- 1 INSERM-UPMC UMRS 975, Brain and Spine Institute, Groupe Hospitalier Pitié-Salpêtrière, Paris, France
- 2 Department of Experimental Psychology, University of Granada, Granada, Spain
- 3 AP-HP, Groupe Hospitalier Pitié-Salpêtrière, Fédération de Neurologie, Paris, France
- 4 Department of Psychology, Catholic University, Milan, Italy
The relationships between spatial attention and conscious perception are currently the object of intense debate. Recent evidence of double dissociations between attention and consciousness cast doubt on the time-honored concept of attention as a gateway to consciousness. Here we review evidence from behavioral, neurophysiologic, neuropsychological, and neuroimaging experiments, showing that distinct sorts of spatial attention can have different effects on visual conscious perception. While endogenous, or top-down attention, has weak influence on subsequent conscious perception of near-threshold stimuli, exogenous, or bottom-up forms of spatial attention appear instead to be a necessary, although not sufficient, step in the development of reportable visual experiences. Fronto-parietal networks important for spatial attention, with peculiar inter-hemispheric differences, constitute plausible neural substrates for the interactions between exogenous spatial attention and conscious perception.
Background
Both “attention” and “consciousness” refer to complex concepts in search of consensus for definition. “Consciousness” can indicate a state of vigilance or wakefulness, which ranges between comatose states to being awake. It can also refer to the conscious processing of a given piece of information, such as being conscious of a person that just entered the room. In this review we will focus on the later meaning. Contrary to what introspection suggests, only a small fraction of all the information reaching our senses can be the object of verbal report or voluntary action. Although verbal reportability is one of the main measures of conscious perception, there are many situations in which we can be conscious of some information that we cannot report, essentially because it vanishes from consciousness very quickly. In this paper, we will review studies that have used verbal reports of perceptual objects as a measure of consciousness. On the other hand, attentional processes refer to a heterogeneous set of functions, subserved by partially distinct neurocognitive systems. We will refer to attention as a mechanism for the selection of information, in its different varieties of orienting, alerting, and executive control (Posner and Cohen, 1984). We will particularly focus on the relationship between distinct forms of spatial attention and conscious perception.
Historically, attention and consciousness have been intrinsically linked. Introspection suggests that when we attend to an object or part of a scene we become conscious of it. Removing attention away from the object makes it fade from consciousness. Although there seems to be a consensus on the fact that some level of general alertness is needed in order to consciously perceive (Robertson et al., 1998; Dehaene and Changeux, 2011; Kusnir et al., 2011), the relationship between spatial attention and conscious perception has proven intriguing and difficult to explore empirically. James (1890) originally provided an influential definition of the interplay between attention and conscious perception: “(attention) is the taking possession of the mind, in clear and vivid form, of one out of several simultaneously possible objects or trains of thought.” This view led many to posit that spatial attention and conscious perception are inextricably related (Posner, 1994; O’Regan and Noë, 2001; Chun and Marois, 2002; Bartolomeo, 2008). Although most of the models do not propose that the mechanism of attention is the mechanism of consciousness itself (Posner, 1994), they implicate that consciousness emerges from the processing of attentional systems that filter out information from our crowded environment. Attentional selection is therefore considered a necessary, although maybe not sufficient, condition for consciousness. Others directly equate attentional capture and consciousness. Simons (2000) for example, distinguished between implicit and explicit attentional capture. Implicit attentional capture refers to stimuli that can speed up performance or affect eye movements without being consciously detected (Theeuwes, 1994; Theeuwes et al., 1998). Explicit attentional capture refers to stimuli that affect performance and are consciously detected. According to Simons (2000), implicit effects on behavior might not embody all aspects of attentional capture, while explicit attentional capture is equated to consciousness, i.e., it is assumed that if participants consciously reported the stimuli is because they captured spatial attention.
Some lines of evidence support the existence of a tight relationship between spatial attention and consciousness. The most classical example of interaction between the two processes is observed in the inattentional blindness paradigm, where salient changes in the features of visual stimuli are missed when unattended (Mack and Rock, 1998), even when stimuli are presented at the fovea. Moreover, such phenomenon is enhanced when the deployment of attention is challenged by increased levels of perceptual load (Lavie, 2006). It has been postulated that inattentional blindness is not produced by a lack of attention but by a lack of expectation (Braun, 2001; Mack, 2001), although expectation can be considered as a form of top-down attention (Asplund et al., 2010). One of the most striking examples of the influence of attention in consciousness has been demonstrated by the selective looking task (Neisser and Becklen, 1975). In one of the versions of this paradigm, participants were attentionally engaged in counting the passes made by two basketball teams. After some time, a man wearing a gorilla costume walked across the display. Surprisingly, 35% of the participants did not see the gorilla, which was instead detected 100% of the times when attention was not engaged in counting the passes (Simons and Chabris, 1999). These and other experiments have provided definitive evidence that important changes in our visual world can be missed when unattended.
Strong evidence supporting the existence of a link between spatial attention and conscious perception also comes from right brain-damaged patients affected by left spatial neglect. These patients suffer from damage to the right parietal lobe, or to its connections with the ipsilateral prefrontal cortex (PFC; Thiebaut de Schotten et al., 2005; Bartolomeo et al., 2007). Patients with left brain damage may also show signs of contralesional, right-sided neglect, albeit more rarely, and usually in a less severe form (Bartolomeo et al., 2001a; Beis et al., 2004). Although patients’ visual capabilities can be intact, severe problems in attentional orienting are observed. Patients frequently miss contralesionally presented stimuli, especially when there is competing information in the ipsilesional visual field. In other words, neglect patients’ acquired inability to orient attention toward the contralesional left hemifield makes them unaware of stimuli presented within the neglected space (Bartolomeo, 2007). This suggests a strong link between the brain circuits underlying spatial orienting and the putative neural correlates of conscious perception (Figure 1).
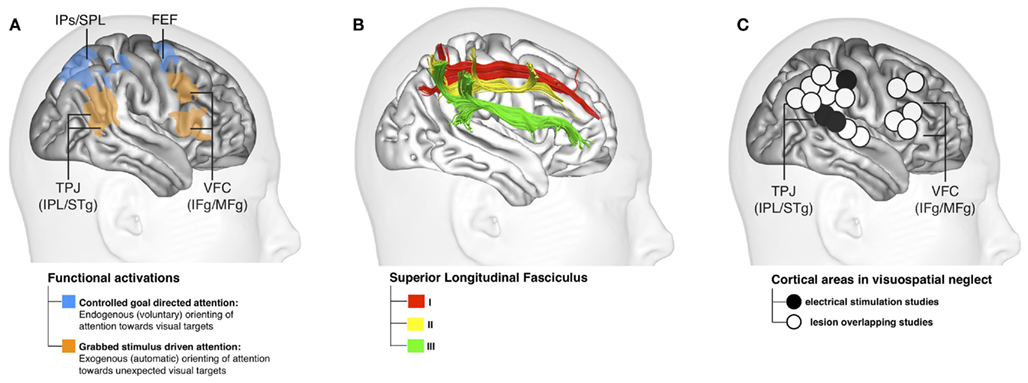
Figure 1. (A) Right-hemisphere networks of visuospatial attention according to Corbetta and Shulman (2002); (B) The three branches of the Superior Longitudinal Fasciculus according to Thiebaut de Schotten et al. (2011); (C) Brain regions associated to visual neglect in different studies (modified from Thiebaut de Schotten et al., 2011). The figure represents the anatomical brain regions associated to spatial attention, the white matter branches that might connect them, and the anatomical overlap of lesions causing neglect after damage to spatial attentional networks.
Dissociations between Spatial Attention and Conscious Perception
Challenging the classical view of attention as a gateway to consciousness, some studies have reported dissociations between some forms of spatial attention and conscious perception. Some of these dissociations have been described in the blindsight patient GY (Kentridge et al., 1999, 2004; Schurger et al., 2006). Blindsight can be observed after lesions in the primary visual cortex of one or both hemispheres (Weiskrantz, 1986). Although patients report to be blind in the contralesional visual field, and therefore not conscious of visual stimulation, they can perform above chance in some tasks such as guessing the orientation of movement (Weiskrantz, 1986). They can also navigate avoiding obstacles in a room, while denying to see them (de Gelder et al., 2008). The study of these patients is especially interesting in research on consciousness, because their accuracy in detecting or discriminating information in the blind hemifield can sometimes be comparable to stimuli reported as being consciously perceived. To study the relationship between spatial attention and conscious perception, Kentridge and colleagues (Kentridge et al., 1999; see also Kentridge et al., 2004) presented the blindsight patient GY with targets in the blind hemifield preceded by endogenous cues in the fovea or exogenous peripheral cues in the blind hemifield. Both cues speeded up responses to targets, even though the patient denied seeing targets as well as peripheral cues. This result demonstrates that after damage to the primary visual cortex, attention can be deployed, and speed up responses, in the absence of consciousness for cues or targets. Thus, GY can pay attention to visual information unavailable to verbal report.
Analogous dissociations between spatial attention and consciousness have been reported in normal observers. In a Posner-type paradigm, where attention was oriented by using spatially predictive central cues (arrows), non-consciously perceived primes (which were masked by subsequent targets) presented at attentionally cued locations, sped up responses when they were color-congruent rather than incongruent with the target (Kentridge et al., 2008). Importantly, in this case attention did not allow participants to consciously report the primes, showing once more that spatial attention can be deployed in the absence of conscious perception of the attended information.
Koch and Tsuchiya (2007) have also recently reviewed some situations in which endogenous or top-down attention can be dissociated from conscious perception. For example, there are some situations in which participants attend without being conscious of the attended information. In visual crowding, for example, the orientation of a grating can be made unconscious, but it still produces an aftereffect that is supposed to require focal attention (He et al., 1996). It has also been demonstrated that priming for invisible (masked) words is only observed if participants are attending to the moment in time where the prime–target pair will occur. However, in this case, attended words do not reach consciousness (Naccache et al., 2002). Feature-based attention can also spread to invisible stimuli (Melcher et al., 2005; Kanai et al., 2006), once again demonstrating that some forms of attention deploy without subsequent conscious perception of the attended information.
There seem to be other situations in which consciousness happens in the near absence of attention. For example, the gist of a visual scene is immune to inattentional blindness (Mack and Rock, 1998), and can be discriminated in 30 ms, too short a time to develop top-down attention. This observation was already made by Posner (1994), who remarked that attention seemed to be needed for focal awareness, but not for awareness of the background (Iwasaki, 1993). With attention focused to the center of the display in a dual task, participants can determine if the scene contains an animal or a vehicle, but cannot perform a simpler task, such as distinguishing a colored disk (Li et al., 2002). Following the feature binding model (Treisman and Gelade, 1980), spatial attention is considered to be important for feature integration but not for single feature extraction. It is possible that when stimuli are complex, feature integration is not necessary, because the processing of multiple single features can be enough to discriminate the object.
It is crucial to note that all these previous studies reviewed by Koch and Tsuchiya (2007) investigated the relationship between endogenous (or top-down) forms of spatial attention and conscious perception. By using magneto-encephalography, it has also been recently reported that endogenous spatial attention, oriented using central arrow cues, can be electrophysiologically dissociated from conscious perception in visual areas of the occipital cortex (Wyart and Tallon-Baudry, 2008). Whether they were attended or not, consciously perceived stimuli modulated mid-frequency gamma-band activity over the contralateral visual cortex, whereas spatial attention modulated high-frequency gamma-band activity, independent of whether targets were consciously perceived or not. This constitutes a neural dissociation of attention and conscious perception, at least in visual areas of the cortex (although see Chica et al., submitted; described below). Finally, opposite effects of endogenous attention and consciousness have been observed on afterimages (van Boxtel et al., 2010). While manipulating attention via a demanding central task, stimulus visibility was simultaneously manipulated using a perceptual suppression procedure. van Boxtel and colleagues demonstrated that attention and consciousness produced opposite effects on afterimages: while attention decreased their duration, consciousness enhanced it. Altogether, the results from these studies suggest that top-down amplification or endogenous attention is neither necessary nor sufficient for consciousness.
Exogenous Spatial Attention Interactions with Conscious Perception
It is now well established that the orienting system of the human brain is not unitary. Spatial attention can be oriented either endogenously (i.e., top-down, guided by task demands, or by goals of the task at hand) or exogenously (i.e., bottom-up, driven by the saliency of stimulation, such as in attentional capture). These attentional systems are implemented in partially different brain regions (Corbetta and Shulman, 2002; Chica et al., 2011a), and produce differential effects on information processing (Klein, 2004; Chica et al., 2006). Important components of these networks include the dorsolateral PFC and the posterior parietal cortex (PPC). Physiological studies indicate that these two structures show interdependence of neural activity (Buschman and Miller, 2007). In the monkey, analogous PPC and PFC areas show coordinated activity when the animal selects a visual stimulus as a saccade target. Importantly, PFC and PPC show distinctive dynamics of interaction when attention is selected by the stimulus (bottom-up or exogenous orienting) or when it is directed by more top-down (or endogenous) goals. Bottom-up signals appear first in the parietal cortex and are characterized by an increase of fronto-parietal coherence in the gamma-band, whereas top-down signals emerge first in the frontal cortex and tend to synchronize in the beta band (Buschman and Miller, 2007). Within the right parietal cortex, regions such as the intraparietal sulcus (IPS) seem related to both endogenous and exogenous spatial attention, while the temporo-parietal junction (TPJ) is exclusively implicated in exogenous spatial attention (Chica et al., 2011a; see also Friedrich et al., 1998). Therefore, the finding that endogenous attention and conscious perception dissociate does not necessarily imply the same conclusion for exogenous attention.
Previous research on patients with right brain damage and left visual neglect (characterized by unawareness for left-sided objects) has consistently demonstrated that consciousness deficits in neglect are systematically associated to impairments of exogenous spatial orienting; endogenous orienting, on the other hand, can be relatively spared, if slowed, in these patients (Bartolomeo and Chokron, 2002). Deficits in exogenous orienting in neglect patients typically take the form of an immediate rightward orienting of attention as soon as the visual scene unfolds (Gainotti et al., 1991; D’Erme et al., 1992), followed by the so called “disengagement deficit” (Posner et al., 1984; Friedrich et al., 1998; Losier and Klein, 2001). When presented with a right-sided, ipsilesional peripheral cue followed by a left-sided, contralesional target, neglect patients respond extremely slow, and may miss the target altogether. This result is usually interpreted as an impairment of the disengagement of attention from ipsilesional stimuli. The deficit is enhanced by the presence of bilateral placeholder markers in the display (Gainotti et al., 1991; D’Erme et al., 1992; Rastelli et al., 2008), which presumably increase attentional capture from ipsilesional, right-sided objects (Bartolomeo et al., 2004). Interestingly, when peripheral cues are made spatially predictive of the future location of the target, the disengagement deficit ameliorates (Bartolomeo and Chokron, 2002). For example, if the ipsilesional cue (presented on the right hemifield) predicts with high probability target appearance on the contralesional (left) hemifield (which is known as counterpredictive cues), participants’ responses are faster and less targets are missed than if the peripheral cue is not spatially predictive (Bartolomeo et al., 2001b; Figure 2). This indicates that brain lesions associated to neglect and causing severe deficits in consciously detecting contralesionally presented information (Thiebaut de Schotten et al., 2005; Bartolomeo et al., 2007), mostly affect exogenous rather than endogenous spatial attention.
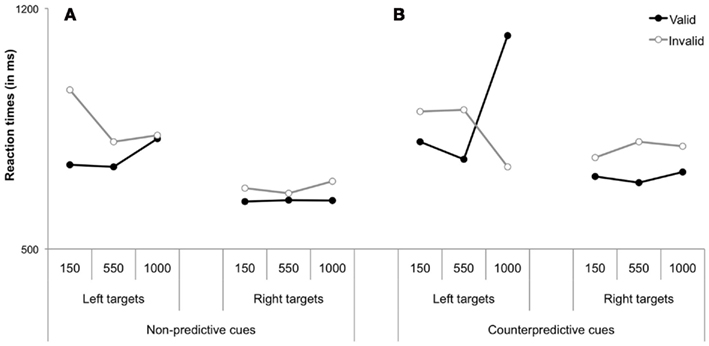
Figure 2. Mean neglect patients’ reaction times to detect a peripheral target preceded by (A) a spatially non-predictive peripheral cue or (B) a counterpredictive peripheral cue, i.e., a cue indicating target appearance at the opposite location (data from Bartolomeo et al., 2001b). The disengagement deficit is observed for left-targets presented at invalid vs. valid locations at the short (50 ms) SOA for non-predictive cues. When cues are counterpredictive, the disengagement deficit decreases (see results for left-sided targets, 50 ms SOA), and neglect patients can take into account the information provided by the cue, responding faster at the attended (invalid) location than at the unattended (valid) location at the longest (1000 ms) SOA for left-presented targets.
This clinical observation made us hypothesize that although endogenous spatial attention can be dissociated from conscious perception (Kentridge et al., 1999; Lamme, 2003; Koch and Tsuchiya, 2007; Wyart and Tallon-Baudry, 2008), exogenous attention might instead be an important antecedent of our conscious experience. To test for this hypothesis, we presented normal participants with near-threshold stimuli, preceded either by central symbolic cues or by peripheral cues (Chica et al., 2011b). In order to avoid the involuntary orienting produced by some central cues, such as arrows (Ristic et al., 2002), we used purely symbolic cues (letters or colors) indicating the more likely location of target appearance. Target contrast was manipulated so that participants could only perceive a proportion of the targets. If attentional orienting increased target conscious perception, more targets should be reported at the attended than at the unattended location. Consistent with previous findings (Kentridge et al., 1999; Lamme, 2003; Koch and Tsuchiya, 2007; Wyart and Tallon-Baudry, 2008) when spatial attention was endogenously oriented using central symbolic cues, weak, or null modulations of conscious reports were observed (Chica et al., 2011b)1. However, exogenous orienting triggered by peripheral cues produced strong and consistent modulations of conscious reports, and was able to increase conscious detection rates at the attended vs. the unattended location (Chica et al., 2011b).
Using electroencephalographic (EEG) recordings, we have also demonstrated that the attentional capture produced by the peripheral cue correlates with subsequent conscious reports of near-threshold targets (Chica et al., 2010, in press). We used non-predictive peripheral cues, which capture spatial attention exogenously, and observed that a cue-related event-related potential (ERP), the P100 component, was strictly linked to subsequent conscious reports (Chica et al., 2010). Importantly, the cue-related P100 was larger for subsequently seen targets than for unseen targets when attentional cues were valid; in contrast, P100 was larger for subsequently unseen than for seen targets when attentional cues were invalid (Figure 3). The P100 component elicited by the cue might well index the capture of attention that the cue produced. Thus, if valid cues captured attention to the location of the impending target, then more targets would be consciously perceived at that location. However, if an invalid cue captured attention to a wrong location, fewer targets would be consciously perceived. Correlations between the attentional capture produced by the cue and subsequent conscious reports were observed even when cue-related responses were considered on a trial-by-trial basis. Using a paradigm in which endogenous and exogenous orienting are manipulated during the same trial, it has also been demonstrated, within the same experimental design, that while exogenous attentional capture interacts with the conscious perception of near-threshold targets, endogenous orienting can be dissociated from conscious reports (Chica et al., in press). This clearly demonstrates that exogenous attention is an important modulator of conscious perception (see also Koivisto et al., 2009), and that the state of the attentional system before the target is presented modulates our conscious experience (see also Super et al., 2003; Linkenkaer-Hansen et al., 2004; Dehaene and Changeux, 2005).
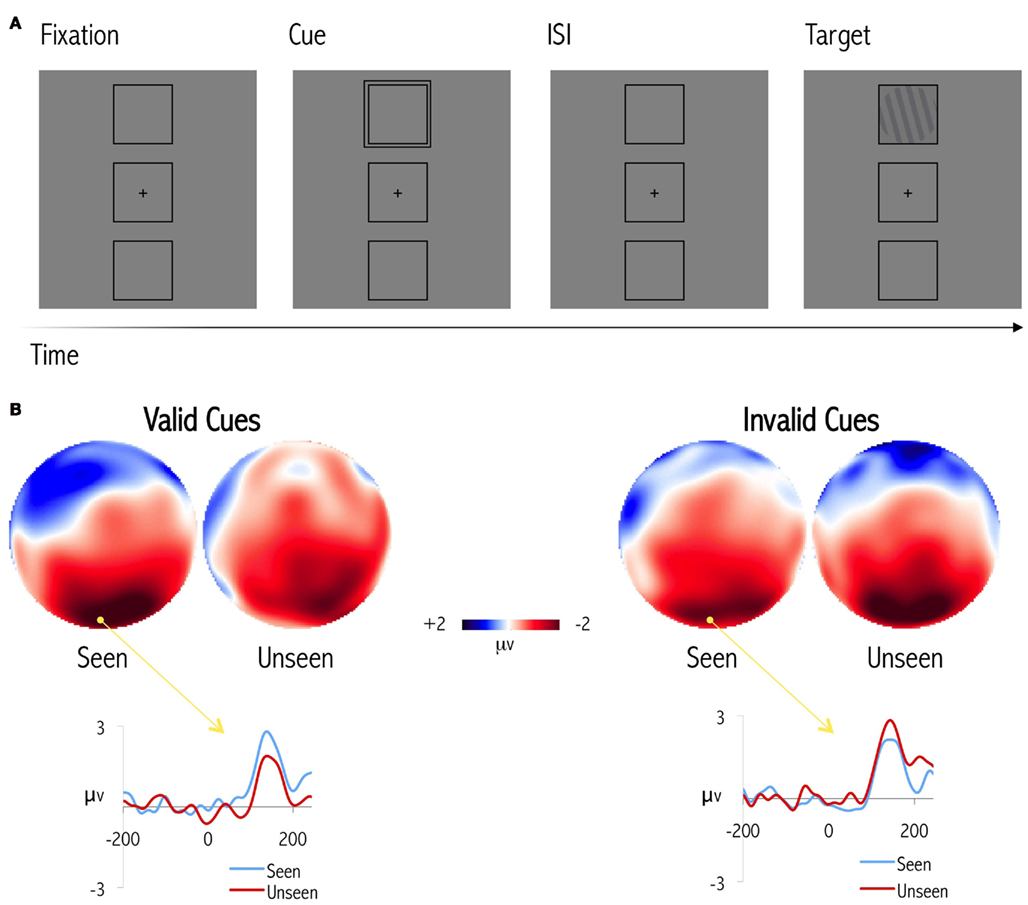
Figure 3. (A) Graphical illustration of a paradigm used to manipulate spatial attention and conscious perception (Chica et al., 2010, 2011b, in press, submitted). (B) Topographic distribution of the P100 effect, 120 ms after cue appearance; and event-related cue-locked potential waveforms for valid and invalid cues, leading to seen and unseen reports (adapted from Chica et al., 2010). The figure shows that for valid cues, P100 is larger for subsequently seen than unseen targets; for invalid cues, P100 is instead larger for subsequently unseen than seen targets.
Although some of the studies reviewed in the previous section have demonstrated that endogenous spatial attention can be dissociated from conscious perception, this has not always been the case. The seminal studies suggesting an interdependence between spatial attention and consciousness, such as inattentional blindness (Mack and Rock, 1998) or the selective looking task (Neisser and Becklen, 1975), manipulated endogenous spatial attention and measured conscious reports. Research on visual search has also demonstrated that at least under certain conditions, salient distractors might not capture attention if they do not share any relevant feature with the target (Folk et al., 1992). For example, when searching for a red letter, only red distractors will capture attention, while other colored distractors will not. This is known as “contingent attentional capture,” a phenomenon that demonstrates how top-down or endogenous expectancies interact with the exogenous attentional capture produced by the stimuli themselves. It is possible that while endogenous attention does not determine our conscious experience when there is no competing stimulation (Wyart and Tallon-Baudry, 2008; Chica et al., 2011b, in press), it does play a role when information has to be selected from crowded environments (such as in the inattentional blindness paradigm or selective looking task; see Koivisto and Revonsuo, 2010; Dehaene and Changeux, 2011), or when endogenous expectancies interact with exogenous attentional capture (such as in “contingent attentional capture” paradigms). This proposal is coherent with models postulating that conscious and non-conscious perception depend on perceptual load; information can be selected out by attention under high levels of perceptual load, while more information can be consciously processed under low levels of perceptual load (Lavie, 2006; Macdonald and Lavie, 2008). Endogenous attention might thus modulate consciousness only when its functioning is required by high levels of perceptual load in crowded environments.
Exogenous Spatial Attention is not Sufficient for Conscious Perception
In the previous section we have reviewed evidence indicating that exogenous spatial attention is an important antecedent of our conscious experience. However, there are also many observations demonstrating that exogenous attentional capture does not always lead to conscious perception. As stated above, exogenous peripheral cues presented in the blind hemifield of the blindsight patient GY, sped up responses to the target, in the absence of conscious perception of the cues or targets (Kentridge et al., 1999, 2008). Similarly, in healthy participants, subliminal peripheral cues have been observed to speed up responses to targets presented at the same spatial location, demonstrating attentional deployment in the absence of consciousness of the visually presented cue (McCormick, 1997; Lambert et al., 1999). Further evidence have demonstrated attentional capture or pre-attentitive orienting to unresolvable Gabor patches embedded among simple luminance patches, while participants could not consciously distinguish between the two (Rajimehr, 2004). This is in line with evidence of attentional capture without consciousness of the feature that captured spatial attention.
Other studies have shown that exogenous spatial attention modulates the processing of masked stimuli that are not consciously perceived (Lachter et al., 2004; Marzouki et al., 2007). In some cases, exogenous peripheral cues are sufficient to generate priming when the primes and targets occupy different spatial locations. This result indicates that exogenous peripheral cues produce effects at early stages of visual processing (Finkbeiner and Forster, 2008), boosting the signals from primary visual areas. However, the presence of the mask disrupts further processing, avoiding conscious perception of the targets.
Woodman and Luck (2003) used an “object substitution masking” paradigm to explore the role of attention in conscious perception. In this paradigm, an object presented in a crowded environment is masked by the presentation of small objects surrounding it; when the mask offsets sometime after the display onset, the masked object is not consciously perceived. In Woodman and Luck’s study, the N2pc ERP component (a N200 observed at parietal sites, reflecting attentional capture) was used to index the orienting of attention to the target. Their results showed that the N2pc was elicited both when the target was consciously perceived and when it was not, leading the authors to conclude that attention and conscious perception are two independent processes, and that orienting of attention did not intrinsically produce conscious reports. However, as noted by the authors, the N2pc ended earlier when targets were not consciously perceived. If N2pc is a correlate of exogenous orienting of attention, these results can be interpreted as supporting the idea that exogenous attention is an important modulator of conscious perception. The fact the N2pc ended earlier when the stimulus was not consciously reported might indicate that even if the target produced an exogenous attentional capture, the corresponding fronto-parietal activation was unable to maintain the exogenous capture of attention long enough to trigger the necessary reverberation of information required for conscious processing (see below).
There is also accumulating evidence demonstrating that distractors can capture exogenous attention in visual search tasks and affect performance and eye movements, while participants are completely unconscious of the presence of these distractors and their influence on their behavior (Theeuwes, 1994; Theeuwes et al., 1998). In oculomotor capture paradigms, attentional capture is reflected by inappropriate eye movements to irrelevant distractors. Importantly, participants are not conscious of the eye movements elicited by distractors during search. All these results clearly indicate that attentional capture does not always lead to the conscious perception of the attended information.
Necessary Conditions for Conscious Perception
The studies reviewed in the two previous sections indicate that at least some forms of attention, such as exogenous spatial attention, might be necessary, although by no means sufficient, for conscious perception. Some models have proposed other processes as necessary for consciousness, such as recurrent processing of information within functionally interconnected brain regions (Dehaene and Naccache, 2001; Dehaene et al., 2006; Lamme, 2006; Fahrenfort et al., 2007). During information processing, two neural processes have been distinguished: a feedforward sweep (earlier activation of cells in successive areas of the visual hierarchy) followed by recurrent processing (recurrent interactions between neurons within an area and other neurons that activated earlier at lower levels). According to a recent model (Lamme, 2003), conscious perception needs recurrent processing. This is an interesting approach because it does not point to any isolated brain region as the neural correlate of conscious perception; instead, the reverberation of information within functionally connected brain regions is deemed important. Transcranial magnetic stimulation (TMS) studies have stressed the importance of recurrent processing. For example, TMS-mediated V1 disruption prevents consciousness at a time point far from the feedforward sweep of information (Walsh and Cowey, 1998). Moreover, TMS in visual area V5 (MT) produces motion sensation, unless V1 is stimulated at a later moment in time (Pascual-Leone and Walsh, 2001), which also indicates the importance of early visual areas in later stages of processing that are crucial for recurrent processing.
So far, for the quest for the neural correlate of consciousness have led to controversial results (see Rees et al., 2002a; Dehaene and Changeux, 2011; for reviews). When contrasting consciously seen vs. unseen stimuli, some authors have proposed that consciousness is related to activity in the thalamus and brain stem (Paus, 2000), in visual areas along the ventral cortical visual stream (Bar and Biederman, 1999; Grill-Spector et al., 2000; Moutoussis and Zeki, 2002; Pins and ffytche, 2003; Ress and Heeger, 2003; Zeki, 2003; Tse et al., 2005), or in parietal and prefrontal regions (Crick and Koch, 1995; Beck et al., 2001; Dehaene and Naccache, 2001; Rees et al., 2002a; Dehaene et al., 2003; Dehaene and Changeux, 2005; Marois and Ivanoff, 2005; Chica et al., submitted).
When using masking procedures, activity in V1 is related to conscious reports, and drawing attention away from the stimulus does not produce activity in fronto-parietal areas, but in visual areas (Tse et al., 2005). Additionally, lesions in cortical visual areas destroy conscious perception (as in cortical blindness, homonymous hemianopia, or cerebral achromatopsia), which also indicate that conscious perception needs the activity of early visual regions. Based on these sort of data, some models propose that visual consciousness resides in each particular area in charge of processing the relevant feature (Zeki, 2003). According to these proposals, consciousness of a color resides in V4, a region of the visual cortex putatively involved in color processing. However, early visual activation is not always sufficient for consciousness. For example, activity in early visual areas can be observed even when participants deny seeing the stimuli (Dehaene et al., 2001; Vuilleumier et al., 2001; Moutoussis and Zeki, 2002; Marois et al., 2004; Sergent et al., 2005). Primary visual cortex can also be selectively activated in response to perceptually indiscriminable orientation information, indicating that V1 is not sufficient for generating conscious reports (Rajimehr, 2004). The existence of high-order processing of orientation in the absence of consciousness has also been reported, demonstrating interactions between V1 and V4, and V1 and V5 (Rajimehr, 2004). Moreover, when invisibility is caused by masking (Dehaene et al., 2001) or dichoptic stimulation (Moutoussis and Zeki, 2002), activity in early visual areas is weak, which can invite the conclusion that consciousness needs a stronger activation of these regions. However, when invisibility is caused by neglect or inattention, activity in early visual areas can be strong (Vuilleumier et al., 2001; Marois et al., 2004; Sergent et al., 2005). In the case of spatial neglect, visual areas are often intact, but patients can act as if they were completely blind for the information presented in the contralesional hemi-space.
Other studies have related the emergence of conscious states to the activity in parieto-frontal structures (Crick and Koch, 1995; Beck et al., 2001; Dehaene and Naccache, 2001; Rees et al., 2002a; Dehaene et al., 2003; Dehaene and Changeux, 2005; Marois and Ivanoff, 2005; Chica et al., submitted). These sets of data have been used to substantiate “high-order” theories of consciousness (see Lau and Rosenthal, 2011; for a recent review), which postulate that consciousness depends on neural activity in prefrontal and parietal regions, although consciousness might not add a significant utility or immediate impact on behavioral and task performance. Other models (Dehaene and Naccache, 2001; Dehaene et al., 2006) also propose the importance of prefrontal and parietal areas for consciousness, and underline the strong links of conscious processing to the flexible control of behavior, cognitive control, and the ability to perform various tasks. Dehaene and his colleagues have proposed a model in which both bottom-up stimulus strength and top-down attentional amplification are jointly needed for conscious perception; however, these features might not always be sufficient for a stimulus to cross the threshold for conscious perception. They propose the existence of two types of non-conscious processes: subliminal and pre-conscious. According to the authors, subliminal processing (i.e., information that does not reach consciousness but can affect our behavior) is the consequence of bottom-up activation of lower sensory areas that is insufficient to trigger a large-scale reverberating process to create the conditions for conscious perception. In contrast, pre-conscious processing refers to neural processes that can potentially access consciousness (i.e., they carry enough activation), but those are temporally inaccessible due to the lack of top-down attentional amplification.
Both Lamme’s (2003) model and Dehaene and colleagues’ model (Dehaene and Naccache, 2001; Dehaene et al., 2006) can be helpful to understand the controversial set of data found when consciously seen and unseen reports have been compared using different paradigms. Masked stimuli produce feedfoward activation in V1, the inferior temporal cortex, frontal eye fields, and the motor cortex. However, neurophysiological manifestations of recurrent interaction are suppressed by backward masking (Lamme and Roelfsema, 2000; Lamme et al., 2002), which prevents the stimulus to reach consciousness. When masked stimuli are unattended, only occipito-temporal activation is recorded (Tse et al., 2005). When they are attended, however, activity is observed in both early visual areas and fronto-parietal regions (Dehaene et al., 2001; Haynes et al., 2005). Nevertheless, attention is not sufficient for a masked stimulus to reach consciousness, because the mask prevents recurrent processing from fronto-parietal regions to visual areas (see below). Similarly, blindsight patients can process (and respond to) unreported visual information, but due to their lesions of the visual cortex, recurrent processing from fronto-parietal regions to visual areas is altered, thus preventing consciousness to occur.
Near-threshold stimuli also differ in the activity they evoke in early visual areas and fronto-parietal regions (Pins and ffytche, 2003; Ress and Heeger, 2003; Palva et al., 2005). Their perception depends on several factors, such as recurrent processing, alertness (Kusnir et al., 2011), the amount of spontaneous activity before stimulus presentation (Super et al., 2003; Linkenkaer-Hansen et al., 2004; Dehaene and Changeux, 2005), and exogenous attentional capture to their spatial location (Chica et al., 2010, 2011b, in press, submitted). Using supra-threshold targets, previous work has consistently demonstrated that exogenous (as well as endogenous) attention increases contrast appearance (see, e.g., Pestilli and Carrasco, 2005; Carrasco, 2006). It could then be argued that exogenous attention increases conscious reports of near-threshold targets by a similar perceptual mechanism as that increasing target contrast at the exogenously attended location. This would imply that, contrary to our proposal, exogenous attention might not be necessary for conscious perception; it might only enhance such conscious perceiving. This interpretation is consistent with models proposing an important role of early visual and/or occipito-temporal areas in conscious perception (Super et al., 2001; Lamme et al., 2002; Pins and ffytche, 2003; Zeki, 2003; Tse et al., 2005; Lamme, 2006). However, when near-threshold stimuli are made invisible under conditions of inattention, late differences involving fronto-parietal activation are often reported for seen vs. unseen stimuli (Vogel et al., 1998; Beck et al., 2001; Rees et al., 2002b; Gross et al., 2004; Marois et al., 2004; Pessoa and Ungerleider, 2004; Haynes et al., 2005; Sergent et al., 2005), which is consistent with models proposing that conscious perception emerges from the recurrent activity of fronto-parietal regions, and its long-distance reverberation with occipital areas (Dehaene and Naccache, 2001; Dehaene et al., 2006).
Recent functional magnetic imaging (fMRI) data from our group (Chica et al., submitted) also support an important role of functionally connected fronto-parietal networks in conscious perception and in the interactions between spatial attention and consciousness. fMRI signals were recorded while participants responded to near-threshold stimuli preceded by peripheral cues. Functional connectivity analyses during the orienting period (i.e., during the processing of the attentional cue, before the target was presented) demonstrated that activity in a slightly right-lateralized fronto-parietal network (including the bilateral superior and inferior parietal lobes, the left frontal eye field, the right insula, and right inferior frontal gyrus) was tightly correlated to spatial attention and conscious reports. Strong coupling within this network correlated with conscious reports when targets were presented at the attended location; however, it correlated with “unseen” reports when targets were presented at unattended locations. Coupling within this network is associated to the efficiency of attentional orienting, which is directly linked to the facilitatory effects of spatial orienting on visual consciousness. Fronto-parietal interactions can therefore be primed by attentional processes, thus increasing the likelihood of conscious reports. Evidence of interactions between spatial attention and consciousness was observed in fronto-parietal regions, but not in lower level visual areas. This result is consistent with previous reports of neural dissociations between spatial attention and consciousness in the visual cortex (Wyart and Tallon-Baudry, 2008).
Based on the fact that some stimuli do not reach consciousness even when they are attended (Cumming and Parker, 1997; Zeki and Marini, 1998; Leopold and Logothetis, 1999; Enns and Di Lollo, 2000; He and MacLeod, 2001; Intriligator and Cavanagh, 2001; Super et al., 2001), Lamme (2003) proposed that attention might not determine whether stimuli reach consciousness, but whether they can be reported. Attention would determine whether the representation of stimuli is stable enough in working memory to allow reportability. For example, in a change blindness paradigm cueing the item that might change can prevent blindness. But blindness is also prevented if the relevant item is cued long after the first stimulus (T1) has disappeared and before the onset of the second stimulus (T2; Becker et al., 2000; Landman et al., 2003). After T1 has disappeared, its representation is accessible, and cuing can select information from working memory. However, when T2 is presented, the representation vanishes, and cuing does not help anymore. This suggests that there is a short-lived, vulnerable, and not easily reportable representation of visual experience, and a more stable and reportable representation form of consciousness. In the case of change blindness, there is a general consensus on the fact that focal spatial attention is needed in order to perceive the change. In the absence of such attentional processes, the contents of visual memory are overwritten by subsequent stimuli and cannot be used to make comparisons (Rensink et al., 1997). Koivisto and Revonsuo (2010) have formulated a related proposal based on ERP studies. Early differences on occipital ERPs (around 200–300 ms after stimulus onset) are proposed to be linked to short-lived, non-reportable representations of visual experiences, while later differences in parieto-frontal sites (around 400 ms after stimulus onset) might be more related to conscious and reportable representations. According to their proposal, spatial attention is a necessary prerequisite for both kinds of representations, at least when there is competition between stimuli (Koivisto et al., 2009).
These proposals are reminiscent of the distinction made by Block (1996) between phenomenal and access consciousness, and of a related, time-honored distinction between a form of immediate experience, not amenable to verbal description, and a reflective form of consciousness that can be verbally reported (Merleau-Ponty, 1942; Bartolomeo and Dalla Barba, 2002). According to the above mentioned evidence, attention seems necessary to go from phenomenal to access consciousness. Based on our recent observations (Chica et al., 2010, 2011b, in press, submitted) we propose that exogenously attended information is always phenomenally represented, which is not the case for endogenously attended information in the absence of exogenous attentional capture. This can explain why endogenous spatial attention can be electrophysiologically dissociated from consciousness (Wyart and Tallon-Baudry, 2008; Chica et al., in press) while exogenous spatial attention is not (Chica et al., 2010, in press, submitted). However, in order to access consciousness and reportability, information has to be endogenously attended in order to enter the reverberating flow of information within fronto-parietal regions (van Gaal and Fahrenfort, 2008). This might be the reason why making peripheral exogenous cues spatially predictive increases the behavioral modulation produced on conscious perception as compared to non-predictive cues, and modulates not only the proportion of consciously reported stimuli and decision criteria, but also the perceptual sensitivity to detect near-threshold stimuli (Chica et al., 2011b). From a physiological point of view, it is plausible that both feedforward processing (perhaps modulated by exogenous attention), and recurrent processing (perhaps enhanced by endogenous attention) in large-scale brain networks are important mechanisms to allow a stable pattern of activity of visual working memory that determines our reportable conscious experience.
Concluding Remarks
Spatial attention and conscious perception have been historically linked, though some recent studies have shown dissociations between the two processes. In the present paper we reviewed evidence indicating that although endogenous or top-down spatial attention can sometimes be dissociated from conscious reports (mainly when there is no competing information), exogenous, or bottom-up spatial attention seems to be an important antecedent of our conscious experience. Nevertheless, exogenous attentional capture per se is not a sufficient condition for conscious access. Other processes such as alerting, recurrent processing and patterns of spontaneous brain activity before the stimulus occurs are proposed as being necessary for a stimulus to be consciously perceived and reported.
Even though during the last decades consciousness studies have provided important insights about conscious and unconscious processing in the human brain, many questions remain unresolved (Lau, 2008). One of the most important issues to be solved experimentally is the search for an objective measure of phenomenal consciousness. Nowadays, consciousness is measured with verbal reports or voluntary action. Although many believe that we are conscious of much information we cannot report, there is a current controversy about the existence of forms of consciousness that would not be amenable to verbal report. It has been argued that consciousness cannot be separated from the brain mechanisms supporting it, such as attention, working memory, or decision taking (Cohen and Dennett, 2011). In classical examples such as the Sperling’s partial report experiment (Sperling, 1960), participants are presented with a display of 9–12 letters. Typically, only some of the items are available to verbal report. However, when cued to report a subset of letters, participants can entirely report whatever subset is cued, which might indicate that at some point they were conscious of the whole subset. Although this result is a crucial argument to claim that we are conscious of more we can report, Cohen and Dennett offer an alternative explanation (not far from Sperling’s original one): once the cue is presented, participants are able to access an unconscious representation before it decays. From this point of view, there would be no form of experience not amenable to conscious report. This proposition strongly links consciousness with high-level cognitive functions such as attention, claiming that only attended items will be consciously represented. Other proposals also posit that the dissociation between phenomenal and access consciousness is equivocal, suggesting that phenomenal consciousness might be caused by perceptual illusions and non-conscious processing (Kouider et al., 2010). These authors propose that perceptual representations vary from complete unawareness of stimuli that can eventually be processed and affect behavior while remaining inaccessible to conscious reports, to complete awareness of information that can be verbally reported. There exist other situations of partial consciousness, which correspond to intermediate cases, with conscious access only at same levels. In this latter case, access can be filled in with perceptual illusions (Kouider et al., 2010). According to this hypothesis, perceptual representations are gradually represented, although conscious access can be an all-or-none process, as proposed by other models (Baars, 1989; Sergent and Dehaene, 2004).
These theoretical and empirical issues become especially relevant in the study of consciousness in non-human animals and in human beings who are incapable of communicating. Some effort is being devoted in this sense, for example in the study of vegetative and minimally conscious states. Simple cognitive tasks are being used to determine the level of consciousness of non-communicative patients. Neurophysiological measures extracted from EEG (Bekinschtein et al., 2009) or fMRI (Cruse and Owen, 2010) are being used to determine the state of consciousness of these patients and even to try to predict whether patients will recover from coma (Faugeras et al., 2011). A better definition and measurement of phenomenal and access consciousness will certainly enable us to better explore the relationships between different forms of spatial and non-spatial attention and consciousness, as well as their underlying brain mechanisms in both the healthy and damaged brain.
Conflict of Interest Statement
The authors declare that the research was conducted in the absence of any commercial or financial relationships that could be construed as a potential conflict of interest.
Acknowledgments
Ana B. Chica was supported with a postdoctoral grant from the Marie Curie Intra-European Program (FP7), Ramón y Cajal fellowship from the Spanish Ministry of Education and Science, and research project (PSI2008-03595PSIC). We thank Michel Thiebaut de Schotten for drawing Figure 1.
Footnote
- ^Other studies have reported significant modulations on the proportion of consciously reported targets when spatial attention was endogenously oriented using central cues (Wyart and Tallon-Baudry, 2008; Hsu et al., 2011). However, in these studies, Signal Detection Theory (Green and Swets, 1966) parameters such as perceptual sensitivity (d′) and response criterion (beta) could not be separately calculated for attended and unattended targets (see Chica et al., 2011b, where these analyses were performed). The fact that more false alarms (conscious reports of target presence when no target was actually presented) were committed when central cues were spatially predictive (12% in Hsu et al., 2011, Experiment 1) than when they were not (6% in Hsu et al., 2011, Experiment 1), together with the finding of significant differences in the general d′ between spatially predictive and non-predictive cues, and close to significance differences in response criterion [t(13) = 1.64, p = 0.11], strongly suggests that participants may have adopted a stricter response criterion to report targets at the unattended vs. the attended location, especially when central cues were spatially predictive of target appearance (Hsu et al., 2011).
References
Asplund, C. L., Todd, J. J., Snyder, A. P., and Marois, R. (2010). A central role for the lateral prefrontal cortex in goal-directed and stimulus-driven attention. Nat. Neurosci. 13, 507–512.
Bar, M., and Biederman, I. (1999). Localizing the cortical region mediating visual awareness of object identity. Proc. Natl. Acad. Sci. U.S.A. 96, 1790–1793.
Bartolomeo, P. (2008). Varieties of attention and of consciousness: evidence from neuropsychology. Psyche 14. Available at: http://www.theassc.org/journal_psyche
Bartolomeo, P., and Chokron, S. (2002). Orienting of attention in left unilateral neglect. Neurosci. Biobehav. Rev. 26, 217–234.
Bartolomeo, P., Chokron, S., and Gainotti, G. (2001a). Laterally directed arm movements and right unilateral neglect after left hemisphere damage. Neuropsychologia 39, 1013–1021.
Bartolomeo, P., Siéroff, E., Decaix, C., and Chokron, S. (2001b). Modulating the attentional bias in unilateral neglect: the effects of the strategic set. Exp. Brain Res. 137, 432–444.
Bartolomeo, P., and Dalla Barba, G. (2002). Varieties of consciousness (Commentary on Perruchet and Vinter: the self-organizing consciousness). Behav. Brain Sci. 25, 331–332.
Bartolomeo, P., Thiebaut de Schotten, M., and Doricchi, F. (2007). Left unilateral neglect as a disconnection syndrome. Cereb. Cortex 17, 2479–2490.
Bartolomeo, P., Urbanski, M., Chokron, S., Chainay, H., Moroni, C., Siéroff, E., Belin, C., and Halligan, P. (2004). Neglected attention in apparent spatial compression. Neuropsychologia 42, 49–61.
Beck, D., Rees, G., Frith, C. D., and Lavie, N. (2001). Neural correlates of change detection and change blindness. Nat. Neurosci. 4, 645–650.
Becker, M. W., Pashler, H., and Anstis, S. M. (2000). The role of iconic memory in change-detection tasks. Perception 29, 273–286.
Beis, J. M., Keller, C., Morin, N., Bartolomeo, P., Bernati, T., Chokron, S., Leclercq, M., Louis-Dreyfus, A., Marchal, F., Martin, Y., Perennou, D., Pradat-Diehl, P., Prairial, C., Rode, G., Rousseaux, M., Samuel, C., Sieroff, E., Wiart, L., and Azouvi, P. and French Collaborative Study Group on Assessment of Unilateral Neglect (GEREN/GRECO). (2004). Right spatial neglect after left hemisphere stroke: qualitative and quantitative study. Neurology 63, 1600–1605.
Bekinschtein, T. A., Dehaene, S., Rohaut, B., Tadel, F., Cohen, L., and Naccache, L. (2009). Neural signature of the conscious processing of auditory regularities. Proc. Natl. Acad. Sci. U.S.A. 106, 1672–1677.
Block, N. (1996). How can we find the neural correlate of consciousness? Trends Neurosci. 19, 456–459.
Braun, J. (2001). It’s great but not necessarily about attention. Commentary on: Arien Mack, and Irvin Rock (1998). Inattentional blindness. Cambridge, MA: The MIT Press. Psyche 7. Available at: http://www.theassc.org/journal_psyche
Buschman, T. J., and Miller, E. K. (2007). Top-down versus bottom-up control of attention in the prefrontal and posterior parietal cortices. Science 315, 1860–1862.
Carrasco, M. (2006). Covert attention increases contrast sensitivity: psychophysical, neurophysiological, and neuroimaging studies. Prog. Brain Res. 154, 33–70.
Chica, A. B., Bartolomeo, P., and Valero-Cabré, A. (2011a). Dorsal and ventral parietal contributions to spatial orienting in the human brain. J. Neurosci. 31, 8143–8149.
Chica, A. B., Lasaponara, S., Chanes, L., Valero-Cabré, A., Doricchi, F., Lupiáñez, J., and Bartolomeo, P. (2011b). Spatial attention and conscious perception: the role of endogenous and exogenous orienting. Atten. Percept. Psychophys. 73, 1065–1081.
Chica, A. B., Botta, F., Lupiáñez, J., and Bartolomeo, P. (in press). Spatial attention and conscious perception: interactions and dissociations between and within endogenous and exogenous processes. Neuropsychologia.
Chica, A. B., Lasaponara, S., Lupiáñez, J., Doricchi, F., and Bartolomeo, P. (2010). Exogenous attention can capture perceptual consciousness: ERP and behavioural evidence. Neuroimage 51, 1205–1212.
Chica, A. B., Lupiáñez, J., and Bartolomeo, P. (2006). Dissociating inhibition of return from the endogenous orienting of spatial attention: evidence from detection and discrimination tasks. Cogn. Neuropsychol. 23, 1015–1034.
Chun, M. M., and Marois, R. (2002). The dark side of visual attention. Curr. Opin. Neurobiol. 12, 184–189.
Cohen, M. A., and Dennett, D. C. (2011). Consciousness cannot be separated from function. Trends Cogn. Sci. (Regul. Ed.) 15, 358–364.
Corbetta, M., and Shulman, G. L. (2002). Control of goal-directed and stimulus-driven attention in the brain. Nat. Rev. Neurosci. 3, 201–215.
Crick, F., and Koch, C. (1995). Are we aware of neural activity in primary visual cortex? Nature 375, 121–123.
Cruse, D., and Owen, A. M. (2010). Consciousness revealed: new insights into the vegetative and minimally conscious states. Curr. Opin. Neurol. 23, 656–660.
Cumming, B. G., and Parker, A. J. (1997). Responses of primary visual cortical neurons to binocular disparity without depth perception. Nature 389, 280–283.
de Gelder, B., Tamietto, M., van Boxtel, G., Goebel, R., Sahraie, A., van den Stock, J., Stienen, B. M., Weiskrantz, L., and Pegna, A. (2008). Intact navigation skills after bilateral loss of striate cortex. Curr. Biol. 18, R1128–R1129.
Dehaene, S., and Changeux, J. P. (2005). Ongoing spontaneous activity controls access to consciousness: a neuronal model for inattentional blindness. PLoS Biol. 3, e141. doi:10.1371/journal.pbio.0030141
Dehaene, S., and Changeux, J. P. (2011). Experimental and theoretical approaches to conscious processing. Neuron 70, 200–227.
Dehaene, S., Changeux, J. P., Naccache, L., Sackur, J., and Sergent, C. (2006). Conscious, preconscious, and subliminal processing: a testable taxonomy. Trends Cogn. Sci. (Regul. Ed.) 10, 204–211.
Dehaene, S., and Naccache, L. (2001). Towards a cognitive neuroscience of consciousness: basic evidence and a workspace framework. Cognition 79, 1–37.
Dehaene, S., Naccache, L., Cohen, L., Bihan, D. L., Mangin, J. F., Poline, J. B., and Rivière, D. (2001). Cerebral mechanisms of word masking and unconscious repetition priming. Nat. Neurosci. 4, 752–758.
Dehaene, S., Sergent, C., and Changeux, J. P. (2003). A neuronal network model linking subjective reports and objective physiological data during conscious perception. Proc. Natl. Acad. Sci. U.S.A. 100, 8520–8525.
D’Erme, P., Robertson, I., Bartolomeo, P., Daniele, A., and Gainotti, G. (1992). Early rightwards orienting of attention on simple reaction time performance in patients with left-sided neglect. Neuropsychologia 30, 989–1000.
Enns, J. T., and Di Lollo, V. (2000). What’s new in visual masking? Trends Cogn. Sci. (Regul. Ed.) 4, 345–352.
Fahrenfort, J. J., Scholte, H. S., and Lamme, V. A. (2007). Masking disrupts reentrant processing in human visual cortex. J. Cogn. Neurosci. 19, 1488–1497.
Faugeras, F., Rohaut, B., Weiss, N., Bekinschtein, T. A., Galanaud, D., Puybasset, L., Bolgert, F., Sergent, C., Cohen, L., Dehaene, S., and Naccache, L. (2011). Probing consciousness with event-related potentials in the vegetative state. Neurology 77, 264–268.
Finkbeiner, M., and Forster, K. I. (2008). Attention, intention and domain-specific processing. Trends Cogn. Sci. (Regul. Ed.) 12, 59–64.
Folk, C. L., Remington, R. W., and Johnston, J. C. (1992). Involuntary covert orienting is contingent on attentional control settings. J. Exp. Psychol. Hum. Percept. Perform. 18, 1030–1044.
Friedrich, F. J., Egly, R., Rafal, R. D., and Beck, D. (1998). Spatial attention deficits in humans: a comparison of superior parietal and temporal-parietal junction lesions. Neuropsychology 12, 193–207.
Gainotti, G., D’Erme, P., and Bartolomeo, P. (1991). Early orientation of attention toward the half space ipsilateral to the lesion in patients with unilateral brain damage. J. Neurol. Neurosurg. Psychiatr. 54, 1082–1089.
Grill-Spector, K., Kushnir, T., Hendler, T., and Malach, R. (2000). The dynamics of object-selective activation correlate with recognition performance in humans. Nat. Neurosci. 3, 837–843.
Gross, J., Schmitz, F., Schnitzler, I., Kessler, K., Shapiro, K., Hommel, B., and Schnitzler, A. (2004). Modulation of long-range neural synchrony reflects temporal limitations of visual attention in humans. Proc. Natl. Acad. Sci. U.S.A. 101, 13050–13055.
Haynes, J. D., Driver, J., and Rees, G. (2005). Visibility reflects dynamic changes of effective connectivity between V1 and fusiform cortex. Neuron 46, 811–821.
He, S., Cavanagh, P., and Intriligator, J. (1996). Attentional resolution and the locus of visual awareness. Nature 383, 334–337.
He, S., and MacLeod, D. I. (2001). Orientation-selective adaptation and tilt after-effect from invisible patterns. Nature 411, 473–476.
Hsu, S. M., George, N., Wyart, V., and Tallon-Baudry, C. (2011). Voluntary and involuntary spatial attentions interact differently with awareness. Neuropsychologia 49, 2465–2474.
Intriligator, J., and Cavanagh, P. (2001). The spatial resolution of visual attention. Cogn. Psychol. 43, 171–216.
Kanai, R., Tsuchiya, N., and Verstraten, F. A. (2006). The scope and limits of top-down attention in unconscious visual processing. Curr. Biol. 16, 2332–2336.
Kentridge, R. W., Heywood, C. A., and Weiskrantz, L. (1999). Attention without awareness in blindsight. Proc. Biol. Sci. 266, 1805–1811.
Kentridge, R. W., Heywood, C. A., and Weiskrantz, L. (2004). Spatial attention speeds discrimination without awareness in blindsight. Neuropsychologia 42, 831–835.
Kentridge, R. W., Nijboer, T. C., and Heywood, C. A. (2008). Attended but unseen: visual attention is not sufficient for visual awareness. Neuropsychologia 46, 864–869.
Klein, R. M. (2004). “On the control of visual orienting,” in Cognitive Neuroscience of Attention, ed. M. I. Posner (New York: Guilford Press), 29–44.
Koch, C., and Tsuchiya, N. (2007). Attention and consciousness: two distinct brain processes. Trends Cogn. Sci. (Regul. Ed.) 11, 16–22.
Koivisto, M., Kainulainen, P., and Revonsuo, A. (2009). The relationship between awareness and attention: evidence from ERP responses. Neuropsychologia 47, 2891–2899.
Koivisto, M., and Revonsuo, A. (2010). Event-related brain potential correlates of visual awareness. Neurosci. Biobehav. Rev. 34, 922–934.
Kouider, S., de Gardelle, V., Sackur, J., and Dupoux, E. (2010). How rich is consciousness? The partial awareness hypothesis. Trends Cogn. Sci. (Regul. Ed.) 14, 301–307.
Kusnir, F., Chica, A. B., Mitsumasu, M. A., and Bartolomeo, P. (2011). Phasic auditory alerting improves visual conscious perception. Conscious. Cogn. 20, 1201–1210.
Lachter, J., Forster, K. I., and Ruthruff, E. (2004). Forty-five years after Broadbent (1958): still no identification without attention. Psychol. Rev. 111, 880–913.
Lambert, A., Naikar, N., McLahan, K., and Aitken, V. (1999). A new component of visual orienting: implicit effects of peripheral information and subthreshold cues on covert attention. J. Exp. Psychol. Hum. Percept. Perform. 25, 321–340.
Lamme, V. A. (2003). Why visual attention and awareness are different. Trends Cogn. Sci. (Regul. Ed.) 7, 12–18.
Lamme, V. A. (2006). Towards a true neural stance on consciousness. Trends Cogn. Sci. (Regul. Ed.) 10, 494–501.
Lamme, V. A., and Roelfsema, P. R. (2000). The distinct modes of vision offered by feedforward and recurrent processing. Trends Neurosci. 23, 571–579.
Lamme, V. A., Zipser, K., and Spekreijse, H. (2002). Masking interrupts figure-ground signals in V1. J. Cogn. Neurosci. 14, 1044–1053.
Landman, R., Spekreijse, H., and Lamme, V. A. (2003). Large capacity storage of integrated objects before change blindness. Vision Res. 43, 149–164.
Lau, H. (2008). “Are we studying consciousness yet?” in Frontiers of Consciousness: Chichele Lectures, eds L. Weiskrantz, and M. Davies (Oxford: Oxford University Press), 245–258.
Lau, H., and Rosenthal, D. (2011). Empirical support for higher-order theories of conscious awareness. Trends Cogn. Sci. (Regul. Ed.) 15, 365–373.
Leopold, D. A., and Logothetis, N. K. (1999). Multistable phenomena: changing views in perception. Trends Cogn. Sci. (Regul. Ed.) 3, 254–264.
Li, F. F., VanRullen, R., Koch, C., and Perona, P. (2002). Rapid natural scene categorization in the near absence of attention. Proc. Natl. Acad. Sci. U.S.A. 99, 9596–9601.
Linkenkaer-Hansen, K., Nikulin, V. V., Palva, S., Ilmoniemi, R. J., and Palva, J. M. (2004). Prestimulus oscillations enhance psychophysical performance in humans. J. Neurosci. 24, 10186–10190.
Losier, B. J., and Klein, R. M. (2001). A review of the evidence for a disengage deficit following parietal lobe damage. Neurosci. Biobehav. Rev. 25, 1–13.
Macdonald, J. S., and Lavie, N. (2008). Load induced blindness. J. Exp. Psychol. Hum. Percept. Perform. 34, 1078–1091.
Mack, A. (2001). Reply to commentaries on: Arien Mack, and Irvin Rock (1998). Inattentional blindness. Cambridge, MA: The MIT Press. Psyche 7. Available at: http://www.theassc.org/journal_psyche
Marois, R., and Ivanoff, J. (2005). Capacity limits of information processing in the brain. Trends Cogn. Sci. (Regul. Ed.) 9, 296–305.
Marois, R., Yi, D. J., and Chun, M. M. (2004). The neural fate of consciously perceived and missed events in the attentional blink. Neuron 41, 465–472.
Marzouki, Y., Grainger, J., and Theeuwes, J. (2007). Exogenous spatial cueing modulates subliminal masked priming. Acta Psychol. (Amst.) 126, 34–45.
McCormick, P. A. (1997). Orienting attention without awareness. J. Exp. Psychol. Hum. Percept. Perform. 23, 168–180.
Melcher, D., Papathomas, T. V., and Vidnyanszky, Z. (2005). Implicit attentional selection of bound visual features. Neuron 46, 723–729.
Moutoussis, K., and Zeki, S. (2002). The relationship between cortical activation and perception investigated with invisible stimuli. Proc. Natl. Acad. Sci. U.S.A. 99, 9527–9532.
Naccache, L., Blandin, E., and Dehaene, S. (2002). Unconscious masked priming depends on temporal attention. Psychol. Sci. 13, 416–424.
Neisser, U., and Becklen, R. (1975). Selective looking: attending to visually specified events. Cogn. Psychol. 7, 480–494.
O’Regan, J. K., and Noë, A. (2001). A sensorimotor account of vision and visual consciousness. Behav. Brain Sci. 24, 939–1031.
Palva, S., Linkenkaer-Hansen, K., Naatanen, R., and Palva, J. M. (2005). Early neural correlates of conscious somatosensory perception. J. Neurosci. 25, 5248–5258.
Pascual-Leone, A., and Walsh, V. (2001). Fast backprojections from the motion to the primary visual area necessary for visual awareness. Science 292, 510–512.
Paus, T. (2000). Functional anatomy of arousal and attention systems in the human brain. Prog. Brain Res. 126, 65–77.
Pessoa, L., and Ungerleider, L. G. (2004). Neural correlates of change detection and change blindness in a working memory task. Cereb. Cortex 14, 511–520.
Pestilli, F., and Carrasco, M. (2005). Attention enhances contrast sensitivity at cued and impairs it at uncued locations. Vision Res. 45, 1867–1875.
Pins, D., and ffytche, D. (2003). The neural correlates of conscious vision. Cereb. Cortex 13, 461–474.
Posner, M. I. (1994). Attention: the mechanisms of consciousness. Proc. Natl. Acad. Sci. U.S.A. 91, 7398–7403.
Posner, M. I., and Cohen, Y. (1984). “Components of visual orienting,” in Attention and Performance X, eds H. Bouma and D. Bouwhuis (London: Lawrence Erlbaum), 531–556.
Posner, M. I., Walker, J. A., Friedrich, F. J., and Rafal, R. D. (1984). Effects of parietal injury on covert orienting of attention. J. Neurosci. 4, 1863–1874.
Rastelli, F., Funes, M. J., Lupiáñez, J., Duret, C., and Bartolomeo, P. (2008). Left visual neglect: is the disengage deficit space- or object-based? Exp. Brain Res. 187, 439–446.
Rees, G., Kreiman, G., and Koch, C. (2002a). Neural correlates of consciousness in humans. Nat. Rev. Neurosci. 3, 261–270.
Rees, G., Wojciulik, E., Clarke, K., Husain, M., Frith, C., and Driver, J. (2002b). Neural correlates of conscious and unconscious vision in parietal extinction. Neurocase 8, 387–393.
Rensink, R. A., O’Regan, J. K., and Clark, J. J. (1997). To see or not to see: the need for attention to perceive changes in scenes. Psychol. Sci. 8, 368–373.
Ress, D., and Heeger, D. J. (2003). Neuronal correlates of perception in early visual cortex. Nat. Neurosci. 6, 414–420.
Ristic, J., Friesen, C. K., and Kingstone, A. (2002). Are eyes special? It depends on how you look at it. Psychon. Bull. Rev. 9, 507–513.
Robertson, I. H., Mattingley, J. B., Rorden, C., and Driver, J. (1998). Phasic alerting of neglect patients overcomes their spatial deficit in visual awareness. Nature 395, 169–172.
Schurger, A., Cowey, A., and Tallon-Baudry, C. (2006). Induced gamma-band oscillations correlate with awareness in hemianopic patient GY. Neuropsychologia 44, 1796–1803.
Sergent, C., Baillet, S., and Dehaene, S. (2005). Timing of the brain events underlying access to consciousness during the attentional blink. Nat. Neurosci. 8, 1391–1400.
Sergent, C., and Dehaene, S. (2004). Is consciousness a gradual phenomenon? Evidence for an all-or-none bifurcation during the attentional blink. Psychol. Sci. 15, 720–728.
Simons, D. J. (2000). Attentional capture and inattentional blindness. Trends Cogn. Sci. (Regul. Ed.) 4, 147–155.
Simons, D. J., and Chabris, C. F. (1999). Gorillas in our midst: sustained inattentional blindness for dynamic events. Perception 28, 1059–1074.
Sperling, G. (1960). The information available in brief visual presentations. Psychol. Monogr. 74, 1–29.
Super, H., Spekreijse, H., and Lamme, V. A. (2001). Two distinct modes of sensory processing observed in monkey primary visual cortex (V1). Nat. Neurosci. 4, 304–310.
Super, H., van der Togt, C., Spekreijse, H., and Lamme, V. A. (2003). Internal state of monkey primary visual cortex (V1) predicts figure-ground perception. J. Neurosci. 23, 3407–3414.
Theeuwes, J. (1994). Stimulus-driven capture and attentional set: selective search for color and visual abrupt onsets. J. Exp. Psychol. Hum. Percep. Perform. 20, 799–806.
Theeuwes, J., Kramer, A. F., Hahn, S., and Irwin, D. E. (1998). Our eyes do not always go where we want them to go: capture of the eyes by new objects. Psychol. Sci. 9, 379–385.
Thiebaut de Schotten, M., Dell’Acqua, F., Forkel, S. J., Simmons, A., Vergani, F., Murphy, D. G. M., and Catani, M. (2011). A lateralized brain network for visuospatial attention. Nat. Neurosci. 14, 1245–1246.
Thiebaut de Schotten, M., Urbanski, M., Duffau, H., Volle, E., Lévy, R., Dubois, B., Volle, E., Lévy, R., Dubois, B., and Bartolomeo, P. (2005). Direct evidence for a parietal-frontal pathway subserving spatial awareness in humans. Science 5744, 2226–2228.
Treisman, A., and Gelade, G. (1980). A feature-integration theory of attention. Cogn. Psychol. 12, 97–136.
Tse, P. U., Martinez-Conde, S., Schlegel, A. A., and Macknik, S. L. (2005). Visibility, visual awareness, and visual masking of simple unattended targets are confined to areas in the occipital cortex beyond human V1/V2. Proc. Natl. Acad. Sci. U.S.A. 102, 17178–17183.
van Boxtel, J. J., Tsuchiya, N., and Koch, C. (2010). Opposing effects of attention and consciousness on afterimages. Proc. Natl. Acad. Sci. U.S.A. 107, 8883–8888.
van Gaal, S., and Fahrenfort, J. J. (2008). The relationship between visual awareness, attention, and report. J. Neurosci. 28, 5401–5402.
Vogel, E. K., Luck, S. J., and Shapiro, K. L. (1998). Electrophysiological evidence for a postperceptual locus of suppression during the attentional blink. J. Exp. Psychol. Hum. Percept. Perform. 24, 1656–1674.
Vuilleumier, P., Sagiv, N., Hazeltine, E., Poldrack, R. A., Swick, D., Rafal, R. D., and Gabrieli, J. D. (2001). Neural fate of seen and unseen faces in visuospatial neglect: a combined event-related functional MRI and event-related potential study. Proc. Natl. Acad. Sci. U.S.A. 98, 3495–3500.
Walsh, V., and Cowey, A. (1998). Magnetic stimulation studies of visual cognition. Trends Cogn. Sci. (Regul. Ed.) 2, 103–110.
Woodman, G. F., and Luck, S. J. (2003). Dissociations among attention, perception, and awareness during object-substitution masking. Psychol. Sci. 14, 605–611.
Wyart, V., and Tallon-Baudry, C. (2008). Neural dissociation between visual awareness and spatial attention. J. Neurosci. 28, 2667–2679.
Keywords: attention, conscious perception, endogenous, exogenous, fronto-parietal networks, neglect
Citation: Chica AB and Bartolomeo P (2012) Attentional routes to conscious perception. Front. Psychology 3:1. doi: 10.3389/fpsyg.2012.00001
Received: 28 September 2011;
Accepted: 03 January 2012;
Published online: 18 January 2012.
Edited by:
Jeroen J. A. van Boxtel, University of California Los Angeles, USAReviewed by:
David Rosenthal, City University of New York, USAGiorgio Marchetti, www.mind-consciousness-language.com, Italy
Copyright: © 2012 Chica and Bartolomeo. This is an open-access article distributed under the terms of the Creative Commons Attribution Non Commercial License, which permits non-commercial use, distribution, and reproduction in other forums, provided the original authors and source are credited.
*Correspondence: Ana B. Chica, Department of Experimental Psychology, University of Granada, Campus de Cartuja S/N, CP 18071 Granada, Spain. e-mail:YW5hY2hpY2FAdWdyLmVz