- 1PhD Program in Cognitive Sciences, National Chung Cheng University, Chiayi City, Taiwan
- 2Center for Research in Cognitive Sciences, National Chung Cheng University, Chiayi City, Taiwan
- 3Department of Psychology, National Chung Cheng University, Chiayi City, Taiwan
Conventional Buddhist texts illustrate meditation as a condition of relaxed alertness that must fend against extreme hypoarousal (sleep, drowsiness) and extreme hyperarousal (restlessness). Theoretical, neurophysiological, and neuroimaging investigations of meditation have highlighted the relaxing effects and hypoarousing without emphasizing the alertness-promoting effects. Here we performed a systematic review supported by an activation-likelihood estimate (ALE) meta-analysis in an effort to counterbalance the surfeit of scholarship emphasizing the hypoarousing and relaxing effects of different forms of Buddhist meditation. Specifically, the current systematic review-cum-meta-analytical review seeks to highlight more support for meditation’s wake-promoting effects by drawing from neuroimaging research during wakefulness and meditation. In this systematic review and meta-analysis of 22 fMRI studies, we aim to highlight support for Buddhist meditation’s wake-promoting or arousing effects by identifying brain regions associated with alertness during meditation. The most significant peaks were localized medial frontal gyrus (MFG) and precuneus. We failed to determine areas ostensibly common to alertness-related meditation such as the medial prefrontal cortex (mPFC), superior parietal lobule, basal ganglia, thalamus, most likely due to the relatively fewer fMRI investigations that used wakefulness-promoting meditation techniques. Also, we argue that forthcoming research on meditation, related to alertness or wakefulness, continues to adopt a multi-modal method to investigate the correlation between actual behaviors and neural networks connected to Buddhist meditation. Moreover, we recommend the implementation of fMRI paradigms on Buddhist meditation with clinically diagnosed participants to complement recent trends in psychotherapy such as mindfulness-based cognitive therapy (MBCT).
Introduction
Meta-analytical evidence of arousing or wake-promoting effects in meditation
Meditation can be defined as a set of mental workouts-cum-exercises that regulate cognition, the self, and emotion, where a specific directing of attention and awareness influences mental and related somatic events. The practice of meditation has gained significant attention in recent years due to its potential to regulate cognition, emotion, and the self. Derived from Buddhist traditions, meditation involves specific directing of attention and awareness toward mental and somatic events, leading to a state of mind characterized by reduced negative emotions and improved positive states of mind (Goleman, 1977; Cardoso et al., 2004). Research in neuroscientific and behavioral investigations has shown the significance of studying meditation states and traits to acquire improved learning of attention, affective and cognitive neuroplasticity, awareness, and various states of mind (Adolphs, 2003; Cahn and Polich, 2006; Lutz et al., 2008a; Raffone and Srinivasan, 2010; Montero-Marin et al., 2019; Raffone et al., 2019). As such, clinical applications of meditation-based mental training initiatives are increasingly being devised and evaluated in various medical contexts and conditions (Kabat-Zinn, 1982; Cahn and Polich, 2006; Hofmann et al., 2010; Lin et al., 2018; Tang and Tang, 2020).
The popularity of meditation programs and practices derived from Buddhist traditions in the West has resulted in these practices being primarily deconstructed from their actual Buddhist meditational goal of “awakening” (Brown and Ryan, 2003; Lopez, 2009; Britton et al., 2014; Kabat-Zinn, 2018) and reconstructed as clinical interventions or forms of “psychological and physiological self-maintenance” (Beary and Benson, 1974; McMahan, 2008; Kabat-Zinn, 2013; Raffone et al., 2019; Tang and Tang, 2020). This has brought about some alterations in the traditional goals of the meditation practices and impacted how scientific work documents the effects of Buddhist meditation (Davidson, 2010; Williams and Kabat-Zinn, 2011; Kabat-Zinn, 2013; Lin et al., 2018; Tang and Tang, 2020). For instance, several Tibetan Buddhist scriptures explain the act of meditation as cultivating a state of mind of relaxed alertness (Amihai and Kozhevnikov, 2014). This mental state is about maintaining a continuous balance between hypoarousal, sleep, excessive relaxation, and mental dullness on one end and hyperarousal, agitation, and restlessness on another (Pandita, 1992; Gunaratana, 2002; Kozhevnikov et al., 2009; Bodhi, 2012; Amihai and Kozhevnikov, 2015; Kozhevnikov, 2019). Moreover, the focus has been given more to the relaxation effects of Buddhist meditation within the modern context without as much attention given to the wake-promoting or arousing effects. Thus, it is the aim of this study to highlight support for the wake-promoting or arousing effects of Buddhist meditation by identifying brain regions associated with alertness during meditation. This is achieved by performing an interdisciplinary systematic cum meta-analytical review to highlight evidence for the wake-promoting or arousing effect of Buddhist meditation.
It is observed that in some recent research, the objective of meditation work has aimed beyond relaxation and decreased arousal (Kozhevnikov et al., 2009; Amihai and Kozhevnikov, 2015; Lin et al., 2018; Basso et al., 2019; Chaudhary, 2019; Kozhevnikov, 2019). Such research includes a consciousness study (Williams and West, 1975; Fenwick et al., 1977; Cahn and Polich, 2006), a sleep equivalence study (Pagano et al., 1976), or a type of hibernation study (Younger et al., 1975) that is flipped by wakefulness (Walker and Berger, 1980). These types of studies usually associate meditation practices with non-specific relaxation techniques (Cooper and Aygen, 1979), where sleep and hypoarousal are seen as a fit instead of an impediment to concentration (Kyabgon, 2003).
When it comes to hypoarousal-related activities, Buddhism views attentive wakefulness as a significantly deep shift in a practitioner’s insight. This shift in perception is called “awakening.” The balance between mental laxity and agitation is achieved through meditation. The act of meditation helps the practitioner to reach a relaxed state that is imbued with zeal, courageous energy, and an alert and sharp awareness in a manner that the meditator is committed to wakefulness and fended against the fetters of sleepiness and tiredness (Nanamoli and Bodhi, 1995; Gyatso, 2001). Buddhist scholars, monks, and enthusiasts acquainted with conventional Buddhist objectives of meditation have criticized Western works on Buddhist meditation practice for their exaggeration of the relaxation part of meditation, which substantially overshadowed other aspects involved in Buddhist meditation (Wallace, 2006; McMahan, 2008).
While meditation programs and practices have gained popularity in the West, their original goals from Buddhist meditation have been altered to fit contemporary needs. However, some modern-day research aims to explore beyond relaxation and decreased arousal associated with meditation practices. Future studies should aim to identify brain regions associated with the wake-promoting or arousing effects of Buddhist meditation, in addition to exploring traditional Buddhist goals, to comprehensively understand the effects of meditation on cognitive (Chaudhary, 2018), affective, and physiological functions. Further, the present study seeks to address the gap in the literature by examining the wake-promoting effects of Buddhist meditation, a less explored aspect of meditation practice. The identification of brain regions associated with alertness during meditation could provide important insights into the mechanisms underlying the wake-promoting effects of meditation, potentially leading to the development of targeted meditation-based interventions for conditions characterized by excessive daytime sleepiness or fatigue. Furthermore, this study contributes to the growing literature on the cognitive and affective benefits of meditation, which has important implications for designing clinical and educational programs to promote mental and emotional well-being.
Association between different forms of Buddhist meditation and attention
Meditation is a widely practiced technique for improving cognitive control and emotional regulation. The different types of meditation practices are often classified into two styles: Focused Attention Meditation (FAM) and Open Monitoring Meditation (OMM). FAM involves focused attention on a given object, while OMM emphasizes open monitoring of experiences (Fujino et al., 2018; Raffone et al., 2019; Engström et al., 2022). In recent years, Loving-Kindness Meditation (LKM) and Compassion Meditation (CM) have gained importance as a third type of meditation, with an emphasis on specific intentions and sentiments (Kakumanu et al., 2019; Raffone et al., 2019; Engström et al., 2022).
Focused Attention Meditation involves sustained attention to a given object. This type of meditation is concerned with achieving a mental state of concentrated, serene focused attention, called “Samatha” (in English, it means quiescence) in Buddhist meditative practice. FAM also involves modulating attentional focus and noticing distractions generated by internal or external sources (Brandmeyer et al., 2019; Raffone et al., 2019; Engström et al., 2022). The monitoring and attentional processes of FAM have been associated with various neural networks involved in sustained and selective attention and attentional control (MacLean et al., 2010). A functional magnetic resonance imaging (fMRI) study conducted by Hasenkamp et al. (2012) revealed significant neural correlations of FA functions. This fMRI investigation also pinpointed distinct brain areas and systems associated with awareness, mind wandering, and shifting attention to and from the object.
Open Monitoring Meditation (OMM) involves non-reactive observation of experiences. This type of meditation is concerned with witnessing upcoming feelings, thoughts, and sensory occurrences within an open “background” of attention. In the event of shifting from an FAM to an OMM form of meditation, the item as the main attention is slowly substituted by maintaining an open presence or awareness (Lutz et al., 2007, 2008a). Behavioral research has revealed that OMM practitioners have improved conflict monitoring and more distributed attentional focus (Chan and Woollacott, 2007; Slagter et al., 2007; Tang et al., 2007; Tang and Tang, 2020). And they exhibit a reduced attentional blink or a more efficient way of allocating resources to serially presented positions. Further, OMM can lead to regulatory impacts on emotional functions from prefrontal modulation of limbic reactions (Hariri et al., 2000; Lieberman et al., 2007; Tang and Tang, 2020 see pp. 109–132).
Apart from FA and OM styles of meditation, LKM and CM are the other two notable types of Buddhist meditation. The practice involves unconditionally executing the sensations and concerns to oneself, other distinctive beings, or all living beings. Visualizing the mental image of the objects or intrinsic rays of light from one’s heart to others or concentrically everywhere to induce compassion and loving-kindness sensations and intentions is an essential component of LKM and CM practices (Khantipalo, 1984; Sujiva, 1991; Raffone et al., 2019). These types of meditation are usually practiced in fitting coordination with OM and FA to decrease unwholesome mental states (e.g., hatred, anger) and develop virtuousness (Buddharakkhita, 1995; The Dalai Lama, 2001; Hofmann et al., 2011).
Expert practitioners of LKM or CM trigger limbic regions and brain systems linked with processing mental states of other individuals (medial PFC, temporal lobes, posterior cingulate cortex, and temporoparietal junction), especially in the right hemisphere. Highly intense forms of CM activate the right inferior parietal lobule in the medial-posterior part of the right insula, dorsal ACC, and the somatosensory cortices (Lutz et al., 2009; Tang and Tang, 2020). Similarly, novice meditators given lessons in a mindfulness-based stress reduction (MBSR) program showed activation in the inferior parietal lobule and viscero-somatic areas such as the insula, right-lateralized network involving the lateral PFC and secondary somatosensory cortex when asked to perform experiential focusing (present moment awareness) (Kabat-Zinn, 1990, 2013; Farb et al., 2007; Baer, 2013).
Furthermore, bilateral activation in the dorsal medial prefrontal cortex and the rostral anterior cingulate cortex was noticed in Vipassana experts compared to beginners (Hölzel et al., 2007). The act of focusing on breathing during the auditory task deactivated the default mode network (DMN) (Buckner et al., 2008) in experienced Zen practitioners in comparison to beginners (Pagnoni et al., 2008). DMN is a set of neural networks that are operative when one is not involved in a work-induced exercise. It has been suggested that DMN is involved in self-referential functions (Buckner and Vincent, 2007; Qin and Northoff, 2011; Shi and He, 2020).
During breath-focused meditation (a form of FA style of meditation) (Pozuelos et al., 2019), it is observed that there is an increase in the BOLD signal in the right and left putamen and the supplementary motor area, and a decrease in activation in the right precuneus, the parietal-temporal cortex, and posterior cingulated cortex. Sustained meditation (falls under the umbrella term of FA type of meditation) increases neural activity in the head of the caudate nucleus bilaterally (Baerentsen, 2001; Baerentsen et al., 2010). In mindfulness meditation (a subjective form of meditation - may or may not include one or more types of meditation) (Grossman, 2010; Chiesa, 2012), it is also observed to decrease neural activity in cortical midline structures (CMS) connected with interoception (bilateral precuneus, right medial prefrontal cortex, and bilateral anterior insula) and substantially increase activity in the right posterior cingulate cortex (Ives-Deliperi et al., 2011).
In other meditation studies, researchers examined Theravada Buddhist monks, who were experienced in both Vipassana (OM/LKM/CM) and Samatha (FA) meditations and noticed that Samatha induces a series of increased activations in the right medial anterior prefrontal cortex and the right and left anterior cingulate cortex while witnessing deactivations in the left hemisphere (Manna et al., 2010). In contrast, those practicing only Vipassana meditation demonstrated activations in the left hemisphere, including the superior temporal gyrus, medial anterior prefrontal cortex, and superior parietal lobule. Similarly, expert Zen practitioners who mindfully observed emotional pictures exhibited a deactivation pattern in the default mode network, unrelated to brain areas responsible for emotional activity (Taylor et al., 2011). Other studies have shown that awareness of mind wandering activates the dorsal anterior cingulate cortex and bilateral anterior insula, while shifting attention from mind wandering to breathing activates the lateral inferior parietal cortex and the lateral and dorsal prefrontal cortex, with stronger activations in the right hemisphere. Sustaining focused attention on breathing activates the dorsolateral prefrontal cortex, which is associated with executive attention (Hasenkamp et al., 2012).
With reference to well-established approaches to the study of attention, attention can be divided into conflict monitoring or executive attention, alerting, and orienting attention (Posner, 2017). Alerting affects the noradrenaline brain network while orienting attention involves the brain’s parietal and frontal areas, including the frontal eye fields and inferior and superior parietal lobes. Conflict monitoring and resolution involve the anterior insula (AI), anterior cingulate cortex (ACC), and basal ganglia (Raz and Buhle, 2006; Braboszcz and Delorme, 2011; Petersen and Posner, 2012; Malinowski, 2013; Tang and Tang, 2020).
In this study, we discuss the phenomenon of alerting, which refers to achieving and sustaining a state of mental readiness that is highly responsive to incoming stimuli. Alerting can be categorized into two types: phasic alertness, which involves enhancing reaction following a warning signal or cue, and tonic alertness, also known as vigilant attention or intrinsic alertness (Langner and Eickhoff, 2013), which refers to the average level of vigilance, arousal, wakefulness, alertness, or mental state of readiness to notice or react to irregular or unanticipated cues. Tonic alertness serves as the cognitive tone for carrying out more complex processes, such as executive control and working memory, and is a fundamental requirement for other forms of attention (Sturm and Willmes, 2001). Previous research has shown that meditation can improve different types of attention, but less work has been done on tonic alertness. While Western scholarship tends to focus on the relaxation benefits of meditation, the positive correlation between arousal/wakefulness and attention has been well-supported in sleep and chronobiology research (Killgore, 2010). The relationship between arousal and meditation is a relatively new area of study in the field.
The link between attention and wakefulness
In the field of meditation, it is mainly observed that the deliberate training of attention (either in an open and receptive or focused and directed way) is an essential part of any meditation. This systematic review underlines open monitoring (OM) and focused attention (FA), the two common forms of attentional training that are paramount in Buddhist and many non-Buddhist meditational practices (Lutz et al., 2008b,2014; Guendelman et al., 2017; Sumantry and Stewart, 2021).
Tonic alertness (Langner and Eickhoff, 2013) is a broad level of alertness, arousal, wakefulness, vigilance or mental state of preparedness to respond to/notice unexpected or irregular stimuli (Fan et al., 2003; Oken et al., 2006). Another way to measure tonic alertness is by its deficit, which is a basic level of sleepiness, absence of sustained attention, or weariness depicted by mind wandering (Stawarczyk et al., 2011) or an attentional lapse (Sturm and Willmes, 2001; Fan et al., 2002; Weissman et al., 2006; Clemens et al., 2011; Arvidsson, 2018). This measure is possible as tonic alertness delivers the cognitive tone for serving more complicated functions, such as executive control and working memory (Posner, 2008; Clemens et al., 2011). Further, all the above-mentioned attention forms depend on tonic alertness (Raz and Buhle, 2006). Moreover, it is worth noticing that research in the pharmacological enhancement of alertness (Valentine and Sweet, 1999; Arnsten and Pliszka, 2011; Pinggal et al., 2022) or alertness training (Sturm et al., 2006; Melnychuk et al., 2018) showing improvements on a vast number of attentional tasks also supports the idea of assuming tonic alertness as a foundational prerequisite for other forms of attention. There exist a plethora of work done on all three types of attention (i.e., executive attention, alerting, and orienting attention) that have been researched in meditation studies (Chambers et al., 2008; Bushell, 2009; Lutz et al., 2009; Prakash et al., 2020; Tanaka et al., 2021), less emphasis has been placed on tonic alertness (Kozhevnikov et al., 2022).
Likewise, tonic alertness has been expressed in terms of improved vigilance or sustained attention (Jha et al., 2007; Kaul et al., 2010; Kozhevnikov, 2019; Valdez, 2019; Simor et al., 2020; Jaeger et al., 2021) but not in terms of increased arousal or wakefulness, an approach influenced by the modern western view that meditation stimulates sleep and relaxation rather than wakefulness or arousal. The favorable association between attention and arousal or wakefulness is apparent in everyday affairs. We all have witnessed, for example, that paying attention, learning, recalling, and regulating emotions are more challenging when we are exhausted or that extremely engaging in attentive activities before going to bed can hinder one’s sleep. The notion that attention is positively correlated with greater arousal and wakefulness is well-established within the fields of sleep and chronobiology (Killgore, 2010; Whitney et al., 2019; Hudson et al., 2020; Mishra et al., 2020), but this correlation has been somewhat less investigated within the domain of meditation research. Thus, this systematic review will explore the effects of Buddhist meditation on the index of sleep proneness or wakefulness, i.e., the activation of wake or sleep-corresponding brain regions.
Our study presents an activation-likelihood estimate (ALE) meta-analysis of functional neuroimaging studies on alertness-related meditation. Our study aims to integrate prior fMRI works on meditation that induce arousal or wakefulness effect, yielding insight into the brain networks that are most commonly associated with alertness-related meditation techniques. To identify studies for the meta-analysis, we included 22 fMRI studies that analyzed activation foci reflecting arousal, wakefulness, or alertness-related activation. We analyzed the operationalization of meditation techniques and experimental paradigms employed by these studies, allowing us to identify disparities within the brain networks associated with various aspects of alertness-related meditation.
Materials and methods
Search strategy (literature search and data sources)
PUBMED database was searched for functional imaging studies focusing on effects of Buddhist Meditation (BM) and Buddhist-derived Meditation Techniques (BdMT) on brain regions underlying tonic alertness or relaxation. As of October 2, 2021, the search terms were the following: ((“buddhis*” [all fields] or “buddhism” [all fields]) + (“meditation” [all fields]) + (“fMRI” [all fields] or “fMRI” [MeSH])); ((“buddhis*” [all fields] or “buddhism” [all fields]) + (“meditation” [all fields]) + (“neuroimaging” [all fields] or “neuroimaging” [MeSH])); ((“buddhis*” [all fields] or “buddhism” [all fields]) + (“meditation” [all fields]) + (“PET” [all fields] or “PET” [MeSH])); ((“buddhis*” [all fields] or “buddhism” [all fields]) + (“meditation” [all fields]) + (“neuro*” [all fields] or “neuro*” [MeSH])) and ((“mindfulness” [all fields] or “mindfulness” [MeSH]) + (“fMRI” [all fields] or “fMRI” [MeSH])). Searches were without limit on publication date and language. Also, the Boolean operators “OR,” and + was used to make the search more precise and accurate. Using of ‘AND’ was avoided because the search engine use this particular Boolean operator by default. The asterisk ‘*’ was used as a wildcard character to search for all words that contain the letters entered. For example, neuro* would find “neuroimaging” or “neurology” or “neuropathy.” A total of 685 exclusive fMRI studies were acknowledged (see Figure 1).
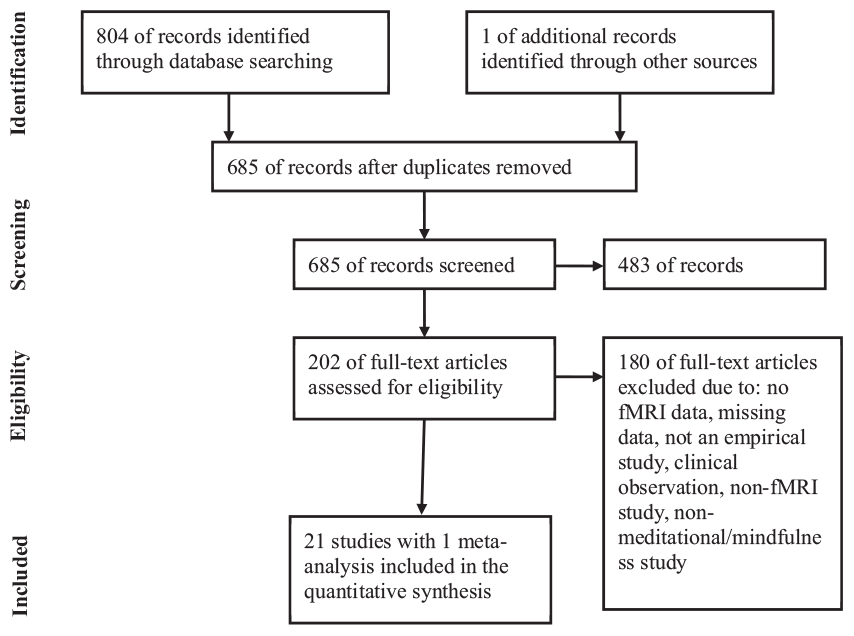
Figure 1. fMRI studies were screened and selected as per the PRISMA flow chart. Selection of fMRI studies was carried out in accordance with PRISMA guidelines for reporting systematic reviews (Moher et al., 2009).
Study selection/eligibility screening/eligibility criteria
The following are the inclusion-exclusion criteria for the systematic review-cum-meta-analyses:
(1) Articles that cited relaxation, arousal, tonic alertness, alertness, Buddhism, neuroimaging, mindfulness, and fMRI within the keywords, abstract, and title were considered and articles that did were rejected. At this screening stage, 202 of the 685 articles met the criterion.
(2) Research articles that employed fMRI were considered, whereas the work that utilized other techniques (i.e., EEG, PET, SPECT, TMS, behavioral efforts, and review articles) was rejected. Additionally, references of screened articles were carefully searched to identify more studies dealing with the brain systems responsible for arousal in Buddhist meditation practices. Further, the inclusion criteria held clinical sound adults and experiments where participants performed BM/BdMT during the fMRI procedure. Amidst a total of 202 studies, only 33 papers met this criterion.
(3) In the final screening process, only studies reporting whole-brain group analyses in a common reference space were considered. It was checked that the study performed a random-effects analysis. Further, both studies addressing only ROI analysis and no coordinates (N = 6), and anatomical studies showing structural changes (N = 7) were removed. This exercise ensured that the chosen neuro-imaging contrasts were as similar as possible (e.g., meditation vs. rest). However, when this homogeneity was not feasible, other comparisons were considered in the selecting articles (like meditation vs. control task). Following the above-stated criteria, data from a total of 22 papers (see Table 1 for the listing of all included studies) were included in the meta-analytical study.
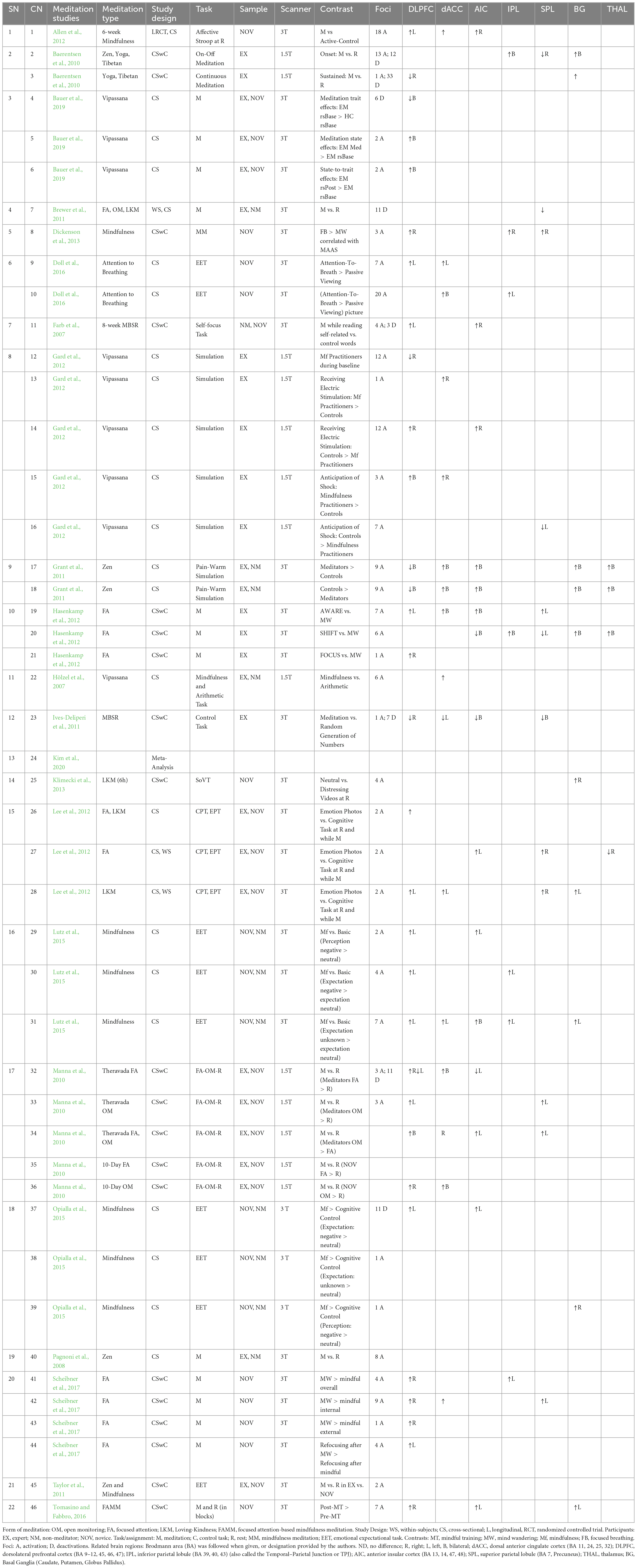
Table 1. Meditation studies depicting the effects of meditation practice on the brain regions related to alertness.
Data collection and extraction
Two reviewers independently searched the PubMed electronic database for relevant studies published up until October 2, 2021. The search strategy included a combination of keywords and MeSH terms related to Buddhist-derived Meditation Techniques (BdMT) on brain regions underlying tonic alertness or relaxation. Any discrepancies between the two reviewers were resolved through discussion or with a third reviewer.
After removing duplicates, the titles and abstracts of the remaining studies were screened for eligibility based on predetermined inclusion and exclusion criteria. Full texts of potentially relevant studies were then assessed for eligibility.
Data extraction was performed independently by two reviewers using a standardized data extraction form. Extracted data included study characteristics (e.g., author, year, country), participant characteristics (e.g., age, sex), intervention details (e.g., type of intervention, duration), outcome measures (e.g., primary and secondary outcomes), and results (e.g., effect sizes, statistical significance).
Any disagreements in data extraction were resolved through discussion or by consulting the third reviewer. If necessary, we contacted authors for additional information or data.
Quality assessment
The risk of bias assessment for the included studies was conducted using the Cochrane Collaboration’s tool for assessing the risk of bias in randomized trials (Higgins et al., 2011). The following domains were evaluated:
(1) Random sequence generation: Was the allocation sequence adequately generated?
(2) Allocation concealment: Was allocation adequately concealed?
(3) Blinding of participants and personnel: Were participants and personnel blinded to the intervention?
(4) Blinding of outcome assessment: Were outcome assessors blinded to the intervention?
(5) Incomplete outcome data: Were incomplete outcome data adequately addressed?
(6) Selective reporting: Was the study free of selective outcome reporting?
(7) Other sources of bias: Were there other potential sources of bias, such as funding or conflicts of interest?
The risk of bias assessment (see Table 2) was performed independently. For each domain, we assessed the risk of bias as low, high, or unclear/not reported. Disagreements were resolved by consensus or consultation. The results of the risk of bias assessment are presented in Table 2.
Data analysis procedures
Loci/voxels selection
Over-all, 40 experiments, where all voxels that explicitly reflected significant arousal-related brain activity, were assessed. All the coordinate spaces (MNI vs. Talairach space and Talairach space vs. MNI) were made uniform by transforming coordinates reported in Talairach coordinates into the MNI space using the mni2tal algorithm (Lancaster et al., 2007) and vice-versa. The linear transformation was calculated using Yale BioImage Suite version 1.2.0, 2020/08/25.1 The current systematic review-cum-meta-analyses included data from 1,139 participants and 302 activation foci.
Statistical approach
A Statistical plot was developed using lists of x, y, and z coordinates in the MNI space. The meta-analysis was performed using the most recent version (Eickhoff et al., 2009, 2012) of the Ginger ALE 3.0.2 software2 for coordinate-based meta-analysis of neuroimaging results (Turkeltaub et al., 2002; Laird et al., 2005). An advantage of Ginger ALE is that it allows random-effects inference by evaluating for above-chance clustering of foci between experiments (Laird et al., 2005, 2009; Turkeltaub et al., 2012). Here, the factor of uncertainty, which is inherent to the actual location of the peaks, is accounted for by modeling each coordinate as a 3D Gaussian function with a 12-mm Full Width at Half the Maximum (FWHM) than a single point. Hence, the localization likelihood distributions define the chance that a provided focus truly lies within a precise voxel (Laird et al., 2005, 2009; Eickhoff et al., 2009, 2012). The statistical effectiveness (or significance) is achieved by a permutation test of randomly induced foci, employing the exact FWHM and the number of foci. Ginger ALE meta-analysis demonstrates that meaningful outcomes are achieved if the convergence throughout the meditation studies happens more plausible than anticipated. But this doesn’t mean that every single or even the bulk of the meditation studies activate a certain region (Eickhoff et al., 2009, 2012). The voxel-wise comparison is checked contrariwise to the null hypothesis of uniformly distributed peaks, presenting a group of ALE values critical for thresholding the probability map. We corrected for multiple comparisons (Laird et al., 2005, 2009; Eickhoff et al., 2009, 2012) using the uncorrected p-value at 1.0E−4 and false-discovery rate (FDR) at p < 0.05 and used a 0 mm3 volume to define a cluster. The ALE thresholded maps values were laid on a canonical T1-weighted structural scan make use of Mango, a multi-image analysis viewer.3 The ALE meta-analysis also provided other outputs, such as information of location, anatomical labels, and cluster size, among others.
Results
After running the ALE meta-analysis, we didn’t find any significant activation with a false-discovery rate (FDR) at p < 0.05, when we used the uniformed MNI space as the input coordinates. But interestingly, we did find significant ALE activation when we inserted the uniformed Talairach space (see Supplementary Appendix Figure 2 and Supplementary Appendix Table 2). Further, we carried out the ALE meta-analysis with an uncorrected P-value of 1.0E−4 (using uniformed MNI space; see Supplementary Appendix Figure 1 and Supplementary Appendix Table 1) and we observed four brain regions - medial frontal gyrus (MFG), precuneus, insula, and inferior parietal lobule (IPL). In view of this review, we didn’t have any significant findings for uniform MNI space for FDR at p < 0.05, and as per the trend in neuroimaging studies, we decided to stick with using MNI space as the main reference for our results. To show credible and uniform anatomical terminology and labels across our meta-analytic study, we compared our coordinates of ALE activation with published meta-analyses, experimental data, and reviews. However, we did find significant results for the uncorrected P-value of 1.0E−4 using uniform MNI space. The same was confirmed when we used the uniform Talairach space coordinate system (see Supplementary Appendix Figure 3 and Supplementary Appendix Table 3).
Medial frontal gyrus
Our ALE analysis displayed invariant activation within the medial frontal gyrus (MFG) in the right hemisphere (see Supplementary Appendix Table 2, Cluster 1). Coordinates in our ALE activation seem to correspond with posterior parts involving Brodmann areas 9, 10, and 32.
Precuneus
The second consistent activation was revealed in the left Precuneus corresponding to the Brodmann area 31. And Brodmann areas 31 and 23 were only observed when ALE analysis was carried out with uncorrected P-value 1.0E−4 (see Supplementary Appendix Table 2, Cluster 2).
Insula
The third activation observed in ALE analysis with uncorrected p-value only (see Supplementary Appendix Table 2, Cluster 3) was in the left insula corresponding with Brodmann areas 13.
Inferior parietal lobule
The fourth activation revealed within the right inferior parietal lobule (IPL) was again only observed with uncorrected p-value (see Supplementary Appendix Table 2, Cluster 4) ALE analysis.
Discussion
The current study seeks to come up with meta-analytical evidence of wake-promoting or arousal effects in Buddhist meditation by rendering from the recent neuroimaging works. In the Buddhist forms of meditation, mindfulness is one of the primary aspects of the technique, exercised by means of executive attention focused on the mind (thought), body, and speech (breathing). An analysis of the neuroimaging studies related to Buddhist meditation practices has been a complex and challenging work due to the following reasons: data collected from various studies comes from multiple forms of meditation (our study reports data collected from more than three types of Buddhist meditation); huge deviation in meditational experiences among different groups of practitioners, varying from a few weeks for novice participants going through MBSR training or comparable programs to hundreds and thousands of hours for adept practitioners and monks; examining various stages of Buddhist meditation (beginner, intermediate and/or advanced stages).
These disparities make it difficult to analyze and interpret the results in a consistent manner, which likely may lead to incorrect conclusions (Chiesa et al., 2011, 2013; Tang et al., 2012). Our systematic review-cum-meta-analyses study showed consistent activations in the right MFG and left precuneus despite above mentioned methodological concerns. Apart from these two invariant activations, the left insula and right IPL also contributed to ALE meta-analysis.
Wakefulness and sleep tendency are linked to distinctive brain regions, which are also noticed to get activated during different meditation practices - both during tasks/at rest (in a trait-related manner) and during meditation (in a state-related manner). In general, reduced alertness and the shift in the wakeful state to the sleeping state are linked to receding activity in most brain regions, mainly frontal regions, whereas the observed increase in activity in the default mode network (DMN) (Rolls et al., 2003; Picchioni et al., 2008; Olbrich et al., 2009). Tonic alertness is correlated with the activity of cortical regions and subcortical systems in the right hemisphere, especially the dorsolateral prefrontal cortex (DLPFC), the dorsal anterior cingulate cortex (dACC), the anterior insula (AC), the IPL, the thalamus, and the brain stem (Posner and Petersen, 1990; Sturm et al., 1999; Sturm and Willmes, 2001; Mottaghy et al., 2006; O’Connell et al., 2006, 2008; Sadaghiani et al., 2009, 2010; Langner and Eickhoff, 2013). Several fMRI investigations have observed that meditation-related activations are more concentrated in the right cerebrum, which very much corresponds to tonic alertness–related brain regions (e.g., inferior parietal lobule, lateral PFC, and anterior Insula) (Lazar et al., 2000, 2005; Farb et al., 2007; Lutz et al., 2008a; Zeidan et al., 2011; Hasenkamp et al., 2012; Sato et al., 2012).
Our systematic review-cum-meta-analyses show a string of activation clusters, located in two regions: right MFG, and left precuneus. Activation of MFG is considered to be engaged in both social cognition (Amodio and Frith, 2006; Molenberghs et al., 2016) and performance monitoring (Van Noordt and Segalowitz, 2012). The medial frontal gyrus (MFG) and precuneus are two brain regions that have been consistently implicated in the practice of Buddhist meditation. Several studies have reported that experienced meditators exhibit increased activation in these regions compared to novices during meditation. The precise mechanisms underlying the involvement of these regions in meditation are not yet fully understood, but several theories have been proposed (Tang and Tang, 2020). One theory suggests that the MFG is involved in attentional control and cognitive flexibility, which are key components of mindfulness meditation. This theory suggests that meditation may enhance these cognitive processes by strengthening the neural circuits in the MFG. Supporting this theory, a study by Tang et al. (2015) found that mindfulness meditation training was associated with increased activation in the MFG during a working memory task, suggesting that meditation may enhance attentional control and cognitive flexibility through its effects on this region. Another theory suggests that the precuneus is involved in self-referential processing and self-awareness, which are important aspects of meditation. This theory suggests that meditation may enhance self-awareness by strengthening the neural circuits in the precuneus. Supporting this theory, a study by Garrison et al. (2015) found that experienced meditators exhibited increased connectivity between the precuneus and other regions involved in self-referential processing compared to novices. Other studies have suggested that the MFG and precuneus may be involved in more general processes related to meditation, such as attention regulation and emotion regulation. For example, a study by Hölzel et al. (2011) found that mindfulness meditation was associated with increased activation in the MFG and precuneus during a task requiring emotion regulation. This finding suggests that these regions may play a role in regulating emotional responses during meditation. Overall, while the precise mechanisms underlying the involvement of the MFG and precuneus in meditation are not yet fully understood, several theories have been proposed based on their known functional significance in attentional control, self-awareness, and emotion regulation. Further research is needed to fully elucidate the specific mechanisms underlying the involvement of these regions in meditation and their potential applications in clinical and non-clinical settings.
Activation of MFG is accompanied by dorsolateral prefrontal cortex (DLPFC, BA 9), anterior prefrontal cortex (BA 10), and dorsal anterior cingulate cortex (dACC, BA 32). En masse, these front part areas are correlated with a broad spectrum of multifarious processes, which comprises language (Poldrack et al., 1999; Katzev and Tu, 2013), executive functions like attention (Japee et al., 2015), and capability to examine emotion and valence (Amodio and Frith, 2006). Also, it is a widely accepted view that the ACC has a specific function in observing and resolution of dissension by deciding within vying processing options based on limited prior internal conscious plans (Pardo et al., 1991), in orienting the body position in reaction to sensorial stimuli (Vogt, 2005), in executive functions (Pardo et al., 1991; Vogt, 2005) and in pain modulation (opioid activity and placebo agency) (Colloca and Benedetti, 2005; Price et al., 2008). Including many of its functions, the dACC is considered to regulate arousal through multiple thalamic nuclei and brain stem noradrenergic activations (Sturm and Willmes, 2001). The ACC has been involved in the volitional regulation of arousal. The left-sided ACC is suggested to be more related to decreased arousal and right-sided activation with increased arousal (Critchley et al., 2000, 2001). Buddhist meditation traditions are seen to be related to increased activity in the dACC, during both tasks (Farb et al., 2010; Grant et al., 2011; Zeidan et al., 2011; Allen et al., 2012; Gard et al., 2012; Lee et al., 2012) and meditation (Brefczynski-Lewis et al., 2007; Hölzel et al., 2007; Manna et al., 2010; Short et al., 2010; Hasenkamp et al., 2012). Further, DLPFC has been observed to have greater neural activity throughout different forms of Buddhist meditation (including focused attention) (Fox et al., 2016). Increased activity levels in the DLPFC correspond to alert wakefulness (Buchsbaum et al., 1989; Sawaya and Ingvar, 1989; Maquet et al., 1990), whereas reduced activity in DLPFC is linked to increased sleep propensity and fatigue (Buchsbaum et al., 1989; Maquet et al., 1996; Braun et al., 1997). Buddhist meditation traditions are seen to be related to increased activity in the DLPFC, both during tasks (Farb et al., 2007; Zeidan et al., 2011; Allen et al., 2012; Lee et al., 2012) and during meditation (Ritskes et al., 2003; Brefczynski-Lewis et al., 2007; Manna et al., 2010; Short et al., 2010; Yu et al., 2011; Hasenkamp et al., 2012).
The second activation cluster observed in our ALE analysis predominantly involves the left precuneus along with the engagement of the dorsal posterior cingulate cortex (dPCC, BA 31). Both precuneus and PCC are a part of the default mode network (DMN), along with the medial prefrontal cortex (mPFC) and inferior parietal lobule (IPL). And it has been demonstrated that DMN constitutes the neural basis of self-referential processing (for example mind-wandering) (Raichle et al., 2001; Greicius et al., 2003), and the areas associated with DMN are mainly accountable for the notion of self and self-representation (Northoff et al., 2006). Corroborating evidence indicates that meditation practices may be linked to decreased DMN activity (Farb et al., 2007, 2010; Travis et al., 2010; Brewer et al., 2011; Taylor et al., 2011; Zeidan et al., 2011; Berkovich-Ohana et al., 2012). Also, look into counter-examples (Goldin et al., 2009, 2012; Goldin and Gross, 2010), rather than indicating mental rousing or mind wandering, activity in DMN is related to reduced levels of alertness and attentiveness (Carciofo et al., 2014) and decreased sympathetic arousal (Nagai et al., 2004). The DMN encloses some of the only brain regions that boost their activity during the transition from wakefulness to sleep (stage 1) (Picchioni et al., 2008; Olbrich et al., 2009), and alertness can be pharmacologically induced by repressing the DMN (Hahn et al., 2007). Thus, mind wandering and DMN activation can be regarded as a cue of drowsiness and mental laxity on a continuum with dreaming and sleep (stage 1) (Fox et al., 2013). From the above stance, we can say that decreased activity in DMN/mind wandering is not a pacifying effect in regard to relaxation/sleep, but relatively a push in the contrasting direction i.e., toward increased vigilance and alertness that offsets mental laxness and sleepiness. Hence, any relevant alterations of the “self” (engagement of cognitive or bodily selves) following meditational experiences should epitomize within the DMN. It is observed that the deactivation of the PCC for expert meditators during meditation is less mind wandering (such as less association with cognitive self) or insignificant goal-achieving exercise as practicing meditation is something like finishing a “task” or assignment (Brewer et al., 2011). Furthermore, the substantial coupling between the DMN and relevant attention and cognitive control areas indicates that decreased mind wandering is possibly executed by means of improved attention control.
Both ALE results were obtained using FDR (only in Talariach space) and uncorrected p-value activated medial frontal gyrus and precuneus. The uncorrected p-value gives two additional clusters comprising left insula, and right IPL (see Supplementary Appendix). The left insula is involved in interoception, or the perception of bodily sensations, while the right IPL is associated with spatial attention and self-other processing. One theory suggests that during meditation, practitioners may engage in introspection and self-awareness, leading to enhanced interoception and self-other processing, which in turn may activate the left insula and right IPL. This idea is supported by a study that found greater activation in the left insula and right IPL during a focused-attention meditation task compared to a control task (Brefczynski-Lewis et al., 2007). Another possible explanation for the involvement of these regions in meditation is related to the concept of decentering, or the ability to observe one’s thoughts and emotions from a detached perspective. This ability has been linked to increased activity in the left insula and right IPL (Farb et al., 2013). Thus, it is possible that meditation training may enhance decentering abilities, leading to increased activation in these regions.
Additionally, the right IPL has been shown to be involved in attentional control and cognitive flexibility, and some studies have suggested that meditation may enhance these abilities (Tang and Posner, 2009; Tang and Tang, 2020). Thus, it is possible that increased activation in the right IPL during meditation reflects enhanced attentional control and cognitive flexibility.
In the neuroscience of meditation, Insula is observed to be a significant brain area. Invariably, it is noticed to sustain interoceptive awareness such as bodily internal and visceral states alongside meta-cognitive awareness (Fleming and Dolan, 2012; Fox et al., 2014; Tang et al., 2015). Apart from underlying interoception (Critchley et al., 2004), the anterior insula (AI) has also been suggested to be the central node of the intrinsic alertness network (Sadaghiani et al., 2010; Langner and Eickhoff, 2013), the substrate of basic consciousness or awareness (Craig, 2009), and corresponds with states of autonomic arousal and wakefulness during meditation (Brefczynski-Lewis et al., 2007; Lutz et al., 2008a). The insula has been seen to have greater activation both during tasks following mindfulness training (Farb et al., 2007, 2010; Grant et al., 2011; Taylor et al., 2011; Zeidan et al., 2011; Allen et al., 2012; Gard et al., 2012) and meditation (Manna et al., 2010; Hasenkamp et al., 2012). Further, insula (anterior part), ACC, and basal ganglia are actively involved in executive attention (or conflict monitoring and resolution) (Raz and Buhle, 2006; Petersen and Posner, 2012). A relatively recent meta-analysis of fMRI studies of meditation has revealed that the insula and ACC brain areas are vital for most meditation techniques and traditions (Fox et al., 2016; Raffone et al., 2019; Tang and Tang, 2020). Likewise, other cross-sectional studies conducted with expert meditators (having experience of thousands of hours), who practice one particular type of meditation technique (focused attention, FA) have exhibited that the insula and ACC display more significant activation corresponded to clinical sound-matched controls (Fox et al., 2016). Following the progress in alerting and executive attention, subsequent longitudinal fMRI investigations demonstrated that brief integrative body-mind training (IBMT) during resting state renders more significant regional blood flow for the insula and ACC in comparison with the relaxation training group (Tang and Posner, 2009; Tang et al. 2015; Lin et al., 2018; Tang and Tang, 2020). Results from our ALE analysis, together with the above findings, provide substantial and coherent evidence that the deployment of attentional control in meditation practice corresponds to the insula and ACC. Furthermore, Insula and ACC displayed enhanced activation after repeated trials, implying that they perhaps are the central agencies of behavioral improvement in attentional control, specifically for executive attention.
Therefore, the involvement of the left insula and right IPL in Buddhist meditation likely reflects the enhanced interoception, self-awareness, self-other processing, decentering abilities, attentional control, and cognitive flexibility that are often associated with meditation practice.
The findings on the involvement of the medial frontal gyrus (MFG), precuneus, insula, and inferior parietal lobule (IPL) in Buddhist meditation have implications for clinical practice. These brain regions are involved in various cognitive processes, including attention, emotional regulation, and self-awareness, which are relevant to many clinical disorders. For example, studies have suggested that dysregulation of the MFG and precuneus is associated with symptoms of depression, anxiety, and post-traumatic stress disorder (PTSD) (Koenigs and Grafman, 2009; Lanius et al., 2010). The involvement of these regions in meditation could therefore have implications for the treatment of these disorders. In addition, the insula and IPL have been implicated in the experience of chronic pain and body awareness, and meditation has been shown to have potential as a treatment for chronic pain (Zeidan et al., 2012). Thus, the findings suggest that meditation may have potential as a complementary therapy for a range of clinical disorders that involve dysregulation of the brain regions implicated in Buddhist meditation.
The increased activation of the MGF and precuneus during meditation has been associated with improvements in attention, emotional regulation, and self-awareness, which are important factors in the treatment of various psychiatric disorders such as anxiety, depression, and addiction. The insula and IPL, on the other hand, have been linked to interoception and empathy, which are critical for the treatment of somatic disorders and certain personality disorders. Furthermore, recent studies have also shown that mindfulness-based interventions, which involve the cultivation of attention and awareness through Buddhist meditation practices, can be effective in reducing symptoms of various psychiatric disorders and improving overall well-being (Brewer et al., 2011). Therefore, the current findings on the involvement of MGF, precuneus, insula, and IPL in Buddhist meditation may provide a neurobiological basis for the therapeutic effects of mindfulness-based interventions and could inform the development of new interventions for clinical populations (Van Dam et al., 2018).
The present study aimed to provide a systematic review and meta-analysis of neuroimaging studies that investigate the arousing effects of Buddhist meditation practices. However, the analysis of these studies has been complicated by several methodological concerns, including the use of various forms of meditation, differences in participants’ meditational experiences, and the examination of different stages of meditation. Therefore, the results should be interpreted with caution due to the inconsistency of methods used across the studies. Additionally, the sample size of the included studies was limited, which may affect the generalizability of the findings. Furthermore, the studies employed different measures to assess wake-promoting or arousal effects, which may limit the conclusions that can be drawn from the results. Moreover, the heterogeneity in participant characteristics, including age, gender, ethnicity, and cultural background, may have influenced the results and restricted the generalizability of the findings. One limitation of Activation Likelihood Estimation (ALE) in our meta-analysis is that it relies on reported coordinates from neuroimaging studies, which can introduce publication bias. Studies with statistically significant results are more likely to be published, leading to an overrepresentation of significant findings in the meta-analysis. This bias can affect the accuracy of the ALE results and may not fully represent the entire body of research on the given topic. Additionally, some of the included studies did not have control groups, which may limit the interpretation of the results. Thus, the current study’s findings should be interpreted cautiously, and future research should address these limitations to provide more robust evidence of the effects of Buddhist meditation practices on brain function.
Conclusion
We performed the foremost meta-analysis on neuroimaging data related to arousing or wake-promoting effects in Buddhist meditation and determined five distinctive brain areas that were correlated to alertness or wakefulness during meditation. They were localized in the frontal and subcortical brain areas. Further, somewhat to our surprise, our ALE analysis failed to find evidence indicating the contribution of brain regions such as mPFC, superior parietal lobule, basal ganglia, and thalamus, which previous studies have suggested crucial to alertness-related Buddhist meditation (Manna et al., 2010; Raffone et al., 2019). This could be ascribable to a relatively smaller number of neuroimaging studies that utilized specific manipulations toward the effects of wakefulness in the different forms of Buddhist meditation. For future investigation, with a sufficient number of studies of different forms of meditation, it would be possible to carry out post hoc analyses of different subsets of meditation to tell apart the cortical activation linked with the different forms of meditation. Furthermore, we consider that future research will be able to link neural data to objective behavior by evaluating clinical data, which in turn may help the scientific community to further appreciate the mental health benefits of meditational practice focusing on alertness and wakefulness.
Data availability statement
The original contributions presented in this study are included in this article/Supplementary material, further inquiries can be directed to the corresponding author.
Author contributions
All authors listed have made a substantial, direct, and intellectual contribution to the work, and approved it for publication.
Funding
This study was supported in part by a grant awarded to the second author (GS) from the Ministry of Science and Technology (MOST) (Grant No. 109-2410-H-194-039-MY2), Taiwan, R.O.C., and Overseas Research Scholarship (ORS) awarded to the first author (IC) by the National Chung Cheng University, Taiwan.
Conflict of interest
The authors declare that the research was conducted in the absence of any commercial or financial relationships that could be construed as a potential conflict of interest.
Publisher’s note
All claims expressed in this article are solely those of the authors and do not necessarily represent those of their affiliated organizations, or those of the publisher, the editors and the reviewers. Any product that may be evaluated in this article, or claim that may be made by its manufacturer, is not guaranteed or endorsed by the publisher.
Supplementary material
The Supplementary Material for this article can be found online at: https://www.frontiersin.org/articles/10.3389/fpsyg.2023.1136983/full#supplementary-material
Footnotes
- ^ https://bioimagesuiteweb.github.io/webapp/mni2tal.html
- ^ https://brainmap.org/
- ^ http://ric.uthscsa.edu/mango/
References
Adolphs, R. (2003). Cognitive neuroscience of human social behavior. Nat. Rev. Neurosci. 4, 165–178. doi: 10.1038/nrn1056
Allen, M., Dietz, M., Blair, K. S., Beek, M v, Rees, G., Vestergaard-Poulsen, P., et al. (2012). Cognitive affective neural plasticity following active-controlled mindfulness intervention. J. Neurosci. 32, 15601–15610. doi: 10.1523/JNEUROSCI.2957-12.2012
Amihai, I., and Kozhevnikov, M. (2014). Arousal vs. relaxation: A comparison of the neurophysiological and cognitive correlates of vajrayana and theravada meditative practices. PLoS One 9:e102990. doi: 10.1371/journal.pone.0102990
Amihai, I., and Kozhevnikov, M. (2015). The influence of buddhist meditation traditions on the autonomic system and attention. BioMed Res. Int 2015:731579. doi: 10.1155/2015/731579
Amodio, D. M., and Frith, C. D. (2006). Meeting of minds: the medial frontal cortex and social cognition. Nat. Rev. Neurosci. 7, 268–277. doi: 10.1038/nrn1884
Arnsten, A. F., and Pliszka, S. R. (2011). Catecholamine influences on prefrontal cortical function: relevance to the treatment of attention deficit/hyperactivity disorder and related disorders. Pharmacol. Biochem. Behav. 99, 211–216. doi: 10.1016/j.pbb.2011.01.020
Arvidsson, A. (2018). Meditation, attention, and the brain: function, structure, and attentional performance. Washington, DC: Spring, doi: 10.13140/RG.2.2.22543.41122
Baer, R. A. (2013). Mindfulness training as a clinical intervention: a conceptual and empirical review. Clin. Psychol. 10, 125–143.
Baerentsen, K. B., Stødkilde-Jørgensen, H., Sommerlund, B., Hartmann, T., Damsgaard-Madsen, J., Fosnaes, M., et al. (2010). An investigation of brain processes supporting meditation. Cogn. Process. 11, 57–84. doi: 10.1007/s10339-009-0342-3
Basso, J. C., McHale, A., Ende, V., Oberlin, D. J., and Suzuki, W. A. (2019). Brief, daily meditation enhances attention, memory, mood, and emotional regulation in non-experienced meditators. Behav. Brain res. 356, 208–220. doi: 10.1016/j.bbr.2018.08.023
Bauer, C., Whitfield-Gabrieli, S., Díaz, J. L., Pasaye, E. H., and Barrios, F. A. (2019). From state-to-trait meditation: reconfiguration of central executive and default mode networks. eNeuro 6, ENEURO.335–ENEURO.318. doi: 10.1523/ENEURO.0335-18.2019
Beary, J. F., and Benson, H. (1974). A simple psychophysiological technique which elicits the hypometabolic changes of the relaxation response. Psychos. Med. 36, 115–120. doi: 10.1097/00006842-197403000-00003
Berkovich-Ohana, A., Glicksohn, J., and Goldstein, A. (2012). Mindfulness-induced changes in gamma band activity— implications for the default mode network, self-reference, and attention. Clin. Neurophysiol. 123, 700–710. doi: 10.1016/j.clinph.2011.07.048
Bodhi, B. (2012). The Numerical Discourses of the Buddha: A New Translation of the Anguttara Nikaya. Boston, MA: Wisdom.
Braboszcz, C., and Delorme, A. (2011). Lost in thoughts: neural markers of low alertness during mind wandering. Neuroimage 54, 3040–3047. doi: 10.1016/j.neuroimage.2010.10.008
Brandmeyer, T., Delorme, A., and Wahbeh, H. (2019). The neuroscience of meditation: classification, phenomenology, correlates, and mechanisms. Progress Brain Res. 244, 1–29. doi: 10.1016/bs.pbr.2018.10.020
Braun, A. R., Balkin, T. J., Wesenten, N. J., Carson, R. E., Varga, M., Baldwin, P., et al. (1997). Regional cerebral blood flow throughout the sleep-wake cycle. An H2(15)O PET study. Brain 120, 1173–1197. doi: 10.1093/brain/120.7.1173
Brefczynski-Lewis, J. A., Lutz, A., Schaefer, H. S., Levinson, D. B., and Davidson, R. J. (2007). Neural correlates of attentional expertise in long-term meditation practitioners. Proc. Natl. Acad. Sci. U. S. A. 104, 11483–11488. doi: 10.1073/pnas.0606552104
Brewer, J. A., Worhunsky, P. D., Gray, J. R., Tang, Y. Y., Weber, J., and Kober, H. (2011). Meditation experience is associated with differences in default mode network activity and connectivity. Proc. Natl. Acad. Sci. U. S. A. 108, 20254–20259. doi: 10.1073/pnas.1112029108
Britton, W. B., Lindahl, J. R., Cahn, B. R., Davis, J. H., and Goldman, R. E. (2014). Awakening is not a metaphor: the effects of Buddhist meditation practices on basic wakefulness. Ann. N. Y. Acad. Sci. 1307, 64–81. doi: 10.1111/nyas.12279
Brown, K., and Ryan, R. (2003). The benefits of being present: mindfulness and its role in psychological well-being. J. Pers. Soc. Psychol. 84, 822–848.
Buchsbaum, M. S., Gillin, J. C., Wu, J., Hazlett, E., Sicotte, N., Dupont, R. M., et al. (1989). Regional cerebral glucose metabolic rate in human sleep assessed by positron emission tomography. Life Sci. 45, 1349–1356. doi: 10.1016/0024-3205(89)90021-0
Buckner, R. L., Andrews-Hanna, J. R., and Schacter, D. L. (2008). The brain’s default network. Ann. N. Y. Acad. Sci. 1124, 1–38.
Buckner, R. L., and Vincent, J. L. (2007). Unrest at rest: Default activity and spontaneous network correlations. Neuroimage 37, 1091–1099. doi: 10.1016/j.neuroimage.2007.01.010
Buddharakkhita, A. (1995). Metta: The Philosophy and Practice of Universal Love. Kandy: Buddhist Publication Society.
Bushell, W. C. (2009). New beginnings: evidence that the meditational regimen can lead to optimization of perception, attention, cognition, and other functions. Ann. N. Y. Acad. Sci. 1172, 348–361. doi: 10.1111/j.1749-6632.2009.04960.x
Cahn, B. R., and Polich, J. (2006). Meditation states and traits: EEG, ERP, and neuroimaging studies. Psychol. Bull. 132, 180–211.
Carciofo, R., Du, F., Song, N., and Zhang, K. (2014). Chronotype and time-of-day correlates of mind wandering and related phenomena. Biol. Rhythm Res. 45, 37–49. doi: 10.1080/09291016.2013.790651
Cardoso, R., de Souza, E., Camano, L., and Leite, J. R. (2004). Meditation in health: an operational definition. Brain Res. 14, 58–60. doi: 10.1016/j.brainresprot.2004.09.002
Chambers, R., Lo, B. C. Y., and Allen, N. B. (2008). The impact of intensive mindfulness training on attentional control, cognitive style, and affect. Cogn. Therapy Res. 32, 303–322. doi: 10.1186/s12913-016-1423-5
Chan, D., and Woollacott, M. (2007). Effects of level of meditation experience on attentional focus: is the efficiency of executive or orientation networks improved? J. Altern. Compl. Med. 13, 651–657.
Chaudhary, I. S. (2018). To analyze the effect of visualization of Tantric Buddhism on the cognitive behavior of a tantric practitioner. Thimpu: Vajrayāna Conference.
Chaudhary, I. S. (2019). Attentional functioning in deity yoga practitioners, Master’s thesis. Pilkhi: Nalanda University.
Chiesa, A. (2012). The difficulty of defining mindfulness: current thought and critical issues. Mindfulness 4, 255–268.
Chiesa, A., Brambilla, P., and Serretti, A. (2011). Neuro-imaging of mindfulness meditations: Implications for clinical practice. Epidemiol. Psychiatr. Sci. 20, 205–210. doi: 10.1017/s204579601100028x
Chiesa, A., Serretti, A., and Jakobsen, J. C. (2013). Mindfulness: top-down or bottom-up emotion regulation strategy? Clin. Psychol. Rev. 33, 82–96. doi: 10.1016/j.cpr.2012.10.006
Clemens, B., Zvyagintsev, M., Sack, A. T., Heinecke, A., Willmes, K., and Sturm, W. (2011). Revealing the functional neuroanatomy of intrinsic alertness using fMRI: methodological peculiarities. PLoS One 6:e25453. doi: 10.1371/journal.pone.0025453
Colloca, L., and Benedetti, F. (2005). Placebos and painkillers: is mind as real as matter? Nat. Rev. 6, 545–552. doi: 10.1038/nrn1705
Cooper, M. J., and Aygen, M. M. (1979). A relaxation technique in the management of hypercholesterolemia. J. Hum. Stress 5, 24–27.
Craig, A. D. (2009). How do you feel–now? The anterior insula and human awareness. Nat. Rev. Neurosci. 10, 59–70. doi: 10.1038/nrn2555
Critchley, H. D., Corfield, D. R., Chandler, M. P., Mathias, C. J., and Dolan, R. J. (2000). Cerebral correlates of autonomic cardiovascular arousal: A functional neuroimaging investigation in humans. J. Physiol. 523, 259–270. doi: 10.1111/j.1469-7793.2000.t01-1-00259.x
Critchley, H. D., Melmed, R. N., Featherstone, E., Mathias, C. J., and Dolan, R. J. (2001). Brain activity during biofeedback relaxation: A functional neuroimaging investigation. Brain 124, 1003–1012. doi: 10.1093/brain/124.5.1003
Critchley, H. D., Wiens, S., Rotshtein, P., Ohman, A., and Dolan, R. J. (2004). Neural systems supporting interoceptive awareness. Nat. Neurosci. 7, 189–195. doi: 10.1038/nn1176
Davidson, R. J. (2010). Empirical explorations of mindfulness: Conceptual and methodological conundrums. Emotion 10, 8–11. doi: 10.1037/a0018480
Dickenson, J., Berkman, E. T., Arch, J., and Lieberman, M. D. (2013). Neural correlates of focused attention during a brief mindfulness induction. Soc. Cogn. Affect. Neurosci. 8, 40–47. doi: 10.1093/scan/nss030
Doll, A., Hölzel, B. K., Mulej Bratec, S., Boucard, C. C., Xie, X., Wohlschläger, A. M., et al. (2016). Mindful attention to breath regulates emotions via increased amygdala-prefrontal cortex connectivity. NeuroImage 134, 305–313. doi: 10.1016/j.neuroimage.2016.03.041
Eickhoff, S. B., Bzdok, D., Laird, A. R., Kurth, F., and Fox, P. T. (2012). Activation likelihood estimation meta-analysis revisited. NeuroImage 59, 2349–2361. doi: 10.1016/j.neuroimage.2011.09.017
Eickhoff, S. B., Laird, A. R., Grefkes, C., Wang, L. E., Zilles, K., and Fox, P. T. (2009). Coordinate-based activation likelihood estimation meta-analysis of neuroimaging data: A random-effects approach based on empirical estimates of spatial uncertainty. Hum. Brain Mapp. 30, 2907–2926. doi: 10.1002/hbm.20718
Engström, M., Willander, J., and Simon, R. (2022). A review of the methodology, taxonomy, and definitions in recent fMRI research on meditation. Mindfulness 13, 541–555. doi: 10.1007/s12671-021-01782-7
Fan, J., McCandliss, B. D., Sommer, T., Raz, A., and Posner, M. I. (2002). Testing the efficiency and independence of attentional networks. J. Cogn. Neurosci. 14, 340–347. doi: 10.1162/089892902317361886
Fan, J., Raz, A., and Posner, M. I. (2003). “Attentional mechanisms,” in Encyclopedia of Neurological Sciences, eds M. Aminoff and R. Daroff (Amsterdam: Elsevier).
Farb, N. A., Anderson, A. K., Mayberg, H., Bean, J., McKeon, D., and Segal, Z. V. (2010). Minding one’s emotions: mindfulness training alters the neural expression of sadness. Emotion 10, 25–33. doi: 10.1037/a0017151
Farb, N. A., Segal, Z. V., and Anderson, A. K. (2013). Mindfulness meditation training alters cortical representations of interoceptive attention. Soc. Cogn. Affect. Neurosci. 8, 15–26. doi: 10.1093/scan/nss066
Farb, N. A., Segal, Z. V., Mayberg, H., Bean, J., McKeon, D., Fatima, Z., et al. (2007). Attending to the present: mindfulness meditation reveals distinct neural modes of self-reference. Soc. Cogn. Affect. Neurosci. 2, 313–322. doi: 10.1093/scan/nsm030
Fenwick, P. B., Donaldson, S., Gillis, L., Bushman, J., Fenton, G. W., Perry, I., et al. (1977). Metabolic and EEG changes during transcendental meditation: an explanation. Biol. Psychol. 5, 101–118. doi: 10.1016/0301-0511(77)90007-2
Fleming, S. M., and Dolan, R. J. (2012). The neural basis of metacognitive ability. Philos. Trans. R. Soc. B Biol. Sci. 367, 1338–1349.
Fox, K. C., Dixon, M. L., Nijeboer, S., Girn, M., Floman, J. L., Lifshitz, M., et al. (2016). Functional neuroanatomy of meditation: A review and meta-analysis of 78 functional neuroimaging investigations. Neurosci. Biobehav. Revi. 65, 208–228. doi: 10.1016/j.neubiorev.2016.03.021
Fox, K. C., Nijeboer, S., Dixon, M. L., Floman, J. L., Ellamil, M., Rumak, S. P., et al. (2014). Is meditation associated with altered brain structure? A systematic review and meta-analysis of morphometric neuroimaging in meditation practitioners. Neurosci. Biobehav. Rev. 43, 48–73. doi: 10.1016/j.neubiorev.2014.03.016
Fox, K. C., Nijeboer, S., Solomonova, E., Domhoff, G. W., and Christoff, K. (2013). Dreaming as mind wandering: evidence from functional neuroimaging and first-person content reports. Front. Hum. Neurosci. 7:412. doi: 10.3389/fnhum.2013.00412
Fujino, M., Ueda, Y., Mizuhara, H., Saiki, J., and Nomura, M. (2018). Open monitoring meditation reduces the involvement of brain regions related to memory function. Sci. Rep. 8:9968. doi: 10.1038/s41598-018-28274-4
Gard, T., Hölzel, B. K., Sack, A. T., Hempel, H., Lazar, S. W., Vaitl, D., et al. (2012). Pain attenuation through mindfulness is associated with decreased cognitive control and increased sensory processing in the brain. Cereb.Cortex 22, 2692–2702. doi: 10.1093/cercor/bhr352
Garrison, K. A., Zeffiro, T. A., Scheinost, D., Constable, R. T., and Brewer, J. A. (2015). Meditation leads to reduced default mode network activity beyond an active task. Cogn. Affect. Behav. Neurosci. 15, 712–720. doi: 10.3758/s13415-015-0358-3
Goldin, P., Ramel, W., and Gross, J. (2009). Mindfulness meditation training and self-referential processing in social anxiety disorder: Behavioral and neural effects. J. Cogn. Psychol. 23, 242–257. doi: 10.1891/0889-8391.23.3.242
Goldin, P., Ziv, M., Jazaieri, H., and Gross, J. J. (2012). Randomized controlled trial of mindfulness-based stress reduction versus aerobic exercise: effects on the self-referential brain network in social anxiety disorder. Front. Hum. Neurosci. 6:295. doi: 10.3389/fnhum.2012.00295
Goldin, P. R., and Gross, J. J. (2010). Effects of mindfulness-based stress reduction (MBSR) on emotion regulation in social anxiety disorder. Emotion 10, 83–91.
Grant, J. A., Courtemanche, J., and Rainville, P. (2011). A nonelaborative mental stance and decoupling of executive and pain-related cortices predicts low pain sensitivity in Zen meditators. Pain 152, 150–156. doi: 10.1016/j.pain.2010.10.006
Greicius, M. D., Krasnow, B., Reiss, A. L., and Menon, V. (2003). Functional connectivity in the resting brain: a network analysis of the default mode hypothesis. Proc. Natl. Acad. Sci. U. S. A. 100, 253–258. doi: 10.1073/pnas.0135058100
Grossman, P. (2010). Mindfulness for psychologists: Paying kind attention to the perceptible. Mindfulness 1, 87–97.
Guendelman, S., Medeiros, S., and Rampes, H. (2017). Mindfulness and emotion regulation: Insights from neurobiological, psychological, and clinical studies. Front. Psychol. 8:220. doi: 10.3389/fpsyg.2017.00220
Hahn, B., Ross, T. J., Yang, Y., Kim, I., Huestis, M. A., and Stein, E. A. (2007). Nicotine enhances visuospatial attention by deactivating areas of the resting brain default network. J. Neurosci. 27, 3477–3489. doi: 10.1523/JNEUROSCI.5129-06.2007
Hariri, A. R., Bookheimer, S. Y., and Mazziotta, J. C. (2000). Modulating emotional responses: effects of a neocortical network on the limbic system. NeuroReport 11, 43–48.
Hasenkamp, W., Wilson-Mendenhall, C. D., Duncan, E., and Barsalou, L. W. (2012). Mind wandering and attention during focused meditation: a fine-grained temporal analysis of fluctuating cognitive states. NeuroImage 59, 750–760. doi: 10.1016/j.neuroimage.2011.07.008
Higgins, J. P. T., Altman, D. G., Gøtzsche, P. C., Jüni, P., Moher, D., Oxman, A. D., et al. (2011). The Cochrane Collaboration’s tool for assessing risk of bias in randomised trials. BMJ 343, d5928. doi: 10.1136/bmj.d5928
Hofmann, S. G., Grossman, P., and Hinton, D. E. (2011). Loving-kindness and compassion meditation: potential for psychological interventions. Clin. Psychol. Rev. 31, 1126–1132.
Hofmann, S. G., Sawyer, A. T., Witt, A. A., and Oh, D. (2010). The effect of mindfulness-based therapy on anxiety and depression: a meta-analytic review. J. Consult. Clin. Psychol. 78, 169–183. doi: 10.1037/a0018555
Hölzel, B. K., Carmody, J., Vangel, M., Congleton, C., Yerramsetti, S. M., Gard, T., et al. (2011). Mindfulness practice leads to increases in regional brain gray matter density. Psychiatry Res. 191, 36–43. doi: 10.1016/j.pscychresns.2010.08.006
Hölzel, B. K., Ott, U., Hempel, H., Hackl, A., Wolf, K., Stark, R., et al. (2007). Differential engagement of anterior cingulate and adjacent medial frontal cortex in adept meditators and non-meditators. Neurosci. Lett. 421, 16–21. doi: 10.1016/j.neulet.2007.04.074
Hudson, A. N., Van Dongen, H., and Honn, K. A. (2020). Sleep deprivation, vigilant attention, and brain function: a review. Neuropsychopharmacology 45, 21–30. doi: 10.1038/s41386-019-0432-6
Ives-Deliperi, V. L., Solms, M., and Meintjes, E. M. (2011). The neural substrates of mindfulness: an fMRI investigation. Soc. Neurosci. 6, 231–242.
Jaeger, D. A., Gawehn, N., Schneider, D. T., and Suchan, B. (2021). Phasic and tonic alertness in preterm 5-year-old healthy children. Child Neuropsychol. 27, 1073–1087. doi: 10.1080/09297049.2021.1919297
Japee, S., Holiday, K., Satyshur, M. D., Mukai, I., and Ungerleider, L. G. (2015). A role of right middle frontal gyrus in reorienting of attention: a case study. Front. Syst. Neurosci. 9:23. doi: 10.3389/fnsys.2015.00023
Jha, A. P., Krompinger, J., and Baime, M. J. (2007). Mindfulness training modifies subsystems of attention. Cogn. Affect. Behav. Neurosci. 7, 109–119. doi: 10.3758/cabn.7.2.109
Kabat-Zinn, J. (1982). An out-patient program in behavioral medicine for chronic pain patients based on the practice of mindfulness meditation: theoretical considerations and preliminary results. General Hosp. Psychiatry 4, 33–47. doi: 10.1016/0163-8343(82)90026-3
Kabat-Zinn, J. (1990). Full Catastrophe Living: Using the Wisdom of your Body and Mind to Face Stress, Pain, and Illness. New York, NY: Dell Publishing.
Kabat-Zinn, J. (2013). Full catastrophe living (revised edition): Using the wisdom of your body and mind to face stress, pain, and illness. Atlanta, GA: Bantam.
Kabat-Zinn, J. (2018). Falling Awake: How to practice Mindfulness in Everyday Life. New York, NY: Hachette Books.
Kakumanu, R. J., Nair, A. K., Sasidharan, A., John, J. P., Mehrotra, S., Panth, R., et al. (2019). State-trait influences of Vipassana meditation practice on P3 EEG dynamics. Progress Brain Res. 244, 115–136. doi: 10.1016/bs.pbr.2018.10.027
Katzev, M., and Tu, O. (2013). Revisiting the functional specialization of left inferior frontal gyrus in phonological and semantic fluency: the crucial role of task demands and individual ability. J. Neurosci. 33, 7837–7845. doi: 10.1523/JNEUROSCI.3147-12.2013
Kaul, P., Passafiume, J., Sargent, C. R., and O’Hara, B. F. (2010). Meditation acutely improves psychomotor vigilance, and may decrease sleep need. Behav. Brain Funct. 6:47. doi: 10.1186/1744-9081-6-47
Khantipalo, B. (1984). Calm and insight. In: A Buddhist Manual for Meditators. London: Curzon Press Ltd.
Killgore, W. D. (2010). Effects of sleep deprivation on cognition. Progress Brain Res. 185, 105–129.
Kim, J., Cunnington, R., and Kirby, J. N. (2020). The neurophysiological basis of compassion: An fMRI meta-analysis of compassion and its related neural processes. Neurosci. Biobehav. Rev. 108, 112–123. doi: 10.1016/j.neubiorev.2019.10.023
Klimecki, O. M., Leiberg, S., Lamm, C., and Singer, T. (2013). Functional neural plasticity and associated changes in positive affect after compassion training. Cereb. Cortex 23, 1552–1561. doi: 10.1093/cercor/bhs142
Koenigs, M., and Grafman, J. (2009). The functional neuroanatomy of depression: distinct roles for ventromedial and dorsolateral prefrontal cortex. Behav. Brain Res. 201, 239–243. doi: 10.1016/j.bbr.2009.03.004
Kozhevnikov, M. (2019). Enhancing human cognition through vajrayana practices. J. Religion Health 58, 737–747. doi: 10.1007/s10943-019-00776-z
Kozhevnikov, M., Irene Strasser, A. V., McDougal, E., Dhond, R., and Samuel, G. (2022). Beyond mindfulness: Arousal-driven modulation of attentional control during arousal-based practices. Curr. Res. Neurobiol. 3:100053. doi: 10.1016/j.crneur.2022.100053
Kozhevnikov, M., Louchakova, O., Josipovic, Z., and Motes, M. A. (2009). The enhancement of visuospatial processing efficiency through Buddhist Deity meditation. Psychol. Sci. 20, 645–653. doi: 10.1111/j.1467-9280.2009.02345.x
Kyabgon, T. (2003). Mind at Ease: Self-Liberation through Mahamudra Meditation. Boston, MA: Shambhala.
Laird, A. R., Eickhoff, S. B., Kurth, F., Fox, P. M., Uecker, A. M., Turner, J. A., et al. (2009). ALE meta-analysis workflows via the brainmap database: progress towards a probabilistic functional brain atlas. Front. Neuroinformatics 3:23. doi: 10.3389/neuro.11.023.2009
Laird, A. R., Fox, P. M., Price, C. J., Glahn, D. C., Uecker, A. M., Lancaster, J. L., et al. (2005). ALE meta-analysis: Controlling the false discovery rate and performing statistical contrasts. Hum. Brain Mapp. 25, 155–164. doi: 10.1002/hbm.20136
Lancaster, J., Tordesillas-Gutiérrez, D., Martinez, M., Salinas, F., Evans, A., Zilles, K., et al. (2007). Bias between MNI and Talairach coordinates analyzed using the ICBM-152 brain template. Hum. Brain Mapp. 28, 1194–1205. doi: 10.1002/hbm.20345
Langner, R., and Eickhoff, S. B. (2013). Sustaining attention to simple tasks: A meta-analytic review of the neural mechanisms of vigilant attention. Psychol. Bull. 139, 870–900. doi: 10.1037/a0030694
Lanius, R. A., Vermetten, E., Loewenstein, R. J., Brand, B., Schmahl, C., Bremner, J. D., et al. (2010). Emotion modulation in PTSD: Clinical and neurobiological evidence for a dissociative subtype. Am. J. Psychiatry 167, 640–647.
Lazar, S. W., Bush, G., Gollub, R. L., Fricchione, G. L., Khalsa, G., and Benson, H. (2000). Functional brain mapping of the relaxation response and meditation. NeuroReport 11, 1581–1585.
Lazar, S. W., Kerr, C. E., Wasserman, R. H., Gray, J. R., Greve, D. N., Treadway, M. T., et al. (2005). Meditation experience is associated with increased cortical thickness. NeuroReport 16, 1893–1897. doi: 10.1097/01.wnr.0000186598.66243.19
Lee, T. M., Leung, M. K., Hou, W. K., Tang, J. C., Yin, J., So, K. F., et al. (2012). Distinct neural activity associated with focused-attention meditation and loving-kindness meditation. PLoS One 7:e40054. doi: 10.1371/journal.pone.0040054
Lieberman, M. D., Eisenberger, N. I., Crockett, M. J., Tom, S. M., Pfeifer, J. H., and Way, B. M. (2007). Putting feelings into words: affect labeling disrupts amygdala activity in response to affective stimuli. Psychol. Sci. 18, 421–428. doi: 10.1111/j.1467-9280.2007.01916.x
Lin, Y., Callahan, C. P., and Moser, J. S. (2018). A mind full of self: Self-referential processing as a mechanism underlying the therapeutic effects of mindfulness training on internalizing disorders. Neurosci. Biobehav. Rev. 92, 172–186. doi: 10.1016/j.neubiorev.2018.06.007
Lopez, D. S. Jr. (2009). Buddhism and Science: A Guide for the Perplexed. Chicago, IL: University of Chicago Press.
Lutz, A., Brefczynski-Lewis, J., Johnstone, T., and Davidson, R. J. (2008a). Regulation of the neural circuitry of emotion by compassion meditation: effects of meditative expertise. PLoS One 3:e1897. doi: 10.1371/journal.pone.0001897
Lutz, A., Slagter, H. A., Dunne, J. D., and Davidson, R. J. (2008b). Attention regulation and monitoring in meditation. Trends Cogn. Sci. 12, 163–169. doi: 10.1016/j.tics.2008.01.005
Lutz, A., Dunne, J. D., and Davidson, R. J. (2007). “Meditation and the neuroscience of consciousness: an introduction,” in Cambridge Handbook of Consciousness, eds P. Zelazo, M. Moscovitch, and E. Thompson (Cambridge, MA: Cambridge University Press), 497–550.
Lutz, A., Jha, A. P., Dunne, J. D., and Saron, C. D. (2015). Investigating the phenomenological matrix of mindfulness-related practices from a neurocognitive perspective. Am. Psychol. 70, 632–658. doi: 10.1037/a0039585
Lutz, A., Slagter, H. A., Rawlings, N. B., Francis, A. D., Greischar, L. L., and Davidson, R. J. (2009). Mental training enhances attentional stability: neural and behavioral evidence. J. Neurosci. 29, 13418–13427. doi: 10.1523/JNEUROSCI.1614-09.2009
Lutz, J., Herwig, U., Opialla, S., Hittmeyer, A., Jäncke, L., Rufer, M., et al. (2014). Mindfulness and emotion regulation–an fMRI study. Soc. Cogn. Affect. Neurosci. 9, 776–785. doi: 10.1093/scan/nst043
MacLean, K. A., Ferrer, E., Aichele, S. R., Bridwell, D. A., Zanesco, A. P., Jacobs, T. L., et al. (2010). Intensive meditation training improves perceptual discrimination and sustained attention. Psychol. Sci. 21, 829–839. doi: 10.1177/0956797610371339
Malinowski, P. (2013). Neural mechanisms of attentional control in mindfulness meditation. Front. Neurosci. 7:8. doi: 10.3389/fnins.2013.00008
Manna, A., Raffone, A., Perrucci, M. G., Nardo, D., Ferretti, A., Tartaro, A., et al. (2010). Neural correlates of focused attention and cognitive monitoring in meditation. Brain Res. Bull. 82, 46–56. doi: 10.1016/j.brainresbull.2010.03.001
Maquet, P., Dive, D., Salmon, E., Sadzot, B., Franco, F., Poirrier, R., et al. (1990). Cerebral glucose utilization during sleep-wake cycle in man determined by positron emission tomography and [18F]2-fluoro-2deoxy-D-glucose method. Brain Res. 513, 136–143. doi: 10.1016/0006-8993(90)91099-3
Maquet, P., Péters, J., Aerts, J., Delfiore, G., Degueldre, C., Luxen, A., et al. (1996). Functional neuroanatomy of human rapid-eye-movement sleep and dreaming. Nature 383, 163–166. doi: 10.1038/383163a0
Melnychuk, M. C., Dockree, P. M., O’Connell, R. G., Murphy, P. R., Balsters, J. H., and Robertson, I. H. (2018). Coupling of respiration and attention via the locus coeruleus: Effects of meditation and pranayama. Psychophysiology 55, e13091. doi: 10.1111/psyp.13091
Mishra, P., Panigrahi, M., and Ankit, D. (2020). Cognition and alertness in medical students: Effects of a single night of partial sleep deprivation. Ann. Neurosci. 27, 57–62. doi: 10.1177/0972753120965083
Moher, D., Liberati, A., Tetzlaff, J., and Altman, D. G., and Prisma Group. (2009). Preferred reporting items for systematic reviews and meta-analyses: the PRISMA statement. PLoS Med. 6:e1000097. doi: 10.1371/journal.pmed.1000097
Molenberghs, P., Johnson, H., Henry, J. D., and Mattingley, J. B. (2016). Understanding the minds of others: A neuroimaging meta-analysis. Neurosci. Biobehav. Rev. 65, 276–291. doi: 10.1016/j.neubiorev.2016.03.020
Montero-Marin, J., Garcia-Campayo, J., Pérez-Yus, M. C., Zabaleta-Del-Olmo, E., and Cuijpers, P. (2019). Meditation techniques v. relaxation therapies when treating anxiety: A meta-analytic review. Psychol. Med. 49, 2118–2133. doi: 10.1017/S0033291719001600
Mottaghy, F. M., Willmes, K., Horwitz, B., Müller, H. W., Krause, B. J., and Sturm, W. (2006). Systems level modeling of a neuronal network subserving intrinsic alertness. NeuroImage 29, 225–233. doi: 10.1016/j.neuroimage.2005.07.034
Nagai, Y., Critchley, H. D., Featherstone, E., Trimble, M. R., and Dolan, R. J. (2004). Activity in ventromedial prefrontal cortex covaries with sympathetic skin conductance level: A physiological account of a “default mode” of brain function. NeuroImage 22, 243–251. doi: 10.1016/j.neuroimage.2004.01.019
Nanamoli, B., and Bodhi, B. (1995). Sekha-patipada Sutta (The Practice for One in Training), in The Middle Length Discourses of the Buddha: A New Translation of the Majjhima Nikaya. Boston, MA: Wisdom Publications.
Northoff, G., Heinzel, A., de Greck, M., Bermpohl, F., Dobrowolny, H., and Panksepp, J. (2006). Self-referential processing in our brain—A meta-analysis of imaging studies on the self. NeuroImage 31, 440–457.
O’Connell, R. G., Bellgrove, M. A., Dockree, P. M., Lau, A., Fitzgerald, M., and Robertson, I. H. (2008). Self-Alert Training: volitional modulation of autonomic arousal improves sustained attention. Neuropsychologia 46, 1379–1390. doi: 10.1016/j.neuropsychologia.2007.12.018
O’Connell, R. G., Bellgrove, M. A., Dockree, P. M., and Robertson, I. H. (2006). Cognitive remediation in ADHD: Effects of periodic non-contingent alerts on sustained attention to response. Neuropsychol. Rehabil. 16, 653–665. doi: 10.1080/09602010500200250
Oken, B., Salinsky, M., and Elsas, S. (2006). Vigilance, alertness, or sustained attention: physiological basis and measurement. Clin. Neurophysiol. 117, 1885–1901.
Olbrich, S., Mulert, C., Karch, S., Trenner, M., Leicht, G., Pogarell, O., et al. (2009). EEG-vigilance and BOLD effect during simultaneous EEG/fMRI measurement. NeuroImage 45, 319–332. doi: 10.1016/j.neuroimage.2008.11.014
Opialla, S., Lutz, J., Scherpiet, S., Hittmeyer, A., Jäncke, L., Rufer, M., et al. (2015). Neural circuits of emotion regulation: a comparison of mindfulness-based and cognitive reappraisal strategies. Eur. Arch. Psychiatry Clin. Neurosci. 265, 45–55. doi: 10.1007/s00406-014-0510-z
Pagano, R. R., Rose, R. M., Stivers, R. M., and Warrenburg, S. (1976). Sleep during transcendental meditation. Science 191, 308–310. doi: 10.1126/science.1108200
Pagnoni, G., Cekic, M., and Guo, Y. (2008). “Thinking about not-thinking”: neural correlates of conceptual processing during Zen meditation. PLoS One 3:e3083. doi: 10.1371/journal.pone.0003083
Pandita, S. U. (1992). In This Very Life: The Liberation Teachings of the Buddha. Boston, MA: Wisdom.
Pardo, J. V., Fox, P. T., and Raichle, M. E. (1991). Localization of a human system for sustained attention by positron emission tomography. Nature 349, 61–64. doi: 10.1038/349061a0
Petersen, S. E., and Posner, M. I. (2012). The attention system of the human brain: 20 years after. Annu. Rev. Neurosci. 35, 73–89. doi: 10.1146/annurev-neuro-062111-150525
Picchioni, D., Fukunaga, M., Carr, W. S., Braun, A. R., Balkin, T. J., Duyn, J. H., et al. (2008). fMRI differences between early and late stage-1 sleep. Neurosci. Lett. 441, 81–85. doi: 10.1016/j.neulet.2008.06.010
Pinggal, E., Dockree, P. M., O’Connell, R. G., Bellgrove, M. A., and Andrillon, T. (2022). Pharmacological manipulations of physiological arousal and sleep-like slow waves modulate sustained attention. J. Neurosci. 42, 8113–8124. doi: 10.1523/JNEUROSCI.0836-22.2022
Poldrack, R. A., Wagner, A. D., Prull, M. W., Desmond, J. E., Glover, G. H., and Gabrieli, J. D. (1999). Functional specialization for semantic and phonological processing in the left inferior prefrontal cortex. Neuroimage 10, 15–35. doi: 10.1006/nimg.1999.0441
Posner, M. I. (2017). Attentional Mechanisms. Reference Module in Neuroscience and Biobehavioral Psychology. Amsterdam: Elsevier.
Posner, M. I., and Petersen, S. E. (1990). The attention system of the human brain. Annu. Rev. Neurosci. 13, 25–42.
Pozuelos, J. P., Mead, B. R., Rueda, M. R., and Malinowski, P. (2019). Short-term mindful breath awareness training improves inhibitory control and response monitoring. Progress Brain Res. 244, 137–163. doi: 10.1016/bs.pbr.2018.10.019
Prakash, R. S., Fountain-Zaragoza, S., Kramer, A. F., Samimy, S., and Wegman, J. (2020). Mindfulness and attention: Current state-of-affairs and future considerations. J. Cogn. Enhance. 4, 340–367. doi: 10.1007/s41465-019-00144-5
Price, D. D., Finniss, D. G., and Benedetti, F. (2008). A comprehensive review of the placebo effect: recent advances and current thought. Annu. Rev. Psychol. 59, 565–590. doi: 10.1146/annurev.psych.59.113006.095941
Qin, P., and Northoff, G. (2011). How is our self-related to midline regions and the default-mode network? NeuroImage 57, 1221–1233. doi: 10.1016/j.neuroimage.2011.05.028
Raffone, A., Marzetti, L., Del Gratta, C., Perrucci, M. G., Romani, G. L., and Pizzella, V. (2019). Toward a brain theory of meditation. Progress Brain Res.244, 207–232. doi: 10.1016/bs.pbr.2018.10.028
Raffone, A., and Srinivasan, N. (2010). The exploration of meditation in the neuroscience of meditation and consciousness. Cogn. Process. 11, 1–7.
Raichle, M. E., MacLeod, A. M., Snyder, A. Z., Powers, W. J., Gusnard, D. A., and Shulman, G. L. (2001). A default mode of brain function. Proc. Natl. Acad. Sci. U. S. A. 98, 676–682. doi: 10.1073/pnas.98.2.676
Ritskes, R., Ritskes-Hoitinga, M., Stodkilde-Jorgensen, H., Baerentsen, K., and Hartman, T. (2003). MRI scanning during Zen meditation: the picture of enlightenment? Construct. Hum. Sci. 8, 85–90.
Rolls, E. T., Inoue, K., and Browning, A. (2003). Activity of primate subgenual cingulate cortex neurons is related to sleep. J. Neurophysiol. 90, 134–142.
Sadaghiani, S., Hesselmann, G., and Kleinschmidt, A. (2009). Distributed and antagonistic contributions of ongoing activity fluctuations to auditory stimulus detection. J. Neurosci. 29, 13410–13417. doi: 10.1523/JNEUROSCI.2592-09.2009
Sadaghiani, S., Scheeringa, R., Lehongre, K., Morillon, B., Giraud, A. L., and Kleinschmidt, A. (2010). Intrinsic connectivity networks, alpha oscillations, and tonic alertness: A simultaneous electroencephalography/functional magnetic resonance imaging study. J. Neurosci. 30, 10243–10250. doi: 10.1523/JNEUROSCI.1004-10.2010
Sato, J. R., Kozasa, E. H., Russell, T. A., Radvany, J., Mello, L. E., Lacerda, S. S., et al. (2012). Brain imaging analysis can identify participants under regular mental training. PLoS One 7:e39832. doi: 10.1371/journal.pone.0039832
Sawaya, R., and Ingvar, D. H. (1989). Cerebral blood flow and metabolism in sleep. Acta Neurol. Scand. 80, 481–491.
Scheibner, H. J., Bogler, C., Gleich, T., Haynes, J. D., and Bermpohl, F. (2017). Internal and external attention and the default mode network. NeuroImage, 148, 381–389. doi: 10.1016/j.neuroimage.2017.01.044
Shi, Z., and He, L. (2020). Mindfulness: Attenuating self-referential processing and strengthening other-referential processing. Mindfulness 11, 599–605. doi: 10.1007/s12671-019-01271-y
Short, B. E., Kose, S., Mu, Q., Borckardt, J., Newberg, A., George, M. S., et al. (2010). Regional brain activation during meditation shows time and practice effects: an exploratory fMRI study. Evid. Based Complement. Altern. Med. 7, 121–127. doi: 10.1093/ecam/nem163
Simor, P., van der Wijk, G., Nobili, L., and Peigneux, P. (2020). The microstructure of REM sleep: Why phasic and tonic? Sleep Med. Rev. 52:101305. doi: 10.1016/j.smrv.2020.101305
Slagter, H. A., Lutz, A., Greischar, L. L., Francis, A. D., Nieuwenhuis, S., Davis, J. M., et al. (2007). Mental training affects distribution of limited brain resources. PLoS Biol. 5:e138. doi: 10.1371/journal.pbio.0050138
Stawarczyk, D., Majerus, S., Maj, M., Van der Linden, M., and D’Argembeau, A. (2011). Mind wandering: phenomenology and function as assessed with a novel experience sampling method. Acta Psychol. 136, 370–381. doi: 10.1016/j.actpsy.2011.01.002
Sturm, W., de Simone, A., Krause, B. J., Specht, K., Hesselmann, V., Radermacher, I., et al. (1999). Functional anatomy of intrinsic alertness: Evidence for a fronto-parietal-thalamic-brainstem network in the right hemisphere. Neuropsychologia 37, 797–805. doi: 10.1016/s0028-3932(98)00141-9
Sturm, W., Thimm, M., Küst, J., Karbe, H., and Fink, G. R. (2006). Alertness-training in neglect: behavioral and imaging results. Restorat. Neurol. Neurosci. 24, 371–384.
Sturm, W., and Willmes, K. (2001). On the functional neuroanatomy of intrinsic and phasic alertness. NeuroImage 14, S76–S84.
Sujiva, V. (1991). Loving-Kindness Meditation. Kota Tinggi: Buddha Dharma Education Association Inc.
Sumantry, D., and Stewart, K. E. (2021). Meditation, mindfulness, and attention: A meta-analysis. Mindfulness 12, 1332–1349. doi: 10.1007/s12671-021-01593-w
Tanaka, M., Nakashima, R., Hiromitsu, K., and Imamizu, H. (2021). Individual differences in the change of attentional functions with brief one-time focused attention and open monitoring meditations. Front. Psychol. 12:716138. doi: 10.3389/fpsyg.2021.716138
Tang, Y. Y., Hölzel, B. K., and Posner, M. I. (2015). The neuroscience of mindfulness meditation. Nat. Rev. Neurosci. 16, 213–225. doi: 10.1038/nrn3916
Tang, Y. Y., Ma, Y., Wang, J., Fan, Y., Feng, S., Lu, Q., et al. (2007). Short-term meditation training improves attention and self-regulation. Proc. Natl. Acad. Sci. U. S. A. 104, 17152–17156. doi: 10.1073/pnas.0707678104
Tang, Y. Y., and Posner, M. I. (2009). Attention training and attention state training. Trends Cogn. Sci. 13, 222–227. doi: 10.1016/j.tics.2009.01.009
Tang, Y. Y., Rothbart, M. K., and Posner, M. I. (2012). Neural correlates of establishing, maintaining, and switching brain states. Trends Cogn. Sci. 16, 330–337. doi: 10.1016/j.tics.2012.05.001
Taylor, V. A., Grant, J., Daneault, V., Scavone, G., Breton, E., Roffe-Vidal, S., et al. (2011). Impact of mindfulness on the neural responses to emotional pictures in experienced and beginner meditators. NeuroImage 57, 1524–1533. doi: 10.1016/j.neuroimage.2011.06.001
The Dalai Lama (2001). An open heart: Practicing compassion in everyday life. Boston, MA: Little Brown and Company.
Tomasino, B., and Fabbro, F. (2016). Increases in the right dorsolateral prefrontal cortex and decreases the rostral prefrontal cortex activation after-8 weeks of focused attention-based mindfulness meditation. Brain Cogn. 102, 46–54. doi: 10.1016/j.bandc.2015.12.004
Travis, F., Haaga, D. A., Hagelin, J., Tanner, M., Arenander, A., Nidich, S., et al. (2010). A self-referential default brain state: patterns of coherence, power, and eLORETA sources during eyes-closed rest and Transcendental Meditation practice. Cogn. Process. 11, 21–30. doi: 10.1007/s10339-009-0343-2
Turkeltaub, P. E., Eden, G. F., Jones, K. M., and Zeffiro, T. A. (2002). Meta-analysis of the functional neuroanatomy of single-word reading: method and validation. NeuroImage 16, 765–780. doi: 10.1006/nimg.2002.1131
Turkeltaub, P. E., Eickhoff, S. B., Laird, A. R., Fox, M., Wiener, M., and Fox, P. (2012). Minimizing within-experiment and within-group effects in Activation Likelihood Estimation meta-analyses. Hum. Brain Mapp. 33, 1–13. doi: 10.1002/hbm.21186
Valentine, E., and Sweet, P. (1999). Meditation and attention: a comparison of the effects of concentrative and mindfulness meditation on sustained attention. Mental Health Religion Culture 2, 59–70.
Van Dam, N. T., van Vugt, M. K., Vago, D. R., Schmalzl, L., Saron, C. D., Olendzki, A., et al. (2018). Mind the hype: A critical evaluation and prescriptive agenda for research on mindfulness and meditation. Perspect. Psychol. Sci. 13, 36–61. doi: 10.1177/1745691617709589
Van Noordt, S. J., and Segalowitz, S. J. (2012). Performance monitoring and the medial prefrontal cortex: a review of individual differences and context effects as a window on self-regulation. Front. Hum. Neurosci. 6:197. doi: 10.3389/fnhum.2012.00197
Vogt, B. A. (2005). Pain and emotion interactions in subregions of the cingulate gyrus. Nat. Rev. Neurosci. 6, 533–544.
Walker, J. M., and Berger, R. J. (1980). Sleep as an adaptation for energy conservation functionally related to hibernation and shallow torpor. Adaptive capabilities of the nervous system. Prog. Brain Res. 53, 255–278. doi: 10.1016/S0079-6123(08)60068-0
Wallace, B. A. (2006). The Attention Revolution: Unlocking the Power of the Focused Mind. Boston, MA: Wisdom.
Weissman, D. H., Roberts, K. C., Visscher, K. M., and Woldorff, M. G. (2006). The neural bases of momentary lapses in attention. Nat. Neurosci. 9, 971–978. doi: 10.1038/nn1727
Whitney, P., Hinson, J. M., and Nusbaum, A. T. (2019). A dynamic attentional control framework for understanding sleep deprivation effects on cognition. Prog. Brain Res. 246, 111–126. doi: 10.1016/bs.pbr.2019.03.015
Williams, M., and Kabat-Zinn, J. (2011). Mindfulness: diverse perspectives on its meaning, origins, and multiple applications at the intersection of science and dharma. Contemp. Buddhism 12, 1–18.
Williams, P., and West, M. (1975). EEG responses to photic stimulation in persons experienced at meditation. Electroencephalogr. Clin. Neurophysiol. 39, 519–522.
Younger, J., Adriance, W., and Berger, R. J. (1975). Sleep during transcendental meditation. Perceptual Motor Skills 40, 953–954.
Yu, X., Fumoto, M., Nakatani, Y., Sekiyama, T., Kikuchi, H., Seki, Y., et al. (2011). Activation of the anterior prefrontal cortex and serotonergic system is associated with improvements in mood and EEG changes induced by Zen meditation practice in novices. Int. J. Psychophysiol. 80, 103–111. doi: 10.1016/j.ijpsycho.2011.02.004
Zeidan, F., Grant, J. A., Brown, C. A., McHaffie, J. G., and Coghill, R. C. (2012). Mindfulness meditation-related pain relief: Evidence for unique brain mechanisms in the regulation of pain. Neurosci. Lett. 520, 165–173. doi: 10.1016/j.neulet.2012.03.082
Keywords: alertness, wakefulness, arousing, fMRI, meta-analysis, Buddhist meditation
Citation: Chaudhary IS, Shyi GC-W and Huang S-TT (2023) A systematic review and activation likelihood estimation meta-analysis of fMRI studies on arousing or wake-promoting effects in Buddhist meditation. Front. Psychol. 14:1136983. doi: 10.3389/fpsyg.2023.1136983
Received: 13 February 2023; Accepted: 22 September 2023;
Published: 27 October 2023.
Edited by:
Shahid Bashir, King Fahad Specialist Hospital Dammam, Saudi ArabiaReviewed by:
Turki Abualait, Imam Abdulrahman Bin Faisal University, Saudi ArabiaAndrew Newberg, Thomas Jefferson University, United States
Copyright © 2023 Chaudhary, Shyi and Huang. This is an open-access article distributed under the terms of the Creative Commons Attribution License (CC BY). The use, distribution or reproduction in other forums is permitted, provided the original author(s) and the copyright owner(s) are credited and that the original publication in this journal is cited, in accordance with accepted academic practice. No use, distribution or reproduction is permitted which does not comply with these terms.
*Correspondence: Shih-Tseng Tina Huang, aHVhbmd0aW5hMTEyM0BnbWFpbC5jb20=