- 1Centre for Language Studies, Radboud University Nijmegen, Nijmegen, Netherlands
- 2International Max Planck Research School for the Language Sciences, Nijmegen, Netherlands
- 3Department of Linguistics, Faculty of Human Sciences, Macquarie University, Sydney, NSW, Australia
- 4Max Planck Institute for Psycholinguistics, Nijmegen, Netherlands
- 5Donders Institute for Brain, Cognition and Behaviour, Radboud University Nijmegen, Nijmegen, Netherlands
Rhyme perception is an important predictor for future literacy. Assessing rhyme abilities, however, commonly requires children to make explicit rhyme judgements on single words. Here we explored whether infants already implicitly process rhymes in natural rhyming contexts (child songs) and whether this response correlates with later vocabulary size. In a passive listening ERP study, 10.5 month-old Dutch infants were exposed to rhyming and non-rhyming child songs. Two types of rhyme effects were analysed: (1) ERPs elicited by the first rhyme occurring in each song (rhyme sensitivity) and (2) ERPs elicited by rhymes repeating after the first rhyme in each song (rhyme repetition). Only for the latter a tentative negativity for rhymes from 0 to 200 ms after the onset of the rhyme word was found. This rhyme repetition effect correlated with productive vocabulary at 18 months-old, but not with any other vocabulary measure (perception at 10.5 or 18 months-old). While awaiting future replication, the study indicates precursors of phonological awareness already during infancy and with ecologically valid linguistic stimuli.
Introduction
Being able to judge whether words rhyme is one of the earliest forms of phonological awareness children develop (Ziegler and Goswami, 2005). Rhyme awareness reflects children’s metalinguistic ability to separate the initial phoneme of a syllable from the rest and is usually assessed with a rhyme judgement task, in which children decide whether a set of words rhymes (e.g., king/ring) or not (e.g., king/pear). Measured from around 4 years of age (Bryant and Bradley, 1978; Bradley and Bryant, 1983), rhyme awareness, among other phonological awareness measures, serves as a standard predictor for future literacy (Wood and Terrell, 1998; Melby-Lervåg et al., 2012).
Rhymes already play a role in the developing lexicons of much younger children. Many frequent words in children’s input rhyme (e.g., hit, bit, pit) (De Cara and Goswami, 2002), presumably resulting in the first words in infants lexicons to also rhyme, rather than to share phonemes at the word onset (e.g., pin/bin vs. pin/pit, respectively) (Zamuner, 2009). It has been suggested that the necessity to differentiate and recognise such similar-sounding words causes children’s initially holistic lexical representations to be re-structured into segmental-level specifications (Metsala and Walley, 1998; Fikkert and Levelt, 2008). Consequently, a growing lexicon might lead to an increase in phonological awareness (Metsala and Walley, 1998; Carroll et al., 2003) and this relationship is probably reciprocal: infants’ developing phonological sensitivity also enables a further growth in the number of lexical representations (Curtin and Zamuner, 2014). In fact, there is a well-established association between pre-school phonological awareness and vocabulary size (e.g., Lonigan et al., 2000; Stadler et al., 2007), where phonological awareness is mainly assessed with explicit rhyme judgement tasks and has been attributed to a need to differentiate words with offset-overlap in growing lexicons (Metsala and Walley, 1998; De Cara and Goswami, 2002, 2003).
Despite the evidence regarding the role of rhyming words in infants’ lexicons, previous studies have been equivocal concerning infants’ ability to recognise rhymes. This is mainly due to differences in test procedures and stimuli: while infants respond to a change from one single-word rhyme pattern to another in the Conditioned-Headturn-Procedure (Hayes et al., 2000, 2009), 9-month-olds may only display spontaneous differentiation between rhyming and non-rhyming child songs (Hahn et al., 2018), but not between rhyming and non-rhyming word lists (Jusczyk et al., 1999). The tentative evidence in favour of infants’ rhyme detection in Hayes et al. (2000, 2009) and Hahn et al. (2018), suggests that infants’ rhyme detection might be subtle. An implicit paradigm, like passive-listening EEG, might therefore be more sensitive to infants’ emerging rhyme abilities (Kooijman et al., 2008).
The results by Hahn et al. (2018) suggest that infants are able to recognise recurring rhymes in their natural linguistic context. Caregivers rhyme and sing for their infants on a daily basis (Ilari, 2005) and it has been suggested that at-home musical and language play contributes to vocabulary growth (Franco et al., 2021) and emergent literacy (Politimou et al., 2019; Krijnen et al., 2020). Specifically, toddlers’ experience with nursery rhymes was associated with several phonological abilities in a number of studies (Bryant and Bradley, 1978; Bradley and Bryant, 1983; Dunst et al., 2011) and caregiver singing during infancy positively influences later vocabulary size (Franco et al., 2021). Potentially, the acoustic shape of songs creates a perceptual boost for infants, due to rhymes in songs being placed at a salient phrase-final position and in a predictable rhythmic context (Kotz and Schwartze, 2010). In the current study, we employ a passive-listening EEG paradigm to answer the following research questions: (1) Do infants process rhyming songs differently from non-rhyming songs? and (2) Is the ability to detect recurring rhymes in songs related to later vocabulary?
The ERP literature on rhyme processing contains a range of effects, including the classic N450 rhyme effect, a negativity for non-rhymes at posterior electrodes elicited during explicit rhyme judgement tasks (Rugg, 1984a,b). There is no consensus whether pre-literate children already show this effect (Wagensveld et al., 2013; Andersson et al., 2018), but one study observing the N450 at this young age found that it correlated with phonological awareness (Andersson et al., 2018). An anterior negativity for rhyming pseudo-words has been reported for 4-year-olds in the absence of a rhyme task (Andersson et al., 2018), suggesting that the anterior negativity reflects implicit automatic rhyme processing. Note, however, that pre-schoolers executing rhyme judgements also displayed an early anterior negativity, which reduced in amplitude with increased letter knowledge (Wagensveld et al., 2013). In the present study, we expect an early negativity for rhymes, most likely at anterior electrode sites, as the infants will not be executing a task and still have limited phonological awareness.
The infant (EEG) research tradition has not yet assessed infants’ rhyme abilities, but laid the foundation by providing evidence for infants’ ability to detect repeated phonemes and words in speech and associating this detection with vocabulary size (see Cristia et al., 2014 for review). Specifically, the ERP word familiarity effect usually occurs between 200 and 500 ms after word onset as a left anterior negativity for the familiar word, which becomes more negative with each repetition. The effect occurs in response to several repetitions (e.g., Kooijman et al., 2005), but also after a single repetition of the same word (e.g., Kidd et al., 2018) in continuous speech, and is influenced by stimulus features and task difficulty (Mills et al., 2004; Junge et al., 2012; Snijders et al., 2020). For example, Snijders et al. (2020) observed a positive word familiarity effect for words occurring in child songs. The word familiarity effect has been established as a reflection of infants’ ability to recognise repeating words in speech (see e.g., Teixidó et al., 2018 for review). Individual differences in the polarity of the ERP word familiarity effect are associated with vocabulary size (Kooijman et al., 2013; Junge and Cutler, 2014; Kidd et al., 2018): Infants with more negative word familiarity effects tend to have larger vocabularies.
The current study builds on the discussed EEG research on rhyme processing with adults and children in combination with the infant word familiarity effect to ask whether infants detect rhymes in songs and whether individual differences in this ability are related to infant vocabularies. We specifically aim to extend the word familiarity effect to another phonological unit: rhymes. We presented 10.5-month-old Dutch infants with child songs of 10 phrases long from Hahn et al. (2018) in a rhyming and non-rhyming version, which only differed in the final pseudo-word at the end of every phrase (e.g., paf, taf, kaf vs. teet, deus, bag).
Two types of effect will be investigated: rhyme sensitivity and rhyme repetition. Rhyme sensitivity will be measured at the first point of diversion between rhyming and non-rhyming songs, i.e., at the end of the second phrase where the rhyme is repeated for the first time in rhyming songs but not repeated in non-rhyming songs. Measuring rhyme sensitivity corresponds to earlier studies where critical words (Junge et al., 2012; Kidd et al., 2018) or rhymes (Andersson et al., 2018) were repeated only once. The rhyme repetition effect will be measured as the averaged response to rhymes occurring at the end of the 3rd through 10th phrase of the songs. Measuring responses to repeated rhymes is comparable to the ERP word familiarity response to words repeated across successive sentences (Kooijman et al., 2005; Junge et al., 2012). For both effects, we expect a left anterior negativity for rhymes, which might be more pronounced in the rhyme repetition effect due to repetition enhancement (Nordt et al., 2016). The onset of the ERP effects is probably slightly later than the 200 ms reported in earlier word familiarity studies (Teixidó et al., 2018), due to the beginning of the phonological overlap being shifted to after the onset phoneme. Finally, both ERP effects will be correlated with Dutch CDI scores (Zink and Lejaegere, 2002) for productive and receptive vocabulary at 10.5 and 18 months, to investigate a possible link between rhyme perception and vocabulary size.
Methods
This study was approved by the local ethics committee and parents of participating infants gave informed consent prior to data collection in accordance with the Declaration of Helsinki.
Participants
In total 40 10.5-month-old infants from monolingual Dutch households were tested, from which 12 infants were excluded from data analysis due to not contributing at least 30 trials each for rhyming and non-rhyming songs (5 infants) or due to having more than two neighbouring noisy channels (7 infants). Twenty-eight datasets were used for the rhyme repetition effect (see section “Statistical analysis” below): mean age: 320 days, range: 304–338, 13 girls. From this subsample, 18 infants contributed enough trials to be analysed for the rhyme sensitivity effect (see section “Statistical analysis” section below). Sample size was determined prior to data collection based on similar studies by Kooijman et al. (2005, 2013) and Junge et al. (2012, 2014).
Stimuli
Song stimuli were taken from Hahn et al. (2018) and comprised of nine novel songs that were unknown to the infants. An example stimulus is depicted in Figure 1. See the original publication for more detail on creation and acoustic characteristics of the songs and a link to the song stimuli.
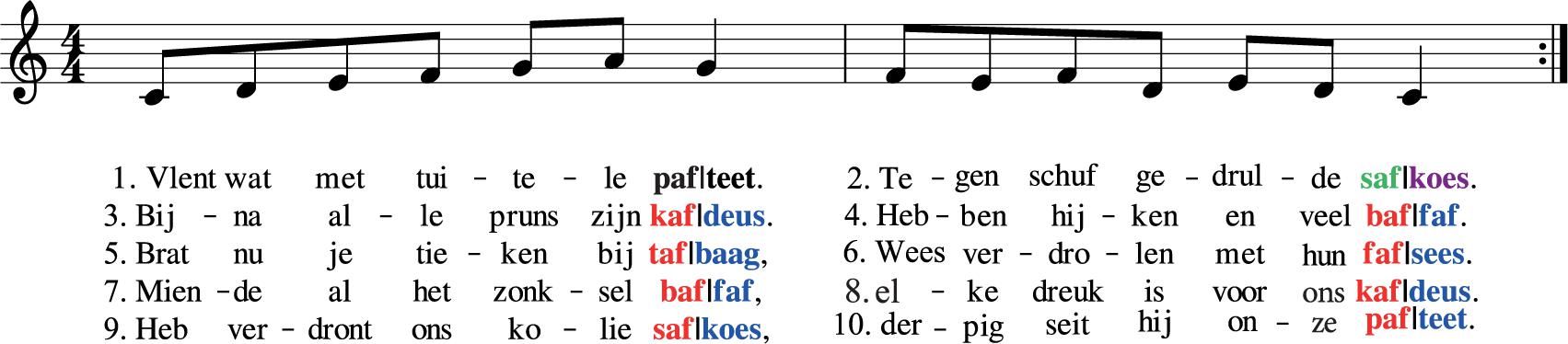
Figure 1. Example Stimulus. Song with lyrics in non-sense Dutch. Critical pseudo-words used to assess the rhyme sensitivity effect at the end of phrase 2 of the songs highlighted in green (rhyme) and purple (non-rhyme); Critical pseudo-words to assess the rhyme repetition effect from phrase 3 to 10 of the songs are highlighted in red (rhyme) and blue (non-rhyme). Stimuli were taken from Hahn et al. (2018). For the analysis, the first phrase-final pseudo-word of every song (in bold) was disregarded, as this cannot rhyme.
Each song occurred in two versions (rhyming and non-rhyming) and consisted of ten phrases (five verses consisting of two phrases each). Song lyrics were in non-sense Dutch to control for word familiarity: content words were replaced with legal Dutch pseudo-words, while function words were regular Dutch function words. Critical words at the end of every phrase comprised of CVC pseudo-words. Rimes of CVC pseudo-words were of medium frequency and all occurred as word ends in the Dutch Celex (Baayen et al., 1995), while the full CVC pseudo-words (including the initial consonant) did not appear in Celex or had a very low frequency (<100 raw). The full songs were on average 33 s long (range rhyming: 28–36 s, range non-rhyming: 28–35 s), including 500 ms between consecutive phrases of every song. The ten single phrases of each song were on average 2.7 s long (range rhyming: 2.2–3.3 s, range non-rhyming: 2.2–3.2 s). Critical pseudo-words were on average 690 ms long (ranges rhyming: 511–1022 ms, range non-rhyming: 489–908 ms). The rhyming and non-rhyming version of a song were identical apart from the final pseudo-word of every phrase (critical pseudo-word). These either rhymed (e.g., paf, taf, kaf) for the rhyming version of a song, or did not rhyme (e.g., teet, deus, bag) for the non-rhyming version.
Procedure
Each test session was run by two experimenters. One experimenter briefed the parents and ran the measurement, while the other entertained the infant during placement of the electrode cap and data collection. The entire testing procedure from arrival to farewell lasted approximately 1 h. During the test session, the infant sat on a caregiver’s lab in an electrically shielded room. Silent baby-friendly movie clips were played at a PC screen in front of the infant. One experimenter sat next to the caregiver to silently entertain the infant during the measurement if necessary. Both caregiver and experimenter listened to masking music over headphones throughout data collection.
Stimulus presentation was controlled by Presentation software (Neurobehavioral Systems, Inc., Berkeley, CA1). The nine song stimuli (ten phrases each) in their two versions (rhyming/non-rhyming) were randomised across two experimental blocks. Songs of the same condition (rhyming/non-rhyming) never occurred more than twice in a row. Data collection lasted around 11 min.
Parents filled in questionnaires on their musical and demographic background and the vocabulary of their child (N-CDI 1 at 10.5 and N-CDI 2B at 18 months of age). So far, only the vocabulary questionnaires have been analysed.
EEG activity was collected from 32 Ag/AgCl electrodes (ActiCAP) using BrainAmp DC and BrainVision Recorder Software (Brain Products GmbH, Germany). Electrode locations were in accordance with an extended 10/20 system: F7/3/4/8, FC5/1/2/6, C3/4, CP5/1/2/6, P7/3/4/8, Fz, FCz, Cz, CPz, Pz, POz for collection of EEG activity. Electro-oculogram (EOG) was recorded using an electrode on the left or right cheek and above the eye (Fp1/2) for vertical EOG, and left and right of the eyes (FT9/10) for horizontal EOG. AFz served as Ground, FCz as online reference. Impedance were typically kept below 25 kΩ. Data was collected with a sampling rate of 500 Hz using an online low-cut off filter of 10 s and high-cut off of 1000 Hz.
Data Preprocessing
EEG data were analysed in MATLAB (The MathWorks, Natick, MA, United States) using the Fieldtrip toolbox (Oostenveld et al., 2011). Data was filtered offline at 0.1–30 Hz. For one infant, a 0.5 Hz high-pass filter was used, due to slow drifts during the measurement. Bad channels were manually removed, as were data segments with flat channels or large artefacts (>150 μV for EEG channels, >250 μV for EOG channels). Eye- and single-electrode noise components were identified using Independent Component Analysis (Makeig et al., 1996) as implemented in the EEGlab toolbox (Delorme and Makeig, 2004) with infomax ICA (Bell and Sejnowski, 1995) on 1-s data snippets.
Critical pseudo-words were the final pseudo-words from phrase 2 to 10 from every song, resulting in 162 possible trials in total (see Figure 1 for an example, the rhyming (green/red) and non-rhyming (purple/blue) pseudo-words are the critical pseudo-words). Raw data was epoched from −200 to 900 ms around critical pseudo-word onset, using 0.1–30 Hz filters (0.5 Hz high-pass filter for one infant), and the eye- and noise-components identified with ICA were removed from the data (average of two eye and three noise components per infant). Critical pseudo-word-epochs were re-referenced to linked-mastoids. For three infants a single mastoid electrode was used as a reference, due to the other reference electrode being noisy. Time-locked data was baseline corrected by normalising waveforms relative to the 200 ms epoch preceding the onset of the critical pseudo-word. Trials containing activity exceeding ±150 μV were removed, leading to exclusion of five infants who did not contribute a minimum of 30 of the 81 possible trials for rhyming and non-rhyming songs. Nine channels were not analysed due to being noisy in too many datasets: F7/F8, F3/F2, Fz, T7/8, P7/8. Further noisy/missing channels were repaired using spline interpolation and a custom neighbourhood structure (a total of 17 channels repaired in 16 infants). Seven infants were excluded from further data analysis due to having more than two neighbouring noisy channels, making channel repair unreliable. Event-related potentials were computed for the remaining 13 channels (FC5/6, FCz, C3/4, Cz, CP5/6, CP1/2, P3/4, Pz) by averaging the rhyming and non-rhyming trials.
Statistical Analysis
Two ERP effects were investigated: (1) the rhyme sensitivity effect, only on ERPs from phrase 2 of the songs, the moment where rhyming and non-rhyming songs first differed (green/purple in example Figure 1) (minimum of five trials per condition for this analysis, Mean (SD) number of trials rhyming: 7 (1.09), and non-rhyming trials: 7 (1.47), n = 18 infants) and (2) the rhyme repetition effect, averaged over ERPs from phrase 3 to 10 of every song (red/blue in example Figure 1, n = 28 infants, Mean (SD) number of trials rhyming: 48 (11.43), and non-rhyming trials: 48 (10.39)). Non-parametric cluster-based permutation tests (Maris and Oostenveld, 2007) were used to evaluate differences in the ERPs between the rhyming and non-rhyming conditions. For these tests, first dependent-samples t-tests are calculated to compare rhyming and non-rhyming conditions (for all 13 remaining electrodes and all time-points between 0 and 900 ms after onset of the critical pseudo-word). Then, clusters are made of neighbouring electrodes and time-points that exceed a threshold alpha of 0.05 (uncorrected). A cluster-level statistic (sum of t-statistics in the cluster) is then computed and, using Monte-Carlo resampling (1000 permutations), a reference distribution is made for random data, to which the observed cluster-statistic is compared to get a Monte Carlo p-value. This effectively controls for multiple comparisons while taking the electrophysiological properties of EEG into account (Maris and Oostenveld, 2007; Luck and Gaspelin, 2017; Sassenhagen and Draschkow, 2019).
From the N-CDI questionnaire for each infant the following scores were derived: comprehension at 10.5 months of age and production and comprehension at 18 months of age. To adhere to previous research (e.g., Kidd et al., 2018), non-parametric Kendall’s Tau rank correlations were calculated to investigate the relationship between ERPs (mean of cluster electrodes and cluster time-points of identified clusters in cluster-based permutation test comparing rhyming and non-rhyming conditions) and the vocabulary scores (not normally distributed).
Results
Rhyme Sensitivity Effect
In response to the first occurrence of the rhyme/non-rhyme (phrase 2 of each song), rhyming pseudo-words induced a more positive ERP waveform compared to non-rhyming pseudo-words (Figure 2A). This difference was not significant (lowest cluster p = 0.6).
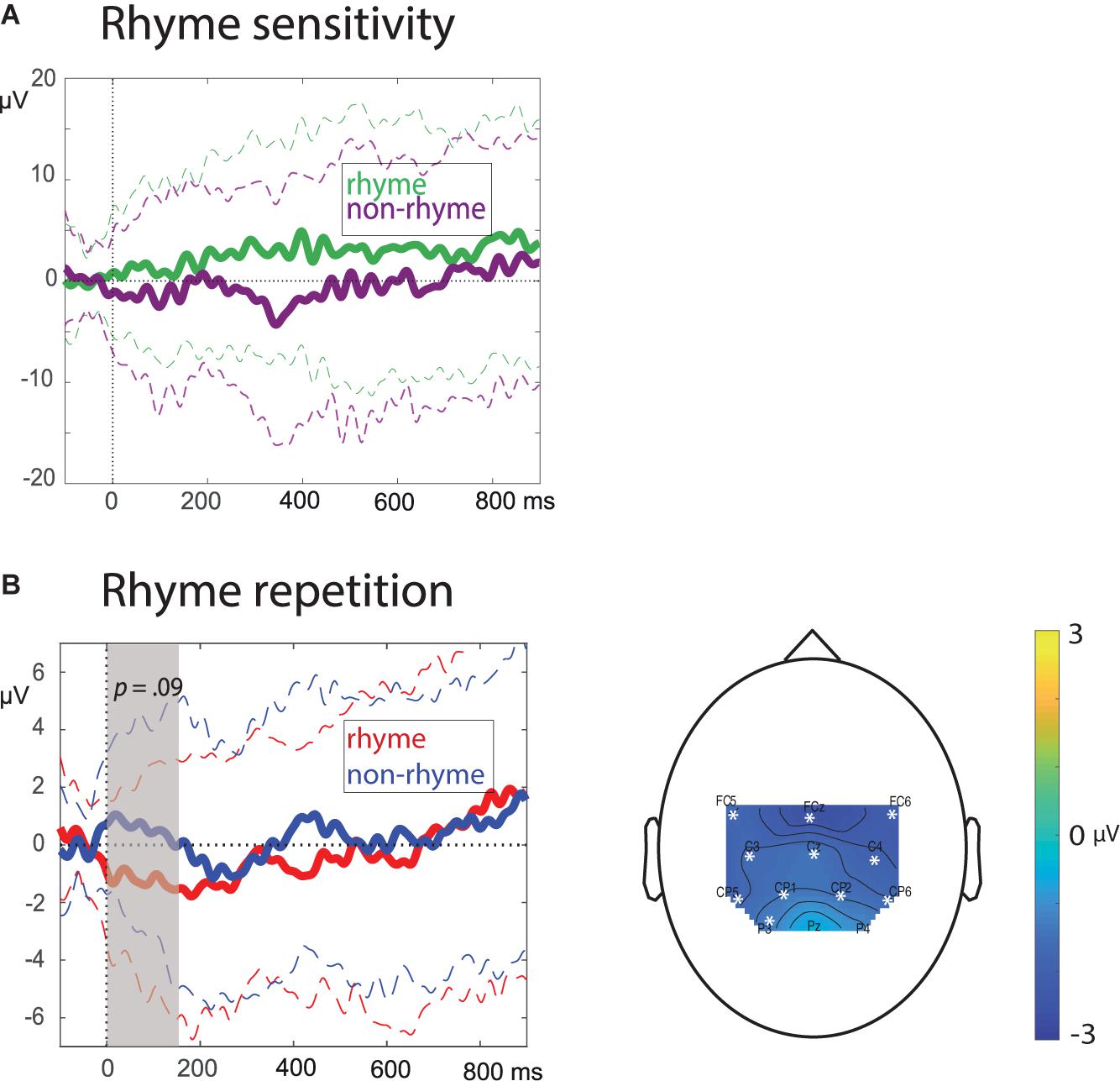
Figure 2. Rhyme sensitivity effect (based on the final pseudo-word of phrase 2 of every song, n = 18 infants) and Rhyme repetition effect (based on the final pseudo-words of phrase 3–10 of the songs, n = 28 infants). Solid lines = Means; dotted lines = ±1 SD; (A): ERPs, averaged over all 13 electrodes; (B) left: ERPs averaged over the electrodes in the largest identified cluster (0–178 ms, p = 0.09), right: topographic isovoltage maps of the difference between rhyme and non-rhyme within the rhyme repetition effect cluster time window (0–178 ms) for all tested electrodes, cluster electrodes are marked with white star.
Rhyme Repetition Effect
ERPs for rhyming pseudo-words occurring at the end of phrase 3–10 of the songs were more negative than ERPs for non-rhyming pseudo-words within the first 200 ms after pseudo-word onset (Figure 2B). None of the identified clusters in the cluster-randomisation test of the 0–900 ms time-window survived multiple comparisons correction (lowest cluster p = 0.09). The cluster with the lowest p-value ranged from 0 to 178 ms and contained all electrodes except for Pz and P4: Mean (SD) rhyme: −1.27 (2.55), Mean (SD) non-rhyme = 0.48 (2.57).
Individual differences in the rhyme repetition effect within the largest identified cluster (mean of all cluster electrodes within the 0–178 ms time window) were significantly correlated with productive vocabulary at 18 months (τ = -0.3, p = 0.03). Infants with a larger negative ERP difference (rhyme more negative than non-rhyme) produced more words at 18 months old (Figure 3). There were no correlations with comprehension at 10.5 or 18 months.
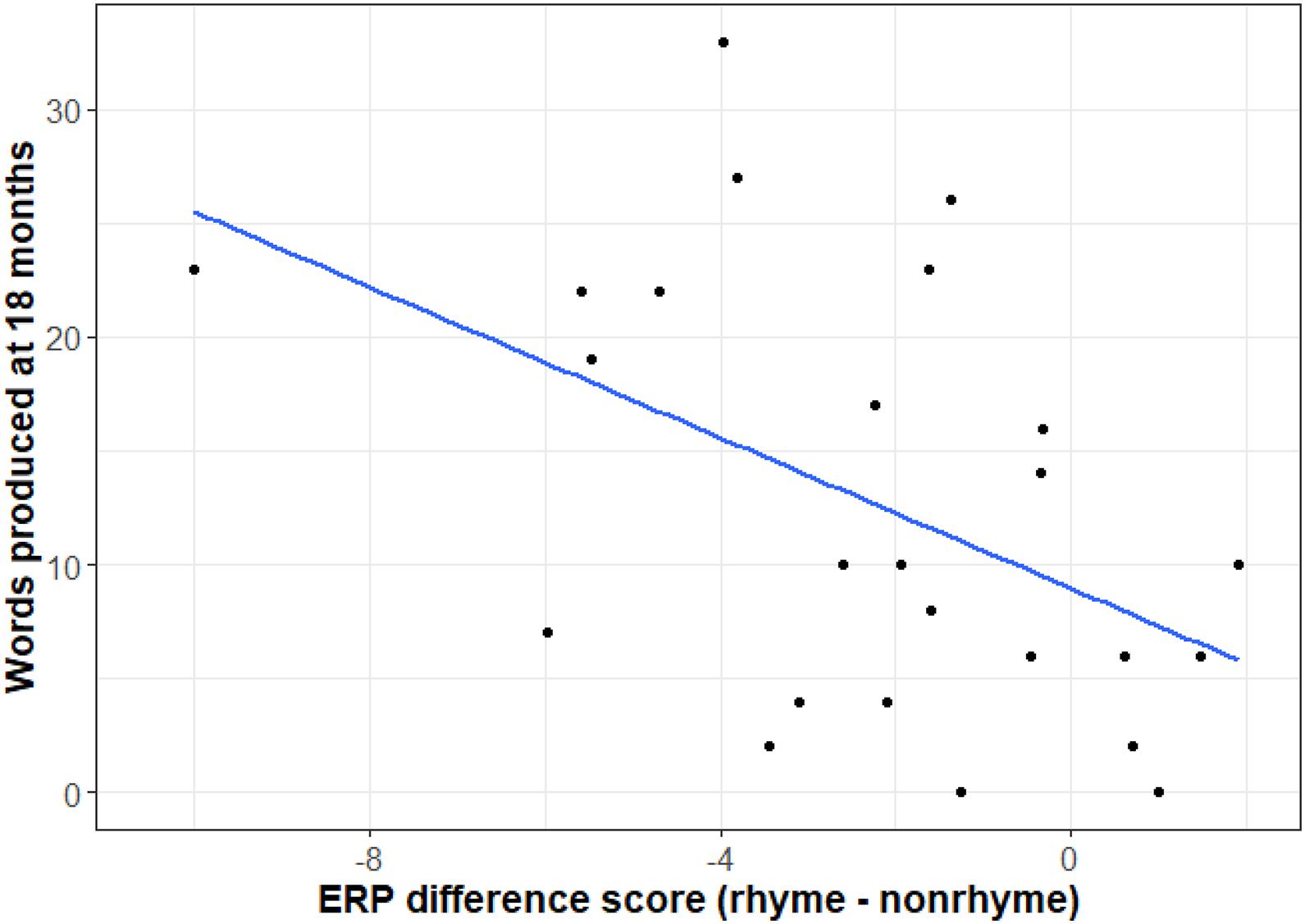
Figure 3. Correlation between words produced at 18 months (y-axis) and ERP difference scores (rhyme – non-rhyme, averaged for the cluster electrodes and time window) on the x-axis: τ = -0.3, p = 0.03. The correlation remains marginally significant when excluding the outlier at (−10, 23): τ = -0.3, p = 0.07.
Discussion
Explicit phonological awareness during preschool years is an important predictor for literacy (Wood and Terrell, 1998; Melby-Lervåg et al., 2012). Potentially, infants’ perception of rhymes during informal language play contributes to emerging phonological awareness skills and vocabulary (Politimou et al., 2019; Krijnen et al., 2020; Franco et al., 2021). Previous studies were equivocal concerning infants’ rhyme abilities (Jusczyk et al., 1999; Hayes et al., 2000, 2009; Hahn et al., 2018), presumably due to behavioural paradigms concealing infants’ subtle processing abilities (Kooijman et al., 2008). The current study employed a passive listening EEG paradigm with 10.5-month-old Dutch infants to explore whether infants differentiate rhyming from non-rhyming pseudo-words in child songs in the absence of an explicit behavioural task. Infants’ response to the first rhymes occurring in each song (at the end of the second phrase, rhyme sensitivity effect) did not render significant results. For rhymes repeated throughout the song (at the end of phrase 3–10, rhyme repetition effect) an early negative effect for rhymes was found that approached conventional significance levels and correlated with productive vocabularies at 18 months.
For the rhyme sensitivity effect there was a numeric indication for rhyming pseudo-words to elicit a sustained positivity (Figure 2A), but the effect did not survive multiple comparison correction. The inconclusive results might be attributable to a lack of statistical power, as data from only 18 infants was available for this analysis, with each infant providing on average only seven trials per condition.
Repeated rhymes occurring at the end of phrase three to ten of the songs elicited a central negativity within the first 200 ms after pseudo-word onset, which was marginally significant when corrected for multiple comparisons. Consequently, this effect delivers tentative evidence for an implicit neural rhyme response in infants. The early negative effect for rhyming pseudo-words is similar to the early negative rhyme effect that was identified by Wagensveld et al. (2013) for single words in pre-literate 5-year-olds, but not 7-year-olds. Later negative effects for rhyming words have also been identified in 3–5 year-olds (Andersson et al., 2018). For complete words repeated in continuous speech, both negative and positive word familiarity effects have been found (see Teixidó et al., 2018). A previous study that used child songs, reported a positive word familiarity effect (Snijders et al., 2020). The opposite polarity in the current study might be attributable to rhymes occurring consistently at the end of the song phrases. The fixed rhyme position might have lessened working memory load in comparison to Snijders et al. (2020), where critical words were occurring at various phrase positions (see Snijders et al., 2020 for more background on polarity differences in the word familiarity effect). In terms of polarity, our negative rhyme effect rather adheres to other studies with recurring spoken single words and rhymes (Wagensveld et al., 2013; Andersson et al., 2018; Teixidó et al., 2018). The rhyme repetition effect observed here occurred immediately after pseudo-word onset, which is different from the word familiarity effect which usually occurs between 200 and 500 ms after word onset (Teixidó et al., 2018), but again similar to the early negative rhyme effect in 5-year-olds identified by Wagensveld et al. (2013). The latency and polarity of our effect have to be interpreted with caution, due to the effect being the result of an average over the 3rd through 10th phrase of the songs. Future studies, with more trials available per phrase of the songs, should investigate whether the effect changes (gradually) in latency and polarity upon every rhyme repetition (Kooijman et al., 2013; Nordt et al., 2016).
Infants with more negative rhyme repetition effects at 10.5 months had larger productive vocabularies at 18 months. This is the first study to report a relationship between infants’ vocabularies and their perception of repeating rhymes in songs. This finding extends previous studies, which established such relationship based on the detection of phonemes and words in fluent speech (see Cristia et al., 2014 for a review).
The functional relevance of rhyme sensitivity for infant development requires further research. Infants might experience no communicative pressure to utilise their implicit knowledge about the syllabic units of onsets and rhymes, due to their small lexicons not yet containing many rhyming words (Johnson, 2016). Rhymes in songs, however, are placed within a particularly intriguing stimulus that is highly ritualised, repetitive, rich in structural cues and progressing at a rather slow pace (Trehub et al., 1993, 1997; Trainor, 1996; Longhi, 2009; Falk and Kello, 2017). The acoustic context of language play might provide infants with a chance to recognise the syllabic structure of rhyming words, while this might be much more difficult in ordinary speech. So far, there is mounting evidence for a relationship between processing and production of spoken nursery rhymes and literacy and phonological awareness skills in pre-schoolers (see Dunst et al., 2011 for review). Based on the current study, songs and nursery rhymes might have an impact on phonological processing and vocabulary already during infancy (see also Franco et al., 2021).
The tentative relationship between implicit rhyme processing and vocabulary observed in the current study requires future replication. Only productive vocabulary, and not word comprehension, was related to early rhyme abilities. This might be due to a more reliable parental estimate of productive vocabulary. Another tentative explanation would be the use of prediction in production (van Alphen et al., 2021), which might also have impacted processing of our predictable rhyming stimuli.
The limited number of trials, electrodes and infants in our sample makes future replication and extension of the study necessary. Future research should also settle to what extent the rhyme effect reported here differs from the ERP word familiarity effect. Both effects are elicited by repeating phonological material in infants’ input and could thus stem from the same underlying auditory processing mechanism. One possible interpretation is that the rhyme effect we identify in the current study is just a word familiarity effect that appears early due to the predictability of the appearance of the rhymes at phrase ends. Solving this issue is impeded by the exclusion of anterior electrodes in the current study, the standard location of measuring the word familiarity effect. An alternative interpretation would be that the effects differ, and possibly depend on the perceived lexicality of the repeated stimuli. The ERP word familiarity effect has mainly been reported for existing words, while the rhyme negativity in pre-schoolers can be elicited by existing as well as pseudo-words (Andersson et al., 2018). Additionally, the ERP word familiarity effect arises from repetition of full words, while the rhyme negativity is based on repetition of syllable nucleus and coda only, and not other kinds of phonological overlap (Wagensveld et al., 2013). Whether infants in the current study recognise the change in syllable onsets between successive rhyming pseudo-words (e.g., paf, taf, kaf) or rather consider them repetitions of the same pseudo-word and ignore onset differences (e.g., paf, paf, paf) is another question that remains for future research (see also Ngon et al., 2013).
The current study complements and extends previous behavioural results (Hahn et al., 2018) with an ERP response for 10.5-month-old infants’ early implicit rhyme detection in a natural rhyming stimulus and a relationship of this ERP response with productive vocabulary at 18 months of age. Implicit rhyme detection in language play might contribute to the development of explicit phonological awareness abilities and vocabulary (Krijnen et al., 2020; Franco et al., 2021). The current early evidence of phonological awareness in infancy might thus reflect the origin of a key predictor of reading achievement (Wood and Terrell, 1998; Ziegler and Goswami, 2005; Melby-Lervåg et al., 2012).
Data Availability Statement
The raw data supporting the conclusions of this article will be made available by the authors, without undue reservation.
Ethics Statement
The studies involving human participants were reviewed and approved by the Ethical Board of the Faculty of Social Sciences, Radboud University Nijmegen, CMO2012/012. Written informed consent to participate in this study was provided by the participants’ legal guardian/next of kin.
Author Contributions
LH and TS conceived and programmed the experiment and analysed the data. LH collected the data, made the figures, and wrote the first draft of the manuscript. All authors reviewed and edited the manuscript.
Conflict of Interest
The authors declare that the research was conducted in the absence of any commercial or financial relationships that could be construed as a potential conflict of interest.
Publisher’s Note
All claims expressed in this article are solely those of the authors and do not necessarily represent those of their affiliated organizations, or those of the publisher, the editors and the reviewers. Any product that may be evaluated in this article, or claim that may be made by its manufacturer, is not guaranteed or endorsed by the publisher.
Acknowledgments
We thank all participating families for contributing their time and effort to our data collection. In addition, we thank Katharina Menn and Iris Schmits for their exceptionally professional and motivating support during study preparation, scheduling and data collection. Clementine Pizzey-Grey and Anna Bolhuis are gratefully acknowledged for their help during data collection and Jessica Askamp and Angela Khadar for their support with general lab procedures and scheduling.
Footnotes
References
Andersson, A., Sanders, L. D., Coch, D., Karns, C. M., and Neville, H. J. (2018). Anterior and posterior erp rhyming effects in 3- to 5-year-old children. Dev. Cogn. Neurosci. 30, 178–190. doi: 10.1016/j.dcn.2018.02.011
Baayen, H., Piepenbrock, R., and Gulikers, L. (1995). The CELEX Lexical Database. Linguistic Data Consortium. Philadelphia, PA: University of Pennsylvania.
Bell, A. J., and Sejnowski, T. J. (1995). An information-maximisation approach to blind separation and blind deconvolution. Neural Comput. 7, 1129–1159. doi: 10.1162/neco.1995.7.6.1129
Bradley, L., and Bryant, P. (1983). Categorizing sounds and learning to read. Nature 301, 491–521. doi: 10.1038/301419a0
Bryant, P., and Bradley, L. (1978). Difficulties in auditory organisation as a possible cause of reading backwardness. Nature 271, 746–747. doi: 10.1038/271746a0
Carroll, J. M., Snowling, M. J., Stevenson, J., and Hulme, C. (2003). The development of phonological awareness in preschool children. Dev. Psychol. 39, 913–923. doi: 10.1037/0012-1649.39.5.913
Cristia, A., Seidl, A., Junge, C., Soderstrom, M., and Hagoort, P. (2014). Predicting individual variation in language from infant speech perception measures. Child Dev. 85, 1330–1345. doi: 10.1111/cdev.12193
Curtin, S., and Zamuner, T. S. (2014). Understanding the developing sound system: interactions between sounds and words. Wiley Interdiscip. Rev. Cogn. Sci. 5, 589–602. doi: 10.1002/wcs.1307
De Cara, B., and Goswami, U. (2002). Similarity relations among spoken words: the special status of rimes in English. Behav. Res. Methods Instrum. Comput. 34, 416–423. doi: 10.3758/BF03195470
De Cara, B., and Goswami, U. (2003). Phonological neighbourhood density: effects in a rhyme awareness task in five-year-old children. J. Child Lang. 30, 695–710. doi: 10.1017/S0305000903005725
Delorme, A., and Makeig, S. (2004). EEGLAB: an open source toolbox for analysis of single-trial EEG dynamics. J. Neurosci. Methods 13, 9–21. doi: 10.1016/j.jneumeth.2003.10.009
Dunst, C., Meter, D., and Hamby, D. (2011). Relationship between young children’s nursery rhyme experiences and knowledge and phonological and print-related abilities. Center Early Lit. Learn. Rev. 4, 1–12.
Falk, S., and Kello, C. T. (2017). Hierarchical organization in the temporal structure of infant-direct speech and song. Cognition 163, 80–86. doi: 10.1016/j.cognition.2017.02.017
Fikkert, P., and Levelt, C. (2008). “How does place fall into place? The lexicon and emergent constraints in children’s developing phonological grammar,” in Contrast in Phonology: Theory, Perception, Acquisition, eds P. Avery, B. E. Dresher, and K. Rice (Berlin: Mouton), 231–268.
Franco, F., Suttora, C., Spinelli, M., Kozar, I., and Fasolo, M. (2021). Singing to infants matters: early singing interactions affect musical preferences and facilitate vocabulary building. J. Child Lang. 48, 1–26. doi: 10.1017/S0305000921000167
Hahn, L. E., Benders, T., Snijders, T. M., and Fikkert, P. (2018). Infants’ sensitivity to rhyme in songs. Infant Behav. Dev. 52, 130–139. doi: 10.1016/j.infbeh.2018.07.002
Hayes, R. A., Slater, A. M., and Longmore, C. A. (2009). Rhyming abilities in 9-month-olds: the role of the vowel and coda explored. Cogn. Dev. 24, 106–112. doi: 10.1016/j.cogdev.2008.11.002
Hayes, R. A., Slater, A., and Brown, E. (2000). Infants’ ability to categorise on the basis of rhyme. Cogn. Dev. 15, 405–419. doi: 10.1016/s0885-2014(01)00036-3
Ilari, B. (2005). On musical parenting of young children: musical beliefs and behaviors of mothers and infants. Early Child Dev. Care 175, 647–660. doi: 10.1080/0300443042000302573
Johnson, E. K. (2016). Constructing a proto-lexicon: an integrative view of infant language development. Annu. Rev. Linguist. 2, 391–412. doi: 10.1146/annurev-linguistics-011415-040616
Junge, C., and Cutler, A. (2014). Early word recognition and later language skills. Brain Sci. 4, 532–559. doi: 10.3390/brainsci4040532
Junge, C., Cutler, A., and Hagoort, P. (2014). Successful word recognition by 10-month-olds given continuous speech both at initial exposure and test. Infancy 19, 179–193. doi: 10.1111/infa.12040
Junge, C., Kooijman, V. M., Hagoort, P., and Cutler, A. (2012). Rapid recognition at 10 months as a predictor of language development. Dev. Sci. 15, 463–473. doi: 10.1111/j.1467-7687.2012.1144.x
Jusczyk, P. W., Goodman, M., and Baumann, A. (1999). Nine-month-olds’ attention to sound similarities in syllables. J. Mem. Lang. 82, 62–82. doi: 10.1006/jmla.1998.2605
Kidd, E., Junge, C., Spokes, T., Morrison, L., and Cutler, A. (2018). Individual differences in infant speech segmentation: achieving the lexical shift. Infancy 23, 770–794. doi: 10.1111/infa.12256
Kooijman, V. M., Hagoort, P., and Cutler, A. (2005). Electrophysiological evidence for prelinguistic infants’ word recognition in continuous speech. Cogn. Brain Res. 24, 109–116. doi: 10.1016/j.cogbrainres.2004.12.009
Kooijman, V. M., Johnson, E. K., and Cutler, A. (2008). “Reflections on reflections of infant word recognition,” in Early Language Development: Bridging Brain and Behaviour, eds A. D. Friederici and G. Thierry (Amsterdam: John Benhamins Publishing Company), 91–114. doi: 10.1075/tilar.5.06koo
Kooijman, V. M., Junge, C., Johnson, E. K., Hagoort, P., and Cutler, A. (2013). Predictive brain signals of linguistic development. Front. Psychol. 4:25. doi: 10.3389/fpsyg.2013.00025
Kotz, S. A., and Schwartze, M. (2010). Cortical speech processing unplugged: a timely subcortico-cortical framework. Trends Cogn. Sci. 14, 392–399. doi: 10.1016/j.tics.2010.06.005
Krijnen, E., van Steensel, R., Meeuwisse, M., Jongerling, J., and Severiens, S. (2020). Exploring a refined model of home literacy activities and associations with children’s emergent literacy skills. Read. Writ. 33, 207–238. doi: 10.1007/s11145-019-09957-4
Longhi, E. (2009). ‘Songese’: maternal structuring of musical interaction with infants. Psychol. Music 37, 195–213. doi: 10.1177/0305735608097042
Lonigan, C. J., Burgess, S. R., and Anthony, J. L. (2000). Development of emergent literacy and early reading skills in preschool children: evidence from a latent-variable longitudinal study. Dev. Psychol. 36, 596–613. doi: 10.1037/0012-1649.36.5.596
Luck, S. J., and Gaspelin, N. (2017). How to get statistically significant effects in any ERP experiment (and why you shouldn’t). Psychophysiology 54, 146–157. doi: 10.1111/psyp.12639
Makeig, S., Bell, A. J., Jung, T.-P., and Sejnowski, T. J. (1996). “Independent component analyssis of electroencephalographic data,” in Advances in Neural Information Processing Systems, eds D. Touretzky, M. Mozer, and M. Hasselmo (Cambridge, MA: MIT Press), 145–151. doi: 10.1109/ICOSP.2002.1180091
Maris, E., and Oostenveld, R. (2007). Nonparametric statistical testing of EEG- and MEG-data. J. Neurosci. Methods 164, 177–190. doi: 10.1016/j.jneumeth.2007.03.024
Melby-Lervåg, M., Lyster, S. A. H., and Hulme, C. (2012). Phonological skills and their role in learning to read: a meta-analytic review. Psychol. Bull. 138, 322–352. doi: 10.1037/a0026744
Metsala, J. L., and Walley, A. C. (1998). “Spoken vocabulary growth and the segmental restructuring of lexical representations: precursors to phonemic awareness and early reading ability,” in Word Recognition in Beginning Literacy, eds J. L. Metsala and L. C. Ehri (Mahwah, NJ: Lawrence Erlbaum Associates), 89–120.
Mills, D. L., Prat, C., Zangl, R., Stager, C. L., Neville, H. J., and Werker, J. F. (2004). Language experience and the organization of brain activity to phonetically similar words: ERP evidence from 14- and 20-month-olds. J. Cogn. Neurosci. 16, 1452–1464. doi: 10.1162/0898929042304697
Ngon, C., Martin, A., Dupoux, E., Cabrol, D., Dutat, M., and Peperkamp, S. (2013). (Non)words, (non)words, (non)words: evidence for a protolexicon during the first year of life. Dev. Sci. 16, 24–34. doi: 10.1111/j.1467-7687.2012.01189.x
Nordt, M., Hoehl, S., and Weigelt, S. (2016). The use of repetition suppression paradigms in developmental cognitive neuroscience. Cortex 80, 61–75. doi: 10.1016/j.cortex.2016.04.002
Oostenveld, R., Fries, P., Maris, E., and Schoffelen, J. M. (2011). FieldTrip: open source software for advanced analysis of MEG, EEG, and invasive electrophysiological data. Comput. Intell. Neurosci. 2011:156869. doi: 10.1155/2011/156869
Politimou, N., Dalla Bella, S., Farrugia, N., and Franco, F. (2019). Born to speak and sing: musical predictors of language development in pre-schoolers. Front. Psychol. 10:948. doi: 10.3389/fpsyg.2019.00948
Rugg, M. D. (1984a). Event-related potentials and the phonological processing of words and non-words. Neuropsychologia 22, 435–443. doi: 10.1016/0028-3932(84)90038-1
Rugg, M. D. (1984b). Event-related potentials in phonological matching tasks. Brain Lang. 23, 225–240. doi: 10.1016/0093-934x(84)90065-8
Sassenhagen, J., and Draschkow, D. (2019). Cluster-based permutation tests of MEG/EEG data do not establish significance of effect latency or location. Psychophysiology 56:e13335. doi: 10.1111/psyp.13335
Snijders, T. M., Benders, T., and Fikkert, P. (2020). Infants segment words from songs – an EEG study. Brain Sci. 10, 1–25. doi: 10.3390/brainsci10010039
Stadler, M. A., Watson, M., and Skahan, S. (2007). Rhyming and vocabulary: effects of lexical restructuring. Commun. Disord. Q. 28, 197–205. doi: 10.1177/1525740107311823
Teixidó, M., François, C., Bosch, L., and Männel, C. (2018). “The role of prosody in early speech segmentation and word-referent mapping: electrophysiological evidence,” in Prosodic Development in First Language Acquisition, eds P. Prieto and N. Esteve Gibert (Amsterdam: John Benjamins), 79–100. doi: 10.1075/tilar.23.05tei
Trainor, L. J. (1996). Infant preferences for infant-directed versus noninfant-directed playsongs and lullabies. Infant Behav. Dev. 19, 83–92. doi: 10.1016/s0163-6383(96)90046-6
Trehub, S. E., Unyk, A. M., and Trainor, L. J. (1993). Maternal singing in cross-cultural perspective. Infant Behav. Dev. 16, 285–295. doi: 10.1016/0163-6383(93)80036-8
Trehub, S. E., Unyk, A. M., Kamenetsky, S. B., Hill, D. S., Trainor, L. J., Henderson, J. L., et al. (1997). Mothers’ and fathers’ singing to infants. Dev. Psychol. 33, 500–507. doi: 10.1037/0012-1649.33.3.500
van Alphen, P., Brouwer, S. M., Davids, N., Dijkstra, E., and Fikkert, P. (2021). Word recognition and word prediction in preschoolers with (a suspicion of) a developmental language disorder: evidence from eye-tracking. J. Speech Lang. Hear. Res. 64, 2005–2021. doi: 10.1044/2021_jslhr-20-00227
Wagensveld, B., van Alphen, P., Segers, E., Hagoort, P., and Verhoeven, L. (2013). The neural correlates of rhyme awareness in preliterate and literate children. Clin. Neurophysiol. 124, 1336–1345. doi: 10.1016/j.clinph.2013.01.022
Wood, C., and Terrell, C. (1998). Preschool phonological awareness and subsequent literacy development. Educ. Psychol. 18, 253–274. doi: 10.1080/0144341980180301
Zamuner, T. S. (2009). The structure and nature of phonological neighbourhoods in children’s early lexicons. J. Child Lang. 36, 3–21. doi: 10.1017/S0305000908008829
Ziegler, J. C., and Goswami, U. (2005). Reading acquisition, developmental dyslexia, and skilled reading across languages: a psycholinguistic grain size theory. Psychol. Bull. 131, 3–29. doi: 10.1037/0033-2909.131.1.3
Keywords: rhyme, songs, vocabulary, ERP, infant
Citation: Hahn LE, Benders T, Fikkert P and Snijders TM (2021) Infants’ Implicit Rhyme Perception in Child Songs and Its Relationship With Vocabulary. Front. Psychol. 12:680882. doi: 10.3389/fpsyg.2021.680882
Received: 15 March 2021; Accepted: 02 August 2021;
Published: 06 September 2021.
Edited by:
Jerker Rönnberg, Linköping University, SwedenReviewed by:
Camillia Bouchon, Université Paris-Est Créteil Val de Marne, FranceMireille Besson, UMR 7291 Laboratoire de Neurosciences Cognitives (LNC), France
Copyright © 2021 Hahn, Benders, Fikkert and Snijders. This is an open-access article distributed under the terms of the Creative Commons Attribution License (CC BY). The use, distribution or reproduction in other forums is permitted, provided the original author(s) and the copyright owner(s) are credited and that the original publication in this journal is cited, in accordance with accepted academic practice. No use, distribution or reproduction is permitted which does not comply with these terms.
*Correspondence: Laura E. Hahn, bC5oYWhuQGxldC5ydS5ubA==; Tineke M. Snijders, dGluZWtlLnNuaWpkZXJzQG1waS5ubA==