- 1Department of Sports Biomechanics, Lakshmibai National Institute of Physical Education, Gwalior, India
- 2Sport Science and Sport Medicine, Singapore Sport Institute, Singapore, Singapore
- 3Physical Education and Sport Science Academic Group, National Institute of Education, Nanyang Technological University, Singapore, Singapore
- 4School of Sport Rehabilitation and Exercises Sciences, University of Essex, Colchester, United Kingdom
- 5Human Performance Laboratory, Department of Physical Activity Sciences, Universidad de Los Lagos, Osorno, Chile
- 6Centro de Investigación en Fisiología del Ejercicio, Facultad de Ciencias, Universidad Mayor, Santiago, Chile
The aim of this meta-analysis was to evaluate the effects of complex training (CT) on sprint, jump, and change of direction (COD) ability among soccer players. After an electronic search, 10 peer-reviewed articles were considered in the meta-analysis. The athletes included in this meta-analysis were amateur to professional level male soccer players (age range, 14–23 years). These studies incorporated CT in soccer players who were compared to a control group. Significant moderate to large improvements were observed in the CT group [sprint: standard mean difference (SMD) = 0.92–1.91; jump: SMD = 0.96–1.58; COD: SMD = 0.97–1.49] when compared to control groups. Subgroup analysis were also conducted based on age, duration, and competitive level. The beneficial effects of CT were greater in players <18 vs. ≥18 years (linear sprinting; SMD = 2.01 vs. −0.13), after ≥8 vs. <8 weeks (jumping and COD; SMD = 1.55–2.01 vs. 0.31–0.64, respectively) and among professional vs. amateur players (linear sprinting and with COD; SMD = 1.53–1.58 vs. 0.08–0.63, respectively). In conclusion, regular soccer training programs may be supplemented with CT to improve sprint, jump, and COD performance. A longer duration of CT (≥8 weeks) seems to be optimal in improving the physical abilities of soccer players. Professional players and <18 years players may benefit more from CT program.
Introduction
Soccer is a team sport which requires players to execute several short-duration maximal- and near-maximal physical efforts such as sprinting, jumping, and change(ing) of direction (COD) in order to overcome opponents during play (Stølen et al., 2005; Barnes et al., 2014). These physical fitness abilities are crucial in determining a player's performance potential during soccer games and tournaments (Castagna et al., 2003; Arnason et al., 2004; Faude et al., 2012), and have been linked with strength and power of the lower extremities (Arnason et al., 2004; Wisløff et al., 2004). To increase strength and power, resistance training (RT) and plyometric (PT)/power training programs are widely used in soccer (De Hoyo et al., 2016; Bauer et al., 2019; Ramirez-Campillo et al., 2020b). The aforementioned training methods induce neuromuscular adaptations, such as enhanced stretch-shortening cycle function, motor unit recruitment, firing frequency, intra- and inter-muscular coordination, and morphological changes (e.g., fiber type or pennation angle), enhancing overall performance (Markovic and Mikulic, 2010; Cormie et al., 2011). Although both RT (Marios et al., 2006; Sander et al., 2013; Silva et al., 2015) and PT (Van De Hoef et al., 2019; Ramirez-Campillo et al., 2020a,b) may improve strength and power, respectively, conducting independent training sessions for each method within a congested weekly micro-cycle may be troublesome for players and coaches.
Alternatively, a pragmatic approach combines both RT and PT methods within a single training work set, allowing more time to be devoted to specific soccer training activities. One such combination is termed as complex training (CT) (Fleck and Kontor, 1986), which commonly involves the performance of an exercise set with a high-load RT exercise, followed immediately by the execution of a low-load plyometric-power exercise. This format of training usually involves the combination of two biomechanically similar exercises with the high-load resistance exercise performed first [e.g., squat at 90% of one repetition maximum (1RM)], followed by the low-load power exercise (e.g., squat jumps) (Fleck and Kontor, 1986; Docherty et al., 2004). Such exercise sequencing stimulates the post-activation potentiation of performance, a phenomenon (Docherty et al., 2004; Hodgson et al., 2005; Carter and Greenwood, 2014; Prieske et al., 2020), that stimulates motor unit recruitment thus increasing the force producing potential of the utilized musculature within a given movement (Healy and Comyns, 2017).
In the last four decades (Fleck and Kontor, 1986), a considerable amount of published studies have analyzed the effects of CT on athletes' physical fitness and some meta-analyses have attempted to congregate the extant literature (Freitas et al., 2017; Bauer et al., 2019; Cormier et al., 2020; Pagaduan and Pojskic, 2020). Some meta-analyses have also been conducted specifically on team sports athletes (Freitas et al., 2017; Cormier et al., 2020). However, these studies included athletes with different sporting backgrounds (i.e., soccer, futsal, basketball, volleyball, rugby, football, water polo, track and field, and handball), potentially biasing the results for a particular sport, such as soccer. Indeed, the effects of PT and RT may vary according to an athlete's athletic history (Izquierdo et al., 2002; Fleck and Kraemer, 2004; De Villarreal et al., 2012; Asadi et al., 2016; Taylor et al., 2018; Vlachopoulos et al., 2018). Moreover, a 5-fold difference may be observed in the magnitude of the effects of CT on the physical fitness performance (i.e., sprinting) of athletes with different team sport background (Freitas et al., 2017).
Therefore, in order to clarify the specific effects of CT (and its moderators; e.g., programme duration) on soccer player's physical fitness performance, the aim of this meta-analysis was to evaluate the effects of CT interventions on sprint, jump, and COD ability among soccer players. With reference to previous studies (Freitas et al., 2017; Kobal et al., 2017; Bauer et al., 2019; Cormier et al., 2020), we hypothesized that CT would be effective in improving soccer players' physical fitness, with greater effects observed as compared to a control condition. Accordingly, our research question was: what are the effects of CT interventions (and its moderators) on sprint, jump, and COD ability among soccer players when compared to a control group?
Methods
The lead investigator (RKT) and a research assistant conducted electronic searches. The PubMed and Google scholar databases were used, conforming to the guidelines set by the Preferred Reported Items for Systematic Reviews and Meta-Analysis (PRISMA) (Moher et al., 2015). This study was registered with the International Platform of Registered Systematic Review and Meta-Analysis Protocols (INPLASY202090079). Articles published up to August 30th, 2020 were considered. Keywords were selected through experts' opinion and a systematic literature review. Using Boolean logic, the following combination of keywords was used in the search databases: “complex training” or “contrast training” or “combination of strength training and plyometrics” and “soccer” or “football.” An example for search strategy used in PubMed was: [(((complex training) OR contrast training) OR (combination of strength training and plyometrics)) AND soccer] OR football. Relevant articles' reference lists were examined to identify further articles for inclusion in the meta-analysis.
Inclusion Criteria and Exclusion Criteria
A PICOS (participants, intervention, comparators, outcomes, and study design) approach was used to rate studies' eligibility (Liberati et al., 2009). The respective inclusion/exclusion criteria adopted in our meta-analysis are reported in Table 1.
Methodological Quality of Studies
The Tool for the Assessment of Study Quality and Reporting in Exercise (TESTEX) was used to assess the quality of studies included in this meta-analysis (Smart et al., 2015). This tool comprises of 12 methodological quality assessment criteria (a full description of each criteria is provided in the results section for the included studies). The maximum score a study can obtain is 15 points, with higher ratings reflecting better study quality and reporting (Smart et al., 2015).
Data Extraction
We extracted from each eligible study, data relating to linear sprints over various distances (5–40-m linear sprint tests), vertical jumping and sprints which incorporated a COD (505, S4 × 5, T-test, BAT, S180 tests and dribbling with or without ball) (Castagna et al., 2003; Arnason et al., 2004; Faude et al., 2012). Means, standard deviations (SD), and sample sizes (n) (Table 2) were extracted by one author (RKT) from the included papers and were corroborated by a second author (DL). Any discrepancy between the authors was resolved through discussion with a third author (RRC). Where data were displayed in a figure or no numerical data were provided by authors after being contacted, validated (r = 0.99, p < 0.001) software (WebPlotDigitizer; https://apps.automeris.io/wpd/) was used to derive numerical data from figures (Drevon et al., 2017). In addition to the study data, sample characteristics, including age, playing level, training frequency, total duration of the intervention, and the type of training protocol used in the study were extracted and recorded.
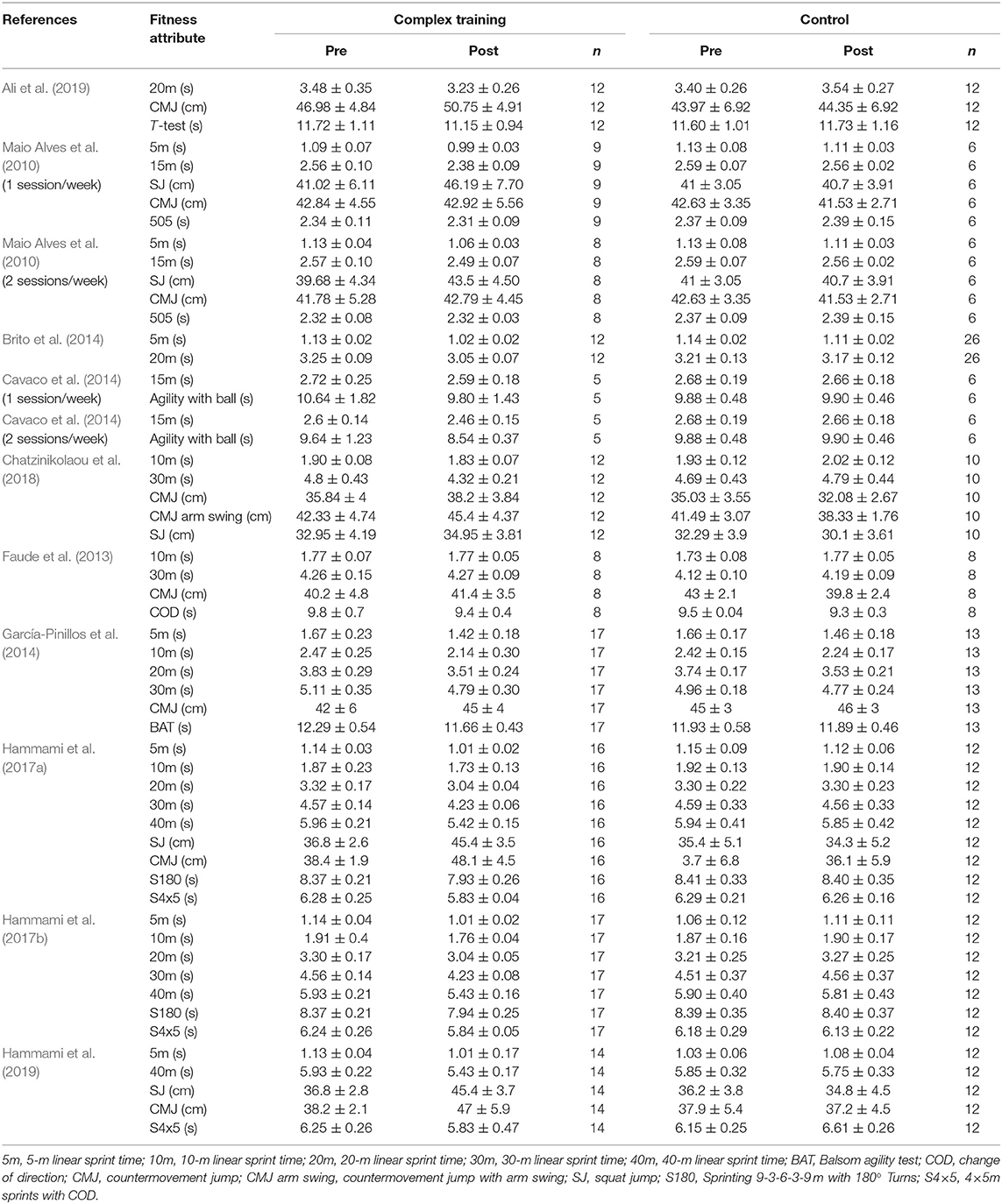
Table 2. The mean ± standard deviation of fitness variables reported for the complex training and control conditions in the included studies.
Statistical Analysis
The meta-analysis was conducted using the Review Manager Software (RevMan 5.3). Statistical significance was set at p ≤ 0.05. The inverse-variance random effects model for meta-analyses was used because it allocates a proportionate weight to trials based on the size of their individual standard errors (Deeks et al., 2008) and facilitates analysis whilst accounting for heterogeneity across studies (Kontopantelis et al., 2013). Effect sizes are represented by the standardized mean difference and are presented alongside 95% confidence intervals (CI). Standardized mean differences were calculated using the following equation (Cohen, 1988):
Where M1 - M2 is the difference in the mean outcome between groups, and SD pooled is the pooled standard deviation of the outcome among subjects, which was calculated using the following equation:
Where is the squared standard deviation of group 1 and is the squared standard deviation of group 2.
The SMD were interpreted with threshold values as follow: <0.2, trivial; 0.2–0.6, small; >0.6–1.2, moderate; >1.2–2.0, large; >2.0–4.0, very large; >4.0, extremely large (Hopkins et al., 2009).
In studies with more than one intervention group, the control group was proportionately divided to facilitate comparison across all participants (Higgins et al., 2008).
Subgroup analyses were performed on the variables that could have influenced the outcome after CT interventions, with median values of continues variables used as cut-off values for grouping [age (<18 vs. ≥18 years), and CT programme duration (<8 vs. ≥8 weeks)]. The subgrouping of studies according to the athlete's competition level [professional vs. amateur (youth, university); as reported in the studies] was also considered. The analyses were conducted when there were at least one study in the subgroups.
The I2 statistics was used to assess the heterogeneity among the studies, with values of <25, 25–75, and >75% interpreted as low, moderate and high heterogeneity, respectively (Higgins et al., 2003; Higgins, 2008). Heterogeneity was assessed in line with a low chi-squares' p-value (Higgins et al., 2003; Higgins, 2008) and a high Tau2 value (Borenstein et al., 2009).
Results
The initial search resulted in the retrieval of 1,373 articles and 22 additional articles were extracted through other sources. After removing duplicates, meta-analysis and systematic reviews 547 articles remained. After screening titles and abstracts for relevance, 516 articles were excluded with 31 full texts being retained. Further screening of articles based on our inclusion and exclusion criteria resulted in the inclusion of 10 studies in the meta-analysis (Maio Alves et al., 2010; Faude et al., 2013; Brito et al., 2014; Cavaco et al., 2014; García-Pinillos et al., 2014; Hammami et al., 2017a,b, 2019; Chatzinikolaou et al., 2018; Ali et al., 2019) (Figure 1).
General Characteristics of Studies
The study characteristics are presented in Table 3. A total of 313 subjects (all males) were included in this meta-analysis with 188 professional players and 125 amateur players. Sprinting time was measured through 5–40-m linear sprint tests. Jumping was measured using jump height in the squat jump and countermovement jump. The sprint time with COD was measured among the included studies using the 505, S4 × 5, T-test, BAT, and S180 tests, with or without dribbling a soccer ball. Considering the different number of COD (i.e., turns) involved among the tests, in a posteriori decision the tests were further divided based on those including ≤ 3 turns and >3 turns. Accordingly, the 505 and S4 × 5 tests involved ≤ 3 turns, while the T-test, BAT, and S180 tests involved >3 turns. Two studies assessed COD with ball, using ≤ 3 turns (Faude et al., 2013) and >3 turns (Cavaco et al., 2014).
Methodological Quality of Included Studies
Table 4 shows the methodological quality of the eligible studies in this meta-analysis. The score of all the studies according to TESTEX criteria ranged from 10 to 13 points. All studies selected for the meta-analysis obtained a score ≥10 points. Therefore, no study was excluded based on its methodological quality.
Meta-Analysis Results for Linear Sprint Performance
A moderate to very large significant improvement was noted after CT (within group, pre-post analysis) for 5-m (SMD = 2.44, 95% CI = 1.34–3.54, p < 0.001), 10-m (SMD = 0.67, 95% CI = 0.34–0.99, p < 0.001), 15-m (SMD = 1.05, 95% CI = 0.46–1.64, p < 0.001), 20-m (SMD = 1.43, 95% CI = 0.74–2.12, p < 0.001), 30-m (SMD = 1.31, 95% CI = 0.27–2.35, p = 0.01), and 40-m linear sprint performance (SMD = 2.65, 95% CI = 2.08–3.23, p < 0.001). The relative weight of each study in the analyses varied between 10.8 and 37.7%, with low to high heterogeneity (I2 = 0–88.0%).
A moderate to large significant difference was noted between CT and control (between group, post analysis) for 5-m (SMD = 1.91, 95% CI = 0.81–3.00, p < 0.001, Figure 2A), 10-m (SMD = 0.92, 95% CI = 0.32–1.53, p = 0.003, Figure 2B), 15-m (SMD = 1.07, 95% CI = 0.31–1.83, p = 0.006, Figure 2C), 20-m (SMD = 1.04, 95% CI = 0.49–1.58, p < 0.001, Figure 2D), and 40-m linear sprint performance (SMD = 1.28, 95% CI = 0.80–1.76, p < 0.001, Figure 2F). The relative weight of each study in the analyses varied between 10.1 and 35.3%, with low to high heterogeneity (I2 = 0–87%). Only 30-m linear sprint showed no difference between CT and control (Figure 2E).
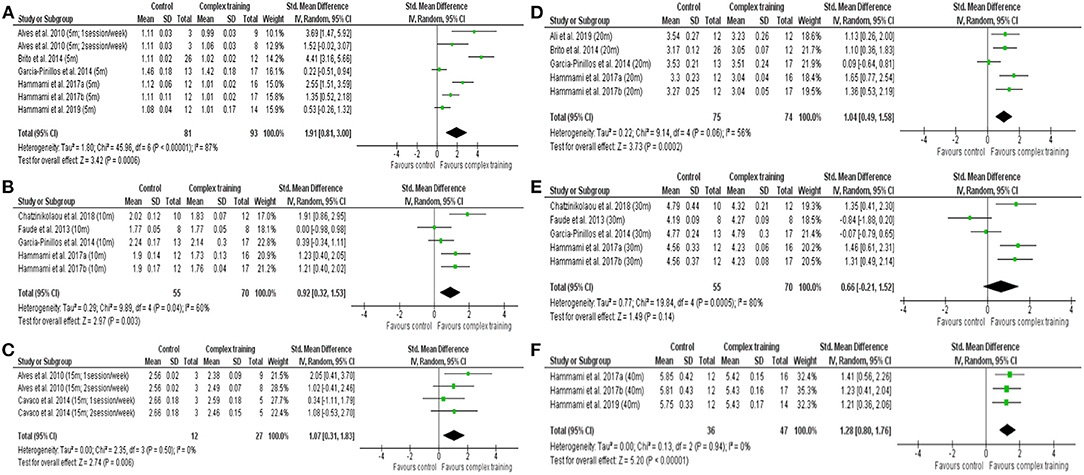
Figure 2. Forest plot of standardized mean difference in post-intervention outcomes between complex training group and control group, including (A) 5-m sprint time, (B) 10-m sprint time, (C) 15-m sprint time, (D) 20-m sprint time, (E) 30-m sprint time, (F) 40-m sprint time. Note: the relative weight of each study in the analysis is indicated by the size of the green squares.
Meta-Analysis Results for Vertical Jump Performance
A moderate to large significant improvement was noted after CT (within group, pre-post analysis) for countermovement jump (SMD = 0.89, 95% CI = 1.43–0.35, p < 0.001) and squat jump height performance (SMD = 1.33, 95% CI = 2.12–0.54, p = 0.001). The relative weight of each study in the analyses varied between 10.4 and 17.9%, with low to high heterogeneity (I2 = 64.0–76.0%).
In addition, a moderate to large significant difference was noted between CT and control (between group, post analysis) for countermovement jump (SMD = 0.96, 95% CI = 1.64–0.29, p = 0.005, Figure 3A) and squat jump height performance (SMD = 1.58, 95% CI = 2.38–0.78, p < 0.001, Figure 3B). The relative weight of each study in the analyses varied between 10.4 and 23.1%, with moderate heterogeneity (I2 = 60.0–73.0%).
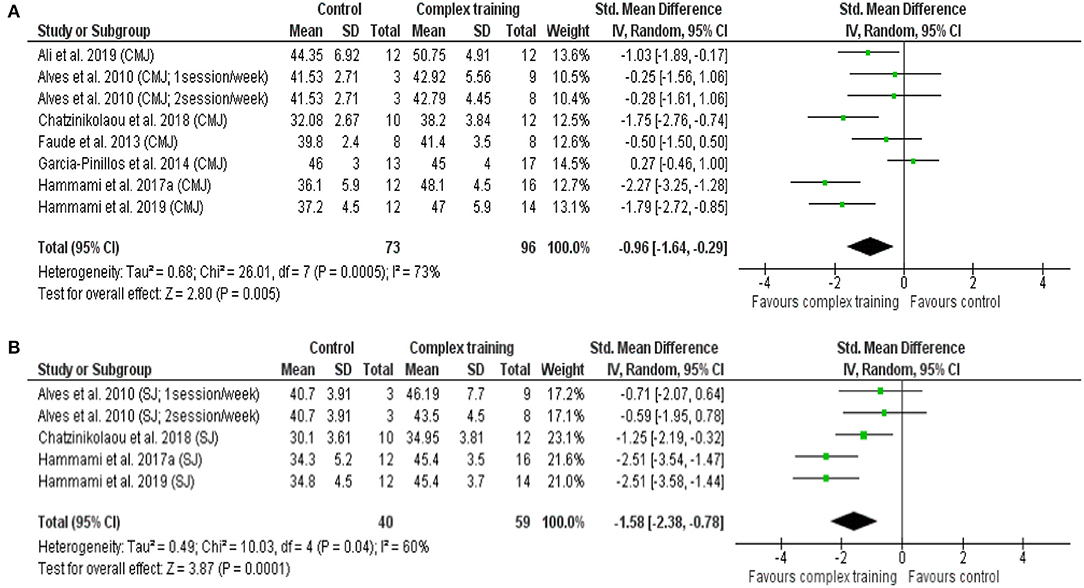
Figure 3. Forest plot of standardized mean difference in post-intervention outcomes between complex training group and control group, including (A) countermovement jump (CMJ) height, (B) squat jump (SJ) height. Note: the relative weight of each study in the analyses is indicated by the size of the green squares.
Meta-Analysis Results for Change of Direction Performance
A moderate to large significant improvement was noted after CT (within group, pre-post analysis) for COD with ≤ 3 turns (SMD = 1.11, 95% CI = 0.33–1.88, p = 0.005) and COD with >3 turns (SMD = 1.24, 95% CI = 0.83–1.64, p < 0.001). The relative weight of each study in the analyses varied between 8.7 and 19.5%, with moderate to high heterogeneity (I2 = 26.0–77.0%).
In addition, a moderate to large significant difference was noted between CT and control (between group, post analysis) for COD with ≤ 3 turns (SMD = 1.49, 95% CI = 0.40–2.58, p = 0.007, Figure 4A) and COD with >3 turns (SMD = 0.97, 95% CI = 0.41–1.53, p < 0.001, Figure 4B). The relative weight of each study in the analysis varied between 4.5 and 23.0%, with moderate to high heterogeneity (I2 = 47.0–82.0%).
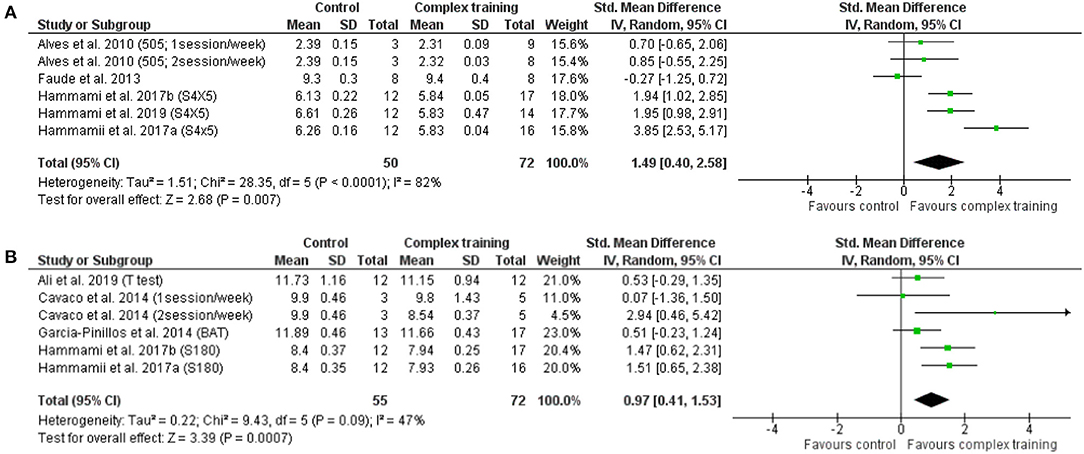
Figure 4. Forest plot of standardized mean difference in post-intervention outcomes between complex training group and control group, including (A) change of direction time with ≤ 3 turns, and (B) change of direction time with >3 turns. Note: the relative weight of each study in the analyses is indicated by the size of the green squares.
Subgroup Analyses
A subgroup analysis was performed based on age (< 18 vs. ≥18 years), playing level (professional vs. amateur), and duration of the training intervention (<8 vs. ≥8 weeks) as covariates (Table 5).
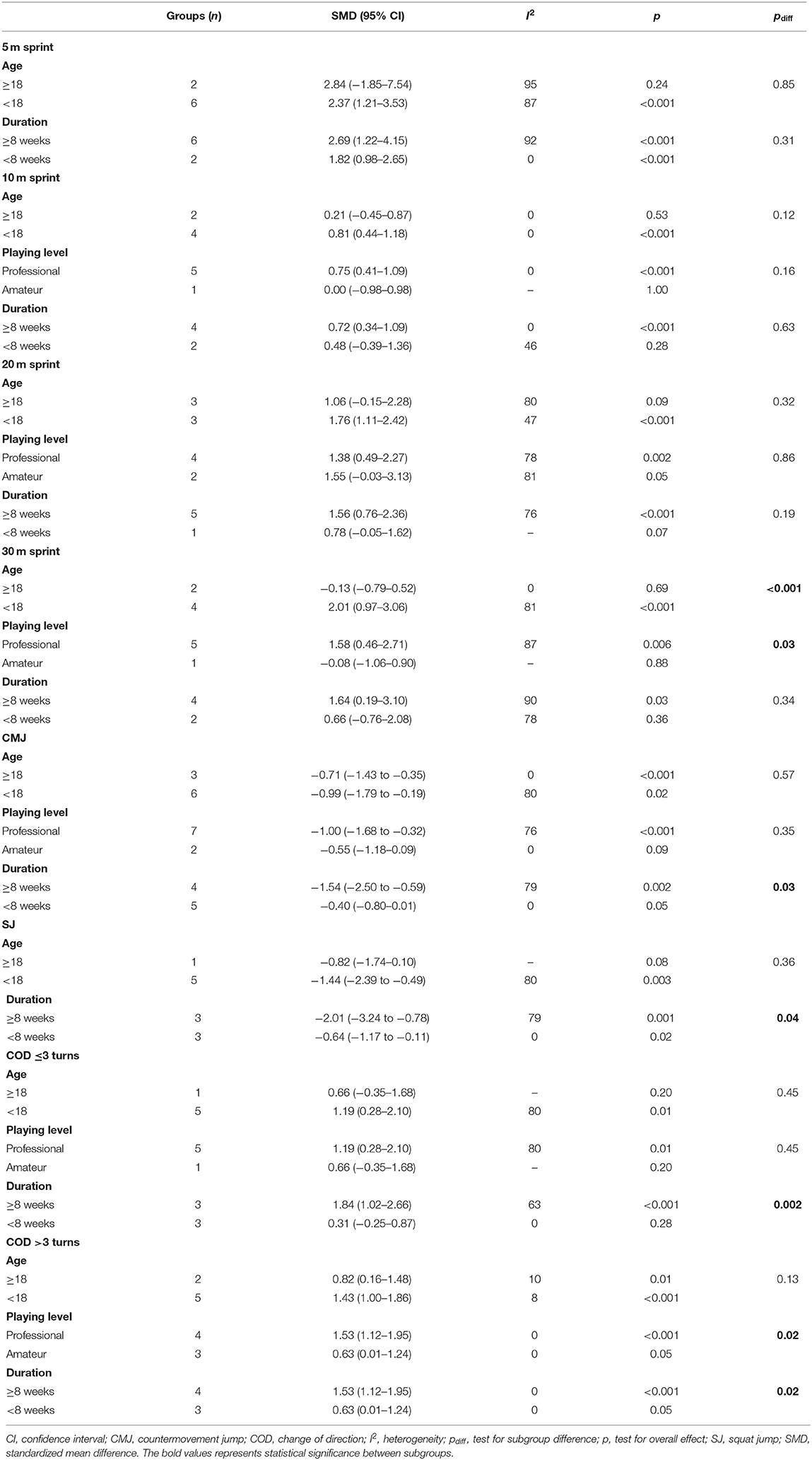
Table 5. Subgroup analyses of potential moderator factors for linear sprint time, vertical jump height and sprint with change of direction time.
Regarding the chronological age of players, a significant difference (p < 0.001, Table 5) in 30-m linear sprint time was noted between players ≥18 years (SMD = −0.13) and <18 years (SMD = 2.01), in favor of younger players, after CT.
For training programme duration, a greater improvement in countermovement jump (SMD = 1.54 vs. 0.40, p = 0.02), squat jump (SMD = 2.01 vs. 0.64, p = 0.04), COD with ≤ 3 turns (SMD = 1.84 vs. 0.31, p = 0.002), and COD >3 turns (SMD = 1.53 vs. 0.63, p = 0.02) was noted after ≥8 weeks compared to <8 weeks of CT (Table 5).
Regarding the competitive level of soccer players, a greater improvement in 30-m linear sprint time (SMD = 1.58 vs. 0.08, p = 0.03) and COD >3 turns (SMD = 1.53 vs. 0.63, p = 0.02) was noted in professional compared to amateur players after CT (Table 5).
Adverse Effect of Complex Training Intervention
Among the included studies, no injuries related to CT were reported. One study (Spineti et al., 2019) reported the total RPE-load for CT and RT intervention. A total RPE-load of 25,201 was reported after 48 sessions of CT, while a total RPE-load of 25,364 was reported after RT.
Discussion
Our meta-analyses indicated that compared to soccer training alone, CT induced moderate to large improvements in linear sprinting time, vertical jump height and sprinting with COD time in soccer players. The potentially beneficial effects derived from CT seem particularly applicable to younger players (<18 vs. ≥18 years, for linear sprinting), after longer training interventions (<8 vs. ≥8 weeks, for jumping and COD) and among players with greater competitive level (professional vs. amateur, for linear sprinting and with COD). Further, among the included studies, no injuries related to CT were reported. Overall, CT seems safe and effective compared to soccer training alone for the improvement of the physical fitness of soccer players.
The improvements in linear sprinting, vertical jumping and sprinting with COD performance following CT may be related to adaptative mechanisms similar to those induced by RT and PT alone, including maximal strength, hormonal milieu, structural, and neuromechanical adaptations, which may be potentiated through a cumulative post-activation performance enhancement effect induced with CT (Sale, 2002; Robbins, 2005; Carter and Greenwood, 2014). Indeed, several CT interventions included in this systematic review observed significant 1RM improvements in soccer players to a similar extent as RT (Faude et al., 2013; Brito et al., 2014; Spineti et al., 2016; Hammami et al., 2017a, 2019; Chatzinikolaou et al., 2018). As maximal strength is strongly related to sprint, jump, and COD in male soccer players (Wisløff et al., 2004), improvements in maximal strength may be an additional and relevant benefit derived from CT. In addition, improvements in sprint, jump, and COD performance after CT may be related to hormonal (Beaven et al., 2011; Ali et al., 2019) (e.g., testosterone increase) and cellular adaptations favorable to strength-power generation. For example, type IIx muscle fibers (i.e., those with greater contraction velocity, power, and rate of force development compared to type IIA and type I fibers) (Bottinelli et al., 1996; Harridge et al., 1996) may be favorably affected (i.e., greater preservation) by CT, even when compared to RT (Stasinaki et al., 2015). Indeed, an early study (Adams et al., 1993) reported that 19 weeks of heavy RT reduced percentage of type IIx fibers, whereas a more recent study (Stasinaki et al., 2015) reported a preservation of type IIx fibers after CT, similar to other studies that have included PT or CT (Macaluso et al., 2012, 2014; Grgic et al., 2020). Although not analyzed in the current study, the combination of RT and PT during CT may allow the summation of cellular and structural adaptations induced by RT and PT. Indeed, increased leg muscle volume was observed in soccer players after CT (Hammami et al., 2017a,b; Hammami et al., 2019). Although increases in muscle volume are usually achieved after RT interventions (ACSM, 2009; Krzysztofik et al., 2019), recent research suggest that PT may also induce significant hypertrophic effects (Grgic et al., 2020; Gumpenberger et al., 2020). Further, such structural adaptations after RT and PT seem not to interfere with neuromechanical adaptations (ACSM, 2009; Markovic and Mikulic, 2010). In fact, CT may favor energy transfer between concentric and eccentric muscle actions, providing better coordination and synchronization of active muscle groups to improve and enhance motor skills (Cronin et al., 2001; Robbins, 2005). Such improvements may include sprinting, vertical jumping and sprinting with COD performance, in addition to other motor skills enhancements such as kicking ability (Cavaco et al., 2014; García-Pinillos et al., 2014). Altogether, CT seems an effective training strategy for the enhancement of sprinting, jumping and sprint with COD. However, future studies should elucidate the effectiveness of CT on such outcomes among soccer players, particularly when compared to other training methods, including RT.
A cumulative post-activation performance enhancement effect induced with CT (Sale, 2002; Robbins, 2005) may help explain the performance enhancement (Tillin and Bishop, 2009; Petisco et al., 2019). Further, as CT combine higher (i.e., RT) and lower loads (i.e., PT) this may have optimized the force-velocity curve of soccer players (Cormie et al., 2011) by ensuring that the prescribed training addressed the two broad components of the continuum. Considering the relevance of the force-velocity spectrum parameters among soccer players (Jimenez-Reyes et al., 2020), it seems plausible that an optimization of the force-velocity spectrum may help to explain the improvements in performance after CT (Jiménez-Reyes et al., 2017; Jimenez-Reyes et al., 2019). Such a combination of high-load low-velocity and low-load high-velocity exercises during CT may favor recruitment of fast-twitch muscle fibers (Gołaś et al., 2016), which are particularly important during maximal-intensity and short-duration actions (e.g., vertical jumping) (Fry et al., 2003; Macaluso et al., 2012). In contrast to our findings, a previous meta-analysis (Bauer et al., 2019) did not observe jumping improvements after CT. However, Bauer et al. (2019) included studies involving a myriad of participants (e.g., schoolchildren, recreational trained students, untrained women, athletes from different sports) and compared the effects of CT with mixed training methods (e.g., RT, PT, mixed methods). In this sense, the results from our meta-analysis may offer a finding specific for soccer players (i.e., male soccer players), a finding that could have been potentially distorted amongst a wider population of athletes or the general population. On the other hand, extrapolation of current findings to other populations should proceed with caution.
Subgroup analyses were conducted to determine the effects of CT on physical fitness according to soccer player's chronological age, competitive level and duration of training programmes. Regarding the subgroup analysis according to the soccer players chronological age (<18 vs. ≥18 years), greater performance enhancements were noted after CT among players <18 years of age. The greater improvement among younger players may be due to the fact that players ≥18 years were already in the later stage of physical development and, hence, possessed a lower ceiling for further adaptation. In addition, players >18 years of age were likely to have performed RT and PT for a longer period of time (i.e., greater training age), experiencing diminishing return from CT (Carter and Greenwood, 2014) in the process. However, our subgroup analysis showed that professional players achieved greater performance improvements after CT compared to amateur players. Professional athletes have been shown to be better responders to post-activation performance enhancement (Carter and Greenwood, 2014). Indeed, professional athletes also tend to possess greater strength levels than amateur athletes, which might have enabled them to maximize the benefits of post-activation performance enhancement with CT (Seitz et al., 2014; Seitz and Haff, 2016; Suchomel et al., 2016). In addition, professional players' may possess a greater proportion of fast-twitch muscle fibers compared to amateur soccer players (Ostojic, 2004), rendering greater benefits from post-activation performance enhancement with CT (Seitz et al., 2014; Seitz and Haff, 2016; Suchomel et al., 2016). Furthermore, professional players may train with greater intensity, with professional athletes possessing greater intent to lift ballistically compared to non-professional athletes (Suchomel et al., 2018). In addition, CT was performed concurrently to soccer practices. It is possible that the number of soccer training sessions and games were greater in professional compared to non-professional players, with the former performing also greater number of sprints, jumps, and high-intensity actions. This may have also concurred to greater overall better neuromuscular adaptations in the professional vs. amateur athletes. Finally, regarding the subgroup analysis according to CT programme duration (<8 vs. ≥8 weeks), greater physical fitness improvements were noted after longer training interventions, in line with previous literature (Aagaard et al., 2001; Blazevich et al., 2007; Bolger et al., 2015). Although not surprising, current findings confirm that longer CT interventions may also induce larger gains in the physical fitness of male soccer players, particularly for jumping and sprints with COD.
Some limitations are acknowledged. Firstly, the findings of our systematic review suggest the absence of CT studies involving female soccer players. Therefore, current findings should not be simply extrapolated to female athletes. Future CT research should involve female soccer players to overcome this shortcoming in literature. Another major shortcoming noted in this systematic review is the lack of studies comparing CT to RT. Future research should focus on this comparison.
Conclusion
The findings of this meta-analysis suggests that supplementing the regular soccer training sessions with CT improves athlete's physical fitness (i.e., sprinting, jumping, and COD ability) compared to soccer training alone. A longer duration of CT (≥8 weeks) seems to be optimal in improving the physical abilities of soccer players. Professional players and <18 years players may benefit more from CT program.
Data Availability Statement
The original contributions presented in the study are included in the article/supplementary material, further inquiries can be directed to the corresponding author.
Author Contributions
All authors made significant contributions, including preparation of the first draft of the manuscript, data collection, analysis of data, interpretation of data, and/or provided meaningful revision and feedback, read, and approved the final manuscript.
Conflict of Interest
The authors declare that the research was conducted in the absence of any commercial or financial relationships that could be construed as a potential conflict of interest.
Acknowledgments
The authors want to thank Gopal Kumar for providing help in initial extraction of studies for this meta-analysis.
References
Aagaard, P., Andersen, J. L., Dyhre-Poulsen, P., Leffers, A. M., Wagner, A., Magnusson, S. P., et al. (2001). A mechanism for increased contractile strength of human pennate muscle in response to strength training: changes in muscle architecture. J. Physiol. 534, 613–623. doi: 10.1111/j.1469-7793.2001.t01-1-00613.x
ACSM (2009). American College of Sports Medicine position stand. Progression models in resistance training for healthy adults. Med. Sci. Sports Exerc. 41, 687–708. doi: 10.1249/MSS.0b013e3181915670
Adams, G. R., Hather, B. M., Baldwin, K. M., and Dudley, G. A. (1993). Skeletal muscle myosin heavy chain composition and resistance training. J. Appl. Physiol. 74, 911–915. doi: 10.1152/jappl.1993.74.2.911
Ali, K., Verma, S., Ahmad, I., Singla, D., Saleem, M., and Hussain, M. E. (2019). Comparison of complex versus contrast training on steroid hormones and sports performance in male soccer players. J. Chiropr. Med. 18, 131–138. doi: 10.1016/j.jcm.2018.12.001
Arnason, A., Sigurdsson, S. B., Gudmundsson, A., Holme, I., Engebretsen, L., and Bahr, R. (2004). Physical fitness, injuries, and team performance in soccer. Med. Sci. Sports Exerc. 36, 278–285. doi: 10.1249/01.MSS.0000113478.92945.CA
Asadi, A., Arazi, H., Young, W. B., and De Villarreal, E. S. (2016). The effects of plyometric training on change-of-direction ability: a meta-analysis. Int. J. Sports Physiol. Perf. 11, 563–573. doi: 10.1123/ijspp.2015-0694
Barnes, C., Archer, D. T., Hogg, B., Bush, M., and Bradley, P. S. (2014). The evolution of physical and technical performance parameters in the English Premier League. Int. J. Sports Med. 35, 1095–1100. doi: 10.1055/s-0034-1375695
Bauer, P., Uebellacker, F., Mitter, B., Aigner, A. J., Hasenoehrl, T., Ristl, R., et al. (2019). Combining higher-load and lower-load resistance training exercises: a systematic review and meta-analysis of findings from complex training studies. J. Sci. Med. Sport 22, 838–851. doi: 10.1016/j.jsams.2019.01.006
Beaven, C. M., Gill, N. D., Ingram, J. R., and Hopkins, W. G. (2011). Acute salivary hormone responses to complex exercise bouts. J. Strength Cond. Res 25, 1072–1078. doi: 10.1519/JSC.0b013e3181bf4414
Blazevich, A. J., Cannavan, D., Coleman, D. R., and Horne, S. (2007). Influence of concentric and eccentric resistance training on architectural adaptation in human quadriceps muscles. J. Appl. Physiol. 103, 1565–1575. doi: 10.1152/japplphysiol.00578.2007
Bolger, R., Lyons, M., Harrison, A. J., and Kenny, I. C. (2015). Sprinting performance and resistance-based training interventions: a systematic review. J. Strength Cond. Res. 29, 1146–1156. doi: 10.1519/JSC.0000000000000720
Borenstein, M., Hedges, L. V., Higgins, J. P., and Rothstein, H. R. (2009). “Identifying and Quantifying Heterogeneity,” in Introduction to Meta-Analysis, eds M. Borenstein, L. V. Hedges, J. P. Higgins and H. R. Rothstein (Chichester, UK: John Wiley & Sons), 107–125.
Bottinelli, R., Canepari, M., Pellegrino, M. A., and Reggiani, C. (1996). Force-velocity properties of human skeletal muscle fibres: myosin heavy chain isoform and temperature dependence. J. Physiol. 495(Pt 2), 573–586. doi: 10.1113/jphysiol.1996.sp021617
Brito, J., Vasconcellos, F., Oliveira, J., Krustrup, P., and Rebelo, A. (2014). Short-term performance effects of three different low-volume strength-training programmes in college male soccer players. J. Hum. Kinet. 40, 121–128. doi: 10.2478/hukin-2014-0014
Carter, J., and Greenwood, M. (2014). Complex training reexamined: review and recommendations to improve strength and power. Strength Cond. J. 36, 11–19. doi: 10.1519/SSC.0000000000000036
Castagna, C., D'ottavio, S., and Abt, G. (2003). Activity profile of young soccer players during actual match play. J. Strength Cond. Res. 17, 775–780. doi: 10.1519/00124278-200311000-00024
Cavaco, B., Sousa, N., Dos Reis, V. M., Garrido, N., Saavedra, F., Mendes, R., et al. (2014). Short-term effects of complex training on agility with the ball, speed, efficiency of crossing and shooting in youth soccer players. J. Hum. Kinet. 43, 105–112. doi: 10.2478/hukin-2014-0095
Chatzinikolaou, A., Michaloglou, K., Avloniti, A., Leontsini, D., Deli, C. K., Vlachopoulos, D., et al. (2018). The trainability of adolescent soccer players to brief periodized complex training. Int. J. Sports Physiol. Perform. 13, 645–655. doi: 10.1123/ijspp.2017-0763
Cohen, J. (1988). “The t tests for means,” in Statistical Power Analysis for the Behavioral Sciences, 2nd Edn., ed J. Cohen (New York, NY: Routledge), 19–40.
Cormie, P., Mcguigan, M. R., and Newton, R. U. (2011). Developing maximal neuromuscular power: part 1–biological basis of maximal power production. Sports Med. 41, 17–38. doi: 10.2165/11537690-000000000-00000
Cormier, P., Freitas, T. T., Rubio-Arias, J. A., and Alcaraz, P. E. (2020). Complex and contrast training: does strength and power training sequence affect performance-based adaptations in team sports? a systematic review and meta-analysis. J. Strength Cond. Res. 34, 1461–1479. doi: 10.1519/JSC.0000000000003493
Cronin, J., Mcnair, P. J., and Marshall, R. N. (2001). Velocity specificity, combination training and sport specific tasks. J. Sci. Med. Sport 4, 168–178. doi: 10.1016/S1440-2440(01)80027-X
De Hoyo, M., Gonzalo-Skok, O., Sañudo, B., Carrascal, C., Plaza-Armas, J. R., Camacho-Candil, F., et al. (2016). Comparative effects of in-season full-back squat, resisted sprint training, and plyometric training on explosive performance in u-19 elite soccer players. J. Strength Cond. Res. 30, 368–377. doi: 10.1519/JSC.0000000000001094
De Villarreal, E. S., Requena, B., and Cronin, J. B. (2012). The effects of plyometric training on sprint performance: a meta-analysis. J. Strength Cond. Res. 26, 575–584. doi: 10.1519/JSC.0b013e318220fd03
Deeks, J. J., Higgins, J. P., and Altman, D. G. (2008). “Analysing data and undertaking meta-analyses,” in Cochrane Handbook for Systematic Reviews of Interventions, eds J. P. Higgins and S. Green (Chichester, UK: John Wiley & Sons), 243–296.
Docherty, D., Robbins, D., and Hodgson, M. (2004). Complex training revisited: a review of its current status as a viable training approach. Strength Cond. J. 26, 52–57. doi: 10.1519/00126548-200412000-00011
Drevon, D., Fursa, S. R., and Malcolm, A. L. (2017). Intercoder reliability and validity of webplotdigitizer in extracting graphed data. Behav. Modif. 41, 323–339. doi: 10.1177/0145445516673998
Faude, O., Koch, T., and Meyer, T. (2012). Straight sprinting is the most frequent action in goal situations in professional football. J. Sports Sci. 30, 625–631. doi: 10.1080/02640414.2012.665940
Faude, O., Roth, R., Di Giovine, D., Zahner, L., and Donath, L. (2013). Combined strength and power training in high-level amateur football during the competitive season: a randomised-controlled trial. J. Sports Sci. 31, 1460–1467. doi: 10.1080/02640414.2013.796065
Fleck, S., and Kontor, K. (1986). Complex training. Strength Cond. J. 8, 66–72. doi: 10.1519/0744-0049(1986)008<0066:CT>2.3.CO
Fleck, S. J., and Kraemer, W. J. (2004). Designing Resistance Training Programs. Champaign, IL: Human Kinetics.
Freitas, T. T., Martinez-Rodriguez, A., Calleja-González, J., and Alcaraz, P. E. (2017). Short-term adaptations following complex training in team-sports: a meta-analysis. PLoS ONE 12:e0180223. doi: 10.1371/journal.pone.0180223
Fry, A. C., Schilling, B. K., Staron, R. S., Hagerman, F. C., Hikida, R. S., and Thrush, J. T. (2003). Muscle fiber characteristics and performance correlates of male Olympic-style weightlifters. J. Strength Cond. Res. 17, 746–754. doi: 10.1519/00124278-200311000-00020
García-Pinillos, F., Martínez-Amat, A., Hita-Contreras, F., Martínez-López, E. J., and Latorre-Román, P. A. (2014). Effects of a contrast training program without external load on vertical jump, kicking speed, sprint, and agility of young soccer players. J. Strength Cond. Res. 28, 2452–2460. doi: 10.1519/JSC.0000000000000452
Gołaś, A., Maszczyk, A., Zajac, A., Mikołajec, K., and Stastny, P. (2016). Optimizing post activation potentiation for explosive activities in competitive sports. J. Hum. Kinet. 52, 95–106. doi: 10.1515/hukin-2015-0197
Grgic, J., Schoenfeld, B. J., and Mikulic, P. (2020). Effects of plyometric vs. resistance training on skeletal muscle hypertrophy: a review. J. Sport Health Sci. doi: 10.1016/j.jshs.2020.06.010. [Epub ahead of print].
Gumpenberger, M., Wessner, B., Graf, A., Narici, M. V., Fink, C., Braun, S., et al. (2020). Remodeling the skeletal muscle extracellular matrix in older age-effects of acute exercise stimuli on gene expression. Int. J. Mol. Sci. 21:7089. doi: 10.3390/ijms21197089
Hammami, M., Gaamouri, N., Shephard, R. J., and Chelly, M. S. (2019). Effects of contrast strength vs. plyometric training on lower-limb explosive performance, ability to change direction and neuromuscular adaptation in soccer players. J. Strength Cond. Res. 33, 2094–2103. doi: 10.1519/JSC.0000000000002425
Hammami, M., Negra, Y., Shephard, R. J., and Chelly, M. S. (2017a). The effect of standard strength vs. contrast strength training on the development of sprint, agility, repeated change of direction, and jump in junior male soccer players. J. Strength Cond. Res. 31, 901–912. doi: 10.1519/JSC.0000000000001815
Hammami, M., Negra, Y., Shephard, R. J., and Chelly, M. S. (2017b). Effects of leg contrast strength training on sprint, agility and repeated change of direction performance in male soccer players. J. Sports Med. Phys. Fitness 57, 1424–1431. doi: 10.23736/S0022-4707.17.06951-1
Harridge, S. D., Bottinelli, R., Canepari, M., Pellegrino, M. A., Reggiani, C., Esbjörnsson, M., et al. (1996). Whole-muscle and single-fibre contractile properties and myosin heavy chain isoforms in humans. Pflugers Arch. 432, 913–920. doi: 10.1007/s004240050215
Healy, R., and Comyns, T. M. (2017). The application of postactivation potentiation methods to improve sprint speed. Strength Cond. J. 39, 1–9. doi: 10.1519/SSC.0000000000000276
Higgins, J. P. (2008). Commentary: heterogeneity in meta-analysis should be expected and appropriately quantified. Int. J. Epidemiol. 37, 1158–1160. doi: 10.1093/ije/dyn204
Higgins, J. P., Deeks, J. J., and Altman, D. G. (2008). “Special Topics in Statistics,” in Cochrane Handbook for Systematic Reviews of Interventions, eds J. P. Higgins and S. Green (Chichester, UK: John Wiley & Sons), 481–529. doi: 10.1002/9780470712184.ch16
Higgins, J. P., Thompson, S. G., Deeks, J. J., and Altman, D. G. (2003). Measuring inconsistency in meta-analyses. BMJ 327, 557–560. doi: 10.1136/bmj.327.7414.557
Hodgson, M., Docherty, D., and Robbins, D. (2005). Post-activation potentiation: underlying physiology and implications for motor performance. Sports Med. 35, 585–595. doi: 10.2165/00007256-200535070-00004
Hopkins, W. G., Marshall, S. W., Batterham, A. M., and Hanin, J. (2009). Progressive statistics for studies in sports medicine and exercise science. Med. Sci. Sports Exerc. 41, 3–13. doi: 10.1249/MSS.0b013e31818cb278
Izquierdo, M., Häkkinen, K., Gonzalez-Badillo, J. J., Ibáñez, J., and Gorostiaga, E. M. (2002). Effects of long-term training specificity on maximal strength and power of the upper and lower extremities in athletes from different sports. Eur. J. Appl. Physiol. 87, 264–271. doi: 10.1007/s00421-002-0628-y
Jimenez-Reyes, P., Garcia-Ramos, A., Parraga-Montilla, J. A., Morcillo-Losa, J. A., Cuadrado-Penafiel, V., Castano-Zambudio, A., et al. (2020). Seasonal changes in the sprint acceleration force-velocity profile of elite male soccer players. J. Strength Cond. Res. doi: 10.1519/JSC.0000000000003513. [Epub ahead of print].
Jiménez-Reyes, P., Samozino, P., Brughelli, M., and Morin, J. B. (2017). Effectiveness of an individualized training based on force-velocity profiling during jumping. Front. Physiol. 7:677. doi: 10.3389/fphys.2016.00677
Jimenez-Reyes, P., Samozino, P., and Morin, J. B. (2019). Optimized training for jumping performance using the force-velocity imbalance: individual adaptation kinetics. PLoS ONE 14:e0216681. doi: 10.1371/journal.pone.0216681
Kobal, R., Loturco, I., Barroso, R., Gil, S., Cuniyochi, R. R., Ugrinowitsch, C., et al. (2017). Effects of different combinations of strength, power, and plyometric training on the physical performance of elite young soccer players. J. Strength Cond. Res. 31, 1468–1476. doi: 10.1519/JSC.0000000000001609
Kontopantelis, E., Springate, D. A., and Reeves, D. (2013). A re-analysis of the Cochrane Library data: the dangers of unobserved heterogeneity in meta-analyses. PLoS ONE 8:e69930. doi: 10.1371/journal.pone.0069930
Krzysztofik, M., Wilk, M., Wojdała, G., and Gołaś, A. (2019). Maximizing muscle hypertrophy: a systematic review of advanced resistance training techniques and methods. Int. J. Environ. Res. Public Health 16:4897. doi: 10.3390/ijerph16244897
Liberati, A., Altman, D. G., Tetzlaff, J., Mulrow, C., Gøtzsche, P. C., Ioannidis, J. P., et al. (2009). The PRISMA statement for reporting systematic reviews and meta-analyses of studies that evaluate health care interventions: explanation and elaboration. J. Clin. Epidemiol. 62, e1–34. doi: 10.1016/j.jclinepi.2009.06.006
Macaluso, F., Isaacs, A. W., Di Felice, V., and Myburgh, K. H. (2014). Acute change of titin at mid-sarcomere remains despite 8 wk of plyometric training. J. Appl. Physiol. 116, 1512–1519. doi: 10.1152/japplphysiol.00420.2013
Macaluso, F., Isaacs, A. W., and Myburgh, K. H. (2012). Preferential type II muscle fiber damage from plyometric exercise. J. Athl. Train 47, 414–420. doi: 10.4085/1062-6050-47.4.13
Maio Alves, J. M., Rebelo, A. N., Abrantes, C., and Sampaio, J. (2010). Short-term effects of complex and contrast training in soccer players' vertical jump, sprint, and agility abilities. J. Strength Cond. Res. 24, 936–941. doi: 10.1519/JSC.0b013e3181c7c5fd
Marios, C., Smilios, I., Sotiropoulos, K., Volaklis, K., Theophilos, P., and Tokmakidis, S. (2006). The effects of resistance training on the physical capacities of adolescent soccer players. J. Strength Cond. Res. 20, 783–791. doi: 10.1519/00124278-200611000-00010
Markovic, G., and Mikulic, P. (2010). Neuro-musculoskeletal and performance adaptations to lower-extremity plyometric training. Sports Med. 40, 859–895. doi: 10.2165/11318370-000000000-00000
Moher, D., Shamseer, L., Clarke, M., Ghersi, D., Liberati, A., Petticrew, M., et al. (2015). Preferred reporting items for systematic review and meta-analysis protocols (PRISMA-P) 2015 statement. Syst. Rev. 4:1. doi: 10.1186/2046-4053-4-1
Ostojic, S. M. (2004). Elite and nonelite soccer players: preseasonal physical and physiological characteristics. Res. Sports Med. 12, 143–150. doi: 10.1080/15438620490460495
Pagaduan, J., and Pojskic, H. (2020). A meta-analysis on the effect of complex training on vertical jump performance. J. Hum. Kinet. 71, 255–265. doi: 10.2478/hukin-2019-0087
Petisco, C., Ramirez-Campillo, R., Hernández, D., Gonzalo-Skok, O., Nakamura, F. Y., and Sanchez-Sanchez, J. (2019). Post-activation potentiation: effects of different conditioning intensities on measures of physical fitness in male young professional soccer players. Front. Psychol. 10:1167. doi: 10.3389/fpsyg.2019.01167
Prieske, O., Behrens, M., Chaabene, H., Granacher, U., and Maffiuletti, N. A. (2020). Time to differentiate postactivation “potentiation” from “performance enhancement” in the strength and conditioning community. Sports Med. 50, 1559–1565. doi: 10.1007/s40279-020-01300-0
Ramirez-Campillo, R., Castillo, D., Raya-González, J., Moran, J., De Villarreal, E. S., and Lloyd, R. S. (2020a). Effects of plyometric jump training on jump and sprint performance in young male soccer players: a systematic review and meta-analysis. Sports Med. 50, 2125–2143. doi: 10.1007/s40279-020-01337-1
Ramirez-Campillo, R., Sanchez-Sanchez, J., Romero-Moraleda, B., Yanci, J., García-Hermoso, A., and Manuel Clemente, F. (2020b). Effects of plyometric jump training in female soccer player's vertical jump height: a systematic review with meta-analysis. J. Sports Sci. 38, 1475–1487. doi: 10.1080/02640414.2020.1745503
Robbins, D. W. (2005). Postactivation potentiation and its practical applicability: a brief review. J. Strength Cond. Res. 19, 453–458. doi: 10.1519/R-14653.1
Sale, D. G. (2002). Postactivation potentiation: role in human performance. Exerc. Sport Sci. Rev. 30, 138–143. doi: 10.1097/00003677-200207000-00008
Sander, A., Keiner, M., Wirth, K., and Schmidtbleicher, D. (2013). Influence of a 2-year strength training programme on power performance in elite youth soccer players. Eur. J. Sport Sci. 13, 445–451. doi: 10.1080/17461391.2012.742572
Seitz, L. B., De Villarreal, E. S., and Haff, G. G. (2014). The temporal profile of postactivation potentiation is related to strength level. J. Strength Cond. Res. 28, 706–715. doi: 10.1519/JSC.0b013e3182a73ea3
Seitz, L. B., and Haff, G. G. (2016). Factors modulating post-activation potentiation of jump, sprint, throw, and upper-body ballistic performances: a systematic review with meta-analysis. Sports Med. 46, 231–240. doi: 10.1007/s40279-015-0415-7
Silva, J., Nassis, G., and Rebelo, A. (2015). Strength training in soccer with a specific focus on highly trained players. Sports Med. Open 1:17. doi: 10.1186/s40798-015-0006-z
Smart, N. A., Waldron, M., Ismail, H., Giallauria, F., Vigorito, C., Cornelissen, V., et al. (2015). Validation of a new tool for the assessment of study quality and reporting in exercise training studies: TESTEX. Int. J. Evid. Based Healthc. 13, 9–18. doi: 10.1097/XEB.0000000000000020
Spineti, J., Figueiredo, T., Bastos, D. E. O. V., Assis, M., Fernandes, D. E. O. L., Miranda, H., et al. (2016). Comparison between traditional strength training and complex contrast training on repeated sprint ability and muscle architecture in elite soccer players. J. Sports Med. Phys. Fitness 56, 1269–1278.
Spineti, J., Figueiredo, T., Willardson, J., Bastos De Oliveira, V., Assis, M., Fernandes De Oliveira, L., et al. (2019). Comparison between traditional strength training and complex contrast training on soccer players. J. Sports Med. Phys. Fitness 59, 42–49. doi: 10.23736/S0022-4707.18.07934-3
Stasinaki, A. N., Gloumis, G., Spengos, K., Blazevich, A. J., Zaras, N., Georgiadis, G., et al. (2015). Muscle strength, power, and morphologic adaptations after 6 weeks of compound vs. complex training in healthy men. J. Strength Cond. Res. 29, 2559–2569. doi: 10.1519/JSC.0000000000000917
Stølen, T., Chamari, K., Castagna, C., and Wisløff, U. (2005). Physiology of soccer: an update. Sports Med. 35, 501–536. doi: 10.2165/00007256-200535060-00004
Suchomel, T. J., Nimphius, S., Bellon, C. R., and Stone, M. H. (2018). The importance of muscular strength: training considerations. Sports Med. 48, 765–785. doi: 10.1007/s40279-018-0862-z
Suchomel, T. J., Nimphius, S., and Stone, M. H. (2016). The importance of muscular strength in athletic performance. Sports Med. 46, 1419–1449. doi: 10.1007/s40279-016-0486-0
Taylor, J. B., Ford, K. R., Schmitz, R. J., Ross, S. E., Ackerman, T. A., and Shultz, S. J. (2018). Sport-specific biomechanical responses to an ACL injury prevention programme: a randomised controlled trial. J. Sports Sci. 36, 2492–2501. doi: 10.1080/02640414.2018.1465723
Tillin, N. A., and Bishop, D. (2009). Factors modulating post-activation potentiation and its effect on performance of subsequent explosive activities. Sports Med. 39, 147–166. doi: 10.2165/00007256-200939020-00004
Van De Hoef, P. A., Brauers, J. J., Van Smeden, M., Backx, F. J. G., and Brink, M. S. (2019). The effects of lower-extremity plyometric training on soccer-specific outcomes in adult male soccer players: a systematic review and meta-analysis. Int. J. Sports Physiol. Perform. 15, 1–15. doi: 10.1123/ijspp.2019-0565
Vlachopoulos, D., Barker, A. R., Ubago-Guisado, E., Williams, C. A., and Gracia-Marco, L. (2018). The effect of a high-impact jumping intervention on bone mass, bone stiffness and fitness parameters in adolescent athletes. Arch. Osteoporosis 13:128. doi: 10.1007/s11657-018-0543-4
Keywords: football, plyometric exercise, post-activation performance enhancement, physical education and training, resistance training, sports
Citation: Thapa RK, Lum D, Moran J and Ramirez-Campillo R (2021) Effects of Complex Training on Sprint, Jump, and Change of Direction Ability of Soccer Players: A Systematic Review and Meta-Analysis. Front. Psychol. 11:627869. doi: 10.3389/fpsyg.2020.627869
Received: 10 November 2020; Accepted: 24 December 2020;
Published: 22 January 2021.
Edited by:
Miguel-Angel Gomez-Ruano, Polytechnic University of Madrid, SpainReviewed by:
Souhail Hermassi, Qatar University, QatarTomás T. Freitas, Catholic University San Antonio of Murcia, Spain
Copyright © 2021 Thapa, Lum, Moran and Ramirez-Campillo. This is an open-access article distributed under the terms of the Creative Commons Attribution License (CC BY). The use, distribution or reproduction in other forums is permitted, provided the original author(s) and the copyright owner(s) are credited and that the original publication in this journal is cited, in accordance with accepted academic practice. No use, distribution or reproduction is permitted which does not comply with these terms.
*Correspondence: Rodrigo Ramirez-Campillo, ci5yYW1pcmV6JiN4MDAwNDA7dWxhZ29zLmNs orcid.org/0000-0003-2035-3279