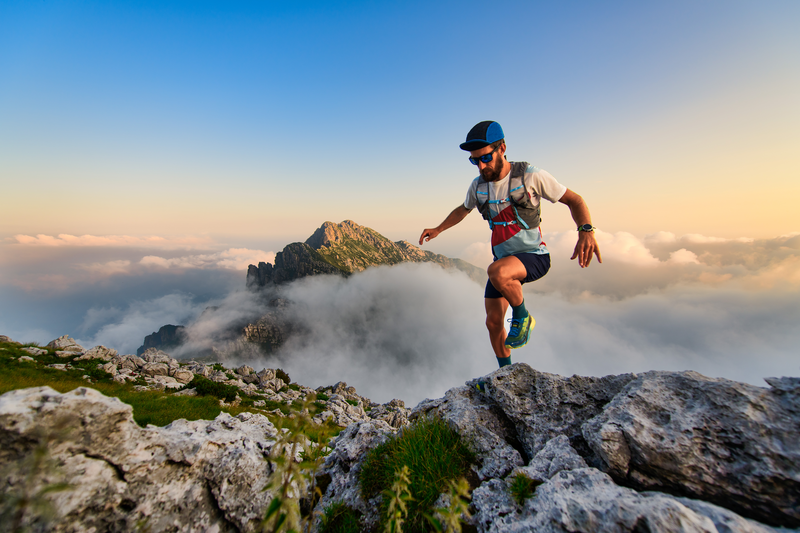
94% of researchers rate our articles as excellent or good
Learn more about the work of our research integrity team to safeguard the quality of each article we publish.
Find out more
ORIGINAL RESEARCH article
Front. Psychol. , 08 June 2015
Sec. Psychology of Language
Volume 6 - 2015 | https://doi.org/10.3389/fpsyg.2015.00638
This article is part of the Research Topic Learning a non-native language in a naturalistic environment: Insights from behavioural and neuroimaging research View all 13 articles
Numerous structural studies have established that experience shapes and reshapes the brain throughout a lifetime. The impact of early development, however, is still a matter of debate. Further clues may come from studying multilinguals who acquired their second language at different ages. We investigated adult multilinguals who spoke three languages fluently, where the third language was learned in classroom settings, not before the age of 9 years. Multilinguals exposed to two languages simultaneously from birth (SiM) were contrasted with multinguals who acquired their first two languages successively (SuM). Whole brain voxel based morphometry revealed that, relative to SuM, SiM have significantly lower gray matter volume in several language-associated cortical areas in both hemispheres: bilaterally in medial and inferior frontal gyrus, in the right medial temporal gyrus and inferior posterior parietal gyrus, as well as in the left inferior temporal gyrus. Thus, as shown by others, successive language learning increases the volume of language-associated cortical areas. In brains exposed early on and simultaneously to more than one language, however, learning of additional languages seems to have less impact. We conclude that – at least with respect to language acquisition – early developmental influences are maintained and have an effect on experience-dependent plasticity well into adulthood.
In recent years, numerous studies on neuronal plasticity have established that training results in structural changes in critically involved cortical brain areas. On a macroscopic level, it has been shown that gray matter (GM) density and GM volume are altered after different kinds of training (for review see Taubert et al., 2010 and Zatorre et al., 2012). Within the domain of neurolinguistics too, ongoing research has demonstrated that acquiring a second language (L2) has a substantial influence on the anatomy of the brain (Li et al., 2014; Stein et al., 2014). This was the case for a variety of language characteristics, such as non-native speech sounds (Golestani et al., 2002, 2007, 2011; Crinion et al., 2006; Wong et al., 2008), acquisition of vocabulary (Grogan et al., 2009, 2012; Hosoda et al., 2013), reading skills (Cummine and Boliek, 2013; Zhang et al., 2013), syntax abilities (Nauchi and Sakai, 2009; Pliatsikas et al., 2014) and executive language control (Elmer et al., 2011; Filippi et al., 2011; Abutalebi et al., 2012, 2013b; Zou et al., 2012). In addition to these studies on specific characteristics of L2 acquisition, research has also been devoted to overall second language proficiency (Amunts et al., 2004; Coggins et al., 2004; Mechelli et al., 2004; Osterhout et al., 2008; Mårtensson et al., 2012; Mohades et al., 2012; Ressel et al., 2012; Schlegel et al., 2012; Stein et al., 2012; García-Pentón et al., 2014; Klein et al., 2014), i.e., to a broad level of L2 proficiency as assessed by overall linguistic testing or by simply comparing groups of bilinguals to groups of monolinguals (Stein et al., 2014; Winkler, unpublished master thesis). Anatomical studies in bilinguals are based on the investigation of GM density changes (e.g., Mechelli et al., 2004; Osterhout et al., 2008; Stein et al., 2012), cortical thickness (e.g., Mårtensson et al., 2012; Klein et al., 2014), and GM volume (e.g., Golestani et al., 2007; Wong et al., 2008). In language-associated areas and, in particular, in areas implicated in control, these studies have generally found alterations due to L2 acquisition, that increase with growing L2 proficiency (for a review, see Li et al., 2014; Stein et al., 2014). Most recently, there have been morphometric studies suggesting that the consequences of bilingualism are related to the form of bilingualism, including not only the age of acquisition and proficiency, but also the context in which the two or more languages are used, i.e., whether speakers are immersed in the language environment, whether they learned the language in a classroom setting and so forth (see the current Special issue).
Neuroscientific studies with multilingual participants, i.e., with subjects speaking at least three languages fluently, are not carried out very often. Only recently, Abutalebi et al. (2013a) combined functional and structural MRI to examine the role of the basal ganglia in multilingual participants. Because of the permanent exposure to a major articulatory load when speaking several languages during a lifetime, the authors hypothesized that there is an enlarged density of gray matter in this area in multilinguals. Indeed, as compared to monolinguals, participants speaking three languages demonstrated increased GM density in the left putamen, which supports the notion of structural plasticity as result of handling a complex articulatory repertoire. In order to compare high cognitive, linguistic, and articulatory demands between multilinguals of two different sorts, namely professional multilingual interpreters versus control multilinguals, Elmer et al. (2014) conducted GM volume analysis on regions previously shown to support language control and executive functions in multilinguals. Interestingly, GM volume was found to be reduced in highly trained multilingual interpreters in a number of regions associated with language control, which suggests that intense training can result in more efficient neural networks, probably due to the pruning of superfluous connections.
Most of the research on the (co)-organization of several languages in the brains of multilinguals has been conducted by using functional brain imaging. Vingerhoets et al. (2003) demonstrated that – in multilinguals with comparable levels of proficiency – late L2 acquisition results in greater activation in L2 than in L1 (Vingerhoets et al., 2003; also shown by Perani et al., 2003; Wartenburger et al., 2003; Kovelman et al., 2008 in bilinguals). In quadrilingual subjects, although there was no clear association between the age of acquisition and the amount of activation, a negative correlation was found between the level of proficiency and the amount of activation (Briellmann et al., 2004). This was also suggested by the data of Abutalebi et al. (2013b) in trilinguals. Bloch et al. (2009) focused on the relation between the age of L2 acquisition and the variability of regional brain activation in Broca’s and Wernicke’s areas in subjects speaking at least three languages fluently and where the L3 had been learned after the age of 9 years. They demonstrated that variability in the representation of the three languages of the individual is related to the age of acquisition of L2, which indicates that early exposure to more than one language gives rise to a language processing network that can accommodate late learned languages.
The present study is based on the work of Bloch et al. (2009) and analyses structural MRI data of subjects fluent in at least three languages. The design of the study allowed us to search for structural differences between simultaneous and successive acquisition of L2. Thus, we suppose that simultaneous (SiM) versus successive or sequential acquisition of L1 and L2 (SuM) is associated with differences in the structural organization of brain areas subserving language processing. More precisely, we hypothesize that groups who acquired L2 later also show higher GM volumes in language-associated regions, as well as in other brain areas belonging to the extended language network (Ferstl et al., 2008). The design of the study provides us, further, with the opportunity to consider the potential role of the late L3 acquired by all participants. Structural differences between simultaneous (SiM) and successive multilinguals (SuMs) could indicate that very early acquired characteristics are maintained over a long period of life.
Forty-four healthy, right-handed [verified by the outcome of the Edinburgh Handedness Inventory (Oldfield, 1971)], non-smoking multilinguals voluntarily participated in this study after receiving information about the investigation and the scanning process and giving their written informed consent. The subjects’ average age at MRI acquisition was 28 years (range 18–37 years) and their female/male ratio was 22/22. The Ethics Committee of the University Hospital of Basel (EKBB, Switzerland) approved the study and confirmed its compliance with all relevant regulatory standards. All subjects were fluent and of medium to high proficiency in at least three languages (see Table 1). They did not differ with respect to their acquisition of their L3, which was comparable within all groups, and acquired at 9 years of age or later at school (see Bloch et al., 2009).
The multilinguals’ age of second language acquisition was defined after analyzing each individual’s language biography (Schütze, 1988; Schwabe, 2003) through oral interviews lasting 2–3 h. These in-depth linguistic biographies are based on the observation that free narrations give a more realistic account of the language history of the participant (Franceschini, 2002). The subjects could then be classified into four different groups of L2 acquisition: 16 (F = 10) simultaneous bilinguals, 8 (F = 3) covert simultaneous bilinguals, 8 (F = 3) sequential bilinguals, and 12 (F = 6) late multilinguals (Bloch et al., 2009). For the purpose of the present study, we re-grouped the participants into two groups:
(1) The simultaneous multilingual (SiM) group (N = 24; F = 13; average age: 27.7 years; age range: 18–36 years) consisting of simultaneous bilinguals, i.e., participants growing up in a bilingual family, where both parents/caregivers spoke different languages, and covert simultaneous bilinguals, i.e., participants growing up in a monolingual family whose language differed from that of the surroundings. This group of bilinguals was exposed to the L2 by the environment parallel to the L1, and for that reason they were grouped together with the simultaneous bilingual group.
(2) The successive multilingual (SuM) group (N = 20; F = 9; average age: 27.8 years; age range: 20–37 years) comprised successive bilinguals who had acquired their L2 subsequently to L1 between their second and fifth year of life, and late multilinguals who had acquired L2 at school when they were at least 9 years of age. Thus, this group covers multilinguals who acquired their L2 at a distinct time point after the acquisition of their L1.
Table 1 shows information on the multilingual profiles of the SiM and SuM groups. Additionally, it gives information about the type of L2 immersion. The level of competence in the individual languages was scaled by self-assessment, using the Common European Reference Framework for Languages (CERR, North, 2000; Council of Europe, 2001). Calculations using the Mann–Whitney U Test revealed no significant differences between SiM and SuM concerning L2 and L3 proficiency (p > 0.05, one-tailed).
As the age of acquisition is not the sole or exclusive influence to alter the structure of the brain, we provide here some additional, post hoc information on the degree of immersion to L2 in our two groups of participants. Immersion has been shown to affect the brain’s anatomy (Pliatsikas et al., 2015) and can be defined as the amount of naturalistic exposure, or immersion, that the speakers receive to that language. It is the degree to which language learners are exposed in their day-to-day activities, (see Pliatsikas and Chondrogianni, 2015).
This study was conducted in the German speaking part of Switzerland. This resulted in the recruited participants having significant exposure to German (the exceptions are subjects 20, 21, 22, 23, and 24 of the SiM group who either acquired Standard German in classroom circumstances as L3 after the age of 9 years or who did not report speaking German at all or learned it as an L4; and subjects 35, 39 and 42 of the SuM group who acquired Standard German in classroom circumstances as L2, see Table 1). Growing up in a German-speaking country makes the context for the acquisition of the L2 – in the cases when Standard German/Swiss German was learned as an L2 – one of early and high immersion by context (due to the linguistic dominance of the environment), or, in the case of the covert simultaneous-participants, a context of medium–high immersion. Similarly, growing up in a German speaking country with at least one caregiver speaking another language than German makes L2 acquisition of high immersion by family. Thus the majority of the members of SiM acquired their L2 by high immersion by family/context or medium-to-high immersion by context. The majority of the members of the SuM group learned L2 in a classroom setting. The subgroup of multilinguals classified as “2nd to 5th year” of age spent a period of their childhood/youth outside a German speaking country or moved to a German-speaking context. Their level of immersion to L2 is thus either high by context (2 out of 8) or temporary high by context (6 out of 8). Therefore, the quality of immersion differs between SiM and SuM (Table 1).
Magnetic resonance images of the 44 subjects were acquired on a 1.5-T Magnetom Vision MRI Scanner (Siemens, Erlangen, Germany) at the University Hospital of Basel. We used a standard head coil to restrict head movements and to limit motion artifacts. A three dimensional (3D) T1-weighted anatomical high-resolution Magnetization Prepared Rapid Gradient Echo (MPRAGE) sequence was applied with repetition time of 9.7 ms, echo time of 4 ms, inversion time of 300 ms, and isotopic spatial resolution 1 mm × 1 mm × 1 mm (see also Bloch et al., 2009). The scans were all screened for major radiological abnormalities or visual artifacts by an experienced neuroradiologist.
These MRI data were analyzed on commercially available Intel-based desktop computers with a Debian Linux 3.1 operating system. The structural images were pre-processed using a Voxel-Based Morphometry (VBM8) toolbox1, as implemented in the Statistical Parametric Mapping software package2. The data were registered with “Diffeomorphic Anatomical Registration Through Exponentiated Lie” (DARTEL) within VBM8, running under the MATLAB 7.11.0 (R2010b) environment (Members of the Wellcome Trust Centre for Neuroimaging, 2009). Accuracy and sensitivity were maximized by creating a study-specific template and segmentation of each subject’s image (Yassa and Stark, 2009). We conducted the following steps: (1) checking for scanner artifacts and major anatomical abnormalities for each subject; (2) aligning and reorientating the scans; (3) using New Segmentation and high-dimensional normalization DARTEL (Ashburner, 2007); (4) checking for homogeneity across the sample; and (5) using 8 mm standard smoothing (Bailey, 2008). The default values for realignment, warping, and normalization were used (Kurth et al., 2010). Finally, realigned, segmented, normalized, and smoothed data were subjected to statistical analysis.
Of the 44 samples of this study two were outliers, with a mean covariance below 2 SDs. Repeated analyses without these two subjects did not change the results (not shown). We therefore decided to retain these two subjects.
Voxel-based morphometry compares images on a voxel basis after spatial normalization using deformation fields that discount macroscopic differences in shape. We estimated between-group differences in GM volume at each intracerebral voxel in standard space by fitting a full-factorial analysis of covariance (ANCOVA), and contrasted the SiM and SuM groups. We modeled age at image acquisition and sex/gender as covariates of no interest, in order to reduce the potential impact of these variables on the GM volume in language-associated brain areas. We identified spatially continuous voxels at a threshold of p < 0.01 (uncorrected; cluster forming threshold; Petersson et al., 1999) and defined a family wise error-corrected cluster-extent threshold of p < 0.05 to infer statistical significance. In order to mark areas with significant GM volume differences on this statistical threshold level, Montreal Neurological Institute (MNI) coordinates were transformed into Talairach space (MNI and Talairach Transformation, 2013; Talairach.org Daemon, 2013).
Gray matter volume was lower in the group of SiMs as compared to SuMs in the following regions: bilaterally in the medial frontal gyrus (MFG) and the left inferior frontal gyrus (IFG; p < 0.001, FWE); in the right IFG and right medial temporal gyrus (MTG); in the left inferior temporal gyrus (ITG); and in the right inferior posterior parietal gyrus (p < 0.05, FWE; Table 2; Figure 1). The opposite contrast SuM > SiM did not reveal any significant results.
FIGURE 1. ANCOVA full factorial 2 × 2. Significant clusters (PFWE-corr < 0.05) with smaller GMV in SiM versus SuM shown in red. Group template used as background image in gray.
The present study of 44 multilinguals is to our knowledge the first VBM study in multilinguals as opposed to numerous studies in bilinguals. It shows that GM volume was higher in the group of multilinguals who learned their L2 successively compared to the multilingual who acquired a second language simultaneously with their L1. Thus, subjects who did not acquire two languages simultaneously (by immersion) in early life but learned them sequentially, mostly in classroom settings, showed larger GM volume patterns in cortical language-associated regions and the extended language network (Ferstl et al., 2008). This result supports our thesis that early simultaneous bilingualism persists in the anatomical make-up of the adult brain.
The exact degree to which the difference between SiM and SuM is or is not influenced by a late learned L3 cannot be determined based on the present design. However, the other way round requires attention: testing trilinguals obliges us to tentatively hypothesize that despite the fact of a late learned L3 the differences based on the age of L2 acquisition persist into adulthood and do not disappear. Thus SiM and SuM remain different even though a late L3 was acquired. It can be assumed that the earlier in life a language experience is made, the more receptive the brain is to new learning and the more efficiently the brain can incorporate new language associated experiences, i.e., further input can be integrated into the same structural substrates. This would then be mirrored in GM patterns, particularly in the lower GM volume for early simultaneous L2 acquisition, including the late learned L3.
Our result corresponds with previous research showing an impact of L2 acquisition on GM volume of the bilingual brain (Mechelli et al., 2004; Osterhout et al., 2008; Stein et al., 2012) as well as research on GM volumetric changes in multilinguals (Elmer et al., 2014). However, the comparison of work applying GM density approaches and studies based on GM volumetric methods remains challenging. VBM is a technique that permits comparisons of the entire brain volume at the single voxel level. In contrast to previous studies (Mechelli et al., 2004; Osterhout et al., 2008; Stein et al., 2012) which reported GM densities rather than volumes, we used “optimized” VBM, which includes an additional modulation step to minimize the potentially confounding effects of errors in stereotactic normalization (Ashburner and Friston, 2001; Good et al., 2001). All images were smoothed using a 8 mm full-width-at-half-maximum Gaussian kernel, as in a previous study (Mechelli et al., 2004). According to the matched filter theorem, the width of the smoothing kernel determines the scale at which morphological changes are most sensitively detected (White et al., 2001). In the present study, we have chosen a rather small smoothing kernel, as this allows us to detect a greater number of regions with small structures and to better compare our results with the GM densities reported by Mechelli et al. (2004). Furthermore, both samples are based on healthy participants, so that we did not expect that regional changes would be very large or would differ much between cortical regions or between the studies. As expected, our results basically confirm the correlation (Mechelli et al., 2004; Stein et al., 2012) and/or association between age of L2 acquisition and GM structure, as reported previously (Osterhout et al., 2008).
In the present study, differences in GM volume were detected in the temporal as well as inferior and medial frontal regions of both hemispheres and the inferior parietal area. These are broadly parts of regions involved in the functional anatomy of language (Price, 2010) and may be linked, with the exception of the right inferior prefrontal cortex, to the “extended language network” outlined in influential work on the functional processing of language comprehension (Ferstl, 2007; Ferstl et al., 2008). Typically, primary language areas for language comprehension and production, such as Wernicke’s and Broca’s areas, did not display any significant difference between the SiM and SuM group. In the related fMRI study on multilingualism by Bloch et al. (2009), no specific group-dependent differences in activation were found in these areas either, which shows, for our population, that these regions are used, irrespectively of when a language is learned. The degree of variability in which they were activated by the three languages is, however, highly dependent on the age of L2 acquisition.
In the following, our GM data are discussed in relation to other studies on structural changes of gray matter linked to overall second language proficiency (Stein et al., 2014). Special attention is given to the bilateral character of our results. In their whole brain analysis of early and late bilinguals, Mechelli et al. (2004) found structural changes in the inferior parietal cortex (IPC) in relation to age; similarly Osterhout et al. (2008) employed an ROI analysis and detected structural alterations in the same region. Here too, GM changes in the IPC were detected and linked to age of acquisition. Others have demonstrated that the GM density of this region is positively correlated with vocabulary acquisition and knowledge, suggesting that this area is important not only for global L2 acquisition but for handling a large vocabulary (Lee et al., 2007; Richardson et al., 2010). Contrary to Mechelli et al. (2004), Osterhout et al. (2008), and Richardson et al. (2010), however, the present work showed changes in the right (x/y/z, 50/-79/48) and not in the left hemispheric IPC and thus supports the conclusions of Lee et al. (2007), who showed that vocabulary mastery predicted GM density in the bilateral IPC.
While Stein et al. (2012), in their longitudinal study on L2 acquisition, reported an increase in GM density in the left inferior frontal cortex (IFC) in close vicinity to the pars triangularis in Broca’s area, the present study detected a bilateral pattern in this region and revealed a difference with respect to the age of L2 acquisition: Stein et al.’s (2012) participants learned L2 as adults, whereas our group of participants acquired their L2 as children. The results in our group of SiMs in IFC is in line with data of a recent cortical thickness study concerning the bilaterality of the structural patterns in this very same region (Klein et al., 2014). Klein et al. (2014) report, however, that thickness correlates positively with age of acquisition in the left IFG and negatively in the right IFG; this opposing interhemispheric effect was not re-enacted in our study where the association of age with higher GM volumes clearly counts for both the right and left IFG.
Our results on bilateral differences between SiM and SuM in GM volume in IFC are corroborated by functional data from bi- and multilinguals showing greater bilateral activation during both L1 and L2 processing than for monolinguals (Hull and Vaid, 2006; Park et al., 2012), with a strong tendency for the right Broca’s homolog to be activated in the L1 of multilinguals (Kaiser et al., 2007). There is still little evidence about the function of the MFG in the context of structural changes due to L2 acquisition, except that its cortical thickness shows alterations after very intensive language training over many months (Mårtensson et al., 2012). Again, our present results reveal structural bilateral modifications in the MFG, whereas Mårtensson et al. (2012) found these only in the left side. Data based on functional MRI studies suggest that the MFG is crucial for text comprehension (Ferstl and von Cramon, 2001).
It is well known that both the inferior and the middle temporal gyrus handle various aspects of lexical semantic representation and processing. Our study is, to our knowledge, the first to present changes in the GM volume due to early L2 acquisition in both the right MTG and left ITG.
Taken together, the right hemispheric trend (as exemplified in r-IPC; bilateral IFC; bilateral MFG; r-MTG) – which characterizes our set of multilinguals when differentiated by the age of L2 acquisition – could be influenced by two additional factors: by the mode of L2 acquisition and/or by the interference of the L3.
Our subjects were carefully selected on the basis of their multilingual profile and had to undergo extensive interviewing for 2–3 h about their three languages. However, some information about their languages was not captured. Thus, for instance, it cannot be excluded with certainty whether any of the simultaneous participants registered with German/Swiss German as L2 grew up in a non-German speaking country and acquired L1 from one caregiver and L2 (German/Swiss German) from a second caregiver. Nevertheless, the presence of immersion as the main access to L2 acquisition remains valid in SiM, as well as the presence of classroom learning in SuM. Future cross-sectional and longitudinal research is needed to identify which of these ways of learning L2, i.e., L2 acquisition based on high immersion by family, high immersion by context, medium-high immersion by context, temporary high immersion by context, or classroom learning has a greater impact on structural GM in relevant brain areas. Most recently, Pliatsikas et al. (2015) demonstrated the effects of immersion on brain structure in young, highly immersed late bilinguals. In their view, “[immersion] can be broadly defined as the degree to which language learners use their non-native language outside the classroom and for their day-to-day activities and usually presupposes that the learners live in an environment where their non-native language is exclusively or mostly used” (see Pliatsikas and Chondrogianni, 2015). Interestingly, structural alterations in white matter were shown to be effected by everyday L2 use in a naturalistic environment, rather than by length of L2 learning or age of onset of L2 learning (Pliatsikas et al., 2015). Thus, our results in the group of SiM can also be interpreted from this perspective showing that naturalistic exposure, rather than age of L2 acquisition, impacts on brain structure. This is not the case for the group of SuM who in their majority acquired L2 as a foreign language in the classroom.
Finally, the impact of the L3 on language-associated brain areas and the extended language network remains to be elucidated. In our experimental setup, the age of L2 acquisition is the variable determining the GM structure early in development. However, the way in which training for additional languages drives GM plasticity in regions already influenced by bilingualism is open to speculation. Additional training might result in pruning of language networks, as suggested by Elmer et al. (2014), or might drive contralateral (right hemisphere) cortical areas to participate in language related tasks.
The results of the present study cannot prove a lifelong plasticity of the brain for languages since the examined subjects were chosen based on the fact of having learnt at least three languages during early or late childhood, respectively, and we do not know about further changes in their brains during adulthood. Neither do the obtained outcomes give any information if there is a critical age for the native-like acquisition of one or many languages, although there are certainly studies showing complex relations between the maturation of the brain in children and the brain’s plasticity to adjust to structural demands of (individual) language development (Brauer et al., 2011).
Modification of the user-options implemented in the analysis software, such as setting the smoothing kernel, can influence subsequent statistical results (Ashburner and Friston, 2001): a larger kernel (12 mm instead of 8 mm) results in greater cluster sizes (Friston, 2003, p. 5). We used a default option of 8 mm as recommended. VBM compares voxel-by-voxel images of different groups and reports MNI standard coordinates for every cluster center, which does not necessarily correspond to the actual cluster localisation in an individual’s brain. Thus VBM statistics do not differentiate between two clusters localized for example in the medial plane. In the present case, the large clusters in the medial frontal gyri on the left and the right are counted as one cluster.
As to the statistical thresholds, it is still very difficult to compare data from different studies. Height thresholds range from puncorr < 0.001 up to pcorr < 0.05 in different studies (Brambati et al., 2004; Eckert et al., 2005; Silani et al., 2005; Hoeft et al., 2007; Steinbrink et al., 2008; Richardson and Price, 2009). Here, an uncorrected height threshold of p < 0.01 was used. The clusters found are therefore large, although there are small differences between the two groups.
Contrary to the successive acquisition of the second language, simultaneous acquisition of L1/L2 (by immersion) from the first year of life on is associated with low GM volume in language-associated regions, in the prefrontal, medial temporal and parietal cortex, in particular. This difference persists even though a late L3 is learned. Growing up in a multilingual environment in early childhood may change the individual’s cortical structure, enforcing it to generally build more efficient synaptic networks for language processing. To further understand structural changes underlying brain plasticity during language learning requires longitudinal studies with homogenous groups of SiM and SuM.
The authors declare that the research was conducted in the absence of any commercial or financial relationships that could be construed as a potential conflict of interest.
Abutalebi, J., Della Rosa, P. A., Castro Gonzaga, A. K., Keim, R., Costa, A., and Perani, D. (2013a). The role of the left putamen in multilingual language production. Brain Lang. 125, 307–315. doi: 10.1016/j.bandl.2012.03.009
Abutalebi, J., Della Rosa, P., Ding, G., Weekes, B., Costa, A., and Green, D. (2013b). Language proficiency modulates the engagement of cognitive control areas in multilinguals. Cortex 49, 905–911. doi: 10.1016/j.cortex.2012.08.018
Abutalebi, J., Della Rosa, P. A., Green, D. W., Hernandez, M., Scifo, P., Keim, R., et al. (2012). Bilingualism tunes the anterior cingulate cortex for conflict monitoring. Cereb. Cortex 22, 2076–2086. doi: 10.1093/cercor/bhr287
Amunts, K., Schleicher, A., and Zilles, K. (2004). Outstanding language competence and cytoarchitecture in Broca’s speech region. Brain Lang. 89, 346–353. doi: 10.1016/S0093-934X(03)00360-2
Ashburner, J., and Friston, K. (2001). Why voxel-based morphometry should be used. Neuroimage 14, 1238–1243. doi: 10.1006/nimg.2001.0961
Bloch, C., Kaiser, A., Kuenzli, E., Zappatore, D., Haller, S., Franceschini, R., et al. (2009). The age of second language acquisition determines the variability in activation elicited by narration in three languages in Broca’s and Wernicke’s area. Neuropsychologia 47, 625–633. doi: 10.1016/j.neuropsychologia.2008.11.009
Brambati, S. M., Termine, C., Ruffino, M., Stella, G., Fazio, F., Cappa, S. F., et al. (2004). Regional reductions of grey matter volume in familial dyslexia. Neurology 63, 742–745.
Brauer, J., Anwander, A., and Friederici, A. D. (2011). Neuroanatomical prerequisites for language functions in the maturing brain. Cereb. Cortex 21, 459–466. doi: 10.1093/cercor/bhq108
Briellmann, R. S., Saling, M. M., Connell, A. B., Waites, A. B., Abbott, D. F., and Jackson, G. D. (2004). A high-field functional MRI study of quadri-lingual subjects. Brain Lang. 89, 531–542. doi: 10.1016/j.bandl.2004.01.008
Coggins, P. E. III, Kennedy, T. J., and Armstrong, T. A. (2004). Bilingual corpus callosum variability. Brain Lang. 89, 69–75. doi: 10.1016/S0093-934X(03)00299-2
Council of Europe. (2001). Common European Framework of Reference for Languages: Learning, Teaching, Assessment. Cambridge: Cambridge University Press.
Crinion, J., Turner, R., Grogan, A., Hanakawa, T., Noppeney, U., Devlin, J. T., et al. (2006). Language control in the bilingual brain. Science 312, 1537–1540. doi: 10.1126/science.1127761
Cummine, J., and Boliek, C. A. (2013). Understanding white matter integrity stability for bilinguals on language status and reading performance. Brain Struct. Funct. 218, 595–601. doi: 10.1007/s00429-012-0466-6
Eckert, M. A., Leonard, C. M., Wilke, M., Eckert, M., Richards, T., Richards, A., et al. (2005). Anatomical signatures of dyslexia in children: unique information from manual and voxel based morphometry brain measures. Cortex 41, 304–315.
Elmer, S., Hänggi, J., and Jäncke, L. (2014). Processing demands upon cognitive, linguistic, and articulatory functions promote grey matter plasticity in the adult multilingual brain: insights from simultaneous interpreters. Cortex 54, 179–189. doi: 10.1016/j.cortex.2014.02.014
Elmer, S., Hänggi, J., Meyer, M., and Jäncke, L. (2011). Differential language expertise related to white matter architecture in regions subserving sensory-motor coupling, articulation, and interhemispheric transfer. Hum. Brain Mapp. 32, 2064–2074. doi: 10.1002/hbm.21169
Ferstl, E. (2007). “The functional neuroanatomy of text comprehension: what’s the story so far?” in Higher Level Language Processes in the Brain: Inference and Comprehension Processes, eds F. Schmalhofer and C. Perfetti (Mahwah, NJ: Lawrence Erlbaum), 53–102.
Ferstl, E., Neumann, J., Bogler, C., and von Cramon, D. Y. (2008). The extended language network: a meta-analysis of neuroimaging studies on text comprehension. Hum. Brain Mapp. 29, 581–593. doi: 10.1002/hbm.20422
Ferstl, E., and von Cramon, D. (2001). The role of coherence and cohesion in text comprehension: an event-related fMRI study. Cogn. Brain Res. 11, 325–340. doi: 10.1016/S0926-6410(01)00007-6
Filippi, R., Richardson, F. M., Dick, F., Leech, R., Green, D. W., Thomas, M. S. C., et al. (2011). The right posterior paravermis and the control of language interference. J. Neurosci. 31, 10732–10740. doi: 10.1523/JNEUROSCI.1783-11.2011
Franceschini, R. (2002). “Sprachbiographien: Erzählungen über Mehrsprachigkeit und deren Erkenntnisinteresse für die Spracherwerbsforschung und die Neurobiologie der Mehrsprachigkeit,” in Biografie linguistiche/Biographies langagières/Biografias linguisticas/Sprachbiografien, eds K. Adamzik and E. Roos (Neuchâtel: Bulletin vals-asla), 19–33.
Friston, K. J. (2003). Statistical parametric mapping. Neurosci. Databases 4, 237–246. doi: 10.1006/nimg.2002.1259
García-Pentón, L., Fernández, A. P., Iturria-Medina, Y., Gillon-Dowens, M., and Carreiras, M. (2014). Anatomical connectivity changes in the bilingual brain. Neuroimage 84, 495–504. doi: 10.1016/j.neuroimage.2013.08.064
Golestani, N., Molko, N., Dehaene, S., LeBihan, D., and Pallier, C. (2007). Brain structure predicts the learning of foreign speech sounds. Cereb. Cortex 17, 575–582. doi: 10.1093/cercor/bhk001
Golestani, N., Paus, T., and Zatorre, R. J. (2002). Anatomical correlates of learning novel speech sounds. Neuron 35, 997–1010.
Golestani, N., Price, C. J., and Scott, S. K. (2011). Born with an ear for dialects? Structural plasticity in the expert phonetician brain. J. Neurosci. 31, 4213–4220. doi: 10.1523/JNEUROSCI.3891-10.2011
Good, C., Johnsrude, I., Ashburner, J., Henson, R., Friston, K., and Frackowiak, R. (2001). A voxel-based morphometric study of ageing in 465 normal adult human brains. Neuroimage 14, 21–36. doi: 10.1006/nimg.2001.0786
Grogan, A., Green, D. W., Ali, N., Crinion, J. T., and Price, C. J. (2009). Structural correlates of semantic and phonemic fluency ability in first and second languages. Cereb. Cortex 19, 2690–2698. doi: 10.1093/cercor/bhp023
Grogan, A., Parker Jones, O., Ali, N., Crinion, J., Orabona, S., Mechias, M. L., et al. (2012). Structural correlates for lexical efficiency and number of languages in non-native speakers of English. Neuropsychologia 50, 1347–1352. doi: 10.1016/j.neuropsychologia.2012.02.019
Hoeft, F., Meyler, A., Hernandez, A., Juel, C., Taylor-Hill, J., Martindale, J., et al. (2007). Functional and morphometric brain dissociation between dyslexia and reading ability. Proc. Natl. Acad. Sci. U.S.A. 104, 4234–4239. doi: 10.1073/pnas.0609399104
Hosoda, C., Tanaka, K., Nariai, T., Honda, M., and Hanakawa, T. (2013). Dynamic neural network reorganization associated with second language vocabulary acquisition: a multimodal imaging study. J. Neurosci. 33, 13663–13672. doi: 10.1523/JNEUROSCI.0410-13.2013
Hull, R., and Vaid, J. (2006). Laterality and language experience. Laterality 11, 436–464. doi: 10.1080/13576500600691162
Kaiser, A., Kuenzli, E., Zappatore, D., and Nitsch, C. (2007). On females’ lateral and males’ bilateral activation during language production: a fMRI study. Int. J. Psychophysiol. 63, 192–198. doi: 10.1016/j.ijpsycho.2006.03.008
Klein, D., Mok, K., Chen, J. K., and Watkins, K. E. (2014). Age of language learning shapes brain structure: a cortical thickness study of bilingual and monolingual individuals. Brain Lang. 131, 20–24. doi: 10.1016/j.bandl.2013.05.014
Kovelman, I., Baker, S. A., and Petitto, L.-A. (2008). Bilingual and monolingual brains compared: a functional magnetic resonance imaging investigation of syntactic processing and a possible ‘neural signature’ of bilingualism. J. Cogn. Neurosci. 20, 153–169. doi: 10.1162/jocn.2008.20011
Lee, H. L., Devlin, J. T., Shakeshaft, C., Stewart, L. H., Brennan, A., Glensman, J., et al. (2007). Anatomical traces of vocabulary acquisition in the adolescent brain. J. Neurosci. 27, 1184–1189. doi: 10.1523/JNEUROSCI.4442-06.2007
Li, P., Legault, J., and Litcofsky, K. A. (2014). Neuroplasticity as a function of second language learning: anatomical changes in the humanbrain. Cortex 58, 301–324. doi: 10.1016/j.cortex.2014.05.001
Mårtensson, J., Eriksson, J., Bodammer, N. C., Lindgren, M., Johansson, M., Nyberg, L., et al. (2012). Growth of language-related brain areas after foreign language learning. Neuroimage 63, 240–244. doi: 10.1016/j.neuroimage.2012.06.043
Mechelli, A., Crinion, J. T., Noppeney, U., O’Doherty, J., Ashburner, J., Frackowiak, R. S., et al. (2004). Neurolinguistics: structural plasticity in the bilingual brain. Nature 431, 757. doi: 10.1038/431757a
Members of the Wellcome Trust Centre for Neuroimaging. (2009). SPM8 Statistical Parametric Mapping (Version 8). Available at: http://www.fil.ion.ucl.ac.uk/spm/software/spm8/
MNI and Talairach Transformation. (2013). Available at: http://www.ebire.org/hcnlab/cortical-mapping/ (accessed March 17, 2013).
Mohades, S. G., Struys, E., Van Schuerbeek, P., Mondt, K., Van De Craen, P., and Luypaert, R. (2012). DTI reveals structural differences in white matter tracts between bilingual and monolingual children. Brain Res. 1435, 72–80. doi: 10.1016/j.brainres.2011.12.005
Nauchi, A., and Sakai, K. L. (2009). Greater leftward lateralization of the inferior frontal gyrus in second language learners with higher syntactic abilities. Hum. Brain Mapp. 30, 3625–3635. doi: 10.1002/hbm.20790
North, B. (2000). The Development of a Common Framework Scale of Language Proficiency. Ph.D. thesis, Thames Valley University, New York, NY: Peter Lang.
Oldfield, R. C. (1971). The assessment and analysis of handedness: the Edinburgh inventory. Neuropsychologia 9, 97–113.
Osterhout, L., Poliakov, A., Inoue, K., McLaughlin, J., Valentine, G., Pitkanen, I., et al. (2008). Second-language learning and changes in the brain. J. Neurolinguistics 21, 509–521. doi: 10.1016/j.jneuroling.2008.01.001
Park, H. R. P., Badzakova-Trajkov, G., and Waldie, K. E. (2012). Language lateralisation in late proficient bilinguals: a lexical decision fMRI study. Neuropsychologia 50, 688–695. doi: 10.1016/j.neuropsychologia.2012.01.005
Perani, D., Abutalebi, J., Paulesu, E., Brambati, S., Scifo, P., Cappa, S. F., et al. (2003). The role of age of acquisition and language usage in early, high-proficient bilinguals: an fMRI study during verbal fluency. Hum. Brain Mapp. 19, 170–182. doi: 10.1002/hbm.10110
Petersson, K. M., Nichols, T. E., Poline, J. B., and Holmes, A. P. (1999). Statistical limitations in functional neuroimaging. II. Signal detection and statistical inference. Philos. Trans. R. Soc. Lond. B Biol. Sci. 354, 1261–1281.
Pliatsikas, C., and Chondrogianni, V. (2015). Learning a non-native language in a naturalistic environment: insights from behavioral and neuroimaging research (Editorial note). Frontiers Special Issue.
Pliatsikas, C., Johnstone, T., and Marinis, T. (2014). Grey matter volume in the cerebellum is related to the processing of grammatical rules in a second language: a structural voxel-based morphometry study. Cerebellum 13, 55–63. doi: 10.1007/s12311-013-0515-6
Pliatsikas, C., Moschopoulou, E., and Saddy, J. D. (2015). The effects of bilingualism on the white matter structure of the brain. Proc. Nat. Acad. Sci. U.S.A. 112, 1334–1337. doi: 10.1073/pnas.1414183112
Price, C. J. (2010). The anatomy of language: a review of 100 fMRI studies published in 2009. Ann. N. Y. Acad. Sci. 1191, 62–88. doi: 10.1111/j.1749-6632.2010.05444.x
Ressel, V., Pallier, C., Ventura-Campos, N., Díaz, B., Roessler, A., Ávila, C., et al. (2012). An effect of bilingualism on the auditory cortex. J. Neurosci. 32, 16597–16601. doi: 10.1523/JNEUROSCI.1996-12.2012
Richardson, F., Michael, T., Filippi, R., Harth, H., and Price, C. J. (2010). Contrasting effects of vocabulary knowledge on temporal and parietal brain structure across lifespan. J. Cogn. Neurosci. 22, 943–954. doi: 10.1162/jocn.2009.21238
Richardson, F. M., and Price, C. J. (2009). Structural MRI studies of language function in the undamaged brain. Brain Struct. Funct. 213, 511–523. doi: 10.1007/s00429-009-0211-y
Schlegel, A. A., Rudelson, J. J., and Tse, P. U. (2012). White matter structure changes as adults learn a second language. J. Cogn. Neurosci. 24, 1664–1670. doi: 10.1162/jocn_a_00240
Schütze, F. (1988). Das Narrative Interview in Interaktionsfeldstudien. Hagen: Fernuniversität Gesamthochschule Hagen.
Schwabe, M. (2003). Review: Gabriele Lucius-Hoene & Arnulf Deppermann (2002). Rekonstruktion narrativer Identität. Ein Arbeitsbuch zur Analyse narrativer Interviews [The Reconstruction of Narrative Identity. A Guide to the Analysis of Narrative Interviews]. Forum Qualitative Sozialforschung / Forum: Qualitative Social Research, 4. Avaialble at: http://www.qualitative-research.net/index.php/fqs/article/view/691/1492
Silani, G., Frith, U., Demonet, J. F., Fazio, F., Perani, D., Price, C., et al. (2005). Brain abnormalities underlying altered activation in dyslexia: a voxel based morphometry study. Brain 128, 2453–2461. doi: 10.1093/brain/awh579
Stein, M., Federspiel, A., Koenig, T., Wirth, M., Strik, W., Wiest, R., et al. (2012). Structural plasticity in the language system related to increased second language proficiency. Cortex 48, 458–465. doi: 10.1016/j.cortex.2010.10.007
Stein, M., Winkler, C., Kaiser, A., and Dierks, T. (2014). Structural brain changes related to bilingualism: does immersion make a difference? Front. Psychol. 5:1116. doi: 10.3389/fpsyg.2014.01116
Steinbrink, C., Vogt, K., Kastrup, A., Müller, H.-P., Juengling, F. D., Kassubek, J., et al. (2008). The contribution of white and grey matter differences to developmental dyslexia: insights from DTI and VBM at 3.0 T. Neuropsychologia 46, 3170–3178. doi: 10.1016/j.neuropsychologia.2008.07.015
Talairach.org Daemon. (2013). Available at: http://www.talairach.org/daemon.html (accessed March 17, 2013).
Taubert, M., Draganski, B., Anwander, K., Müller, K., Horstmann, A., Villringer, A., et al. (2010). Dynamic properties of human brain structure: learning-related changes in cortical areas and associated fiber connections. J. Neurosci. 30, 11670–11677. doi: 10.1523/JNEUROSCI.2567-10.2010
Vingerhoets, G., Van Borsel, J., Tesink, C., van den Noort, M., Deblaere, K., Seurinck, R., et al. (2003). Multilingualism: an fMRI study. Neuroimage 20, 2181–2196.
Wartenburger, I., Heekeren, H. R., Abutalebi, J., Cappa, S. F., Villringer, A., and Perani, D. (2003). Early setting of grammatical processing in the bilingual brain. Neuron 37, 159–170.
White, T., O’Leary, D., Magnotta, V., Arndt, S., Flaum, M., and Andreasen, N. (2001). Anatomic and functional variability: the effects of filter size in group fMRI data analysis. Neuroimage 13, 577–588. doi: 10.1006/nimg.2000.0716
Wong, P. C. M., Warrier, C. M., Penhune, V. B., Roy, A. K., Sadehh, A., Parrish, T. B., et al. (2008). Volume of left Heschl’s gyrus and linguistic pitch learning. Cereb. Cortex 18, 828–836. doi: 10.1093/cercor/bhm115
Yassa, M. A., and Stark, C. E. L. (2009). A quantitative evaluation of cross-participant registration techniques for MRI studies of the medial temporal lobe. Neuroimage 44, 319–327. doi: 10.1016/j.neuroimage.2008.09.016
Zatorre, R. J., Fields, R. D., and Johansen-Berg, H. (2012). Plasticity in grey and white: neuroimaging changes in brain structure during learning. Nat. Neurosci. 15, 528–536. doi: 10.1038/nn.3045
Zhang, M., Li, J., Chen, C., Mei, L., Xue, G., Lu, Z., et al. (2013). The contribution of the left mid-fusiform cortical thickness to Chinese and English reading in a large Chinese sample. Neuroimage 65, 250–256. doi: 10.1016/j.neuroimage.2012.09.045
Keywords: multilingualism, bilingualism, age of L2 acquisition, magnetic resonance imaging, gray matter volume
Citation: Kaiser A, Eppenberger LS, Smieskova R, Borgwardt S, Kuenzli E, Radue E-W, Nitsch C and Bendfeldt K (2015) Age of second language acquisition in multilinguals has an impact on gray matter volume in language-associated brain areas. Front. Psychol. 6:638. doi: 10.3389/fpsyg.2015.00638
Received: 16 June 2014; Accepted: 01 May 2015;
Published online: 08 June 2015.
Edited by:
Vicky Chondrogianni, University of Edinburgh, UKReviewed by:
Judith F. Kroll, The Pennsylvania State University, USACopyright © 2015 Kaiser, Eppenberger, Smieskova, Borgwardt, Kuenzli, Radue, Nitsch and Bendfeldt. This is an open-access article distributed under the terms of the Creative Commons Attribution License (CC BY). The use, distribution or reproduction in other forums is permitted, provided the original author(s) or licensor are credited and that the original publication in this journal is cited, in accordance with accepted academic practice. No use, distribution or reproduction is permitted which does not comply with these terms.
*Correspondence: Anelis Kaiser, Department of Social Psychology and Social Neuroscience, Institute of Psychology, University of Bern, Fabrikstrasse 8, 3012 Bern, Switzerland,YW5lbGlzLmthaXNlckBwc3kudW5pYmUuY2g=
†These authors have shared first authorship.
‡These authors have shared last authorship.
Disclaimer: All claims expressed in this article are solely those of the authors and do not necessarily represent those of their affiliated organizations, or those of the publisher, the editors and the reviewers. Any product that may be evaluated in this article or claim that may be made by its manufacturer is not guaranteed or endorsed by the publisher.
Research integrity at Frontiers
Learn more about the work of our research integrity team to safeguard the quality of each article we publish.