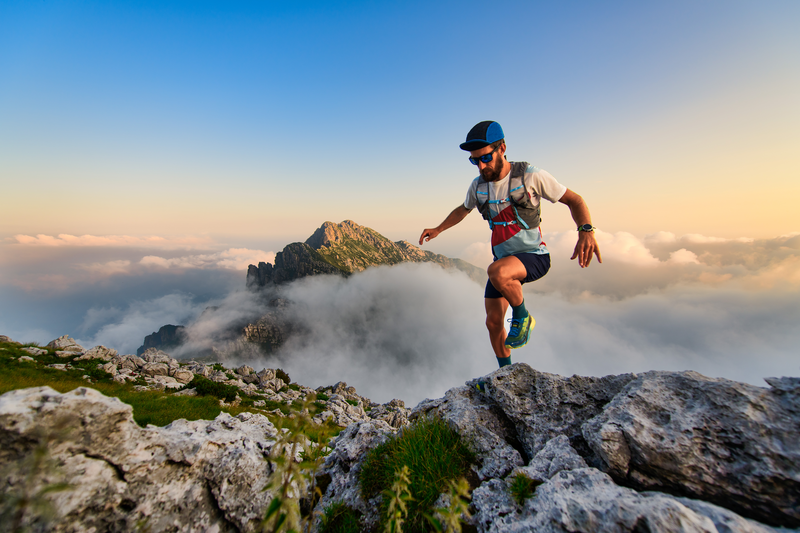
94% of researchers rate our articles as excellent or good
Learn more about the work of our research integrity team to safeguard the quality of each article we publish.
Find out more
ORIGINAL RESEARCH article
Front. Psychiatry
Sec. ADHD
Volume 16 - 2025 | doi: 10.3389/fpsyt.2025.1475889
The final, formatted version of the article will be published soon.
You have multiple emails registered with Frontiers:
Please enter your email address:
If you already have an account, please login
You don't have a Frontiers account ? You can register here
Background: Attention Deficit Hyperactivity Disorder (ADHD) is a common neurodevelopmental disorder characterized by inattention, hyperactivity, and impulsivity. A core cognitive deficit in ADHD is executive function (EF) impairment, which significantly impacts daily life. Methylphenidate (MPH) is a widely used first-line treatment for ADHD, but objective biomarkers to assess treatment response are lacking. The aim of this study was to investigate the effects of MPH on executive function and identify potential neural biomarkers of response in children with ADHD using electroencephalogram (EEG). Methods: A total of 26 boys with ADHD (mean age 8.64 ± 1.30 years) participated in the study. All participants were treated with 18 mg/day of oral extended-release MPH in the morning for 8 weeks. Executive function was assessed using the BRIEF2 and Digit Span Test (DST), and eventrelated potentials (ERP) were measured at baseline and after 8 weeks of MPH treatment. Results: After 8 weeks of MPH treatment, significant improvements were observed in several executive function domains. BRIEF2 scores, including inhibition, self-monitoring, shifting, emotional control, initiation, working memory, planning/organisation, task monitoring, and material organization, were significantly reduced (P < 0.05). Behavioral performance in the Go/NoGo task also improved, with shorter correct response times and higher accuracy rates (P = 0.002, P = 0.009). EEG results revealed
Keywords: Attention Deficit Hyperactivity Disorder, Children, Executive Function, Methylphenidate, P300
Received: 08 Aug 2024; Accepted: 24 Feb 2025.
Copyright: © 2025 Wang, Yang, Yang, Yang, Yang, Wen, Wang, Liu and Luo. This is an open-access article distributed under the terms of the Creative Commons Attribution License (CC BY). The use, distribution or reproduction in other forums is permitted, provided the original author(s) or licensor are credited and that the original publication in this journal is cited, in accordance with accepted academic practice. No use, distribution or reproduction is permitted which does not comply with these terms.
* Correspondence:
Rong Luo, West China Second University Hospital, Sichuan University, Chengdu, China
Disclaimer: All claims expressed in this article are solely those of the authors and do not necessarily represent those of their affiliated organizations, or those of the publisher, the editors and the reviewers. Any product that may be evaluated in this article or claim that may be made by its manufacturer is not guaranteed or endorsed by the publisher.
Research integrity at Frontiers
Learn more about the work of our research integrity team to safeguard the quality of each article we publish.