example, synchronization with music during endurance-based activities (treadmill running tasks) allows for increased time spent practicing (Terry et al., 2012). More globally, results from a meta-analytic review support “the use of music listening across a range of physical activities to promote more positive affective valence, enhance physical performance (i.e., ergogenic effect), reduce perceived exertion, and improve physiological efficiency” (Terry et al., 2020, p. 91).
As a conclusion, the present review provides new elements to understand the inter- and intra-variability of the SMT, and we hope that our recommendations will be taken into account in future studies investigating performance in rhythmic production and perception tasks.
Author contributions
AD and JT primarily conducted this systematic review and wrote the first draft of the manuscript. EM provided expertise on the methodology for conducting a systematic review and participated in the discussions for the selection of articles. AD, EM, and JT collected all the information from the selected articles, provided feedback, and revised the manuscript. All authors contributed to the article and approved the submitted version.
Acknowledgments
We would like to thank the editor and the two reviewers for their very constructive comments and suggestions.
Conflict of interest
The authors declare that the research was conducted in the absence of any commercial or financial relationships that could be construed as a potential conflict of interest.
Publisher's note
All claims expressed in this article are solely those of the authors and do not necessarily represent those of their affiliated organizations, or those of the publisher, the editors and the reviewers. Any product that may be evaluated in this article, or claim that may be made by its manufacturer, is not guaranteed or endorsed by the publisher.
References
Amrani, A. K., and Golumbic, E. Z. (2020). Spontaneous and stimulus-driven rhythmic behaviors in ADHD adults and controls. Neuropsychologia 146, 107544. doi: 10.1016/j.neuropsychologia.2020.107544
Aridan, N., and Mukamel, R. (2016). Activity in primary motor cortex during action observation covaries with subsequent behavioral changes in execution. Brain Behav. 6, 550. doi: 10.1002/brb3.550
Assaneo, M. F., Rimmele, J. M., Sanz Perl, Y., and Poeppel, D. (2021). Speaking rhythmically can shape hearing. Nat. Hum. Behav. 5, 71–82. doi: 10.1038/s41562-020-00962-0
Aubin, L., Mostafaoui, G., Schmidt, R., Serré, H., and Marin, L. (2021). Effects of unintentional coordination on attentional load. Hum. Mov. Sci. 80, 102880. doi: 10.1016/j.humov.2021.102880
Avanzino, L., Lagravinese, G., Bisio, A., Perasso, L., Ruggeri, P., and Bove, M. (2015). Action observation: mirroring across our spontaneous movement tempo. Sci. Rep. 5, 10325. doi: 10.1038/srep10325
Baruch, C., Panissal-Vieu, N., and Drake, C. (2004). Preferred perceptual tempo for sound sequences: comparison of adults, children, and infants. Percept. Motor Skills 98, 325–339. doi: 10.2466/pms.98.1.325-339
Baudouin, A., Vanneste, S., and Isingrini, M. (2004). Age-related cognitive slowing: the role of spontaneous tempo and processing speed. Exp. Aging Res. 30, 225–239. doi: 10.1080/03610730490447831
Bauer, A.-K. R., Kreutz, G., and Herrmann, C. S. (2015). Individual musical tempo preference correlates with EEG beta rhythm. Psychophysiology 52, 600–604. doi: 10.1111/psyp.12375
Bégel, V., Bachrach, A., Dalla Bella, S., Laroche, J., Clément, S., Riquet, A., et al. (2022a). Dance improves motor, cognitive, and social skills in children with developmental cerebellar anomalies. Cerebellum 21, 264–279. doi: 10.1007/s12311-021-01291-2
Bégel, V., Benoit, C. E., Correa, A., Cutanda, D., Kotz, S. A., and Dalla Bella, S. (2017). “Lost in time” but still moving to the beat. Neuropsychologia 94, 129–138. doi: 10.1016/j.neuropsychologia.2016.11.022
Bégel, V., Dalla Bella, S., Devignes, Q., Vandenbergue, M., Lemaître, M.-P., and Dellacherie, D. (2022b). Rhythm as an independent determinant of developmental dyslexia. Dev. Psychol. 58, 339–358. doi: 10.1037/dev0001293
Bégel, V., Demos, A. P., Wang, M., and Palmer, C. (2022c). Social interaction and rate effects in models of musical synchronization. Front. Psychol. 13, 865536. doi: 10.3389/fpsyg.2022.865536
Bégel, V., Seilles, A., and Dalla Bella, S. (2018). Rhythm workers: a music-based serious game for training rhythm skills. Music Sci. 1, 2059204318794369. doi: 10.1177/2059204318794369
Benoit, C. E., Dalla Bella, S., Farrugia, N., Obrig, H., Mainka, S., and Kotz, S. A. (2014). Musically cued gait-training improves both perceptual and motor timing in Parkinson's disease. Front. Hum. Neurosci. 8, 494. doi: 10.3389/fnhum.2014.00494
Bichsel, O., Gassert, R., Stieglitz, L., Uhl, M., Baumann-Vogel, H., Waldvogel, D., et al. (2018). Functionally separated networks for self-paced and externally-cued motor execution in Parkinson's disease: evidence from deep brain recordings in humans. NeuroImage 177, 20–29. doi: 10.1016/j.neuroimage.2018.05.012
Bisio, A., Avanzino, L., Lagravinese, G., Biggio, M., Ruggeri, P., and Bove, M. (2015). Spontaneous movement tempo can be influenced by combining action observation and somatosensory stimulation. Front. Behav. Neurosci. 9, 228. doi: 10.3389/fnbeh.2015.00228
Blais, M., Jucla, M., Maziero, S., Albaret, J. M., Chaix, Y., and Tallet, J. (2021). The differential effects of auditory and visual stimuli on learning, retention and reactivation of a perceptual-motor temporal sequence in children with developmental coordination disorder. Front. Hum. Neurosci. 15, 616795. doi: 10.3389/fnhum.2021.616795
Bobin-Bègue, A., Provasi, J., Marks, A., and Pouthas, V. (2006). Influence of auditory tempo on the endogenous rhythm of non-nutritive sucking. Eur. Rev. Appl. Psychol. 56, 239–245. doi: 10.1016/j.erap.2005.09.006
Boulanger, N., Buisseret, F., Dehouck, V., Dierick, F., and White, O. (2020). Adiabatic invariants drive rhythmic human motion in variable gravity. Phys. Rev. E 102, e062403. doi: 10.1103/PhysRevE.102.062403
Bouvet, C. J., Varlet, M., Dalla Bella, S., Keller, P. E., and Bardy, B. G. (2020). Accent induced stabilization of spontaneous auditory-motor synchronization. Psychol. Res. 84, 2196–2209. doi: 10.1007/s00426-019-01208-z
Bouvet, C. J., Varlet, M., Dalla Bella, S., Keller, P. E., Zelic, G., and Bardy, B. G. (2019). Preferred frequency ratios for spontaneous auditory-motor synchronization: dynamical stability and hysteresis. Acta Psychol. 196, 33–41. doi: 10.1016/j.actpsy.2019.03.007
Bove, M., Tacchino, A., Pelosin, E., Moisello, C., Abbruzzese, G., and Ghilardi, M. F. (2009). Spontaneous movement tempo is influenced by observation of rhythmical actions. Brain Res. Bullet. 80, 122–127. doi: 10.1016/j.brainresbull.2009.04.008
Braun Janzen, T., Koshimori, Y., Richard, N. M., and Thaut, M. H. (2021). Rhythm and music-based interventions in motor rehabilitation: current evidence and future perspectives. Front. Hum. Neurosci. 15, 789467. doi: 10.3389/fnhum.2021.789467
Brennan, M., and Kirkland, J. (1982). Classification of infant cries using descriptive scales. Infant Behav. Dev. 5, 341–346. doi: 10.1016/S0163-6383(82)80044-1
Byblow, W., Carson, R., and Goodman, D. (1994). Expressions of asymmetries and anchoring in bimanual coordination. Hum. Mov. Sci. 13, 3–28. doi: 10.1016/0167-9457(94)90027-2
Byblow, W., Summers, J. J., Lewis, G. N., and Thomas, J. (2002). Bimanual coordination in Parkinson's disease: deficits in movement frequency, amplitude, and pattern switching. Mov. Disord. 17, 20–29. doi: 10.1002/mds.1281
Byblow, W. D., and Goodman, D. (1994). Performance asymmetries in multifrequency coordination. Hum. Mov. Sci. 13, 147–174. doi: 10.1016/0167-9457(94)90034-5
Carson, R. G., Riek, S., Byblow, W. D., Abernethy, B., and Summers, J. J. (1999). The timing of intralimb coordination. J. Motor Behav. 31, 113–118. doi: 10.1080/00222899909600982
Clark, C. N., Golden, H. L., and Warren, J. D. (2015). Acquired amusia. Handb. Clin. Neurol. 129, 607–631. doi: 10.1016/B978-0-444-62630-1.00034-2
Cochen De Cock, V., Dotov, D., Damm, L., Lacombe, S., Ihalainen, P., Picot, M. C., et al. (2021). BeatWalk: personalized music-based gait rehabilitation in Parkinson's disease. Front. Psychol. 12, 655121. doi: 10.3389/fpsyg.2021.655121
Collyer, C. E., Broadbent, H. A., and Church, R. M. (1994). Preferred rates of repetitive tapping and categorical time production. Percept. Psychophys. 55, 443–453. doi: 10.3758/BF03205301
Daigmorte, C., Tallet, J., and Astésano, C. (2022). On the foundations of rhythm-based methods in Speech Therapy. Proc. Speech Prosody 2022, 47–51. doi: 10.21437/SpeechProsody.2022-10
Dalla Bella, S., Benoit, C.-E., Farrugia, N., Keller, P. E., Obrig, H., Mainka, S., et al. (2017). Gait improvement via rhythmic stimulation in Parkinson's disease is linked to rhythmic skills. Sci. Rep. 7, 42005. doi: 10.1038/srep42005
Dalla Bella, S., Dotov, D., Bardy, B., and de Cock, V. C. (2018). Individualization of music-based rhythmic auditory cueing in Parkinson's disease. Ann. N. Y. Acad. Sci. 2018, 13859. doi: 10.1111/nyas.13859
De Pretto, M., Deiber, M.-P., and James, C. E. (2018). Steady-state evoked potentials distinguish brain mechanisms of self-paced versus synchronization finger tapping. Hum. Mov. Sci. 61, 151–166. doi: 10.1016/j.humov.2018.07.007
Delevoye-Turrell, Y., Dione, M., and Agneray, G. (2014). Spontaneous motor tempo is the easiest pace to act upon for both the emergent and the predictive timing modes. Proc. Soc. Behav. Sci. 126, 121–122. doi: 10.1016/j.sbspro.2014.02.338
Delevoye-Turrell, Y., Wilquin, H., and Giersch, A. (2012). A ticking clock for the production of sequential actions: where does the problem lie in schizophrenia? Schizophr. Res. 135, 51–54. doi: 10.1016/j.schres.2011.12.020
Dosseville, F., and LaRue, J. (2002). Effects of apnea on motor and cardiac rhythms. Auton. Neurosci. 99, 134–140. doi: 10.1016/S1566-0702(02)00133-9
Dosseville, F., Moussay, S., Larue, J., Gauthier, A., and Davenne, D. (2002). Physical exercise and time of day: influences on spontaneous motor tempo. Percept. Motor Skills 95, 965–972. doi: 10.1177/003151250209500301
Drake, C., Jones, M. R., and Baruch, C. (2000). The development of rhythmic attending in auditory sequences: attunement, referent period, focal attending. Cognition 77, 251–288. doi: 10.1016/S0010-0277(00)00106-2
Drewing, K., Aschersleben, G., and Li, S. C. (2006). Sensorimotor synchronization across the life span. Int. J. Behav. Dev. 2006, 165025406066764. doi: 10.1177/0165025406066764
Droit, S., Boldrini, A., and Cioni, G. (1996). Rhythmical leg movements in low-risk and brain-damaged preterm infants. Early Hum. Dev. 44, 201–213. doi: 10.1016/0378-3782(95)01709-7
Ejiri, K. (1998). Relationship between rhythmic behavior and canonical babbling in infant vocal development. Phonetica 55, 226–237. doi: 10.1159/000028434
Eriksson, P. O., Häggman-Henrikson, B., Nordh, E., and Zafar, H. (2000). Co-ordinated mandibular and head-neck movements during rhythmic jaw activities in man. J. Dental Res. 79, 1378–1384. doi: 10.1177/00220345000790060501
Fitzpatrick, P., Schmidt, R. C., and Lockman, J. J. (1996). Dynamical patterns in the development of clapping. Child Dev. 67, 2691–2708. doi: 10.2307/1131747
Flasskamp, A., Kotz, S. A., Schlegel, U., and Skodda, S. (2012). Acceleration of syllable repetition in Parkinson's disease is more prominent in the left-side dominant patients. Parkinson. Relat. Disord. 18, 343–347. doi: 10.1016/j.parkreldis.2011.11.021
Forrester, L., and Whitall, J. (2000). Bimanual finger tapping: effects of frequency and auditory information on timing consistency and coordination. J. Motor Behav. 32, 176–191. doi: 10.1080/00222890009601369
Frankford, S. A., Heller Murray, E. S., Masapollo, M., Cai, S., Tourville, J. A., Nieto-Castañón, A., et al. (2021). The neural circuitry underlying the “rhythm effect” in stuttering. J. Speech Lang. Hear. Res. 64, 2325–2346. doi: 10.1044/2021_JSLHR-20-00328
Frey, A., Lessard, A., Carchon, I., Provasi, J., and Pulido, L. (2022). Rhythmic training, literacy, and graphomotor skills in kindergarteners. Front. Psychol. 13, 959534. doi: 10.3389/fpsyg.2022.959534
Gabbard, C., and Hart, S. (1993). Foot-tapping speed in children ages 4 to 6 years. Percept. Motor Skills 77, 91–94. doi: 10.2466/pms.1993.77.1.91
Getchell, N. (2006). Age and task-related differences in timing stability, consistency, and natural frequency of children's rhythmic, motor coordination. Dev. Psychobiol. 48, 675–685. doi: 10.1002/dev.20186
Getchell, N., Forrester, L., and Whitall, J. (2001). Individual differences and similarities in the stability, timing consistency, and natural frequency of rhythmic coordinated actions. Res. Quart. Exerc. Sport 72, 13–21. doi: 10.1080/02701367.2001.10608927
Grahn, J. A. (2012). Neural mechanisms of rhythm perception: current findings and future perspectives. Top. Cogn. Sci. 4, 585–606. doi: 10.1111/j.1756-8765.2012.01213.x
Häggman-Henrikson, B., Zafar, H., and Eriksson, P. O. (2002). Disturbed jaw behavior in whiplash-associated disorders during rhythmic jaw movements. J. Dental Res. 81, 747–751. doi: 10.1177/0810747
Hammerschmidt, D., Frieler, K., and Woellner, C. (2021). Spontaneous motor tempo: investigating psychological, chronobiological, and demographic factors in a large-scale online tapping experiment. Front. Psychol. 12, 677201. doi: 10.3389/fpsyg.2021.677201
Hansen, E. A., Nøddelund, E., Nielsen, F. S., Sørensen, M. P., Nielsen, M. Ø., Johansen, M., et al. (2021). Freely chosen cadence during ergometer cycling is dependent on pedalling history. Eur. J. Appl. Physiol. 121, 3041–3049. doi: 10.1007/s00421-021-04770-w
Hansen, E. A., and Ohnstad, A. E. (2008). Evidence for freely chosen pedalling rate during submaximal cycling to be a robust innate voluntary motor rhythm. Exp. Brain Res. 186, 365–373. doi: 10.1007/s00221-007-1240-5
Hatsopoulos, N. G., and Warren, W. H. (1996). Resonance tuning in rhythmic arm movements. J. Motor Behav. 28, 3–14. doi: 10.1080/00222895.1996.9941728
Hattori, Y., Tomonaga, M., and Matsuzawa, T. (2015). Distractor effect of auditory rhythms on self-paced tapping in chimpanzees and humans. PLoS ONE 10, e0130682. doi: 10.1371/journal.pone.0130682
Hine, K., Abe, K., Kinzuka, Y., Shehata, M., Hatano, K., Matsui, T., et al. (2022). Spontaneous motor tempo contributes to preferred music tempo regardless of music familiarity. Front. Psychol. 13, 952488. doi: 10.3389/fpsyg.2022.952488
Horin, A. P., Harrison, E. C., Rawson, K. S., and Earhart, G. M. (2021). Finger tapping as a proxy for gait: similar effects on movement variability during external and self-generated cueing in people with Parkinson's disease and healthy older adults. Ann. Phys. Rehabil. Med. 64, 101402. doi: 10.1016/j.rehab.2020.05.009
James, E. G., Hong, S. L., and Newell, K. M. (2009). Development of dynamic stability in children's rhythmic movement. Dev. Psychobiol. 51, 465–473. doi: 10.1002/dev.20385
Karageorghis, C., Kuan, G., and Schiphof-Godart, L. (2021). Music in sport: from conceptual underpinnings to applications. Soc. Transpar. Open. Replicat. Kinesiol. 2021, B1023. doi: 10.51224/B1023
Kay, B. A., Kelso, J. A., Saltzman, E. L., and Schöner, G. (1987). Space–time behavior of single and bimanual rhythmical movements: data and limit cycle model. J. Exp. Psychol. 13, 178–192. doi: 10.1037/0096-1523.13.2.178
Keil, A., Elbert, T., Rockstroh, B., and Ray, W. J. (1998). Dynamical aspects of motor and perceptual processes in schizophrenic patients and healthy controls. Schizophr. Res. 33, 169–178. doi: 10.1016/S0920-9964(98)00069-3
Kohler, E., Keysers, C., Umiltà, M. A., Fogassi, L., Gallese, V., and Rizzolatti, G. (2002). Hearing sounds, understanding actions: action representation in mirror neurons. Science 297, 846–848. doi: 10.1126/science.1070311
Konczak, J., Ackermann, H., Hertrich, I., Spieker, S., and Dichgans, J. (1997). Control of repetitive lip and finger movements in Parkinson's disease: influence of external timing signals and simultaneous execution on motor performance. Mov. Disord. 12, 665–676. doi: 10.1002/mds.870120507
Konoike, N., and Nakamura, K. (2020). Cerebral substrates for controlling rhythmic movements. Brain Sci. 10, 514. doi: 10.3390/brainsci10080514
Kumai, M. (1999). Relation between self-paced and synchronized movement in persons with mental retardation. Percept. Motor Skills 89, 395–402. doi: 10.2466/pms.1999.89.2.395
Kuno, A., Akiyama, M., Yamashiro, C., Tanaka, H., Yanagihara, T., and Hata, T. (2001). Three-dimensional sonographic assessment of fetal behavior in the early second trimester of pregnancy. J. Ultras. Med. 20, 1271–1275. doi: 10.7863/jum.2001.20.12.1271
LaGasse, A. B. (2013). Influence of an external rhythm on oral motor control in children and adults. J. Music Ther. 50, 6–24. doi: 10.1093/jmt/50.1.6
Lagravinese, G., Bisio, A., Ruggeri, P., Bove, M., and Avanzino, L. (2017). Learning by observing: the effect of multiple sessions of action-observation training on the spontaneous movement tempo and motor resonance. Neuropsychologia 96, 89–95. doi: 10.1016/j.neuropsychologia.2016.09.022
Launay, J., Grube, M., and Stewart, L. (2014). Dysrhythmia: a specific congenital rhythm perception deficit. Front. Psychol. 5, 18. doi: 10.3389/fpsyg.2014.00018
Lê, M., Blais, M., Jucla, M., Chauveau, N., Maziero, S., Biotteau, M., et al. (2020a). Procedural learning and retention of audio-verbal temporal sequence is altered in children with developmental coordination disorder but cortical thickness matters. Dev. Sci. 2020, e13009. doi: 10.1111/desc.13009
Lê, M., Quémart, P., Potocki, A., Gimenes, M., Chesnet, D., and Lambert, E. (2020b). Rhythm in the blood: the influence of rhythm skills on literacy development in third graders. J. Exp. Child Psychol. 198, 104880. doi: 10.1016/j.jecp.2020.104880
Leong, V., Kalashnikova, M., Burnham, D., and Goswami, U. (2017). The temporal modulation structure of infant-directed speech. Open Mind 1, 78–90. doi: 10.1162/OPMI_a_00008
Loehr, J. D., and Palmer, C. (2011). Temporal coordination between performing musicians. Quart. J. Exp. Psychol. 64, 2153–2167. doi: 10.1080/17470218.2011.603427
Malcolm, B. R., Foxe, J. J., Butler, J. S., Molholm, S., and De Sanctis, P. (2018). Cognitive load reduces the effects of optic flow on gait and electrocortical dynamics during treadmill walking. J. Neurophysiol. 120, 2246–2259. doi: 10.1152/jn.00079.2018
Martin, E., Blais, M., Albaret, J.-M., Pariente, J., and Tallet, J. (2017). Alteration of rhythmic unimanual tapping and anti-phase bimanual coordination in Alzheimer's disease: a sign of inter-hemispheric disconnection? Hum. Mov. Sci. 55, 43–53. doi: 10.1016/j.humov.2017.07.007
Martínez Pueyo, A., García-Ruiz, P. J., Feliz, C. E., Garcia Caldentey, J., Del Val, J., and Herranz, A. (2016). Reaction time and rhythm of movement in Huntington's disease. J. Neurol. Sci. 362, 115–117. doi: 10.1016/j.jns.2015.12.037
McAuley, J. D., Jones, M. R., Holub, S., Johnston, H. M., and Miller, N. S. (2006). The time of our lives: life span development of timing and event tracking. J. Exp. Psychol. 135, 348–367. doi: 10.1037/0096-3445.135.3.348
McCombe Waller, S., and Whitall, J. (2004). Fine motor control in adults with and without chronic hemiparesis: baseline comparison to nondisabled adults and effects of bilateral arm training. Archiv. Phys. Med. Rehabil. 85, 1076–1083. doi: 10.1016/j.apmr.2003.10.020
McPherson, T., Berger, D., Alagapan, S., and Fröhlich, F. (2018). Intrinsic rhythmicity predicts synchronization-continuation entrainment performance. Sci. Rep. 8, 11782. doi: 10.1038/s41598-018-29267-z
Michaelis, K., Wiener, M., and Thompson, J. C. (2014). Passive listening to preferred motor tempo modulates corticospinal excitability. Front. Hum. Neurosci. 8, 252. doi: 10.3389/fnhum.2014.00252
Monier, F., and Droit-Volet, S. (2018). Synchrony and emotion in children and adults. Int. J. Psychol. 53, 184–193. doi: 10.1002/ijop.12363
Monier, F., and Droit-Volet, S. (2019). Development of sensorimotor synchronization abilities: motor and cognitive components. Child Neuropsychol. 25, 1043–1062. doi: 10.1080/09297049.2019.1569607
Moussay, S., Dosseville, F., Gauthier, A., Larue, J., Sesboüe, B., and Davenne, D. (2002). Circadian rhythms during cycling exercise and finger-tapping task. Chronobiol. Int. 19, 1137–1149. doi: 10.1081/CBI-120015966
Murata, J., Matsukawa, K., Shimizu, J., Matsumoto, M., Wada, T., and Ninomiya, I. (1999). Effects of mental stress on cardiac and motor rhythms. J. Auton. Nerv. Syst. 75, 32–37. doi: 10.1016/S0165-1838(98)00171-4
Oldfield, R. C. (1971). The assessment and analysis of handedness: the Edinburgh inventory. Neuropsychologia 9, 97–113. doi: 10.1016/0028-3932(71)90067-4
Oléron, G., Fraisse, P., Siffre, M., and Zuili, N. (1970). Les variations circadiennes du temps de réaction et du tempo spontané au cours d'une expérience “hors du temps”. L'Année Psychologique 70, 347–356. doi: 10.3406/psy.1970.27900
Ozernov-Palchik, O., and Patel, A. D. (2018). Musical rhythm and reading development: does beat processing matter? Ann. N. Y. Acad. Sci. 2018, 13853. doi: 10.1111/nyas.13853
Page, M. J., McKenzie, J. E., Bossuyt, P. M., Boutron, I., Hoffmann, T. C., Mulrow, C. D., et al. (2021). The PRISMA 2020 statement: an updated guideline for reporting systematic reviews. Systemat. Rev. 10, 89. doi: 10.1186/s13643-021-01626-4
Palmer, C., Lidji, P., and Peretz, I. (2014). Losing the beat: deficits in temporal coordination. Philos. Trans. Royal Soc. Lond. Ser. B Biol. Sci. 369, 20130405. doi: 10.1098/rstb.2013.0405
Peretz, I. (2016). Neurobiology of congenital amusia. Trends Cogn. Sci. 20, 857–867. doi: 10.1016/j.tics.2016.09.002
Pfordresher, P. Q., Greenspon, E. B., Friedman, A. L., and Palmer, C. (2021). Spontaneous production rates in music and speech. Front. Psychol. 12, 611867. doi: 10.3389/fpsyg.2021.611867
Phillips-Silver, J., Toiviainen, P., Gosselin, N., Piché, O., Nozaradan, S., Palmer, C., et al. (2011). Born to dance but beat deaf: a new form of congenital amusia. Neuropsychologia 49, 961–969. doi: 10.1016/j.neuropsychologia.2011.02.002
Poeppel, D., and Assaneo, M. F. (2020). Speech rhythms and their neural foundations. Nat. Rev. 21, 322–334. doi: 10.1038/s41583-020-0304-4
Provasi, J., Anderson, D. I., and Barbu-Roth, M. (2014a). Rhythm perception, production, and synchronization during the perinatal period. Front. Psychol. 5, 1048. doi: 10.3389/fpsyg.2014.01048
Provasi, J., and Bobin-Bègue, A. (2003). Spontaneous motor tempo and rhythmical synchronisation in 2½- and 4-year-old children. Int. J. Behav. Dev. 27, 220–231. doi: 10.1080/01650250244000290
Provasi, J., Doyère, V., Zélanti, P. S., Kieffer, V., Perdry, H., El Massioui, N., et al. (2014b). Disrupted sensorimotor synchronization, but intact rhythm discrimination, in children treated for a cerebellar medulloblastoma. Res. Dev. Disabil. 35, 2053–2068. doi: 10.1016/j.ridd.2014.04.024
Puyjarinet, F., Bégel, V., Lopez, R., Dellacherie, D., and Dalla Bella, S. (2017). Children and adults with Attention-Deficit/Hyperactivity Disorder cannot move to the beat. Sci. Rep. 7, 11295. doi: 10.1038/s41598-017-11295-w
Repp, B. H. (2005). Sensorimotor synchronization: a review of the tapping literature. Psychon. Bullet. Rev. 12, 969–992. doi: 10.3758/BF03206433
Repp, B. H., and Su, Y.-H. (2013). Sensorimotor synchronization: a review of recent research (2006–2012). Psychon. Bullet. Rev. 20, 403–452. doi: 10.3758/s13423-012-0371-2
Rizzolatti, G., and Craighero, L. (2004). The mirror-neuron system. Ann. Rev. Neurosci. 27, 169–192. doi: 10.1146/annurev.neuro.27.070203.144230
Robles-García, V., Corral-Bergantiños, Y., Espinosa, N., García-Sancho, C., Sanmartín, G., Flores, J., et al. (2016). Effects of movement imitation training in Parkinson's disease: a virtual reality pilot study. Parkinson. Relat. Disord. 26, 17–23. doi: 10.1016/j.parkreldis.2016.02.022
Rocha, S., Southgate, V., and Mareschal, D. (2020). Infant spontaneous motor tempo. Dev. Sci. 24, e13032. doi: 10.1111/desc.13032
Rocha, S., Southgate, V., and Mareschal, D. (2021). Rate of infant carrying impacts infant spontaneous motor tempo. Royal Soc. Open Sci. 8, 210608. doi: 10.1098/rsos.210608
Roche, R., Wilms-Floet, A. M., Clark, J. E., and Whitall, J. (2011). Auditory and visual information do not affect self-paced bilateral finger tapping in children with DCD. Hum. Mov. Sci. 30, 658–671. doi: 10.1016/j.humov.2010.11.008
Roerdink, M., Lamoth, C. J. C., van Kordelaar, J., Elich, P., Konijnenbelt, M., Kwakkel, G., et al. (2009). Rhythm perturbations in acoustically paced treadmill walking after stroke. Neurorehabil. Neural Repair 23, 668–678. doi: 10.1177/1545968309332879
Rose, D., Cameron, D. J., Lovatt, P. J., Grahn, J. A., and Annett, L. E. (2020). Comparison of spontaneous motor tempo during finger tapping, toe tapping and stepping on the spot in people with and without Parkinson's disease. J. Mov. Disord. 13, 47–56. doi: 10.14802/jmd.19043
Rose, D., Ott, L., Guérin, S. M. R., Annett, L. E., Lovatt, P., and Delevoye-Turrell, Y. N. (2021). A general procedure to measure the pacing of body movements timed to music and metronome in younger and older adults. Sci. Rep. 11, 3264. doi: 10.1038/s41598-021-82283-4
Rousanoglou, E. N., and Boudolos, K. D. (2006). Rhythmic performance during a whole body movement: dynamic analysis of force-time curves. Hum. Mov. Sci. 25, 393–408. doi: 10.1016/j.humov.2005.12.004
Rubia, K., Taylor, A., Taylor, E., and Sergeant, J. A. (1999). Synchronization, anticipation, and consistency in motor timing of children with dimensionally defined attention deficit hyperactivity behaviour. Percept. Motor Skills 89, 1237–1258. doi: 10.2466/pms.1999.89.3f.1237
Ruspantini, I., Saarinen, T., Belardinelli, P., Jalava, A., Parviainen, T., Kujala, J., et al. (2012). Corticomuscular coherence is tuned to the spontaneous rhythmicity of speech at 2–3 Hz. J. Neurosci. 32, 3786–3790. doi: 10.1523/JNEUROSCI.3191-11.2012
Sakamoto, M., Tazoe, T., Nakajima, T., Endoh, T., Shiozawa, S., and Komiyama, T. (2007). Voluntary changes in leg cadence modulate arm cadence during simultaneous arm and leg cycling. Exp. Brain Res. 176, 188–192. doi: 10.1007/s00221-006-0742-x
Sarasso, P., Barbieri, P., Del Fante, E., Bechis, L., Neppi-Modona, M., Sacco, K., et al. (2022). Preferred music listening is associated with perceptual learning enhancement at the expense of self-focused attention. Psychon. Bullet. Rev. 29, 2108–2121. doi: 10.3758/s13423-022-02127-8
Sardroodian, M., Madeleine, P., Voigt, M., and Hansen, E. A. (2014). Frequency and pattern of voluntary pedalling is influenced after one week of heavy strength training. Hum. Mov. Sci. 36, 58–69. doi: 10.1016/j.humov.2014.05.003
Schellekens, J. M., Scholten, C. A., and Kalverboer, A. F. (1983). Visually guided hand movements in children with minor neurological dysfunction: response time and movement organization. J. Child Psychol. Psychiatr. Allied Discipl. 24, 89–102. doi: 10.1111/j.1469-7610.1983.tb00106.x
Scheurich, R., Pfordresher, P. Q., and Palmer, C. (2020). Musical training enhances temporal adaptation of auditory-motor synchronization. Exp. Brain Res. 238, 81–92. doi: 10.1007/s00221-019-05692-y
Scheurich, R., Zamm, A., and Palmer, C. (2018). Tapping into rate flexibility: musical training facilitates synchronization around spontaneous production rates. Front. Psychol. 9, 458. doi: 10.3389/fpsyg.2018.00458
Schwartze, M., Keller, P. E., and Kotz, S. A. (2016). Spontaneous, synchronized, and corrective timing behavior in cerebellar lesion patients. Behav. Brain Res. 312, 285–293. doi: 10.1016/j.bbr.2016.06.040
Schwartze, M., Keller, P. E., Patel, A. D., and Kotz, S. A. (2011). The impact of basal ganglia lesions on sensorimotor synchronization, spontaneous motor tempo, and the detection of tempo changes. Behav. Brain Res. 216, 685–691. doi: 10.1016/j.bbr.2010.09.015
Schwartze, M., and Kotz, S. A. (2015). The timing of regular sequences: production, perception, and covariation. J. Cogn. Neurosci. 27, 1697–1707. doi: 10.1162/jocn_a_00805
Serrien, D. J. (2009). Verbal-manual interactions during dual task performance: an EEG study. Neuropsychologia 47, 139–144. doi: 10.1016/j.neuropsychologia.2008.08.004
Sidhu, S. K., and Lauber, B. (2020). Freely chosen cadence during cycling attenuates intracortical inhibition and increases intracortical facilitation compared to a similar fixed cadence. Neuroscience 441, 93–101. doi: 10.1016/j.neuroscience.2020.06.021
Slater, J., Ashley, R., Tierney, A., and Kraus, N. (2018). Got rhythm? Better inhibitory control is linked with more consistent drumming and enhanced neural tracking of the musical beat in adult percussionists and nonpercussionists. J. Cogn. Neurosci. 30, 14–24. doi: 10.1162/jocn_a_01189
Sofianidis, G., Hatzitaki, V., Grouios, G., Johannsen, L., and Wing, A. (2012). Somatosensory driven interpersonal synchrony during rhythmic sway. Hum. Mov. Sci. 31, 553–566. doi: 10.1016/j.humov.2011.07.007
Sotirakis, H., Stergiou, N., Patikas, D. A., and Hatzitaki, V. (2020). Age induced modifications in the persistency of voluntary sway when actively tracking the complex motion of a visual target. Neurosci. Lett. 738, 135398. doi: 10.1016/j.neulet.2020.135398
Stewart, L., von Kriegstein, K., Warren, J. D., and Griffiths, T. D. (2006). Music and the brain: disorders of musical listening. Brain 129, 2533–2553. doi: 10.1093/brain/awl171
Suzuki, K., and Ando, J. (2018). Genetic and environmental influences on personal and rhythmic-motor-activity tempo in children: a twin study. Japan. Psychol. Res. 60, 170–178. doi: 10.1111/jpr.12188
Tajima, M., and Choshi, K. (1999). Pattern formation in polyrhythmic tapping at a self-paced tempo. Percept. Motor Skills 88, 1160–1168. doi: 10.2466/pms.1999.88.3c.1160
Terry, P. C., Karageorghis, C. I., Curran, M. L., Martin, O. V., and Parsons-Smith, R. L. (2020). Effects of music in exercise and sport: a meta-analytic review. Psychol. Bullet. 146, 91–117. doi: 10.1037/bul0000216
Terry, P. C., Karageorghis, C. I., Saha, A. M., and D'Auria, S. (2012). Effects of synchronous music on treadmill running among elite triathletes. J. Sci. Med. Sport 15, 52–57. doi: 10.1016/j.jsams.2011.06.003
Tomyta, K., and Seki, Y. (2020). Effects of motor style on timing control and EEG waveforms in self-paced and synchronization tapping tasks. Neurosci. Lett. 739, 135410. doi: 10.1016/j.neulet.2020.135410
Tranchant, P., and Peretz, I. (2020). Basic timekeeping deficit in the Beat-based Form of Congenital Amusia. Sci. Rep. 10, 8325. doi: 10.1038/s41598-020-65034-9
Tranchant, P., Vuvan, D. T., and Peretz, I. (2016). Keeping the beat: a large sample study of bouncing and clapping to music. PLoS ONE 11, e0160178. doi: 10.1371/journal.pone.0160178
Treisman, M. (1963). Temporal discrimination and the indifference interval: implications for a model of the “internal clock”. Psychol. Monogr. 77, 1–31. doi: 10.1037/h0093864
Turgeon, M., and Wing, A. M. (2012). Late onset of age-related difference in unpaced tapping with no age-related difference in phase-shift error detection and correction. Psychol. Aging 27, 1152–1163. doi: 10.1037/a0029925
Van Wassenhove, V. (2022). Temporal disorientations and distortions during isolation. Neurosci. Biobehav. Rev. 137, 104644. doi: 10.1016/j.neubiorev.2022.104644
Vanneste, S., Pouthas, V., and Wearden, J. H. (2001). Temporal control of rhythmic performance: a comparison between young and old adults. Exp. Aging Res. 27, 83–102. doi: 10.1080/036107301750046151
Varlet, M., Marin, L., Issartel, J., Schmidt, R. C., and Bardy, B. G. (2012). Continuity of visual and auditory rhythms influences sensorimotor coordination. PLoS ONE 7, e44082. doi: 10.1371/journal.pone.0044082
Verzini de Romera, A. M. (1989). Industrial noise: some of its effects on human beings. Appl. Acoust. 28, 127–145. doi: 10.1016/0003-682X(89)90015-7
Volman, M. J. M., Laroy, M. E., and Jongmans, M. J. (2006). Rhythmic coordination of hand and foot in children with Developmental Coordination Disorder. Child 32, 693–702. doi: 10.1111/j.1365-2214.2006.00679.x
Wagener, D. S., and Colebatch, J. G. (1997). The influence of peripheral load on resetting voluntary movement by cortical stimulation: importance of the induced twitch. Exp. Brain Res. 117, 87–96. doi: 10.1007/s002210050202
Whitall, J., Forrester, L., and Song, S. (1999). Dual-finger preferred-speed tapping: effects of coordination mode and anatomical finger and limb pairings. J. Motor Behav. 31, 325–339. doi: 10.1080/00222899909600998
Wiener, M., Lohoff, F. W., and Coslett, H. B. (2011). Double dissociation of dopamine genes and timing in humans. J. Cogn. Neurosci. 23, 2811–2821. doi: 10.1162/jocn.2011.21626
Wittmann, M., von Steinbüchel, N., and Szelag, E. (2001). Hemispheric specialisation for self-paced motor sequences. Brain Res. 10, 341–344. doi: 10.1016/S0926-6410(00)00052-5
Wright, S. E., and Palmer, C. (2020). Physiological and behavioral factors in musicians' performance tempo. Front. Hum. Neurosci. 14, 311. doi: 10.3389/fnhum.2020.00311
Wurdeman, S. R., Myers, S. A., and Stergiou, N. (2013). Transtibial amputee joint motion has increased attractor divergence during walking compared to non-amputee gait. Ann. Biomed. Eng. 41, 806–813. doi: 10.1007/s10439-012-0705-2
Yahalom, G., Simon, E. S., Thorne, R., Peretz, C., and Giladi, N. (2004). Hand rhythmic tapping and timing in Parkinson's disease. Parkinson. Relat. Disord. 10, 143–148. doi: 10.1016/j.parkreldis.2003.10.001
Yu, L., and Myowa, M. (2021). The early development of tempo adjustment and synchronization during joint drumming: a study of 18- to 42-month-old children. Infancy 26, 635–646. doi: 10.1111/infa.12403
Zamm, A., Wang, Y., and Palmer, C. (2018). Musicians' natural frequencies of performance display optimal temporal stability. J. Biol. Rhyth. 33, 432–440. doi: 10.1177/0748730418783651
Zelaznik, H. N., Spencer, R. M., and Doffin, J. G. (2000). Temporal precision in tapping and circle drawing movements at preferred rates is not correlated: further evidence against timing as a general-purpose ability. J. Motor Behav. 32, 193–199. doi: 10.1080/00222890009601370
Zhao, Z., Salesse, R. N., Marin, L., Gueugnon, M., and Bardy, B. G. (2017). Likability's effect on interpersonal motor coordination: exploring natural gaze direction. Front. Psychol. 8, 1864. doi: 10.3389/fpsyg.2017.01864
Keywords: SMT, rhythm, intertap interval, intra-individual, inter-individual, variability, frequency
Citation: Desbernats A, Martin E and Tallet J (2023) Which factors modulate spontaneous motor tempo? A systematic review of the literature. Front. Psychol. 14:1161052. doi: 10.3389/fpsyg.2023.1161052
Received: 07 February 2023; Accepted: 02 August 2023;
Published: 18 October 2023.
Edited by:
Vassilis Sevdalis, University of Gothenburg, SwedenReviewed by:
Nicolas Farrugia, IMT Atlantique Bretagne-Pays de la Loire, FranceSinead Rocha, Anglia Ruskin University, United Kingdom
Copyright © 2023 Desbernats, Martin and Tallet. This is an open-access article distributed under the terms of the Creative Commons Attribution License (CC BY). The use, distribution or reproduction in other forums is permitted, provided the original author(s) and the copyright owner(s) are credited and that the original publication in this journal is cited, in accordance with accepted academic practice. No use, distribution or reproduction is permitted which does not comply with these terms.
*Correspondence: Anaïs Desbernats, YW5haXMuZGVzYmVybmF0c0BpbnNlcm0uZnI=; YW5haXMuZGVzYmVybmF0cy5wcm9AZ21haWwuY29t; Jessica Tallet, amVzc2ljYS50YWxsZXRAaW5zZXJtLmZy