- Psychology and Brain Science, Boston University, Boston, MA, United States
This hypothesis is that anorexia nervosa (AN) is a biologically driven disorder, and mammalian hibernation may offer clues to its pathogenesis. Using this approach, this hypothesis offers suggestions for employing heart rate variability as an early diagnostic test for AN; employing the ketogenic diet for refeeding patients, attending to omega 3:6 ratio of polyunsaturated fatty acids (PUFAs) in the refeeding diet; and exploring clinical trials of the endocannabinoid-like agent, palmitoylethanolamde for patients with AN. This hypothesis also explores the role of lipids and autoimmune phenomena in AN, and suggest a lipodomics study to search for antibodies in the serum on patients with AN.
Introduction
Anorexia nervosa (AN) is a severe disorder characterized by self-starvation, hyperactivity, and distorted body image (Kaye et al., 2009; American Psychiatric Association, 2013). The onset is often around puberty, and it is more common in females than males. The illness is difficult to treat, is frequently marked by relapses, and has the highest mortality of any psychiatric illness. It is associated with a high co-morbidity with substance abuse, especially alcoholism (Zipfel et al., 2000; Root et al., 2010). Recent data suggest patients with AN have an increased incidence of autoimmune disorders (Raevuori et al., 2014; Zerwas et al., 2017).
The pathogenesis and etiology are unknown, and thus developing effective treatments is very challenging. The framework for understanding the disorder has evolved from a primarily psychodynamic conflict (Bruch, 1978); to manifestation of Western Cultural pressure to be thin (Ahern et al., 2008); to a complex neurobehavioral disorder with strong evidence for underlying genetic vulnerability (Kaye et al., 2013; Yilmaz et al., 2015).
In 2003 Gusinger, advanced a very interesting hypothesis titled, “Adapted to Flee Famine: Adding an Evolutionary Perspective on Anorexia Nervosa” (Guisinger, 2003). She noted:
“The consensus of the field is that AN develops when psychopathology and social pressures to be thin act in concert with biological vulnerability. What if researchers have assumed the wrong direction of causality? Several lines of evidence, considered together, suggest that rather than psychological or medical pathology causing the bizarre behaviors and cognitions of AN, it is weight loss that leads to the symptoms. If the interpretation of the direction of causality is reconsidered, a number of discordant observations fall into place. The symptoms of AN are proposed to be biological responses to low body weight. AN is likely to result from a complex of adaptations selected in the prehistoric past when humans lived as sparsely distributed nomadic foragers. Although no longer adaptive, some individuals' bodies respond to very low body weight (caused by dieting or any reason) as though they must migrate from famine conditions” (Guisinger, 2003).
It is notoriously difficult to explore the circumstances at the onset of AN, because usually many months pass between the earliest changes in eating and the diagnosis of AN (Cottee-Lane et al., 2004). Although many qualitative studies have address the patients' experience of treatment for AN, there is a marked absence of reports of how the disorder took hold (Espindola and Blay, 2009; Bezance and Holiday, 2013; Sibeoni et al., 2017). Several books by recovered patients support the concept that benign weight loss preceded the psychological symptoms, rather than vice versa (Hornbacher, 1998; Arnold, 2013). Carrie Arnold, a science reporter who is also in recovery from AN noted:
“I spent the fall semester of my junior year studying in Scotland and returned carrying wonderful memories, gifts for all and yes, five extra pounds…I began the spring of my junior year with an innocent, earnest desire to work out regularly and shed those five pounds…Ten ponds came off before I even noticed…Looking back, I realized that I can say: there. That's when I first became anorexic. I wasn't clinically underweight at that point, but I was unable to start eating on my own, I was terrified of gaining weight, and I was unable to see what the issue was.” (Arnold, 2013).
With the hope that a radical reframing of the illness might open new avenues of treatment, this author makes the presumption that cultural and psychological issues make dieting more prevalent, but the etiology of AN is biologically driven. Another assumption is that weight loss from any cause including benign dieting, activates a series of metabolic signals that cascade into self-starvation, and hyper-exercise in genetically susceptible individuals.
Metaphors draw attention to similarities between two separate, seemingly unrelated entities, and while innately oversimplified, can be powerful tools to illuminate and clarify issues that otherwise are lost in complexity. In that spirit, and borrowing from Gusinger's hypothesis, this author offers a metaphor between AN and mammalian hibernation (Scolnick and Mostofsky, 2014). The latter is an extreme metabolic adaptation to food depletion and cold, and AN is a metabolic mal-adaptation to transient decreased food intake. Much about hibernation remains a mystery, but it is becoming clear that the signals to enter hibernation in the autumn, and emerge in the spring are not dependent on unique metabolic pathways. Rather, hibernation signals use the same metabolic pathways present in all mammals, including humans (Schwartz and Andrews, 2013).
While not claiming this is the “whole picture” this metaphor is consistent with some features of AN—the geographic pattern showing high prevalence in temperate climates, and rarity in the tropics (Njenga and Kangether, 2004; Hoek et al., 2005; Vazquez et al., 2006; Gutierrez et al., 2017), the subtle worsening in winter months (Favaro and Santonastaso, 2009; Fraga et al., 2015), the frequently observed hypothermia (Nishita et al., 1985), and the tendency for many patients to cross over from pure restriction to binging which resembles the hibernating mammal's seasonal pattern of binging in late summer and autumn followed by starvation in winter (Eddy et al., 2008).
Methodology
This is presented solely as a hypothesis andz is not an exhaustive literature review. In a sense the methodology was to approach the disorder of AN with no preconceptions except the assumption that It is biologically driven, then to read widely, and to see if any coherent themes emerged from disparate areas. The hibernation theory emerged after reading the literature on changes in fatty acids in AN, and noting similar studies in hibernation. Public Med was the only source used, and the method for finding studies was totally theoretical. Oftentimes one article would lead to many more. Some topics, such as heart rate variability in AN, ketogenic diet in AN, or endocannabinoid changes in patients with AN had less than 20 articles, thus all papers referenced in Public Med were reviewed, and all the relevant articles are referenced in the text. Other topics, such as the physiology of endocannabinoids (McPortland et al., 2014; Maccarrone et al., 2015), eicosanoids (Dennis and Norris, 2015), soluble epoxide hydrolase (sEH) (Swardfager et al., 2018), and lipodomic analysis (Sethis and Brietzke, 2017) have a vast literature. For the interested reader, there are current recent reviews of these fields, and this author is not an expert on these areas.
Bradycardia in Hibernation and Heart Rate Variability in AN
It has long been known that black bears are immobile during hibernation, but if disturbed can wake up in seconds, and mount a vigorous defense. Elegant research implanting bears with pacemakers before releasing them back in the wild, have documented that extreme bradycardia is the norm while hibernating (Laske et al., 2011). Heart rates average 8 beats/min in winter and 130 beats/min in the summer. The longest period of asystole was 14 s with a breathing rate of 1.53 breaths/min. Respiratory sinus arrythmia dampening proved to be a very sensitive measure of arousal, noted before any behavioral change or increase in pulse. Respiratory sinus arrhythmia refers to the oscillations between breathing and heart rate, which are present in all mammals (Grossman and Taylor, 2007). Normally heart beats increase during inspiration and decrease during expiration. Exaggeration of this pattern reflects increased vagal tone, and correlates with bradycardia. Statistical analysis of the oscillations formed by plotting breathing rate and heart rate over time, derived from a 15 min EKG, is a field of physiology known as heart rate variability (Billman et al., 2015). Heart rate variability is a more sensitive indicator of vagal tone than simply measuring the pulse.
Clinically, it is widely known that patients with AN demonstrate bradycardia, with estimates that 70% of patients show heart rates <50 beats/min at presentation (Yaham et al., 2013). At least 15 studies have examined heart rate variability parameters in patients with AN, and almost all found high values for respiratory sinus arrhythmia (Galetta et al., 2003; Cong et al., 2004; Platisa et al., 2006; Muraldo et al., 2007; Dhoble et al., 2008; Ishizawa et al., 2009; Mazurak et al., 2011a; Palova et al., 2012; Jacoangeli et al., 2013; Bomba et al., 2014). The few studies that did not find elevated respiratory sinus arrythmia, included patients in various states of recovery (Vigo et al., 2008). A recent literature review noted the lack of consistency and standardized practices in measuring heart rate intervals, yet the general finding that AN is a state of increased RSA is strong (Mazurak et al., 2011b). These findings are a bit of a paradox because, in many studies, higher heart rate variability and respiratory sinus arrythmia has been associated with improved cardiac health (Buccelleti et al., 2009), general good health (Tsuhi et al., 1994), and emotional resilience (Hildebrandt et al., 2016). It is interesting that oftentimes patients with AN superficially appear to be healthy, especially at the onset of the disorder before the cachexia becomes evident. Heart rate variability might be a useful tool in diagnosing early onset AN.
Ketogenic Diet and AN
In mammals, glucose is the major source for cellular fuel under most conditions, but in states where cellular glucose is insufficient such as untreated diabetes mellitus, fasting, semi-starvation, extreme exercising, and notably hibernation, the metabolism switches to using more fat (Andrews et al., 2009). In these situations, fatty acids are broken down in the mitochondria into acetyl Co-A, which enters the Krebs cycle to produce cellular energy. The liver begins to synthesize beta hydroxyacetate, acetoacetate, and acetone from the excess acetyl-CoA (see Figure 1). These three compounds are collectively called ketone bodies. It has recently been discovered that astrocytes also have the capacity to generate ketones (Le Foll and Levin, 2016). An elegant and daring study by Owens and Cahill in 1967 of in-hospital monitoring of three obese patients during a 5–6 week fast, including multiple catheterization of liver and brain, demonstrated that after 3 days of starvation the metabolism of the brain undergoes radical transformation (Owen et al., 1967). Beta hydroxybutyrate and acetoacetate replace glucose as the primary fuel.
Patients with AN, subsist on a semi-starvation diet, and often have elevated serum ketones. This raises the issue of whether the state of ketosis might be metabolically, and psychologically rewarding for patients (Scolnick, 2017). This would be important because it is possible to reproduce the ketotic state without starvation, by invoking the ketogenic diet.
The ketogenic diet is a very high fat, low carbohydrate diet that continues to provide adequately nourishment while the metabolism becomes ketosis driven. It replicates starvation, but the individual does not starve. It has great scientific interest because it is an effective treatment for pediatric seizures (Wilder, 1921; Freeman et al., 2009), and has proven to be safe and surprisingly well-tolerated, when both the child and parent see the results (Freeman et al., 2007). Recently small pilot studies suggest it might be effective for neurodegenerative disorders such as Parkinsons and Alzheimers diseases (Vanitallie et al., 2005; Henderson, 2008).
Two animal clinical trials, one from 1971 and one from 2008 demonstrated that a very high fat diet interrupts self-starvation in a rodent model of anorexia (Barbotiak and Wilson, 1971; Brown et al., 2008). The activity based anorexia model, although not perfect, is considered the best animal model because it replicates the self-starvation, hyperactivity, and changes in leptin, ghrelin, and cortisol seen in human AN (Gutierrez, 2013). The model is induced by housing the rodent in a cage with ad lib access to a running wheel, and exposing it to slightly restricted feeding times. The rodent who develops activity based anorexia starts to run excessively, lose weight, and self-starve.
There are no human trials of the ketogenic diet in patients with AN.
Omega 3: Omega 6 Ratios in Hibernators and in AN
Current research shows that lipid pathways especially polyunsaturated fatty acids (PUFAs) are major signaling agents in hibernation (Geiser and Kenagy, 1987; Geiser, 1990; Hill and Florant, 2000; Gerson et al., 2008; Ruf and Arnold, 2008; Arnold et al., 2011). For decades, isolated clinical and research studies have reported abnormalities in lipid metabolism in patients with AN (Halmi and Fry, 1974; Holman et al., 1995; Curatola et al., 2004; Zák et al., 2005; Swenne et al., 2011). Within the framework of the metaphor, this author presents a highly speculative theory that lipid pathways are key to causing and perhaps treating AN.
A brief review of the complex biochemistry of lipids, shows that the building blocks for most complex lipids are the fatty acids, which are classified as short-chain if there are 2–6 carbons; medium-chain if there are 6–12 carbons; or long-chain if there are 14 or more carbons. They are further classified by the number of double bonds, which gives the chain more flexibility. Saturated fatty acids have no double bonds; monounsaturated fatty acids have one double bond; and PUFAs have more than one double bond (see Figure 2).
The PUFAs can be divided into several families, based on where along the carbon chain, the double bonds occur (see Table 1). These are referred to as omega families, “omega” signifying “backwards” because the carbon at the methyl end of the chain is numbered carbon 1, which is backward from classical chemical numbering which numbers the carbons from the acidic end (Holman, 1964). The omega 3 fatty acids first double bond is at the third carbon; the omega 6 fatty acids double bonds start at carbon 6. Two of these fatty acids are essential in humans, alpha linolenic acid from the omega 3 family, and linoleic acid from the omega 6 family. These essential fatty acids must be obtained from the diet. A series of elongation and desaturation enzymes act on these fatty acids to derive the remainder of the fatty acids in both families. Longer chain PUFAs can also be obtained from the diet, but because they can also be metabolically synthesized, they are not considered essential.
Mammals cannot convert omega 3 fatty acids to omega 6, and vice versa. Thus, a constant theme in PUFA metabolism is the competition between the omega 3 and omega 6 families, as they share the same metabolic pathways.
The ratio remains an intriguing window into metabolic changes, and once again it is worthwhile to examine the hibernating animal. Several studies have noted dramatic seasonal oscillations in serum fatty acids, with respect to degree of saturation, and the ratio of omega 3:6 fatty acids in hibernators (Ruf and Arnold, 2008; Arnold et al., 2011). In late autumn, when the weather cools, the phospholipid makeup of cellular membranes in the heart become enriched with omega 3 highly unsaturated fatty acids, and when the animal emerges from hibernation the ratio reverses. An evolutionary highly conserved adaptation to ambient cold, seen from bacteria to mammals is that the cell membrane maintains its viscosity by altering proportions of fatty acids (Ernst et al., 2016).
Two studies, one of normal adults living in Antwerp Belgium, and another of patients with seasonal affective disorder in California have shown humans also experience seasonal variation in PUFAs and omega 3:6 ratios (DeVriese et al., 2004; Hennebelle et al., 2017). In both these studies the oscillations were much more subtle than those seen in hibernators, but nevertheless present.
As far as AN, multiple studies have consistently demonstrated very profound distortions in serum PUFA profiles, compared to normal, although there are no studies focusing on seasonal changes. Interestingly, most of these have found a skewing toward more omega 3 fatty acids over omega 6, which is opposite from changes seen in populations eating the Western diet. This might reflect the preference for salads, and avoidance of meat, observed in many patients with AN.
In the last few decades there has been a lot of discussion about how the Western diet has become skewed from our traditional diet, and this might be contributing to heart disease, diabetes, obesity, and other disorders (Simopoulous, 2016). Omega 3 fatty acids are plentiful in green leafy vegetables, and wild fish; whereas seeds and meats are rich in omega 6 fatty acids. Many studies have shown that the Western diet has shifted from a balanced omega 3:6 ratio to one that heavily favors omega 6 fatty acids over the omega 3 family (Blasbalg et al., 2017). At one point, there was enthusiasm that supplementing the Western diet with pills containing omega 3 fish oils would lead to improved cardiac health. As larger public health studies have been conducted, there are more negative results, thus unhappily confirming that lipid metabolism is complex, and simply resupplying a fatty acid, akin to vitamin replacement for classical nutritional deficiency disorders is not effective (Rice et al., 2016). Similarly, a pilot trial of omega-3 supplementation with patients with AN was disappointing although there was a possible mild decrease in anxiety (Ayton et al., 2004).
Shih et al. has focused on the omega 3:omega 6 refeeding ratio in patients with AN with a nuanced approach targeting a specific enzyme named sEH (Shih et al., 2017).
To understand the implications of this pathway, Figure 3 summarizes the complex web of PUFA enzyme oxidation products. There are three known oxidative enzymes:
cyclooxygenase(COX);
lipooxenase (LOX);
cytochrome P-450 enzymes (CYP).
As shown in Figure 3, these enzymes act upon the PUFAs (in Figure 3 arachidonic acid is depicted), and form a myriad of compounds commonly known as the prostaglandins and leukotrienes. These products are present in most cells of the body and are locally active and short acting. It was originally thought that the 20-carbon omega 6 PUFA, arachidonic acid was the precursor to all the compounds in this family, and this pathway is therefore often referred to as the eicosanoid (eicos is Greek for 20) pathway. Recent work suggests many PUFAs from both the omega 3 and omega 6 families are subject to enzymatic oxidation (Spector and Kim, 2015). The innate competition between the omega 6 and omega 3 families is important in this area, as it is becoming clear that different PUFAs are oxidized to different prostaglandins. The different prostaglandins have different properties which are frequently antagonistic; some are inflammatory while others are anti-inflammatory; some are vasoconstrictor and others are vasodilators.
In 2014, a preliminary genetic study discovered that a gene coding for sEH protein was associated with an increased risk of AN (Zeeland et al., 2014). Soluble epoxide hydrolase (sEH) acts on epoxy eicosatienoic acids, transforming them into diols. As shown in Figure 3, epoxy eicosatienoic acids, in turn are produced when PUFA are oxidized via the Cytochrome P450 pathway. Shih performed a lipodomic and metabolomic comparison of the serum from 36 healthy controls, 30 ill patients with AN, and 30 “recovered” patients with AN, measuring PUFAS as well as 80 oxidation products (Shih et al., 2016). Results showed that ill patients had high sEH product/substrate ratios implying increased activity; and “recovered” patients also showed increased sEH activity compared to controls. She also noted that omega 6:omega 3 PUFA profile was lower in ill patients than in controls, and that the ratio was inversely associated with anxiety in patients with AN. She is thus exploring whether the epoxide and subsequent diol derived from omega 6 PUFAs have different physiology from the epoxide and subsequent diol derived from the omega 3 PUFAs, and whether this can cause the patient to avoid certain foods that promote a subtle inflammatory state (Shih, 2017).
The Endocannabinoid System (ECS)
The endocannabinoid system is also a byproduct of PUFA metabolism, although the discovery was independent of the prostaglandin pathways. Raphael Mechoulan's laboratory isolated and chemically characterized tetrahydrocannabinoid (THC) as the active ingredient in marijuana in 1964 (Mechoulam et al., 1967) (see Figure 4A), but it was not until 1998 that THC was demonstrate to stimulate specific receptors in the brain and periphery of humans (Devane et al., 1988). These receptors were characterized and labeled cannabinoid receptor 1 (CB1) and cannabinoid receptor 2 (CB2). Subsequently, it was found that endogenous compounds derived from arachidonic acid were able to also stimulate these identical receptors (Devane et al., 1992; Mechoulam et al., 1995). These “endocannabinoids” known chemically as arachidonoylethanolamde (AEA) or more commonly referred to as anandamine (Sanskrit for bliss) (see Figure 4B); and 2-arachidonooylglycerol (2AG) (see Figure 4C) are very different chemically from tetrahydrocannabinol (THC), the active agent in marijuana. While only anandamide and 2AG are known to stimulate the CB1 and CB2, other fatty acid derived ethanolamides (see Figures 4E,G) act in concert with anandamine and 2AG, and thus are referred to as “endocannabinoid-like agents” (Ho et al., 2008). It is important to note that the classic endocannabinoids, and the “endocannbinoid-like agents” are derived from the polyunsaturated fatty acids, arachidonic acid (see Figure 4D) and palmitic acid (see Figure 4F) respectively.
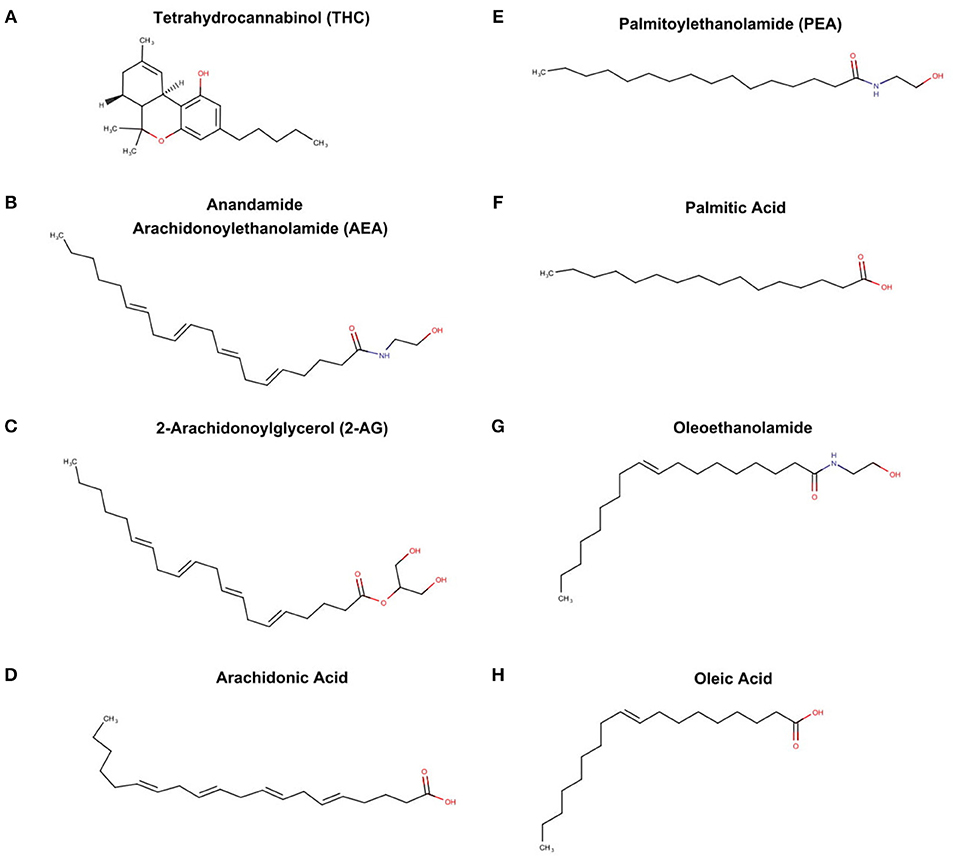
Figure 4. THC from marijuana plant, endocannabinoid, and endocannabinoid-like agents (A) tetrahydrocannabinoid (THC), (B) anandamide arachidonoylethanolamide (AEA), (C) 2-arachidonoylglycerol (2-AG), (D) archidonic acid, (E) palmitoylethanolamide, (F) palmitic acid, (G) oleoethanolamide, and oleic acid (H).
Analogous to prostaglandins, these compounds act locally, are short acting, and set in motion myriad and sometimes antagonistic effects. While much remains unknown, it is clear the ECS is intimately involved with very basic physiology such as circadian rhythms, feeding behaviors, pain sensation, and reward feelings (Vaughn et al., 2010; Lu and Mackie, 2018). Adding to the complexity, anandamine, and 2AG are subject to oxidation by the same enzymes that are part of the eicosanoid pathways, and thus there is potential for tremendous cross-talk between prostaglandins and endocannabinoids (Rouzer and Marnett, 2011).
Monteleone et al. has examined the ECS in patients with AN (Monteleone et al., 2005, 2012, 2015). The pattern of serum endocannabinods differs between normal controls, patients in recovery from anorexia, and patients with acute anorexia, especially when subjects were presented with a task of eating a favorite food when sated, which is described as hedonic eating.
A recent encouraging study, employing the activity based anorexia model found that pretreatment of rats with THC or a synthetic CB1 agonist significantly attenuated the excess running, and starvation in the affected rodents and, normalized leptin and cortisol profiles (Scherma et al., 2017).
Because the ECS is complex, and not completely mapped there is a great deal of risk using pharmaceuticals to manipulate the system. This is illustrated by the case of Rimonobant, a CB1 antagonist that had proven effective in preliminary trials in inducing weight loss in obese patients (Sam et al., 2011). Although it was never approved in the United States, it was marketed in Europe in 2006, and removed worldwide in 2008 when a significant increased incidence of depression and suicide was noted in patients prescribed Rimonobant.
Palmitoylethanolamide, commonly called PEA is a fatty-acid-ethanaolamide derived from the 16 carbon saturated palmitic acid that is plentiful in palm oil. PEA was isolated from egg yolks in 1957, and reports of its use medically for various inflammatory conditions date from the late 1960s (Hesselink, 2013). It is produced in Europe as a regulated nutraceutical, and easily available on the internet. While some have advocated for pilot studies in patients with AN, there have been no clinical trials (Scolnick, 2018).
Phospholipids Oxidation Products as Epitopes for Auto Antibodies
There has long been a clinical impression that many patients with AN develop autoimmune diseases. Two recent epidemiological studies from Scandinavia confirm the association of autoimmune disorders and AN. A 2014 study from Helsinki followed all 2,342 adolescents hospitalized for eating disorders from 1995–2011 and found 8.9% developed an autoimmune disorder compared to an expected 5.4% (Zerwas et al., 2017). A study from Denmark following 1 million children and adolescents found that adolescents with AN had a 64% higher hazard ratio for autoimmune/inflammatory disease than normal controls (Raevuori et al., 2014).
Clinically, a possible association has been noted between pediatric autoimmune disorder associated with streptococcus (PANDAS) and isolated cases of AN (Calkin and Carandan, 2007). The proposed pathophysiology is that antibodies develop to the streptococcus and cross-react with brain tissue, similar to the situation of acute chorea associated with rheumatic fever. Likewise, clinicians have commented on the co-occurrence of AN and systemic lupus erythematosis (Toulany et al., 2014).
A genome-wide association study (GWAS) comprising 3,495 AN cases and 10,982 controls, found one highly significant locus on chromosome 12 (rs4622308) which has been noted on GWASs for rheumatoid arthritis and type 1 diabetes; and regions surrounding the locus region has been implicated in other autoimmune disorders (Duncan et al., 2017).
The evidence for autoimmune antibodies in AN is very preliminary. Fetissov et al. found that patients with AN and bulimia nervosa have autoantibodies against two hypothalamic peptides involved in hunger/satiety; alpha-melanocyte-stimulating-hormone and ACTH, in a pattern that differs from normal controls (Fetissov et al., 2002). They also noted that anxiety and obsessions, core traits of ill patients with eating disorders, correlate with the level of autoantibodies in the serum (Fetissov et al., 2005).
It is intriguing to imagine that 1 day AN might be managed as an autoimmune disorder. While highly speculative, we note the emerging science linking oxidation products of PUFAs to pathological autoantibodies. Briefly, in addition to enzyme-linked PUFA oxidation, via the COX, LOX, and CYP enzyme systems, lipids are also subject to oxidation by non-enzymatic reactions. These reactions tend to be self-generating and lead to formation of lipid hydroperoxides, and reactive aldehydes, which can react with neighboring proteins (Kurien et al., 2006). It has been suggested that these oxidation-derived-protein complexes, can function as epitopes (antibody-inducers) and lead to antibodies against self-proteins. Using a murine model of systemic lupus erythemtosis, Otaki et al. found antibodies that cross reacted both to DNA, which is the hallmark diagnostic test for lupus, and an aldehyde product arising from peroxidation of an omega 6 PUFA (Otaki et al., 2010). Quintana et al. recently reported on using lipodomic analysis and antigen microarrays to discover autoantibodies to lipid products that can be used as biomarkers for multiple sclerosis (Quintana et al., 2012).
Linking PUFA abnormalities in patients with AN to autoimmune processes has not been investigated.
Implications of the Hypotheses
This speculative hypothesis, focusing on an analogy between AN and rogue hibernation has given rise to four specific suggestions for exploratory studies.
First, a pilot study comparing the HRV of patients with new onset AN with normal healthy fit adolescents might lead to a non-invasive test for early diagnosis and screening of at-risk populations such as student athletes.
Second, a pilot trial of ketogenic diet in refeeding from AN is suggested. The animal data obtained from activity based anorexia model is encouraging, and the diet is in clinical use for pediatric seizures, and is safe for this population.
Third, a pilot trial of palmitoylethaloamide, an endocannabinoid-like agent, in patients with AN is also suggested. Data from the activity based animal model is very encouraging, although THC and a synthetic CB1 agonist were used, not palmitoylethanolamide. As noted, synthetic agents commonly have untoward effects, and would require extensive study, but palmitoylethanolamide has a long track record of safety.
Fourth, a pilot study of serum from patients with acute AN, recovered AN, and normal controls using lipidomics and antigen arrays, akin to the referenced study of patients with multiple sclerosis, might lead to identification of biomarkers.
Returning to the analogy with hibernation, there are currently efforts underway to apply the techniques of lipidomics, genomics, and proteinomics to decipher the metabolic signals of hibernation (Vermillion et al., 2015). It might be a very opportune time to share information across divergent fields of study in an attempt to find treatments for AN.
Author Contributions
The author confirms being the sole contributor of this work and has approved it for publication.
Conflict of Interest Statement
The author declares that the research was conducted in the absence of any commercial or financial relationships that could be construed as a potential conflict of interest.
References
Ahern, A. L., Bennett, K. M., and Hetherington, M. M. (2008). Internalization of the ultra-thin ideal: positive implicit associations with underweight fashion models are associated with drive for thinness in young women. Eat. Disord. 16, 294–307. doi: 10.1080/10640260802115852
American Psychiatric Association (2013). Diagnostic and Statistical Manual of Mental Disorders, 5th Edn. Washington DC: American Psychiatric Association.
Andrews, M. T., Russeth, K. P., Drewes, L. R., and Henry, P. G. (2009). Adaptive mechanisms regulate preferred utilization of ketones in the heart and brain of a hibernating mammal during arousal from torpor. Am. J. Physiol. Regul. Integr. Comp. Physiol. 296, R383–R393. doi: 10.1152/ajpregu.90795.2008
Arnold, C. (2013). Decoding Anorexia: How Breakthroughs in Science Offer Hope for Eating Disorders. New York, NY; East Sussex, London: Routledge; Taylor & Francis.
Arnold, W., Ruf, T., Frey-Roos, F., and Bruns, U. (2011). Diet independent remodeling of cellular membranes precedes seasonally changing temperature in a hibernator. PLoS ONE 6:e18641. doi: 10.1371/journal.pone.0018641
Ayton, A. K., Azaz, A., and Horrobin, D. F. (2004). A pilot open case series of ethyl-EPA supplementation in the treatment of anorexia nervosa. Prostaglandins Leukot. Essent. Fatty Acids 71, 205–209. doi: 10.1016/j.plefa.2004.03.007
Barbotiak, J. J., and Wilson, A. S. (1971). Effect of diet on self starvation in the rat. J. Nutr. 102, 1543–1536. doi: 10.1093/jn/102.11.1543
Bezance, J., and Holiday, J. (2013). Adolescents with anorexia nervosa have their say: a review of qualitative recovery from anorexia nervosa. Eur. Eat. Dis. Rev. 21, 352–360. doi: 10.1002/erv.2239
Billman, G. E., Huikuir, H. V., Sacha, J., and Trimmer, K. (2015). An introduction to heart rate variability; methodological consideration and clinical applications. Front. Physiol. 6:55. doi: 10.3389/fphys.2015.00055
Blasbalg, T. L., Hibbein, J. R., Ramsden, C. E., Majchrzad, S. F., and Rawlings, R. R. (2017). Changes in consumption of omega 3 and omega 6 fatty acids in the United States during the 20th century. Am. J. Clin. Nutr. 93, 950–962. doi: 10.3945/ajcn.110.006643
Bomba, M., Corbetta, F., Gambera, A., Nicosia, F., Bonini, L., Neri, F., et al. (2014). Heart rate variability in adolescents with functional hypothalamic amenorrhea and anorexia nervosa. Psychiatry Res. 215, 400–409. doi: 10.1016/j.psychres.2013.11.012
Brown, A. J., Avena, N. M., and Hoebel, B. G. (2008). A high fat diet prevents and reverses the development of activity-based anorexia in rats. Int. J. Eat. Disord. 41, 383–389. doi: 10.1002/eat.20510
Bruch, H. (1978). The Golden Cage: The Enigma of Anorexia Nervosa. Cambridge, MA: Harvard University Press.
Buccelleti, E., Gilard, E., Scaini, E., Galluto, L., Oersiani, R., Biondi, A., et al. (2009). Heart rate variability and myocardial infarction; systematic literature review and meta-analysis. Eur. Rev. Ed. Pharmacol. Sci. 13, 299–307.
Calkin, C. V., and Carandan, C. G. (2007). Clinical case rounds in child and adolescent psychiatry: certain eating disorders may be a neuropsychiatric manifestation of PANDAS: case report. J. Can. Acad. Child Adolesc. Psychiatry. 16, 132–135.
Cong, N. D., Saikawa, T., Ogawa, R., Hara, M., Takahashi, N., and Sakata, T. (2004). Reduced 24-hour ambulatory blood pressure and abnormal heart rate variability in anorexia nervosa. Heart 90, 563–564. doi: 10.1136/hrt.2003.024356
Cottee-Lane, D., Pistrang, N., and Bryant-Waugh, R. (2004). Childhood onset anorexia nervosa; the experience of parents. Eur. Eat. Disord. Rev. 12, 169–177. doi: 10.1002/erv.560
Curatola, G., Camilloni, M. A., Vignini, A., Nanetti, L., Boscaro, M., and Mazzanti, L. (2004). Chemical physical properties of lipoproteins in anorexia nervosa. Eur. J. Clin. Invest. 34, 747–751. doi: 10.1111/j.1365-2362.2004.01415.x
Dennis, E. A., and Norris, P. C. (2015). eicosanoid storm in infection and inflammation. review article. Nat. Rev. Immunol. 5, 511–523. doi: 10.1038/nri3859
Devane, W. A., Dysarz, F., Johnson, M., Melvin, L., and Howlett, A. (1988). Determination and characterization of a cannabinoid receptor in rat brain. Mol. Pharmacol. 34, 605–613.
Devane, W. A., Hanus, L., Breuer, A., Pertwee, R., Stevenson, L., Griffin, G., et al. (1992). Isolation and structure of a brain constituent that binds to the cannabinoid receptor. Science 258, 1946–1949. doi: 10.1126/science.1470919
DeVriese, S. R., Christopher, A. B., and Maes, M. (2004). In humans, the seasonal variation in polyunsaturated fatty acids is related to the seasonal variation in violent suicide and serotonergic markers of violent suicide. Prostaglandins Leukot. Essent. Fatty Acids 71, 13–18. doi: 10.1016/j.plefa.2003.12.002
Dhoble, A., Puttarajappa, C., Patel, M. B., and Thakur, R. K. (2008). Heart rate variability in a man with anorexia. Am. J. Med. 121, e5–e6. doi: 10.1016/j.amjmed.2008.07.020
Duncan, L., Yilmaz, Z., Gaspar, H., Walter, R., Goldstein, J., Antila, V., et al. (2017). Significant locus and metabolic genetic correlations revealed in genome-wide association study of anorexia nervosa. Am. J. Psychiatry 174, 850–858. doi: 10.1176/appi.ajp.2017.16121402
Eddy, K. T., Dorer, D. J., Franko, D. L., Tahilani, K., Thompson-Brenner, H., and Herzog, D. B. (2008). Diagnostic crossover in anorexia nervosa and bulimia nervosa: implications for DSM-V. Am. J. Psychiatry 165, 245–250. doi: 10.1176/appi.ajp.2007.07060951
Ernst, R., Ejsing, C. S., and Antonny, B. (2016). Homeoviscous adaptation and the regulation of membrane lipids. J. Mol. Biol. 428, 4776–4791. doi: 10.1016/j.jmb.2016.08.013
Espindola, C. R., and Blay, S. L. (2009). Anorexia treatment from the patient perspective: a meta-analysis of qualitative studies. Ann. Clin. Psychiatry 21, 38–48.
Favaro, A., and Santonastaso, P. (2009). Seasonality and the prediction of weight at resumption of menses in anorexia nervosa. Fertil. Steril. 91, 1395–1397. doi: 10.1016/j.fertnstert.2008.04.055
Fetissov, S. O., Hallman, J., Oreland, L., Af Klinteberg, B., Grenbäck, E., Hulting, A. L., et al. (2002). Autoantibodies against alpha MSH ACTH and LHRH in anorexia and bulimia nervosa patients. Proc. Natl. Acad. Sci. U.S.A. 99, 17155–17160. doi: 10.1073/pnas.222658699
Fetissov, S. O., Harro, J., Jaanisk, M., Järv, A., Podar, I., Allik, J., et al. (2005). Autoantibodies against neuropeptides are associated with psychological traits in eating disorders. Proc. Natl. Acad. Sci. U.S.A. 102, 14865–14870. doi: 10.1073/pnas.0507204102
Fraga, A., Caggianess, V., Carrera, O., Graell, M., Morande, G., and Gutierrez, E. (2015). Seasonal BMI differences between restrictive and purging anorexia nervosa subtypes. Int. J. Eat. Dis. 48, 35–41. doi: 10.1002/eat.22357
Freeman, J. M., Kossoff, E. H., Freeman, J. B., and Kelly, M. T. (2007). The ketogenic Diet; A Treatment for Children and Others with Epilepsy, 4th Edn. New York, NY: Demos Medical Publishing.
Freeman, J. M., Vining, E. P., Kossoff, E. H., Pyzik, P. L., Ye, X., and Goodman, S. N. (2009). A blinded, crossover study of the efficacy of the ketogenic diet. Epilepsia 50, 322–325. doi: 10.1111/j.1528-1167.2008.01740.x
Galetta, F., Franzoni, F., Prattichizzo, F., Rolla, M., Santoro, G., and Pentimone, F. (2003). Heart rate variability and left ventricular diastolic function in anorexia nervosa. J. Adolesc. Health 32, 416–421. doi: 10.1016/S1054-139X(03)00048-X
Geiser, F. (1990). Influence of polyunsaturated and saturated dietary lipids on adipose tissue, brain, and mitochondrial membrane fatty acid composition of a mammalian hibernator. Biochim. Biophys. Acta 1046,159–166. doi: 10.1016/0005-2760(90)90183-X
Geiser, F., and Kenagy, G. J. (1987). Polyunsaturated lipid diet lengthens torpor and reduces body temperature in a hibernator. Am. J. Physiol. 252, R897–901. doi: 10.1152/ajpregu.1987.252.5.R897
Gerson, A. R., Brown, J. C. L., Thomas, R., Bernards, M. A., and Staples, J. F. (2008). Effects of dietary polyunsaturated fatty acids on mitochondrial metabolism in mammalian hibernation. J. Exp. Biol. 211, 2689–2699. doi: 10.1242/jeb.013714
Grossman, P., and Taylor, E. W. (2007). Toward understanding respiratory sinus arrhythmia: relations to cardiac vagal tone, evolution and biobehavioral functions. Biol. Psychol. 74, 263–285. doi: 10.1016/j.biopsycho.2005.11.014
Guisinger, S. (2003). Adapted to flee famine - adding an evolutionary perspective to anorexia nervosa. Psychol. Rev. 110, 745–761. doi: 10.1037/0033-295X.110.4.745
Gutierrez, E. (2013). A rat in the labyrinth of anorexia nervosa: contributions of the activity-based anorexia rodent model to the understanding of anorexia nervosa. Int. J. Eat. Disord. 46, 289–301. doi: 10.1002/eat.22095
Gutierrez, E., Carrera, O., Vazquez, R., Puime, A., Fraga, A., and Claver, E. (2017). The association of anorexia nervosa and climate revisited: a bibliometric perspective. Int. J. Emerg. Mental Health Hum. Resilien. 19, 1–7. doi: 10.4172/1522-4821.1000371
Henderson, S. T. (2008). Ketone bodies as a therapeutic for Alzheimers disease. Neurotherapeutics 5, 470–480. doi: 10.1016/j.nurt.2008.05.004
Hennebelle, M., Ooki, Y., Yang, J., Hammock, B. D., Levitt, A. J., Taha, A., et al. (2017). Altered soluble epoxide hydrolase-derived oxylipins in patients with seasonal major depression: an exploratory study. Psychiatry Res. 252, 94–101. doi: 10.1016/j.psychres.2017.02.056
Hesselink, J. M. (2013). Evolution in pharmacologic thinking around the natural analgesic palmitoylethanolamide: from nonspecific resistance to PPAR alpha agonist and effective nutraceutical. J. Pain Res. 6, 625–634. doi: 10.2147/JPR.S48653
Hildebrandt, L. K., McCall, C., Engen, H. G., and Singer, T. (2016). Cognitive flexibility, heart rate variability, and resilience predict fine-grained regulation during prolonged threat. Psychophysiology 53, 880–890. doi: 10.1111/psyp.12632
Hill, V. L., and Florant, G. L. (2000). The effect of a linseed oil diet on hibernation in yellow-bellied marmots. Physiol. Behav. 68, 431–437. doi: 10.1016/S0031-9384(99)00177-8
Ho, W. S. V., Barrett, D. A., and Randall, M. D. (2008). “Entourage” effect of N-palmitoylethanolamide and N-oleoylethanolamide on vasorelaxation to anandamide occur through TRPV1 receptors. Br. J. Pharmacol. 155, 837–846. doi: 10.1038/bjp.2008.324
Hoek, H. W., van Harten, P. N., Hermans, K. M., Katzman, M. A., and Matroos, G. E. (2005). The incidence of anorexia nervosa on Curacao. Am. J. Psychiatry 162, 748–752. doi: 10.1176/appi.ajp.162.4.748
Holman, R. T. (1964). Nutritional and metabolic interrelationships between fatty acids. Fed. Proc. 23, 1062–1067.
Holman, R. T., Adams, C. E., Nelson, R. A., Grater, S. J. E., Jaskiewicz, J. A., Johnson, S., et al. (1995). Patients with anorexia nervosa demonstrate deficiencies of selected essential fatty acids, compensatory changes in nonessential fatty acids and decreased fluidity of plasma lipids. J. Nutr. 125, 901–907.
Ishizawa, T., Yoshiuhi, K., Takimoto, Y., Yamamoto, Y., and Akabayashi, A. (2009). Heart rate and blood pressure variability and baroreflex sensitivity in patients with anorexia nervosa. Psychosom. Med. 70, 695–700. doi: 10.1097/PSY.0b013e31817bb090
Jacoangeli, F., Mezzasalma, F. S., Canto, G., Colica, C., De Lorenzo, A., and Iellamo, F. (2013). Baroreflex sensitivity and heart rate variability are enhanced in patients with anorexia nervosa. Int. J. Cardiol. 162, 263–264. doi: 10.1016/j.ijcard.2012.10.073
Kaye, W. H., Fudge, J. L., and Paulus, M. (2009). New insights into symptoms and neurocircuit function of anorexia nervosa. Nat. Rev. Neurosci. 101, 573–584. doi: 10.1038/nrn2682
Kaye, W. H., Wierenga, C. E., Bailer, U. F., Simmons, A. N., and Bischoff-Grethe, A. (2013). Nothing tastes as good as skinny feels: the neurobiology of anorexia nervosa. Trends Neurosci. 36,110–120. doi: 10.1016/j.tins.2013.01.003
Kurien, B. T., Hensley, K., Bachmann, M., and Scofield, R. H. (2006). Oxidatively modified auto antigens in autoimmune disease. Free Radic. Biol. Med. 41, 549–556. doi: 10.1016/j.freeradbiomed.2006.05.020
Laske, T. G., Garshelis, D. L., and Iaizzo, P. A. (2011). Monitoring the wild black bear's reaction to human and environmental stressors. BMC Physiol. 11:13. doi: 10.1186/1472-6793-11-13
Le Foll, C., and Levin, B. E. (2016). Fatty acid induced astrocyte ketone production and the control of food intake. Am. J. Physiol. Regul. Integr. Comp. Physiol. 310, R1186–1192. doi: 10.1152/ajpregu.00113.2016
Lu, H. C., and Mackie, K. (2018). An introduction to the endogenous cannabinoid system. Biol. Psychiatry 79, 516–525. doi: 10.1016/j.biopsych.2015.07.028
Maccarrone, M., Bab, I., Biro, T., Cabral, G. A., Dey, S. K., Di Marzo, B., and Mechoulam, R. (2015). Endocannabinoid signaling at the periphery: 50 years after THC. Trends Pharmacol. Sci. 36, 277–296. doi: 10.1016/j.tips.2015.02.008
Mazurak, N., Enck, P., Muth, E., Teufel, M., and Zipfel, S. (2011b). Heart rate variability as a measure of cardiac autonomic function in anorexia nervosa: A review of the literature. Eur. Eat. Disord. Rev. 19, 87–99 doi: 10.1002/erv.1081
Mazurak, N., Stein, J., Kipphan, S., Muth, E. R., Teufel, M., Zipfel, S., and Enck, P. (2011a). Heart rate variability in anorexia nervosa and the irritable bowel syndrome. Neurogastroenterol. Motil. 23, e470–e478. doi: 10.1111/j.1365-2982.2011.01785.x
McPortland, J. M., Guy, G. W., and DiMarzo, V. (2014). Care and feeding of the endocannabinoid system: a systematic review of potential clinical interventions that upregulate the endocannabinoid system. PLoS ONE 9:e89566. doi: 10.1371/journal.pone.0089566
Mechoulam, R., Ben-Shabat, S., Hanus, L., Ligumsky, M., Kaminski, N. E., Schatz, A. R., et al. (1995). Identification of an endogenous 2-monoglyceride, present in canine gut, that binds to cannabinoid receptors. Biochem. Pharmacol. 50, 83–90. doi: 10.1016/0006-2952(95)00109-D
Mechoulam, R., Braun, P., and Gaoni, Y. (1967). A stereospecific synthesis of tetrahydrocannabinoids. J. Am. Chem. Soc. 89, 4552–4554. doi: 10.1021/ja00993a072
Monteleone, A. M., Di Marzo, V., Aveta, T., Pisciteeli, F., Dalle Grave, R., Scognamiglio, P., et al. (2015). Deranged endocannabinoid responses to hedonic eating in underweight and recently weight-restored patients with anorexia nervosa. Am. J. Clin. Nutr. 101, 262–269. doi: 10.3945/ajcn.114.096164
Monteleone, P., Maatias, I., Martiadis, V., De Petrocellis, L., Maj, M., and Di Marzo, V. (2005). Blood levels of the endocannainoid anandamide are increased in anorexia nervosa and in binge-eating disorder, but not in bulimia nervosa. Neuropsychopharmacology 30, 1216–1221. doi: 10.1038/sj.npp.1300695
Monteleone, P., Piscitelli, F., Scognamiglio, P., Monteleone, A. M., Canestrelli, B., Di Marzo, V., et al. (2012). Hedonic eating is associated with increased peripheral levels of ghrelin and the endocannainoid 2-arachidonoyl-glycerol in healthy humans: a pilot study. J. Clinic. Endocrinol. Metab. 97, E917–E924. doi: 10.1210/jc.2011-3018
Muraldo, G., Casu, M., Galchero, M., Bugnolo, A., Patrone, V., Cerro, P. F., et al. (2007). Alternations in the autonomic control of heart rate variability in patients with anorexia nervosa: correlations between sympathovagal activity, clinical features, and leptin. J. Endocrinol. Invest. 30, 356–362. doi: 10.1007/BF03346310
Nishita, J. K., Ellinwood, E. H., and Rockwell, W. J. K. (1985). Hypothermia in anorexia nervosa; an increase in nonshivering thermogenesis? Int. J. Eat. Disord. 4, 307–319.
Njenga, F. G., and Kangether, R. N. (2004). Anorexia nervosa in Kenya. East African Med. J. 81, 188–193. doi: 10.4314/eamj.v81i4.9153
Otaki, N., Chikazawa, M., Nagae, R., Shimozu, Y., Shibata, T., Ito, S., et al. (2010). Identification of a lipid peroxidation product as the source of oxidation-specific epitopes recognized by anti-DNA autoantibodies. J. Biol. Chem. 285, 33834–33842. doi: 10.1074/jbc.M110.165175
Owen, O. E., Morgan, A. P., Kemp, H. G., Sullivan, J. M., Herrera, M. G., and Cahill, G. F. (1967). Brain metabolism during fasting. J. Clin. Invest. 46, 1589–1595. doi: 10.1172/JCI105650
Palova, S., Havlin, J., and Charvat, J. (2012). The association of heart rate variability examined in supine and standing position ambulatory blood pressure monitoring in anorexia nervosa. Neuro. Endocrinol. Lett. 33, 196–200.
Platisa, M. M., Nestorovic, Z., Damjanovic, S., and Gal, V. (2006). Linear and non-linear heart rate variability measures in chronic and acute phases of anorexia nervosa. Clin. Physiol. Funct. Imag. 26, 54–60. doi: 10.1111/j.1475-097X.2005.00653.x
Quintana, F. J., Yeste, A., Weiner, H. L., and Covacu, R. (2012). Lipids and lipid-reactive antibodies as biomarkers for multiple sclerosis. J. Neuroimmunol. 248, 53–57. doi: 10.1016/j.jneuroim.2012.01.002
Raevuori, A., Haukka, J., Vaarala, O., Suvisaari, J. M., Gissler, M., Grainer, M., et al. (2014). The increased risk for autoimmune diseases in patients with eating disorders. PLoS ONE 9:e104845. doi: 10.1371/journal.pone.0104845
Rice, H. B., Bernasconi, A., Mak, C., Harris, W. S., von Schacky, C., and Calder, P. C. (2016). Conducting omega 3 clinical trials with cardiovascular outcomes: proceedings of a workshop held in ISSFAS 2014. Prostaglandins Leukot. Essent. Fatty Acids 107, 30–42. doi: 10.1016/j.plefa.2016.01.003
Root, T. L., Pinheiro, A. P., Thornton, L., Strober, M., Fernandez-Aranda, F., Brandt, H., et al. (2010). Substance use disorders in women with anorexia nervosa. Int. J. Eat. Disord. 43, 14–21. doi: 10.1002/eat.20670
Rouzer, C. A., and Marnett, L. J. (2011). Endocanabinoid oxygenation by cyclooxygenases, lipoxygenases, and cytochrom P450. Cross Talk between the eicosanoid and endocannabionid signaling pathways. Chem. Rev. 111, 5899–5921. doi: 10.1021/cr2002799
Ruf, T., and Arnold, W. (2008). Effects of polyunsaturated fatty acids on hibernation and torpor: a review and hypothesis. Am. J. Physiol. Regul. Integr. Comp. Physiol. 294, R1044–R1052. doi: 10.1152/ajpregu.00688.2007
Sam, A. H., Salem, V., and Ghatei, M. (2011). Rimonabant: from RIO (rimonabant in obesity) to ban. J. Obes. 2011:432607. doi: 10.1155/2022/432607
Scherma, M., Sata, V., Collu, R., Boi, M. F., Usai, P., Fratta, W., et al. (2017). Cannabinoid CB1/CB2 receptor agonist attenuate hyperactivity and body weight loss in a rat model of activity-based anorexia. Br. J. Pharmacol. 174, 2682–2695. doi: 10.1111/bph.13892
Schwartz, C., and Andrews, M. T. (2013). Circannual transitions in gene expression; lessons from seasonal adaptations. Curr. Top. Dev. Biol. 105, 247–273. doi: 10.1016/B978-0-12-396968-2.00009-9
Scolnick, B. (2017). Ketogenic diet and anorexia nervosa. Med. Hypothesis 109, 150–152. doi: 10.1016/j.mehy.2017.10.011
Scolnick, B. (2018). Treatment of anorexia nervosa with palmitoylethanolamide. Med. Hypotheses 116, 54–60. doi: 10.1016/j.mehy.2018.04.010
Scolnick, B., and Mostofsky, D. I. (2014). Anorexia nervosa: a rogue hibernation? Med. Hypotheses. 82, 231–235. doi: 10.1016/j.mehy.2013.12.003
Sethis, S., and Brietzke, E. (2017). Recent advances in lipidomics: analytic and clinical perspectives. Prostaglandins Other Mediat. 128–129, 8–16. doi: 10.1016/j.prostaglandins.2016.12.002
Shih, P. B. (2017). Integrating multiomic biomarkers and postprandial metabolism to develop personalized treatment for anorexia nervosa. Prostaglandins Other Lipid Med. 132, 69–76. doi: 10.1016/j.prostaglandins.2017.02.002
Shih, P. B., Morisseau, C., Le, T., Woodside, B., and German, J. B. (2017). Personalized polyunsaturated fatty acids as a potential adjunctive treatment for anorexia nervosa. Prostaglandins Other Lipid. Mediat. 133, 11–19. doi: 10.1016/j.prostaglandins.2017.08.010
Shih, P. B., Yang, J., Morisseau, C., German, J. B., Zeeland, A. A., Armando, A. M., et al. (2016). Dysregulation of soluble epoxide hydrolase and lipidomic profiles in anorexia nervosa. Mol. Psychiatry 21, 537–546. doi: 10.1038/mp.2015.26
Sibeoni, J., Orri, M., Valentin, M., Podlipski, M. A., Colin, S., Pradere, J., et al. (2017). Metasynthesis of the views about treatment of anorexia nervosa in adolescents: perspectives of adolescents, parents, and professionals. PLoS ONE 12:e0169493. doi: 10.1371/journal.pone.0169493
Simopoulous, A. P. (2016). An increase in the omega 6/omega 3 fatty acid ratio increase risk for obesity. Nutrients 8:128. doi: 10.3390/nu8030128
Spector, A. A., and Kim, H. Y. (2015). Cytochrome P450 epoxygenase pathway of polyunsaturated fatty acid metabolism. Biochim. Biophys. Acta 1851, 356–365. doi: 10.1016/j.bbalip.2014.07.020
Swardfager, W., Hennebelle, M., Yu, D., Hammock, B. D., Levitt, A. J., Hashimoto, K., et al. (2018). Metabolic inflammatory/vascular comorbidity in psychiatric disorders: soluble epoxide hydrolase as a possible new target. Neurosci. Biobehav. Rev. 87, 56–66. doi: 10.1016/j.neubiorev.2018.01.010
Swenne, I., Rosling, A., Tenglad, S., and Bessby, B. (2011). Essential fatty acid status in teenage girls with eating disorders and weight loss. Acta Paediatr. 100, 1610–1615. doi: 10.1111/j.1651-2227.2011.02400.x
Toulany, A., Katzman, D. K., Kaufman, M., Hiraki, L. T., and Silverman, E. D. (2014). Chicken or the egg: anorexia nervosa and systemic lupus erythematosus in children and adolescents. Pediatrics 133, e1–e4. doi: 10.1542/peds.2012-3048
Tsuhi, H., Venditti, F. J., Manders, E. S., Evans, J. C., Larson, M. G., Feldman, C. L., et al. (1994). Reduced heart rate variability and mortality risk in an elderly cohort from the framingham study. Circulation 90, 878–883. doi: 10.1161/01.CIR.90.2.878
Vanitallie, T. B., Nonas, C., DiRocco, A., Boyar, K., Hyams, K., and Heymsfield, S. B. (2005). Treatment of parkinsons disease with diet-induced hyperketonemia - a feasibility study. Neurology 64:728e730. doi: 10.1212/01.WNL.0000152046.11390.45
Vaughn, L. K., Denning, G., Stuhr, K. L., de Wit, H., Hill, M. N., and Hillard, C. J. (2010). Endocannabinoid signaling: has it got rhythm? Br. J. Pharmacol. 160, 530–545. doi: 10.1111/j.1476-5381.2010.00790.x
Vazquez, R., Carrera, O., Birmingham, L., and Gutierrez, E. (2006). Exploring the association between anorexia nervosa and geographic latitude. Eat. Weight Disord. 11, 1–8. doi: 10.1007/BF03327745
Vermillion, K. L., Jagtap, P., Johnson, J. E., Griffin, T. J., and Andrews, M. T. (2015). Characterizing cardiac molecular mechanisms of mammalian hibernation via quantitative proteogenomics. J. Proteome Res. 14, 4702–4804. doi: 10.1021/acs.jproteome.5b00575
Vigo, D. E., Castro, M. N., Dorpinghaus, A., Weidema, H., Cardinali, D. P., Siri, L. N., et al. (2008). Nonlinear analysis of heart rate variability in patients with eating disorders. World J. Biol. Psychiatry 9, 183–189. doi: 10.1080/15622970701261604
Yaham, M., Spitz, M., Sandler, L., Heron, N., Roguin, N., and Turgeman, Y. (2013). The significance of bradycardia in anorexia nervosa. Int. J. Angiol. 22, 83–94. doi: 10.1055/s-0033-1334138
Yilmaz, Z., Hardaway, J. A., and Bulik, C. M. (2015). Genetics and epigenetics of eating disorders. Adv. Genomics Genet. 5, 131–150. doi: 10.2147/AGG.S55776
Zák, A., Vecka, M., Twrzicka, E., Hrubý, M., Novák, F., Papezová, H., et al. (2005). Composition of plasma fatty acids and non-cholesterol sterols in anorexia nervosa. Physiol. Res. 54, 443–451.
Zeeland, A. A., Bloss, C. S., Tewhey, R., Bansal, V., Torkamani, A., Libiger, O., et al. (2014). Evidence for role of EPHX2 gene variants in anorexia nervosa. Mol. Psychiatry 19, 724–732. doi: 10.1038/mp.2013.91
Zerwas, S., Larsen, J. T., Petersen, L., Thornton, L. M., Quaranta, M., Koch, S. V., et al. (2017). Eating disorders, autoimmune and autoinflammatory disease: study of entire danish population 1980-2006 and followed to 2012. Pediatrics 140:e20162089. doi: 10.1542/peds.2016-2089
Keywords: anorexia nervosa, hibernation, lipidomic profiling, ketogenic di, palmitoylethanolamide
Citation: Scolnick B (2018) Hypothesis: Clues From Mammalian Hibernation for Treating Patients With Anorexia Nervosa. Front. Psychol. 9:2159. doi: 10.3389/fpsyg.2018.02159
Received: 25 May 2018; Accepted: 19 October 2018;
Published: 12 November 2018.
Edited by:
Emilio Gutierrez, Universidad de Santiago de Compostela, SpainReviewed by:
Nicholas T. Bello, Rutgers University, The State University of New Jersey, United StatesMaria Angeles Gomez Martínez, Pontifical University of Salamanca, Spain
Copyright © 2018 Scolnick. This is an open-access article distributed under the terms of the Creative Commons Attribution License (CC BY). The use, distribution or reproduction in other forums is permitted, provided the original author(s) and the copyright owner(s) are credited and that the original publication in this journal is cited, in accordance with accepted academic practice. No use, distribution or reproduction is permitted which does not comply with these terms.
*Correspondence: Barbara Scolnick, c2NvbG5pY2tAYnUuZWR1