- 1Faculty of Psychology, University of Vienna, Vienna, Austria
- 2Institute of Psychology, University of Regensburg, Regensburg, Germany
- 3Faculty of Education and Psychology, Freie Universität Berlin, Berlin, Germany
Introduction
Rhythmic perceptual stimulation, i.e., the presentation of periodic stimuli via sensory input pathways (e.g., auditory or visual; Thut et al., 2011; Calderone et al., 2014) induces resonant brain responses at the presentation frequencies. Since rhythmic perceptual stimulation does not perturbate the neural system beyond its normal operating range (Obleser and Kayser, 2019) and is non-invasive, it has also become a promising tool for neuromodulation in cognitive and developmental neuroscience. In particular, recent work focused on applying rhythmic perceptual stimulation to uncover the functional relevance of neural oscillations at specific frequencies, and their links with cognitive processes in early development, including attention (Christodoulou et al., 2018) and learning (Köster et al., 2019a).
However, an open debate in the adult literature (e.g., Keitel et al., 2014; Haegens, 2020; Meyer et al., 2020; Doelling and Assaneo, 2021; van Bree et al., 2022) emphasizes the need to ascertain whether rhythmic perceptual stimulation directly alters (i.e., stimulates) intrinsic brain oscillations, a phenomenon referred to as entrainment, or rather elicits a series of perceptually evoked potentials independent of endogenous oscillatory activity, referred to as stimulus-tracking (Capilla et al., 2011; Notbohm et al., 2016).
There is a growing body of research with adults showing that perceptual entrainment of endogenous neural oscillations is indeed possible (Herrmann, 2001; Haegens and Zion Golumbic, 2018; Lakatos et al., 2019; Obleser and Kayser, 2019), as demonstrated by its effects on behavioral outcomes such as memory performance (Köster et al., 2019b) and temporal predictions (Daume et al., 2021), and its interplay with individual intrinsic frequencies (Notbohm et al., 2016; Gulbinaite et al., 2019). Yet, conclusive evidence for entrainment is still lacking, especially from developmental populations.
Here, we bring forward the challenges of interpreting results from rhythmic perceptual stimulation studies with infants and children and discuss how insights from the adult literature can help us adequately examine the entrainment hypothesis in developmental research. We argue that neural entrainment is indeed possible in the developing brain, but that further critical evidence is needed to pin down the underlying neural mechanisms. We discuss the implications of distinguishing entrainment from stimulus tracking for the application of rhythmic perceptual stimulation in developmental neuroscience and suggest potential avenues for future research.
Entrainment vs. Stimulus-Tracking
Oscillations can be modified through synchronization with an external periodic stimulus, a phenomenon called entrainment. By definition, during entrainment, endogenous neural oscillations align with the temporal structure of the exogenous stimulus (Thut et al., 2011; Obleser and Kayser, 2019). It has been proposed that entrainment may facilitate information sampling and sensory selection, underlying various cognitive and perceptual processes (Lakatos, 2008).
Stimulus-tracking refers to the occurrence of frequency-following brain responses to a rhythmic stimulus that, unlike entrainment, show no direct interference with ongoing internal oscillations and related cognitive and perceptual processes (Keitel et al., 2014, 2019; Haegens, 2020). The main difference between the two phenomena is that during stimulus-tracking, a rhythmic external stimulus elicits a frequency following neural sensory response, but ongoing internal brain rhythms are not perturbated. Whereas during entrainment, the stimulus “hijacks” the ongoing internal rhythms at the stimulation frequency, which then become altered and align with the external stimulus (Figure 1).
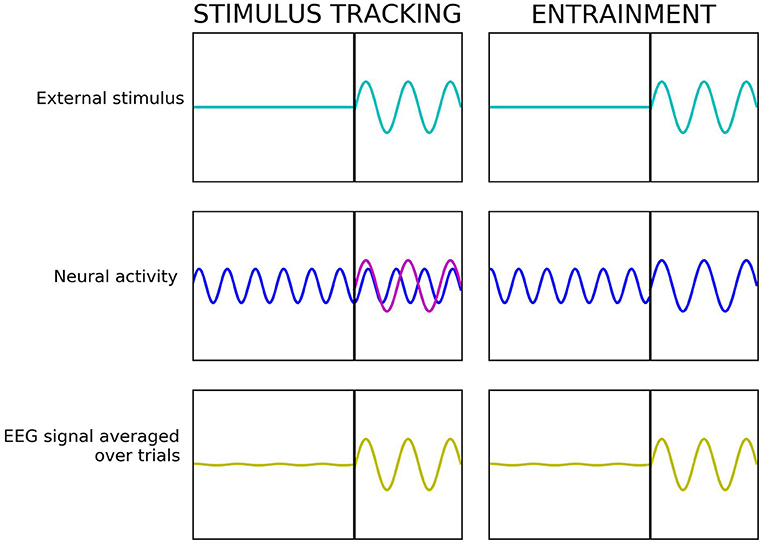
Figure 1. Stimulus-tracking vs. entrainment—simplified example. In all the panels, the x-axis reflects the time and the y-axis—the amplitude of the signal. In stimulus-tracking (left), rhythmic external stimulation (light blue) causes a frequency-following neural sensory response (magenta), but the internal rhythms (dark blue) are not perturbated. In entrainment (right), rhythmic external stimulation (light blue) causes a resonant response at the frequency of the external stimulus by “hijacking” ongoing internal rhythms (dark blue). In both cases, the resulting electroencephalogram (EEG) signal (yellow) looks the same.
The phenomenon of stimulus-tracking forms the basis of frequency-tagging, a method commonly applied in visual perception research: tagging multiple, simultaneously presented stimuli with different frequencies elicits steady-state responses (SSRs), thus the visual processing of each element can be assessed separately (Müller et al., 2003). Importantly, frequency-tagging considers SSRs independent from the preferred oscillatory frequency of the involved sensory networks (Keitel et al., 2014).
Analytically distinguishing between entrainment and stimulus-tracking solely based on brain activity during rhythmic perceptual stimulation is not possible, as both are characterized by the synchronization between an exogenous stimulus and neural rhythms (Thut et al., 2011; Obleser and Kayser, 2019; Haegens, 2020). However, differentiating between the two phenomena is crucial for studies aiming to cause neuromodulation effects or to test hypotheses about the functionality of endogenous brain rhythms through applying the method of rhythmic perceptual stimulation. There are also implications for paradigms that rely on this methodology to assess attention and perception processes without a specific focus on the underlying oscillatory activity.
Some have postulated that for entrainment to be differentiated from phase-locked responses, it would require that endogenous neural oscillations decouple from the external stimulus and outlive it, particularly in the narrow frequency band in which these oscillations operate (Capilla et al., 2011; Haegens and Zion Golumbic, 2018), but it is not a universally accepted criterion (see e.g., Doelling and Assaneo, 2021). Nonetheless, alterations of endogenous oscillations would likely manifest on the behavioral level and thus could be experimentally tested (Lakatos, 2008).
In the adult literature, there have been two significant ways in particular to establish entrainment: by the alteration of behavioral performance, and by the alignment/matching between the stimulation frequency and the individual internal oscillations of participants. In the following, we discuss both methods and outline associated challenges as well as future directions for entrainment research with infants and children.
Behavioral Evidence for Entrainment
Endogenous brain rhythms at different frequency bands have differing functional correlates, e.g., the theta band has been linked to memory processes (Klimesch, 1999; Friese et al., 2013), while the alpha band has been associated with attentional processes (Klimesch, 2012). If rhythmic perceptual stimulation is able to entrain these endogenous brain rhythms, then we should be able to observe distinct behavioral effects of stimulation at external frequencies corresponding to different EEG frequency bands (Herrmann et al., 2016).
Good examples of this line of research supporting this notion are studies examining memory-enhancing effects of rhythmic sensory stimulation at the theta frequency, which have accumulated in the recent years (see Hanslmayr et al., 2019 for a review). For example, it has been shown that presenting audiovisual and visual stimuli at the theta, but not the alpha frequency improves later memory of these stimuli (Clouter et al., 2017; Köster et al., 2019b). What is important, this effect is dependent on the phase synchrony between the external stimulus and the brain response (Clouter et al., 2017; Wang et al., 2018), as well as the power of the response (Köster et al., 2019b), which further suggests that the behavioral effects of rhythmic perceptual stimulation are caused by neural entrainment. Interestingly, the behavioral effects of entrainment of the theta rhythm seem to persist beyond the period of stimulation, as Roberts et al. (2018) found improved source memory in participants exposed to rhythmic sensory stimulation (content-wise irrelevant to the memorized stimuli) between learning and test phase. To conclude, research with adults provides accumulating evidence for the modulation of cognitive processes through rhythmic perceptual stimulation.
Entrainment at Individual Stimulation Frequencies
It has been argued that an important feature of entrainment is that it should occur for external rhythms close to the neural oscillator's intrinsic rate (so-called “eigenfrequency”; Obleser and Kayser, 2019; Haegens, 2020). Indeed, Notbohm et al. (2016) demonstrated that rhythmic visual flicker at frequencies closer to participants' intrinsic frequencies of the alpha rhythm caused a more pronounced phase coupling between the external rhythm and the neural response. Moreover, a number of studies have found that the behavioral effects (i.e., performance impairment) of stimulation in the alpha band on participants' attention are predicted by how close the stimulation frequencies are to participants' endogenous alpha peaks (de Graaf et al., 2013; Gulbinaite et al., 2017). Finally, Köster et al. (2019b) successfully entrained memory functions by stimulating participants' brain activity at their individual theta vs. alpha frequencies.
However, more studies examining other frequency bands and an improved understanding of the generators behind the intrinsic brain rhythms are needed. Ideally, future studies would also examine the behavioral effects of individually optimized vs. not individually optimized stimulation frequencies within the same frequency bands. In developmental populations, EEG frequency ranges change with age by moving toward higher frequencies (Marshall et al., 2002), thus we would also expect individual frequencies to shift toward the higher ends of the spectra with age. Observing behavioral effects of rhythmic sensory stimulation at frequencies optimized (vs. non-optimized) to specific age groups would support the notion of entrainment effects.
Entrainment in Developmental Research
Previous studies with infants and children applied rhythmic auditory and/or visual stimulation as a tool to assess attention (e.g., Robertson et al., 2012; Köster et al., 2017; Christodoulou et al., 2018), face perception (e.g., de Heering and Rossion, 2015; Peykarjou et al., 2017), speech perception and language learning (e.g., Telkemeyer et al., 2009; Attaheri et al., 2022), and related developmental disorders such as dyslexia (e.g., Colling et al., 2017), as well as musical rhythm perception (e.g., Cirelli et al., 2016). In these studies, stimulation frequencies were chosen either arbitrarily, to index brain responses to stimuli of interest (e.g., a face appearing at 1.2 Hz among a stream of images updated at 6 Hz in the study by de Heering and Rossion, 2015), or were motivated by hypotheses about naturally occurring frequencies in infants' environment (e.g., sounds with spectral properties resembling naturalistic speech in the study by Telkemeyer et al., 2009). Another approach aimed to capture infants' stimulus-tracking by reconstructing features of natural stimuli based on brain responses or by predicting brain activity from stimulus features (e.g., Jessen et al., 2021). However, very few developmental studies to date applied rhythmic visual stimulation with the explicit goal to entrain endogenous rhythms (e.g., Köster et al., 2019a), an approach that can help to uncover the functionality of brain oscillations in early cognitive and perception processes.
Recent debates in the developmental literature challenged the interpretation of findings from rhythmic perceptual stimulation studies since the underlying mechanisms of entrainment are still not well-understood. As an example, Köster et al. (2019a) used this method to assess the functional role of infants' theta (4 Hz) and alpha (6 Hz) oscillations in the cognitive processing of unexpected events. Findings revealed that infants' visually entrained theta, but not alpha oscillations sharply increased for unexpected vs. expected events, in line with evidence on the critical role of theta oscillations in early learning (Begus and Bonawitz, 2020; Köster et al., 2020). However, in a commentary, using simulated data, Keitel et al. (2021) argued that the results from Köster et al. (2019a) could potentially be explained by a stimulus-tracking account: unexpected events could enhance the negative central (Nc) component of the event related potential (ERP) (Kayhan et al., 2019), leading to a difference in ERPs between conditions without the involvement of functionally relevant oscillations elicited by the stimulus. Although the simulated data did not closely reflect the observed data (see Köster et al., 2021b), this debate highlights the need to differentiate between entrainment and stimulus-tracking when interpreting data from rhythmic perceptual stimulation studies.
Avenues for Future Research
Here, we argued that further evidence is needed on how perceptually entrained rhythms interact with ongoing oscillatory dynamics in the developing brain, so that a comprehensive theoretical and analytical framework could be established for developmental entrainment research. Studies in the field need to investigate the effects of rhythmic perceptual stimulation by including behavioral outcome measures, and by using individually optimized stimulation frequencies. However, commonly applied paradigms in adult entrainment research need to be adjusted for developmental studies (Wass et al., 2022).
Measuring altered behavior of infants following rhythmic perceptual stimulation—though challenging—can be achieved by subsequent memory paradigms that assess learning and memory performance via preferential looking (Begus et al., 2015), imitation (Köster et al., 2021a), or habituation and dishabituation (Choi et al., 2020). In case of older children, behavioral outcomes of entrainment such as attention, learning and memory effects can be tested by incorporating subsequent playful tasks into the experimental design. Observed behavioral effects linked to sensory stimulation would provide causal evidence for neural oscillatory entrainment. Applying stimulation at individualized frequencies could be another non-invasive way to ascertain entrainment in developmental samples, by testing the alignment of endogenous oscillations following sensory stimulation with infants' or children's individual vs. other frequencies. The two approaches can be combined to contrast behavioral changes following entrainment to individualized vs. other frequencies.
Taken together, we need a deeper understanding of the effects of rhythmic perceptual stimulation on endogenous oscillatory activity to establish if entrainment occurs in the developing brain, and if so, whether it can be considered as a tool for neuromodulation to test the functional aspects of specific neural rhythms in early cognitive development.
Author Contributions
ABá and ABr wrote the manuscript. SH and MK contributed to revising and editing the manuscript. All authors contributed to the article and approved the submitted version.
Funding
This work was supported by an FWF grant awarded to SH (Grant number: P 33853) and a D-A-CH research grant awarded to MK and SH by the DFG and FWF jointly (Grant numbers: KO 6028/1-1; I 4332). We are grateful for the open access funding provided by the University of Vienna.
Conflict of Interest
The authors declare that the research was conducted in the absence of any commercial or financial relationships that could be construed as a potential conflict of interest.
Publisher's Note
All claims expressed in this article are solely those of the authors and do not necessarily represent those of their affiliated organizations, or those of the publisher, the editors and the reviewers. Any product that may be evaluated in this article, or claim that may be made by its manufacturer, is not guaranteed or endorsed by the publisher.
References
Attaheri, A., Choisdealbha, Á. N., Di Liberto, G. M., Rocha, S., Brusini, P., Mead, N., et al. (2022). Delta- and theta-band cortical tracking and phase-amplitude coupling to sung speech by infants. NeuroImage 247, 118698. doi: 10.1016/j.neuroimage.2021.118698
Begus, K., and Bonawitz, E. (2020). The rhythm of learning: theta oscillations as an index of active learning in infancy. Dev. Cogn. Neurosci. 45, 100810. doi: 10.1016/j.dcn.2020.100810
Begus, K., Southgate, V., and Gliga, T. (2015). Neural mechanisms of infant learning: differences in frontal theta activity during object exploration modulate subsequent object recognition. Biol. Lett. 11, 20150041. doi: 10.1098/rsbl.2015.0041
Calderone, D. J., Lakatos, P., Butler, P. D., and Castellanos, F. X. (2014). Entrainment of neural oscillations as a modifiable substrate of attention. Trends Cogn. Sci. 18, 300–309. doi: 10.1016/j.tics.2014.02.005
Capilla, A., Pazo-Alvarez, P., Darriba, A., Campo, P., and Gross, J. (2011). Steady-state visual evoked potentials can be explained by temporal superposition of transient event-related responses. PLoS ONE 6, e14543. doi: 10.1371/journal.pone.0014543
Choi, D., Batterink, L., Black, A. K., Paller, K., and Werker, J. F. (2020). Preverbal infants discover statistical word patterns at similar rates as adults: evidence from neural entrainment. Psychol. Sci. 31, 1161–1173. doi: 10.1177/0956797620933237
Christodoulou, J., Leland, D. S., and Moore, D. S. (2018). Overt and covert attention in infants revealed using steady-state visually evoked potentials. Dev. Psychol. 54, 803–815. doi: 10.1037/dev0000486
Cirelli, L. K., Spinelli, C., Nozaradan, S., and Trainor, L. J. (2016). Measuring neural entrainment to beat and meter in infants: effects of music background. Front. Neurosci. 10, 229. doi: 10.3389/fnins.2016.00229
Clouter, A., Shapiro, K. L., and Hanslmayr, S. (2017). Theta phase synchronization is the glue that binds human associative memory. Curr. Biol. 27, 3143–3148. doi: 10.1016/j.cub.2017.09.001
Colling, L. J., Noble, H. L., and Goswami, U. (2017). Neural entrainment and sensorimotor synchronization to the beat in children with developmental dyslexia: an EEG study. Front. Neurosci. 11, 360. doi: 10.3389/fnins.2017.00360
Daume, J., Wang, P., Maye, A., Zhang, D., and Engel, A. K. (2021). Non-rhythmic temporal prediction involves phase resets of low-frequency delta oscillations. NeuroImage 224, 117376. doi: 10.1016/j.neuroimage.2020.117376
de Graaf, T. A., Gross, J., Paterson, G., Rusch, T., Sack, A. T., and Thut, G. (2013). Alpha-band rhythms in visual task performance: phase-locking by rhythmic sensory stimulation. PLoS ONE 8, e60035. doi: 10.1371/journal.pone.0060035
de Heering, A., and Rossion, B. (2015). Rapid categorization of natural face images in the infant right hemisphere. Elife 4, e06564. doi: 10.7554/eLife.06564
Doelling, K. B., and Assaneo, M. F. (2021). Neural oscillations are a start toward understanding brain activity rather than the end. PLoS Biol. 19, e3001234. doi: 10.1371/journal.pbio.3001234
Friese, U., Köster, M., Hassler, U., Martens, U., Trujillo-Barreto, N., and Gruber, T. (2013). Successful memory encoding is associated with increased cross-frequency coupling between frontal theta and posterior gamma oscillations in human scalp-recorded EEG. NeuroImage. 66, 642–647. doi: 10.1016/j.neuroimage.2012.11.002
Gulbinaite, R., Roozendaal, D. H. M., and VanRullen, R. (2019). Attention differentially modulates the amplitude of resonance frequencies in the visual cortex. NeuroImage 203, 116146. doi: 10.1016/j.neuroimage.2019.116146
Gulbinaite, R., van Viegen, T., Wieling, M., Cohen, M. X., and VanRullen, R. (2017). Individual alpha peak frequency predicts 10 Hz flicker effects on selective attention. J. Neurosci. 37, 10173–10184. doi: 10.1523/JNEUROSCI.1163-17.2017
Haegens, S. (2020). Entrainment revisited: a commentary on Meyer, Sun, and Martin (2020). Lang. Cogn. Neurosci. 35, 1119–1123. doi: 10.1080/23273798.2020.1758335
Haegens, S., and Zion Golumbic, E. (2018). Rhythmic facilitation of sensory processing: a critical review. Neurosci. Biobehav. Rev. 86, 150–165. doi: 10.1016/j.neubiorev.2017.12.002
Hanslmayr, S., Axmacher, N., and Inman, C. S. (2019). Modulating human memory via entrainment of brain oscillations. Trends Neurosci. 42, 485–499. doi: 10.1016/j.tins.2019.04.004
Herrmann, C. S. (2001). Human EEG responses to 1-100 Hz flicker: resonance phenomena in visual cortex and their potential correlation to cognitive phenomena. Exp. Brain Res. 137, 346–353. doi: 10.1007/s002210100682
Herrmann, C. S., Strüber, D., Helfrich, R. F., and Engel, A. K. (2016). EEG oscillations: from correlation to causality. Int. J. Psychophysiol. 103, 12–21. doi: 10.1016/j.ijpsycho.2015.02.003
Jessen, S., Obleser, J., and Tune, S. (2021). Neural tracking in infants – An analytical tool for multisensory social processing in development. Dev. Cogn. Neurosci. 52, 101034. doi: 10.1016/j.dcn.2021.101034
Kayhan, E., Meyer, M., O'Reilly, J. X., Hunnius, S., and Bekkering, H. (2019). Nine-month-old infants update their predictive models of a changing environment. Dev. Cogn. Neurosci. 38, 100680. doi: 10.1016/j.dcn.2019.100680
Keitel, C., Keitel, A., Benwell, C. S. Y., Daube, C., Thut, G., and Gross, J. (2019). Stimulus-driven brain rhythms within the alpha band: the attentional-modulation conundrum. J. Neurosci. 39, 3119–3129. doi: 10.1523/JNEUROSCI.1633-18.2019
Keitel, C., Obleser, J., Jessen, S., and Henry, M. J. (2021). Frequency-specific effects in infant electroencephalograms do not require entrained neural oscillations: a commentary on Köster et al. (2019). Psychol. Sci. 32, 966–971. doi: 10.1177/09567976211001317
Keitel, C., Quigley, C., and Ruhnau, P. (2014). Stimulus-driven brain oscillations in the alpha range: Entrainment of intrinsic rhythms or frequency-following response? J. Neurosci. 34, 10137–10140. doi: 10.1523/JNEUROSCI.1904-14.2014
Klimesch, W. (1999). EEG alpha and theta oscillations reflect cognitive and memory performance: a review and analysis. Brain Res. Rev. 29, 169–195. doi: 10.1016/S0165-0173(98)00056-3
Klimesch, W. (2012). Alpha-band oscillations, attention, and controlled access to stored information. Trends Cogn. Sci. 16, 606–617. doi: 10.1016/j.tics.2012.10.007
Köster, M., Castel, J., Gruber, T., and Kärtner, J. (2017). Visual cortical networks align with behavioral measures of context-sensitivity in early childhood. NeuroImage 163, 413–418. doi: 10.1016/j.neuroimage.2017.08.008
Köster, M., Kayhan, E., Langeloh, M., and Hoehl, S. (2020). Making Sense of the World: Infant Learning from a Predictive Processing Perspective. Perspect. Psychol. Sci. 15, 562–571. doi: 10.1177/1745691619895071
Köster, M., Langeloh, M., and Hoehl, S. (2019a). Visually entrained theta oscillations increase for unexpected events in the infant brain. Psychol. Sci. 30, 1656–1663. doi: 10.1177/0956797619876260
Köster, M., Langeloh, M., Kliesch, C., Kanngiesser, P., and Hoehl, S. (2021a). The value of subsequent memory paradigms in uncovering neural mechanisms of early social learning. NeuroImage 234, 117978. doi: 10.1016/j.neuroimage.2021.117978
Köster, M., Langeloh, M., Michel, C., and Hoehl, S. (2021b). Young infants process prediction errors at the theta rhythm. NeuroImage 236, 118074. doi: 10.1016/j.neuroimage.2021.118074
Köster, M., Martens, U., and Gruber, T. (2019b). Memory entrainment by visually evoked theta-gamma coupling. NeuroImage 188, 181–187. doi: 10.1016/j.neuroimage.2018.12.002
Lakatos, P. (2008). Entrainment of neuronal oscillations as a mechanism of attentional selection. Science 320, 5872. doi: 10.1126/science.1154735
Lakatos, P., Gross, J., and Thut, G. (2019). A new unifying account of the roles of neuronal entrainment. Curr. Biol. 29, 890–905. doi: 10.1016/j.cub.2019.07.075
Marshall, P. J., Bar-Haim, Y., and Fox, N. A. (2002). Development of the EEG from 5 months to 4 years of age. Clin. Neurophysiol. 113, 1199–1208. doi: 10.1016/S1388-2457(02)00163-3
Meyer, L., Sun, Y., and Martin, A. E. (2020). Synchronous, but not entrained: exogenous and endogenous cortical rhythms of speech and language processing. Lang. Cogn. Neurosci. 35, 1089–1099. doi: 10.1080/23273798.2019.1693050
Müller, M. M., Malinowski, P., Gruber, T., and Hillyard, S. A. (2003). Sustained division of the attentional spotlight. Nature. 424, 309–312. doi: 10.1038/nature01812
Notbohm, A., Kurths, J., and Herrmann, C. S. (2016). Modification of brain oscillations via rhythmic light stimulation provides evidence for entrainment but not for superposition of event-related responses. Front. Hum. Neurosci. 10, 10. doi: 10.3389/fnhum.2016.00010
Obleser, J., and Kayser, C. (2019). Neural entrainment and attentional selection in the listening brain. Trends Cogn. Sci. 23, 913–926. doi: 10.1016/j.tics.2019.08.004
Peykarjou, S., Hoehl, S., Pauen, S., and Rossion, B. (2017). Rapid categorization of human and ape faces in 9-month-old infants revealed by fast periodic visual stimulation. Sci. Rep. 7, 12526. doi: 10.1038/s41598-017-12760-2
Roberts, B. M., Clarke, A., Addante, R. J., and Ranganath, C. (2018). Entrainment enhances theta oscillations and improves episodic memory. Cogn. Neurosci. 9, 181–193. doi: 10.1080/17588928.2018.1521386
Robertson, S. S., Watamura, S. E., and Wilbourn, M. P. (2012). Attentional dynamics of infant visual foraging. Proc. Natl. Acad. Sci. U. S. A. 109, 11460. doi: 10.1073/pnas.1203482109
Telkemeyer, S., Rossi, S., Koch, S. P., Nierhaus, T., Steinbrink, J., Poeppel, D., et al. (2009). Sensitivity of newborn auditory cortex to the temporal structure of sounds. J. Neurosci. 29, 14726–14733. doi: 10.1523/JNEUROSCI.1246-09.2009
Thut, G., Schyns, P. G., and Gross, J. (2011). Entrainment of perceptually relevant brain oscillations by non-invasive rhythmic stimulation of the human brain. Front. Psychol. 2, 170. doi: 10.3389/fpsyg.2011.00170
van Bree, S., Alamia, A., and Zoefel, B. (2022). Oscillation or not—Why we can and need to know (commentary on Doelling and Assaneo, 2021). Eur. J. Neurosci. 55, 201–204. doi: 10.1111/ejn.15542
Wang, D., Clouter, A., Chen, Q., Shapiro, K. L., and Hanslmayr, S. (2018). Single-trial phase entrainment of theta oscillations in sensory regions predicts human associative memory performance. J. Neurosci. 38, 6299–6309. doi: 10.1523/JNEUROSCI.0349-18.2018
Keywords: neural entrainment, stimulus-tracking, rhythmic perceptual stimulation, steady-state response (SSR), frequency-tagging, cognitive development
Citation: Bánki A, Brzozowska A, Hoehl S and Köster M (2022) Neural Entrainment vs. Stimulus-Tracking: A Conceptual Challenge for Rhythmic Perceptual Stimulation in Developmental Neuroscience. Front. Psychol. 13:878984. doi: 10.3389/fpsyg.2022.878984
Received: 18 February 2022; Accepted: 11 April 2022;
Published: 06 May 2022.
Edited by:
Elena Nava, University of Milano-Bicocca, ItalyReviewed by:
Kaili Clackson, University of Cambridge, United KingdomCopyright © 2022 Bánki, Brzozowska, Hoehl and Köster. This is an open-access article distributed under the terms of the Creative Commons Attribution License (CC BY). The use, distribution or reproduction in other forums is permitted, provided the original author(s) and the copyright owner(s) are credited and that the original publication in this journal is cited, in accordance with accepted academic practice. No use, distribution or reproduction is permitted which does not comply with these terms.
*Correspondence: Anna Bánki, YW5uYS5iYW5raSYjeDAwMDQwO3VuaXZpZS5hYy5hdA==