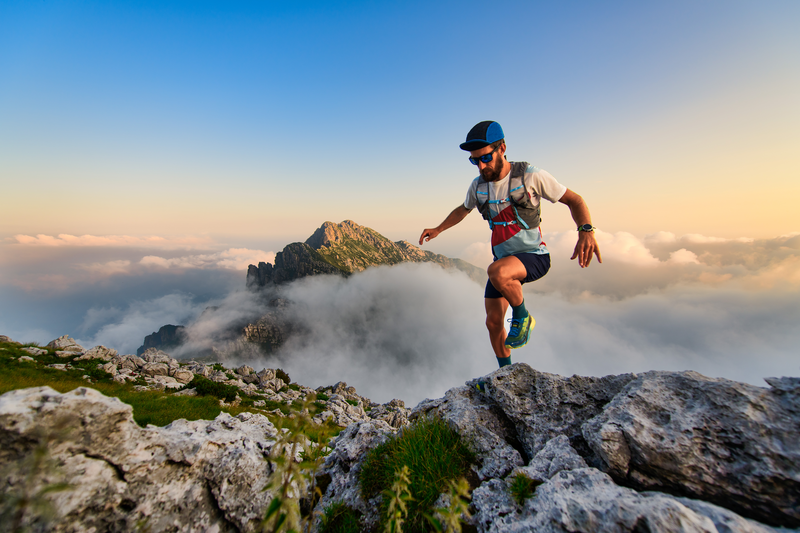
94% of researchers rate our articles as excellent or good
Learn more about the work of our research integrity team to safeguard the quality of each article we publish.
Find out more
MINI REVIEW article
Front. Psychiatry , 07 January 2025
Sec. Mood Disorders
Volume 15 - 2024 | https://doi.org/10.3389/fpsyt.2024.1492415
This article is part of the Research Topic Non-Neuronal Cells in Neurodegenerative Diseases and Psychiatric Disorders View all 5 articles
In this review, it is evaluated the progress in the application of stem cell therapy to ameliorate the symptoms of bipolar disorder, major depression, schizophrenia, and autism. These disorders are highly prevalent in clinical medicine and are responsible for high levels of psychosocial disability among patients. All of them share common biomedical features, such as complex and variable genetic substrates, significant susceptibility to environmental changes, and insufficient knowledge of their pathogenesis. In addition, the responsiveness of patients to pharmacological treatment is heterogeneous, and in some cases, no treatment is available. Therefore, the development of stem cell-based regenerative medicine and its possible combination with emerging therapeutic approaches that promote neural plasticity are expected to advance neuropsychiatry in the next few decades.
The identification of stem cells in adult organisms, including humans, combined with the design of methodological approaches to transform adult cells into pluripotent stem cells (iPSCs) (1), has opened a new field of extraordinary importance in medicine for the progress of diagnosis and biological characterization of distinct pathologies and for the treatment of diseases for which we previously had no pharmacological tools (2).
Physiologically, stem cells are undifferentiated cells that self-renew and eventually differentiate into specific cell lineages (3). In adults, stem cells are present in many tissues and organs and often occupy niches that maintain their “stemness” (4). Under normal conditions, these cells are attracted by their neighboring tissues to replace damaged cells, ensuring tissue homeostasis (5). However, their regenerative potential is insufficient to overcome a pathological situation. In clinical practice, autologous or allogeneic stem cells can be isolated from the bone marrow, adipose tissue, or umbilical cord blood and expanded in vitro to obtain a sufficient number of cells required for cell therapy. A hallmark in modern medicine was the development of iPSCs by genetic reprogramming adult somatic cells in vitro (6), thus overcoming the limitations in obtaining the number of cells required for therapeutic procedures.
The beneficial effects expected from the utilization of stem cell-based medicine are not only due to the direct replacement of damaged tissues (7) but also to the release of paracrine factors and extracellular vesicles (exosomes) that interact with the target tissues (8, 9) (Figure 1). In fact, it has been found that exosomes delivered by stem cells to the milieu may substitute the employ of the living stem cells. Stem cells, can also be used as carriers for drug delivery to injured organs (2, 10, 11). The latter is favored because of the tropism of stem cells toward damaged tissues (12). In the nervous system, a limitation of the therapeutic use of stem cells is the difficulty of overcoming the blood–brain barrier by noninvasive mechanisms. Advances in the nasal administration of cells or cell products may circumvent the use of invasive approaches (13). The use of experimental animals for the study of psychiatric disorders is limited, but, stem cells grown in different culture assays provide a valuable tool for modeling the pathophysiological substrates of neuropsychiatric disorders. Thus, a complementary and equally important medical application of stem cell technology is the development of tridimensional cultures from iPSCs obtained from patients. These cultures, termed “organoids,” can be directed to differentiate into specific tissue structures and allow monitoring the pathogenic basis of the disease and to test their response to pharmacological treatments, thus predicting their efficacy in a personalized fashion (14, 15).
Figure 1. Schematic representation of the central events of stem cell therapy. From left to right: 1) potential source of stem cells; 2) administration procedure; 3) expected effects of stem cell administration. ST, stem cells ready for infusion or engraftment, EX, exosomes released by stem cells.
In the last years important advances have been achieved in obtaining neural-like cells for in vitro modelling psychiatric disorders, avoiding reprograming and methodological limitations of iPSC derived from the patients, such as accessibility to obtain the cells, efficiency and time required for differentiation, economical cost, and, of note, the maintenance of the epigenetic signature of the patient cells that is loss during reprograming process (16). Among the sources of neural cells, it can be emphasized the olfactory neuroepithelium (17–19), but somatic cells (i.e. fibroblasts) transfected with lineage-determining transcription factors via gene editing that not require reprogramming (20), and mesenchymal stem cells, or circulating pluripotent monocytes transdifferentiated by growing in conditioned culture medium provide also satisfactory results (21).
The purpose of this review is to summarize the current status of the application of stem cell technology to ameliorate the symptomatology of bipolar disorder (BD), major depression (MD), schizophrenia (SQZ), and Autism Spectrum Disorder (ASD). These disorders are most prevalent in clinical medicine occupying the second (Depressive disorders), seventeenth (SQZ) and twenty-first (ASD) places in the last rank generated by the “Global Burden of Diseases, Injuries, and Risk Factors Study” (22) that estimates the global disease burden, on the basis of incidence, prevalence, and disability-adjusted life- years (DALYs) for 371 diseases at both country and regional levels. The advancement of stem cell-based regenerative medicine and its possible combination with emerging therapeutic approaches that promote neural plasticity opens a promising panorama for the advancement of neuropsychiatry (23).
BD is a highly prevalent disorder, affecting more than 1% of the world population. It is characterized by alternating manic and major depressive episodes, and usually develops in young individuals. Despite the influence of environmental factors on the evolution of BD, its genetic origin is accepted. However, rather than single mutations, complex and variable genetic modifications have been identified in individuals and families (24). Since many decades, clinical treatment to reduce manic episodes of BD patients relays in the administration of mood stabilizers (lithium, valproate, and lamotrigine) although their action mechanism was not fully understood (25) and a considerable number of patients fail to respond to the medication.
Studies using stem cell technology have paid much attention to decipher the molecular and cellular basis of the disorder and to unravel the action mechanism of mood stabilizers, including the variability in the responsiveness to lithium treatment (26–30). Organoids made with iPSC obtained from BD patients, revealed important molecular and structural alterations respect to control subjects that are ameliorated by addition of lithium to the culture medium, and, importantly, this in vitro assay replicates the responsiveness of the patient to treatment (26, 29–31). This property constitutes a major step to the development of personalized medicine in clinical practice.
The use of organoid technology provided important advances in the understanding of BD pathogenesis (26). Functional and transcriptional studies have revealed increased expression and response to pro-inflammatory cytokines (IL-6) in astrocytes derived from patient iPSCs (31), supporting a potential therapeutic effect of anti-inflammatory treatments (32), to inhibit neuroinflammation (27). Furthermore, genes regulating neuronal differentiation, and plasticity, such as the Wnt/β-catenin signaling pathway appear altered in BD-patients (33, 34), and the influence of Wnt signaling inhibitors in the vitro assay opened new approaches for the treatment of the disorder (35). The microRNA miR34 is another target of lithium altered in BD-derived organoids. It has been proposed that its detection in plasma might be a biomarker, to distinguish lithium responding and non-responding patients (36).
In contrast with the advances obtained in the pathogenesis and conventional pharmacological treatment of the disorder via stem-cell technology, clinical trials for cell therapy in BD are currently in their early stages (37). Several research centers have registered clinical trials using stem cells in combination with conventional mood stabilizers; however, to our knowledge, these results have not yet been published.
MDD is a multifactorial psychiatric disorder characterized by persistent sadness, low self-esteem, and a loss of interest in for environmental stimuli, accompanied by various cognitive and physical symptoms. MDD has the highest prevalence in Western countries and is responsible for social disabilities and suicidal behaviors (38). The pathogenesis of depression involves an intricate combination of genetic, environmental, and neurobiological factors. Genetic predisposition plays a significant role, with multiple genes contributing to vulnerability to depression, particularly when combined with environmental triggers, such as chronic stress or trauma (39–42).
Neurobiologically, MDD is associated with the dysregulation of key neurotransmitter systems, particularly serotonin, norepinephrine, and dopamine, which are crucial for mood regulation (43). Recently, the glutamatergic system has been implicated (44). Additional pathogenic alterations include dysregulation of the hypothalamic-pituitary-adrenal axis, alterations in the gut microbiota (45, 46), microglia and astrocyte modifications in response to inflammatory stimuli (47, 48), and neuroplasticity deficits (49). Collectively, these factors contribute to the onset, progression, and recurrence of depressive episodes.
Selective serotonin reuptake inhibitors are the first-line treatment for MDD. However, at least 30% of the patients are resistant to treatment (treatment-resistant depression; TRD) owing to unknown neurobiological mechanisms. Considering the severity of the disease, this limitation makes it necessary to develop effective treatments for TRD. Combining distinct therapies and the development of novel treatments such as ketamine, psychodynamic drugs, or transcranial magnetic stimulation have provided hopeful results (50, 51).
Stem cell therapy might address neurobiological deficits in TRD (52); however, most data are largely derived from preclinical animal studies (53). Treatment with umbilical cord stem cells (54) or adipose-derived mesenchymal stem cells (55) has revealed positive results in mouse models of depression-like behavior. Studies devoted to improving cardiac ischemia using umbilical cord stem cells have shown that this treatment ameliorated depression-like behavior caused by ischemia (56). In these studies, the effects of cell therapy were associated with the immunomodulatory and anti-inflammatory properties of stem cells (57). A complementary beneficial effect proposed for stem cell therapy is the stimulation of endogenous neurogenesis and neuroplasticity (58–60) or the protection of neurons from induced apoptosis (61). Implantation of encapsulated mesenchymal stem cells into the lateral ventricle of rats ameliorated depression-like behavior, promoting neurogenesis in the subventricular zone and dentate gyrus of the hippocampus (60). Remarkably, the trophic influence of stem cells may be substituted with exosomes obtained from cultured bone marrow mesenchymal stem cells (61). In humans, a preliminary pilot study in 16 female TRD patients subjected to 4 intravenous injections of umbilical cord stem cells (250 x106 cells and 1-week intervals) showed an ameliorative effect on cognitive impairment, and helped overcome resistance to conventional treatment (62). Together, those findings suggest that new treatments combining stem cells and drugs with active neuroplastic activity, such as ketamine (63), may provide more efficient therapies for TRD (50). However, the use of stem cell therapy in humans still requires further animal testing (64). It must be evaluated if cell infusion is enough to induce beneficial effects, or if treatments require neural engraftment. It is also required to know if anti-depressant drugs modify the therapeutic efficiency of stem cells, or the importance of the stage of differentiation of the stem cells. At the present, I have identified three active clinical assays registered in the platform Clintrial.gov designed to evaluating the safety, efficacy and tolerability of stem cells and exosomes for the treatment of depression (NCT02675556: Phase I trial that investigates the administration of allogeneic MSCs in TDR patients that lack posted results; NCT03522545: Phase I trial, at recruiting stage, that evaluates the safety and efficacy of allogeneic bone marrow-derived MSCs in BD patients; NCT03265808: Phase I/II trial, lacking posted results, that investigates the administration of allogeneic MSCs in patients with alcohol use disorder and major depression).
SCZ is a severe and prevalent chronic behavioral and cognitive disorder characterized by broad and heterogeneous clinical symptoms, including hallucinations, delusions, cognitive impairment, and social withdrawal. Its pathophysiology is complex and involves critical interactions between genetic and environmental factors (65). SCZ is highly heritable (66), and dozens of genomic loci have been associated with this disorder (67). Current treatment options include psychosocial interventions and antipsychotic drugs that often cause undesired side effects; most importantly, these treatments remain largely ineffective in almost one-third of patients (68).
The characterization of pathogenic alterations in neural progenitors from SCZ patients is currently an intense area of research (19, 69–71). Organoid, and other in vitro technologies, have provided substantial advances in our knowledge of the molecular basis of SCZ (72). According to these studies, a core physio-pathogenic feature of SCZ is an unbalanced specification of excitatory and inhibitory neurons together with mitochondrial alterations that increase oxidative stress (73). Dysregulation of neuronal differentiation may be secondary to deficiencies in Wnt signaling (72), which, as mentioned above, is also a feature shared by BD (34). Remarkably, it has been detected a dysregulation of circulating stem cells displaying neural lineage markers in SCZ patients experiencing their first psychotic episode that might serve as a biological marker of SCZ (74).
Currently, monoamine-based antipsychotic drugs are the conventional pharmacological treatments for SCZ; however, they have several adverse effects and limited effectiveness on negative and cognitive symptoms (75). Hence, developing innovative therapeutic approaches in modern psychiatry is challenging. Advances in regenerative medicine have promoted growing interest in stem cells as a potential novel treatment for SCZ (76). Preclinical studies have demonstrated the beneficial effects of a single intravascular infusion of human umbilical cord stem cells on SCZ-related behaviors induced by amphetamine administration in mice (77). Considering that the blood–brain barrier prevents cells from reaching the neuronal centers and the regulation of IL-10, this effect was explained by the immunomodulatory influence of stem cells. This interpretation is supported by recent experiments demonstrating the alleviation of neuroinflammation and synaptic damage repair by regulating the activity of microglia in a maternal immune activation rodent model (78). Moreover, stem cells may also confer beneficial effects via local neuroprotective mechanisms, as shown in both intracranial transplantation of mesenchymal stem cells (MSCs) and intranasal delivery of MSC-derived extracellular vesicles, which alleviated behavioral and biochemical deficits in mouse models of drug-induced SCZ (79, 80). Human studies are still limited to a pilot study, without control patients, designed to assess the safety of the procedure and to analyze cortical activity in a cohort of 15 patients with SCZ (F20.6 in the International Classification of Diseases-10) who received 4 intravenous injections of umbilical cord blood cells (250 × 106 at 2-week intervals). Of note, three months after the last injection, fMRI analysis showed increased cerebral cortical activity in both the anterior and posterior components of the verbal working memory loop, which was interpreted as due to induced neuronal plasticity (76).
ASD is a heterogeneous neurodevelopmental condition that manifests in early childhood and persists throughout life. Symptoms of ASD include social and communication impairments along with repetitive, stereotyped behaviors that are frequently associated with other neuropsychiatric and non-psychiatric diseases (81). Genetic and environmental factors are implicated in the development of ASD. Genetic risk factors are complex and involve variants ranging from point mutations to large copy number variants that are either inherited or spontaneous (82). Dysregulation of the gut microbiota has been proposed as an environmental factor in the disease (83). Causal heterogeneity, together with variability in clinical phenotypes, prevents the establishment of a precise basis for the disorder (84), but its pathogenesis includes immune dysregulation, mitochondrial dysfunction, and increased oxidative stress (85).
Despite intense efforts devoted to basic and clinical research (86–90), the design of effective pharmacological treatments for the core symptoms of ASD remains elusive (91). Considering that neuroinflammation, neuronal cell damage, and oxidative stress area central events in ASD, cell therapies using stem cells of different origins have been explored. Many preclinical studies in animal models (92, 93) and human clinical trials (94, 95) have been conducted over the last decade. Except for the absence of therapeutic efficacy reported by Dawson et al. (96) that might be explained by the therapeutic protocol employed in the trial (97), the administration of umbilical cord blood cells (98–102), or autologous bone marrow stem cells (94, 103), using distinct protocols (intrathecal/intravenous) improved autism symptoms without major adverse events. In some studies, clinical improvement was considered low (104); however, in other trials, the treatment modified the spectral characteristics of the electroencephalogram (99) and improved the structural brain connectivity detectable by white matter tractography (101, 105).
Overall, the positive findings described above are largely explained by the immunoregulatory properties of stem cells (101, 105); however, whether this is the only mode of action has been questioned (85).
The stem cell technology, via in vitro modeling, is an active area of research that, in spite of some limitations (16), is providing great advances in the understanding the pathophysiology of neuropsychiatric disorders (14). Here, I have summarized the efforts of researchers to add mental disorders to the list of pathologies that could benefit from stem cell therapy. In theory, stem cell therapy may benefit psychiatric disorders by two different mechanisms: firstly, being integrated into the target neural regions to substitute deficient neurons; and, secondly, employing the cells as source of factors that ameliorate the structural deficits observed in the mental disorders (Figure 1). The first approach, requires the grafting of progenitors into the target neural tissues. This approach is still at initial preclinical stage. The infusion of stem cells with therapeutic purpose is at a more advanced stage of research. A number of published pilot studies evaluated the effectiveness of stem cell therapy to ameliorate symptomatology in MDD, SCZ, and ASD, and all of them concluded that, as observed in other pathologies, the methodology is safe, and devoid of serious adverse events. A critical aspect for the use of stem cell treatments in psychiatry is the importance of providing adequate information on risks/benefits to obtain adequate consent from the patient or their legal guardians, especially, in patients who lack decision-making capacity.
Based on the reported observations, most studies conclude that the effects of stem cell therapy have to do with immune regulation, protecting astroglia and microglia from neuroinflammatory damage, which in turn improves neuronal functions. Additionally, there is a yet uncharacterized trophic effect that promotes neuronal plasticity. Importantly, some reports suggest that cell therapy could be replaced with exosomes derived from stem cells (9, 106). To date, we do not have viable protocols as to determining the most appropriate cell type to use or the method of administration but the data reviewed here are encouraging and suggest that the regenerative treatments alone or in cooperation with other therapeutic approaches could offer solutions in the coming decades to resolve neuropsychiatric pathologies for which we currently have no effective therapies.
RV: Writing – original draft, Writing – review & editing.
The author(s) declare that no financial support was received for the research, authorship, and/or publication of this article.
The author declares that the research was conducted in the absence of any commercial or financial relationships that could be construed as a potential conflict of interest.
All claims expressed in this article are solely those of the authors and do not necessarily represent those of their affiliated organizations, or those of the publisher, the editors and the reviewers. Any product that may be evaluated in this article, or claim that may be made by its manufacturer, is not guaranteed or endorsed by the publisher.
1. Takahashi K, Yamanaka S. Induction of pluripotent stem cells from mouse embryonic and adult fibroblast cultures by defined factors. Cell. (2006) 126:663–76. doi: 10.1016/j.cell.2006.07.024
2. Mei R, Wan Z, Yang C, Shen X, Wang R, Zhang H, et al. Advances and clinical challenges of mesenchymal stem cell therapy. Front Immunol. (2024) 15:1421854. doi: 10.3389/fimmu.2024.1421854
3. Ulfig A, Jakob U. Redox heterogeneity in mouse embryonic stem cells individualizes cell fate decisions. Dev Cell. (2024) 30:S1534–5807(24)00445-3. doi: 10.1016/j.devcel.2024.07.008
4. Farahzadi R, Valipour B, Montazersaheb S, Fathi E. Targeting the stem cell niche micro-environment as therapeutic strategies in aging. Front Cell Dev Biol. (2023) 11:1162136. doi: 10.3389/fcell.2023.1162136
5. Liesveld JL, Sharma N, Aljitawi OS. Stem cell homing: From physiology to therapeutics. Stem Cells. (2020) 38:1241–53. doi: 10.1002/stem.3242
6. Takahashi K, Tanabe K, Ohnuki M, Narita M, Ichisaka T, Tomoda K, et al. Induction of pluripotent stem cells from adult human fibroblasts by defined factors. Cell. (2007) 131:861–72. doi: 10.1016/j.cell.2007.11.019
7. Vieira R, Mariani JN, Huynh NPT, Stephensen HJT, Solly R, Tate A, et al. Young glial progenitor cells competitively replace aged and diseased human glia in the adult chimeric mouse brain. Nat Biotechnol. (2024) 42:719–30. doi: 10.1038/s41587-023-01798-5
8. Zhang Y, Zhao Y, Song X, Luo H, Sun J, Han C, et al. Modulation of stem cells as therapeutics for severe mental disorders and cognitive impairments. Front Psychiatry. (2020) 11:80. doi: 10.3389/fpsyt.2020.00080
9. Darwish M, El Hajj R, Khayat L, Alaaeddine N. Stem cell secretions as a potential therapeutic agent for autism spectrum disorder: A narrative review. Stem Cell Rev Rep. (2024) 20:1252–72. doi: 10.1007/s12015-024-10724-4
10. Panda B, Sharma Y, Gupta S, Mohanty S. Mesenchymal stem cell-derived exosomes as an emerging paradigm for regenerative therapy and nano-medicine: A comprehensive review. Life (Basel). (2021) 11:784. doi: 10.3390/life11080784
11. Zhao W, Li K, Li L, Wang R, Lei Y, Yang H, et al. Mesenchymal stem cell-derived exosomes as drug delivery vehicles in disease therapy. Int J Mol Sci. (2024) 25:7715. doi: 10.3390/ijms25147715
12. Torre-Pérez N, Montero JA, Zuzarte-Luis V, García-Porrero JA, Rubio N, Blanco J, et al. Migration and differentiation of human umbilical cord stem cells after heart injury in chicken embryos. Stem Cells Dev. (2009) 18:27–36. doi: 10.1089/scd.2007.0239
13. Villar-Gómez N, Ojeda-Hernandez DD, López-Muguruza E, García-Flores S, Bonel-García N, Benito-Martín MS, et al. Nose-to-brain: the next step for stem cell and biomaterial therapy in neurological disorders. Cells. (2022) 11:3095. doi: 10.3390/cells11193095
14. Villanueva R. Advances in the knowledge and therapeutics of schizophrenia, major depression disorder, and bipolar disorder from human brain organoid research. Front Psychiatry. (2023) 14:1178494. doi: 10.3389/fpsyt.2023.1178494
15. Perrottelli A, Marzocchi FF, Caporusso E, Giordano GM, Giuliani L, Melillo A, et al. Advances in the understanding of the pathophysiology of schizophrenia and bipolar disorder through induced pluripotent stem cell models. J Psychiatry Neurosci. (2024) 49:E109–25. doi: 10.1503/jpn.230112
16. Bellon A. Comparing stem cells, transdifferentiation and brain organoids as tools for psychiatric research. Trans Psychiatry. (2024) 14:127. doi: 10.1038/s41398-024-02780-8
17. Flores-Ramos M, Ramírez-Rodríguez GB, Guiza-Zayas R, Solares-Bravo M, Rodríguez-Bores L. Relationship between inflammatory markers in human olfactory neural progenitor cells and antidepressant response. J Psychiatr Res. (2024) 171:277–85. doi: 10.1016/j.jpsychires.2024.01.047
18. Sánchez-Florentino ZA, Romero-Martínez BS, Flores-Soto E, Serrano H, Montaño LM, Valdés-Tovar M, et al. Potential of olfactory neuroepithelial cells as a model to study schizophrenia: A focus on GPCRs (Review). Int J Mol Med. (2024) 53:7. doi: 10.3892/ijmm.2023.5331
19. Idotta C, Pagano MA, Tibaldi E, Cadamuro M, Saetti R, Silvestrini M, et al. Neural stem/progenitor cells from olfactory neuroepithelium collected by nasal brushing as a cell model reflecting molecular and cellular dysfunctions in schizophrenia. World J Biol Psychiatry. (2024) 25:317–29. doi: 10.1080/15622975.2024.2357096
20. Pang ZP, Yang N, Vierbuchen T, Ostermeier A, Fuentes DR, Yang TQ, et al. Induction of human neuronal cells by defined transcription factors. Nature. (2011) 476:220–3. doi: 10.1038/nature10202
21. Bellon A, Wegener A, Lescallette AR, Valente M, Yang SK, Gardette R, et al. Transdifferentiation of human circulating monocytes into neuronal-like cells in 20 days and without reprograming. Front Mol Neurosci. (2018) 11:323. doi: 10.3389/fnmol.2018.00323
22. GBD 2021 Diseases and Injuries Collaborators. Global incidence, prevalence, years lived with disability (YLDs), disability-adjusted life-years (DALYs), and healthy life expectancy (HALE) for 371 diseases and injuries in 204 countries and territories and 811 subnational locations, 1990-2021: a systematic analysis for the Global Burden of Disease Study 2021. Lancet. (2024) 403:2133–61. doi: 10.1016/S0140-6736(24)00757-8
23. Narzisi A, Halladay A, Masi G, Novarino G, Lord C. Tempering expectations: considerations on the current state of stem cells therapy for autism treatment. Front Psychiatry. (2023) 14:1287879. doi: 10.3389/fpsyt.2023.1287879
24. Mullins N, Forstner AJ, O’Connell KS, Coombes B, Coleman JRI, Qiao Z, et al. Genome-wide association study of more than 40,000 bipolar disorder cases provides new insights into the underlying biology. Nat Genet. (2021) 53:817–29. doi: 10.1038/s41588-021-00857-4
25. Osete JR, Akkouh IA, Ievglevskyi O, Vandenberghe M, de Assis DR, Ueland T, et al. Transcriptional and functional effects of lithium in bipolar disorder iPSC-derived cortical spheroids. Mol Psychiatry. (2023) 28:3033–43. doi: 10.1038/s41380-023-01944-0
26. Hudock A, Leal ZP, Sharma A, Mei A, Santos R, Marchetto MC. Exploring mood disorders and treatment options using human stem cells. Genet Mol Biol. (2024) 47:e20230305. doi: 10.1590/1678-4685-GMB-2023-0305
27. Pachinsion DM. Progress and challenges in using human stem cells for biological and therapeutics discovery: neuropsychiatric disorders. Stem Cells. (2016) 34:523–36. doi: 10.1002/stem.2295
28. Stern S, Santos R, Marchetto MC, Mendes APD, Rouleau GA, Biesmans S, et al. Neurons derived from patients with bipolar disorder divide into intrinsically different sub-populations of neurons, predicting the patients’ responsiveness to lithium. Mol Psychiatry. (2018) 23:1453–65. doi: 10.1038/mp.2016.260
29. Mishra HK, Wei H, LeRoux M, Ko I, Rohr KE, Nievergelt CM, et al. Differential contributions of circadian clock genes to cell survival in bipolar disorder patient derived neuronal progenitor cells distinguishes lithium responders and non-responders. Res Sq. (2024), rs.3.rs–4331810. doi: 10.21203/rs.3.rs-4331810/v1
30. Osete JR, Akkouh IA, de Assis DR, Szabo A, Frei E, Hughes T, et al. Lithium increases mitochondrial respiration in iPSC-derived neural precursor cells from lithium responders. Mol Psychiatry. (2021) 26:6789–805. doi: 10.1038/s41380-021-01164-4
31. Vadodaria KC, Mendes APD, Mei A, Racha V, Erikson G, Shokhirev MN, et al. Altered neuronal support and inflammatory response in bipolar disorder patient-derived astrocytes. Stem Cell Rep. (2021) 16:825–35. doi: 10.1016/j.stemcr.2021.02.004
32. Wu X, Jiang J, Gu Z, Zhang J, Chen Y, Liu X. Mesenchymal stromal cell therapies: immunomodulatory properties and clinical progress. Stem Cell Res Ther. (2020) 11:345. doi: 10.1186/s13287-020-01855-9
33. Madison JM, Zhou F, Nigam A, Hussain A, Barker DD, Nehme R, et al. Characterization of bipolar disorder patient-specific induced pluripotent stem cells from a family reveals neurodevelopmental and mRNA expression abnormalities. Mol Psychiatry. (2015) 20:703–17. doi: 10.1038/mp.2015.7
34. Santos R, Linker SB, Stern S, Mendes APD, Shokhirev MN, Erikson G, et al. Deficient LEF1 expression is associated with lithium resistance and hyperexcitability in neurons derived from bipolar disorder patients. Mol Psychiatry. (2021) 26:2440–56. doi: 10.1038/s41380-020-00981-3
35. Zhao WN, Cheng C, Theriault KM, Sheridan SD, Tsai LH, Haggarty SJ. A high-throughput screen for Wnt/β-catenin signaling pathway modulators in human iPSC-derived neural progenitors. J Biomol Screen. (2012) 17:1252–63. doi: 10.1177/1087057112456876
36. Coradduzza D, Garroni G, Congiargiu A, Balzano F, Cruciani S, Sedda S, et al. MicroRNAs, stem cells in bipolar disorder, and lithium therapeutic approach. Int J Mol Sci. (2022) 23:10489. doi: 10.3390/ijms231810489
37. Soares JC. Stem Cell Clinical Trial for Treatment-Resistant Bipolar Depression (2024). Available online at: https://med.uth.edu/psychiatry/stem-cell-clinical-trial-for-treatment-resistant-bipolar-depression (Accessed December 19, 2024).
38. Friedrich MJ. Depression is the leading cause of disability around the world. JAMA. (2017) 317:1517. doi: 10.1001/jama.2017.3826
39. Flint J, Kendler KS. The genetics of major depression. Neuron. (2014) 81:1214. doi: 10.1016/j.neuron.2014.02.033
40. Peterson RE, Cai N, Dahl AW, Bigdeli TB, Edwards AC, Webb BT, et al. Molecular genetic analysis subdivided by adversity exposure suggests etiologic heterogeneity in major depression. Am J Psychiatry. (2018) 175:545–54. doi: 10.1176/appi.ajp.2017.17060621
41. Zhu J, Chen X, Lu B, Li XY, Wang ZH, Cao LP, et al. Transcriptomic decoding of regional cortical vulnerability to major depressive disorder. Commun Biol. (2024) 7:960. doi: 10.1038/s42003-02
42. Wang R, Su Y, O’Donnell K, Caron J, Meaney M, Meng X, et al. Differential interactions between gene expressions and stressors across the lifespan in major depressive disorder. J Affect Disord. (2024) 362:688–97. doi: 10.1016/j.jad.2024.07.069
43. Nestler EJ, Carlezon WA. The mesolimbic dopamine reward circuit in depression. Biol Psychiatry. (2006) 59:1151–9. doi: 10.1016/j.biopsych.2005.09.018
44. Baek JH, Park H, Kang H, Kim R, Kang JS, Kim HJ. The role of glutamine homeostasis in emotional and cognitive functions. Int J Mol Sci. (2024) 25:1302. doi: 10.3390/ijms25021302
45. McGuinness AJ, Davis JA, Dawson SL, Loughman A, Collier F, O’Hely M, et al. A systematic review of gut microbiota composition in observational studies of major depressive disorder, bipolar disorder and schizophrenia. Mol Psychiatry. (2022) 27:1920–35. doi: 10.1038/s41380-022-01456-3
46. Qu S, Yu Z, Zhou Y, Wang S, Jia M, Chen T, et al. Gut microbiota modulates neurotransmitter and gut-brain signaling. Microbiol Res. (2024) 287:127858. doi: 10.1016/j.micres.2024.127858
47. Byrne ML, Whittle S, Allen NB. The role of brain structure and function in the association between inflammation and depressive symptoms: A systematic review. Psychosom Med. (2016) 78:389–400. doi: 10.1097/PSY.0000000000000311
48. Li B, Yang W, Ge T, Wang Y, Cui R. Stress induced microglial activation contributes to depression. Pharmacol Res. (2022) 179:106145. doi: 10.1016/j.phrs.2022.106145
49. Serafini G. Neuroplasticity and major depression, the role of modern antidepressant drugs. World J Psychiatry. (2012) 2:49–57. doi: 10.5498/wjp.v2.i3.49
50. Sachdeva P, Ji S, Ghosh S, Ghosh S, Raghunath M, Kim H, et al. Plausible role of stem cell types for treating and understanding the pathophysiology of depression. Pharmaceutics. (2023) 15:814. doi: 10.3390/pharmaceutics15030814
51. Arubuolawe OO, Folorunsho IL, Busari AK, Ibeneme C, Diala AB, Afolabi VI, et al. Combination of transcranial magnetic stimulation and ketamine in treatment-resistant depression: A systematic review. Cureus. (2024) 16:e64712. doi: 10.7759/cureus.64712
52. Chang Z, Wang QY, Li LH, Jiang B, Zhou XM, Zhu H, et al. Potential plausible role of stem cell for treating depressive disorder: a retrospective review. Mol Neurobiol. (2024) 61:4454–72. doi: 10.1007/s12035-023-03843-5
53. do Prado-Lima PAS, Costa-Ferro ZSM, Souza BSF, da Cruz IBM, Lab B. Is there a place for cellular therapy in depression? World J Psychiatry. (2021) 11:553–67. doi: 10.5498/wjp.v11.i9.553
54. Li J, Wang H, Du C, Jin X, Geng Y, Han B, et al. hUC-MSCs ameliorated CUMS-induced depression by modulating complement C3 signaling-mediated microglial polarization during astrocyte-microglia crosstalk. Brain Res Bull. (2020) 163:109–19. doi: 10.1016/j.brainresbull.2020.07.004
55. Huang X, Fei GQ, Liu WJ, Ding J, Wang Y, Wang H, et al. Adipose-derived mesenchymal stem cells protect against CMS-induced depression-like behaviors in mice via regulating the Nrf2/HO-1 and TLR4/NF-kappaB signaling pathways. Acta Pharmacol Sin. (2020) 41:612–9. doi: 10.1038/s41401-019-0317-6
56. Zhang Y, Wang X, Li Y, Liu R, Pan J, Tang X, et al. Human umbilical cord mesenchymal stem cells ameliorate depression by regulating Jmjd3 and microglia polarization in myocardial infarction mice. Psychopharmacol (Berl). (2021) 238:2973–84. doi: 10.1007/s00213-021-05912-w
57. Baraniak PR, McDevitt TC. Stem cell paracrine actions and tissue regeneration. Regener Med. (2010) 5:121–43. doi: 10.2217/rme.09.74
58. Coquery N, Blesch A, Stroh A, Fernández-Klett F, Klein J, Winter C, et al. Intrahippocampal transplantation of mesenchymal stromal cells promotes neuroplasticity. Cytotherapy. (2012) 14:1041–53. doi: 10.3109/14653249.2012.694418
59. Darkazalli A, Ismail AA, Abad N, Grant SC, Levenson CW. Use of human mesenchymal stem cell treatment to prevent anhedonia in a rat model of traumatic brain injury. Restor Neurol Neurosci. (2016) 34:433–41. doi: 10.3233/RNN-150628
60. Kin K, Yasuhara T, Kameda M, Tomita Y, Umakoshi M, Kuwahara K, et al. Cell encapsulation enhances antidepressant effect of the mesenchymal stem cells and counteracts depressive-like behavior of treatment-resistant depressed rats. Mol Psychiatry. (2020) 25:1202–14. doi: 10.1038/s41380-018-0208-0
61. Guo H, Huang B, Wang Y, Zhang Y, Ma Q, Ren Y. Bone marrow mesenchymal stem cells-derived exosomes improve injury of hippocampal neurons in rats with depression by upregulating microRNA-26a expression. Int Immunopharmacol. (2020) 82:106285. doi: 10.1016/j.intimp.2020.106285
62. Smulevich AB, Dubnitskaya EB, Voronova EI, Morozova YV, Radaev SM. Efficiency of umbilical cord blood cells in patients with treatment-resistant depressions. Bull Exp Biol Med. (2016) 160:583–8. doi: 10.1007/s10517-016-3222-8
63. Collo G, Merlo-Pich E. Ketamine enhances structural plasticity in human dopaminergic neurons: Possible relevance for treatment-resistant depression. Neural Regener Res. (2018) 13:645–646. doi: 10.4103/1673-5374.230288
64. Vaz A, Salgado A, Patrício P, Pinto L. Patient-derived induced pluripotent stem cells: Tools to advance the understanding and drug discovery in Major Depressive Disorder. Psychiatry Res. (2024) 339:116033. doi: 10.1016/j.psychres.2024.116033
65. Robinson N, Bergen SE. Environmental risk factors for schizophrenia and bipolar disorder and their relationship to genetic risk: current knowledge and future directions. Front Genet. (2021) 12:686666. doi: 10.3389/fgene.2021.686666
66. Sullivan PF, Kendler KS, Neale MC. Schizophrenia as a complex trait: evidence from a meta-analysis of twin studies. Arch Gen Psychiatry. (2003) 60:1187–92. doi: 10.1001/archpsyc.60.12.1187
67. Golov AK, Kondratyev NV, Kostyuk GP, Golimbet AVE. Novel approaches for identifying the molecular background of schizophrenia. Cells. (2020) 9:246. doi: 10.3390/cells9010246
68. Elkis H, Buckley PF. Treatment-resistant schizophrenia. Psychiatr Clin North Am. (2016) 39:239–65. doi: 10.1016/j.psc.2016.01.006
69. Sánchez-Florentino ZA, Romero-Martínez BS, Flores-Soto E, Montaño LM, Sommer B, Valdés-Tovar M, et al. Altered PLCβ/IP3/ca2+ Signaling pathway activated by GPRCs in olfactory neuronal precursor cells derived from patients diagnosed with schizophrenia. Biomedicines. (2024) 12:2343. doi: 10.3390/biomedicines12102343
70. Stahl A, Heider J, Wüst R, Fallgatter AJ, Schenke-Layland K, Volkmer H, et al. Patient iPSC-derived neural progenitor cells display aberrant cell cycle control, p53, and DNA damage response protein expression in schizophrenia. BMC Psychiatry. (2024) 24:757. doi: 10.1186/s12888-024-06127-x
71. Xi S, Xue B, Wang Y, Shan Z, Song T, Sun J, et al. Generation of patient-derived induced pluripotent stem cell line UJSi004-A from ultra-treatment-resistant schizophrenia. Stem Cell Res. (2024) 81:103575. doi: 10.1016/j.scr.2024.103575
72. Sawada T, Chater TE, Sasagawa Y, Yoshimura M, Fujimori-Tonou N, Tanaka K, et al. Developmental excitation-inhibition imbalance underlying psychoses revealed by single-cell analyses of discordant twins-derived cerebral organoids. Mol Psychiatry. (2020) 25:2695–711. doi: 10.1038/s41380-020-0844-z
73. Robicsek O, Karry R, Petit I, Salman-Kesner N, Müller FJ, Klein E, et al. Abnormal neuronal differentiation and mitochondrial dysfunction in hair follicle-derived induced pluripotent stem cells of schizophrenia patients. Mol Psychiatry. (2013) 18:1067–76. doi: 10.1038/mp.2013.67
74. Kucharska-Mazur J, Tarnowski M, Dołęgowska B, Budkowska M, Pędziwiatr D, Jabłoński M, et al. Novel evidence for enhanced stem cell traficking in antipsychotic-naïve subjects during their first psychotic episode. J Psychiatr Res. (2014) 49:18–24. doi: 10.1016/j.jpsychires.2013.10.016
75. Correll CU, Rubio JM, Kane JM. What is the risk-benefit ratio of long-term antipsychotic treatment in people with schizophrenia? World Psychiatry. (2018) 17:149–60. doi: 10.1002/wps.20516
76. Ternovoy S, Ustyuzhanin D, Morozova Y, Shariya M, Roldan-Valadez E, Smirnov V. Functional MRI evince the safety and efficacy of umbilical cord blood cells therapy in patients with schizophrenia. Schizophr Res. (2020) 224:175–7. doi: 10.1016/j.schres.2020.09.028
77. You MJ, Bang M, Park HS, Yang B, Jang KB, Yoo J, et al. Human umbilical cord-derived mesenchymal stem cells alleviate schizophrenia-relevant behaviors in amphetamine-sensitized mice by inhibiting neuroinflammation. Transl Psychiatry. (2020) 10:123. doi: 10.1038/s41398-020-0802-1
78. Cui X, Chen T, Xue Y, Wu Z, Yan P, Yang Y, et al. Human umbilical cord blood mesenchymal stem cells mediate microglia activation and improve anxiety-like behavior in MIA-induced offspring of schizophrenic rats. Prog Neuropsychopharmacol Biol Psychiatry. (2024) 133:111010. doi: 10.1016/j.pnpbp.2024.111010
79. Tsivion-Visbord H, Perets N, Sofer T, Bikovski L, Goldshmit Y, Ruban A, et al. Mesenchymal stem cells derived extracellular vesicles improve behavioral and biochemical deficits in a phencyclidine model of schizophrenia. Transl Psychiatry. (2020) 10:305. doi: 10.1038/s41398-020-00988-y
80. Zhong XL, Huang Y, Du Y, He LZ, Chen YW, Cheng Y, et al. Unlocking the therapeutic potential of exosomes derived from nasal olfactory mucosal mesenchymal stem cells: restoring synaptic plasticity, neurogenesis, and neuroinflammation in schizophrenia. Schizophr Bull. (2024) 50:600–14. doi: 10.1093/schbul/sbad172
81. Lai MC, Kassee C, Besney R, Bonato S, Hull L, Mandy W, et al. Prevalence of co-occurring mental health diagnoses in the autism population: a systematic review and meta-analysis. Lancet Psychiatry. (2019) 6:819–29. doi: 10.1016/S2215-0366(19)30289-5
82. Havdahl A, Niarchou M, Starnawska A, Uddin M, van der Merwe C, Warrier V. Genetic contributions to autism spectrum disorder. Psychol Med. (2021) 51:2260–73. doi: 10.1017/S0033291721000192
83. Yu R, Hafeez R, Ibrahim M, Alonazi WB, Li B. The complex interplay between autism spectrum disorder and gut microbiota in children: A comprehensive review. Behav Brain Res. (2024) 473:115177. doi: 10.1016/j.bbr.2024.115177
84. Kereszturi É. Database-assisted screening of autism spectrum disorder related gene set. Mol Brain. (2024) 17:55. doi: 10.1186/s13041-024-01127-0
85. Gevezova M, Sbirkov Y, Sarafian V, Plaimas K, Suratanee A, Maes M. Autistic spectrum disorder (ASD)—Gene, molecular and pathway signatures linking systemic inflammation, mitochondrial dysfunction, transsynaptic signalling, and neurodevelopment. Brain Behav Immun Health. (2023) 30:100646. doi: 10.1016/j.bbih.2023.100646
86. Miao C, Shen Y, Lang Y, Li H, Gong Y, Liu Y, et al. Biomimetic nanoparticles with enhanced rapamycin delivery for autism spectrum disorder treatment via autophagy activation and oxidative stress modulation. Theranostics. (2024) 14:4375–92. doi: 10.7150/thno.95614
87. Dessus-Gilbert ML, Nourredine M, Zimmer L, Rolland B, Geoffray MM, Auffret M, et al. NMDA antagonist agents for the treatment of symptoms in autism spectrum disorder: a systematic review and meta-analysis. Front Pharmacol. (2024) 15:1395867. doi: 10.3389/fphar.2024.1395867
88. Silva FAE, Morais J P, Mira Coelho A. Evaluation of the behavioral effect of psychostimulants in children with autism spectrum disorder: A cross-sectional study. Neuropediatrics. (2024) 6. doi: 10.1055/s-0044-1788891
89. Oz M, Kury LA, Sadek B, Mahgoub MO. The role of nicotinic acetylcholine receptors in the pathophysiology and pharmacotherapy of autism spectrum disorder: Focus on alpha7 nicotinic receptors. Int J Biochem Cell Biol. (2024) 174:106634. doi: 10.1016/j.biocel.2024.106634
90. Kaye AD, Allen KE, Smith Iii VS, Tong VT, Mire VE, Nguyen H, et al. Emerging treatments and therapies for autism spectrum disorder: A narrative review. Cureus. (2024) 16:e63671. doi: 10.7759/cureus.63671
91. Yenkoyan K, Ounanian Z, Mirumyan M, Hayrapetyan L, Zakaryan N, Sahakyan R, et al. Advances in the treatment of autism spectrum disorder: current and promising strategies. Curr Med Chem. (2024) 31:1485–511. doi: 10.2174/0109298673252910230920151332
92. Perets N, Segal-Gavish H, Gothelf Y, Barzilay R, Barhum Y, Abramov N, et al. Long term beneficial effect of neurotrophic factors-secreting mesenchymal stem cells transplantation in the BTBR mouse model of autism. Behav Brain Res. (2017) 331:254–60. doi: 10.1016/j.bbr.2017.03.047
93. Ha S, Park H, Mahmood U, Ra JC, Suh YH, Chang KA. Human adipose-derived stem cells ameliorate repetitive behavior, social deficit and anxiety in a VPA-induced autism mouse model. Behav Brain Res. (2017) 317:479–84. doi: 10.1016/j.bbr.2016.10.004
94. Sharma A, Gokulchandran N, Sane H, Nagrajan A, Paranjape A, Kulkarni P, et al. Autologous bone marrow mononuclear cell therapy for autism: an open label proof of concept study. Stem Cells Int. (2013) 2013:623875. doi: 10.1155/2013/623875
95. Price J. Cell therapy approaches to autism: a review of clinical trial data. Mol Autism. (2020) 11:37. doi: 10.1186/s13229-020-00348-z
96. Dawson G, Sun JM, Baker J, Carpenter K, Compton S, Deaver M, et al. A phase II randomized clinical trial of the safety and efficacy of intravenous umbilical cord blood infusion for treatment of children with autism spectrum disorder. J Pediatr. (2020) 222:164–173.e5. doi: 10.1016/j.jpeds.2020.03.011
97. Nguyen LT, Nguyen PH, Hoang DM. A phase II randomized clinical trial of the safety and efficacy of intravenous umbilical cord blood infusion for treatment of children with autism spectrum disorder. J Pediatr. (2020) 230:271–2. doi: 10.1016/j.jpeds.2020.11.063
98. Lv YT, Zhang Y, Liu M, Qiuwaxi JN, Ashwood P, Cho SC, et al. Transplantation of human cord blood mononuclear cells and umbilical cord-derived mesenchymal stem cells in autism. J Transl Med. (2013) 11:196. doi: 10.1186/1479-5876-11-196
99. Murias M, Major S, Compton S, Buttinger J, Sun JM, Kurtzberg J, et al. Electrophysiological biomarkers predict clinical improvement in an open-label trial assessing efficacy of autologous umbilical cord blood for treatment of autism. Stem Cells Transl Med. (2018) 7:783–91. doi: 10.1002/sctm.18-0090
100. Chez M, Lepage C, Parise C, Dang-Chu A, Hankins A, Carroll M. Safety and observations from a placebo-controlled, crossover study to assess use of autologous umbilical cord blood stem cells to improve symptoms in children with autism. Stem Cells Transl Med. (2018) 7:333–41. doi: 10.1002/sctm.17-0042
101. Carpenter KLH, Major S, Tallman C, Chen LW, Franz L, Sun J, et al. White matter tract changes associated with clinical improvement in an open-label trial assessing autologous umbilical cord blood for treatment of young children with autism. Stem Cells Transl Med. (2019) 8:138–47. doi: 10.1002/sctm.18-0251
102. Morozova YV, Smirnov VN, Makarov IV, Emelina DA. The use of umbilical cord blood nucleated cells in the treatment of regressive autism: A case report. Consort Psychiatr. (2023) 4:39–47. doi: 10.17816/CP9300
103. Kobinia GS, Zaknun JJ, Pabinger C, Laky B. Case report: autologous bone marrow derived intrathecal stem cell transplant for autistic children - A report of four cases and literature review. Front Pediatr. (2021) 9:620188. doi: 10.3389/fped.2021.620188
104. Sharifzadeh N, Ghasemi A, Tavakol Afshari J, Moharari F, Soltanifar A, Talaei A, et al. Intrathecal autologous bone marrow stem cell therapy in children with autism: A randomized controlled trial. Asia Pac Psychiatry. (2021) 13:e12445. doi: 10.1111/appy.12445
105. Simhal AK, Carpenter KLH, Kurtzberg J, Song A, Tannenbaum A, Zhang L. Changes in the geometry and robustness of diffusion tensor imaging networks: Secondary analysis from a randomized controlled trial of young autistic children receiving an umbilical cord blood infusion. Front Psychiatry. (2022) 13:1026279. doi: 10.3389/fpsyt.2022.1026279
Keywords: bipolar disorder, schizophrenia, major depression, treatment-resistant depression, autism spectrum disorder
Citation: Villanueva R (2025) Stem cell therapy for the treatment of psychiatric disorders: a real hope for the next decades. Front. Psychiatry 15:1492415. doi: 10.3389/fpsyt.2024.1492415
Received: 06 September 2024; Accepted: 16 December 2024;
Published: 07 January 2025.
Edited by:
Enrique Estudillo, Manuel Velasco Suárez National Institute of Neurology and Neurosurgery, MexicoReviewed by:
Adolfo Lopez, Hospital Juárez de México, MexicoCopyright © 2025 Villanueva. This is an open-access article distributed under the terms of the Creative Commons Attribution License (CC BY). The use, distribution or reproduction in other forums is permitted, provided the original author(s) and the copyright owner(s) are credited and that the original publication in this journal is cited, in accordance with accepted academic practice. No use, distribution or reproduction is permitted which does not comply with these terms.
*Correspondence: Rosa Villanueva, cm9zdnBAdGVsZWZvbmljYS5uZXQ=
Disclaimer: All claims expressed in this article are solely those of the authors and do not necessarily represent those of their affiliated organizations, or those of the publisher, the editors and the reviewers. Any product that may be evaluated in this article or claim that may be made by its manufacturer is not guaranteed or endorsed by the publisher.
Research integrity at Frontiers
Learn more about the work of our research integrity team to safeguard the quality of each article we publish.