- 1Department of Crop Science and Horticulture, College of Agriculture and Environmental Sciences, Makerere University, Kampala, Uganda
- 2National Livestock Resources Research Institute, National Agricultural Research Organization, Kampala, Uganda
- 3Makerere University Regional Centre for Crop Improvement (MaRCCI), College of Agriculture and Environmental Sciences, Makerere University, Kampala, Uganda
- 4Council for Scientific and Industrial Research (CSIR)– Savanna Agriculture Research Institute, Tamale, Ghana
- 5Department of Horticultural Sciences, North Carolina State University, Raleigh, NC, United States
A comprehensive understanding of the genetics of resistance is essential for developing an effective breeding strategy to create germplasm resistant to Fusarium Ear Rot. This study aimed to determine the general combining ability (GCA), specific combining ability (SCA), and heritability of resistance to infection by Fusarium verticillioides in tropical maize. Using the North Carolina II mating design, six inbred lines as females and seven as males were crossed to produce 42 hybrids, which were evaluated across five environments using artificial inoculation. At harvest, the hybrids were scored for Fusarium Ear Rot (FER) infection using a 1-9 severity scale. Significant GCA effects for the parents and SCA effects for the hybrids were observed. The narrow-sense heritability estimate was 0.22, while the broad-sense heritability was 0.73, and the additive genetic effects, as represented by GCA (m+f), were more significant than non-additive effects. The inbred parents JPS25-13, JPS26-125, JPS26-86, JPS25-11, JPS25-5, JPS25-7, and JPS25-9 were identified as the best general combiners for FER resistance. These lines, with favorable general combining ability effects for resistance to Fusarium verticillioides, are strong candidates for breeding resistant varieties.
1 Introduction
Maize is one of the most important cereal crops globally due to its high yield potential and its widespread use as food, animal feed, and for various industrial applications (Ju et al., 2017). In sub-Saharan Africa, maize serves as a critical source of food security and economic development (Cairns and Prasanna, 2018), with over 300 million Africans relying on it as their primary staple food (Badu-Apraku and Fakorede, 2017). In Uganda, maize is a staple food for many households in both rural and urban areas (Epule et al., 2021), with total production in the 2020 agricultural year estimated at approximately 3.5 million metric tonnes (MT) from around 2.0 million hectares (ha) of planted area (UBOS, 2020).
Despite its importance, maize production faces numerous biotic and abiotic challenges, including Fusarium Ear Rot (FER) caused by Fusarium verticillioides. This fungal pathogen is widespread and infects various plant species, including maize, millet, rice, wheat, and sorghum (Xu et al., 2023). In maize, it causes ear, stalk, and root rots (Logrieco et al., 2002) and can lead to yield losses of up to 50% (Ding et al., 2008; Magarini et al., 2024). FER is typically favored by warm and dry conditions, although warm and wet conditions following inoculation at silking can also promote disease development (Afolabi et al., 2007). This fungus poses a major problem not only due to the yield losses it causes but also because it produces fumonisin, a mycotoxin harmful to both humans and animals. In Uganda, studies by Atukwase et al. (2009; 2012) reported occurrence of fumonisin in major maize growing districts. A recent study by Yli-Mattila and Sundheim, (2022) reported that Uganda is among African countries with high levels of fumonisins (FUM) in maize, ranging from 270 to 10,000 µg/kg, exceeding the advisory levels of 2000 µg/kg set by the U.S. Food and Drug Administration (FDA) and regulatory limits of 2000–4000 µg/kg set by the East African Community (EAC) and European Union (EU) (Atukwase et al., 2009; Niyibituronsa et al., 2020; Yli-Mattila and Sundheim, 2022). These high levels of fumonisins identified in Uganda are worrying, therefore awareness and management of this fungus is an important step in controlling human exposure to the toxins. Common practices like the use of insecticides, fungicides, or other agronomic approaches have been reported to be environmentally unfriendly, ineffective and, and they increase the costs of maize production (Lanubile et al., 2017; Ayesiga et al., 2023).
Breeding for resistance is the most economical, effective, and environmentally friendly approach to control Fusarium Ear Rot (FER) and reduce the risk of fumonisin accumulation in maize (Netshifhefhe et al., 2018; Ouko et al., 2020). To develop cultivars resistant to F. verticillioides, it is essential to identify and utilize sources with strong resistance. However, most commercially available maize cultivars worldwide lack specific resistance to F. verticillioides (Tembo et al., 2022) and the known sources of resistance predominantly originate from temperate regions, where they are poorly adapted to the growing conditions in sub-Saharan Africa and often have limited combining ability for yield (Warburton et al., 2009; Tembo et al., 2022). Several studies have reported genetic variation for resistance to FER (Lanubile et al., 2011; Butrón et al., 2015; Stagnati et al., 2019). Efforts to understand the mechanisms and genetics of resistance to F. verticillioides infection in maize have highlighted that resistance to FER is quantitatively inherited and is largely associated with additive genetic effects, along with a significant genotype by environment component (Ding et al., 2008; Reid et al., 2009; Mesterházy et al., 2012; Balconi et al., 2014; Butrón et al., 2015; Maschietto et al., 2015; Tembo et al., 2022). Some studies have reported that resistance to Fusarium Ear Rot involves both additive and nonadditive genetic effects, highlighting the complexity of the trait and the need to consider both types of genetic contributions in breeding programs (Chen et al., 2012; Lanubile et al., 2017; de Jong et al., 2018). However, no complete resistance to FER has been identified in maize (Eller et al., 2008). Mukanga et al. (2010) found high genotype × environment interaction (G × E) for ear rot-causing fungi, implying that different genotypes respond variably to infection across different environments. Tembo et al. (2022) studied twelve inbred lines from Seed Co used as the females mated to 12 tester lines from the International Institute of Tropical Agriculture (IITA) using the North Carolina Design II. The 144 F1 hybrids and six check hybrids were evaluated in Zimbabwe under artificial inoculation with F. verticillioides. The GCA and SCA effects for F. verticillioides incidence were variable across sites for the lines and the testers. The study identified six southern African inbred lines with desirable GCA for FER and could be used as resistance sources. Both additive and nonadditive effects were implicated in resistance to FER. Rose et al. (2016) evaluated 18 inbred lines for resistance to FER in five locations in South Africa and found significant environment X genotype interactions and also reported additive gene effects were involved in resistance to FER.
Efficient breeding for resistance to FER requires a thorough understanding of its genetic basis; combining ability and heritability are essential factors when studying the genetics of crop traits (Ma Teresa et al., 1994). The objectives of this study were to evaluate the resistance of F1 hybrids to F. verticillioides and to determine the mode of gene action conditioning resistance to Fusarium Ear Rot.
2 Materials and methods
2.1 Plant materials and generation of F1 hybrids
In this study, thirteen inbred lines (Table 1) with varying levels of resistance to FER were used to investigate the genetic action behind the resistance to FER. The parents were chosen based on their contrasting responses to FER observed in preliminary screening trials, and their genetic diversity (Ayesiga et al., 2022, 2023), which aimed to maximize the potential for detecting combining ability effects. These parental lines encompass a substantial portion of the breeding material currently used in national programs, making the findings relevant to ongoing breeding programs in the region. The lines are owned and maintained by the National Agricultural Research Organization (NARO), ensuring accessibility for further research and development. All possible crosses between the seven males resistant lines and six females susceptible lines were made using North Carolina II mating design (Comstock and Robinson, 1952) under controlled pollination conditions to produce 42 hybrids. These hybrids were then evaluated in three locations in Uganda as described below.
2.2 Description of the study sites
Nakyesasa falls in the mid-altitude agro-ecological zone, located at 0° 32’N and 32° 35´E, at 1150 meters above sea level, the soil type is sandy clay loam and is classified as Orthic Ferrasol. The mean annual rainfall at Nakyesasa is 1270 mm with a bimodal distribution (March–July and September–November). Buginyanya is located at 1°12’48.0”N and 34°23’35.0”E, at 1877 meters above sea level and is characterized with well drained dark clay loam and red sand clay loam with a sticky texture and is classified as Plinthic Umbric. The region receives a bi-modal pattern of rainfall, with the wettest months being April and October and the annual average rainfall in the zone ranges from 1,200mm to 2,000mm. Bulindi is located at 0°16’N, 32052’E’, at 1144 meters above sea level, the soil is Sandy loam and is classified as Acric Ferralsol. The mean annual rainfall at Bulindi is 1338 mm, with a bimodal distribution (March–July and September–November).
2.3 Field evaluation
Forty-two single-cross hybrids were evaluated in this trial, conducted across three sites (Nakyesasa, Buginyanya, and Bulindi) over two growing seasons (A and B). Uganda has two seasons; season A which runs from February to June and season B runs from September to December. Buginyanya season A (E1) was planted on 22nd/04/2022; Nakyesasa season A (E2) was planted on 14th/04/2022; Nakyesasa season B (E3) was planted on 7th/09/2022; Buginyanya season B (E4) was planted on 30th/09/2022 and Bulindi season B (E5) was planted on 24th/09/2022. The experiment followed a 6 × 7 alpha lattice design with two replications at each location. Each hybrid was planted in two-row plots measuring 5 meters in length, with rows spaced 0.75 meters apart and 0.25 meters between plants. Two seeds were sown per hill, and after four weeks, the seedlings were thinned to one plant per hill. Standard agronomic practices, including weeding and appropriate fertilizer applications, were applied consistently across all trials. Although cycle length was not directly evaluated in this study, artificial inoculations were standardized at seven days after flowering to ensure consistency in FER resistance evaluation across hybrids following the technique outlined by Ayesiga et al. (2023), in brief, fully colonized toothpicks were used to inoculate the maize ears approximately seven days after flowering. Inoculation was conducted by piercing through the middle of the primary ear of five plants per plot. Paper bags were used to cover the ears to avoid allo-infection. At maturity, the inoculated ears from each plot were harvested, and FER symptoms were assessed based on the percentage of infected area using the following nine-point scale: 1 = 0% (no visible disease symptom), 2 = 1%, 3 = 2%–5%, 4 = 6%–10%, 5 = 11%–20%, 6 = 21%–40%, 7 = 41%–60%, 8 = 61%–80%, and 9 = 81%–100% (Supplementary Figure S1). Scores of 1–2 were classified as “good,” 3–5 as “intermediate,” and 6–9 as “poor.” (Tembo et al., 2022).
2.4 Data analysis
2.4.1 Analysis of variance
The data was subjected to Analyses of variance in R software using the agricolae package (De Mendiburu, 2021) in R software (R Core Team, 2021),. Each location-season combination was considered an environment. Environments and replications were treated as fixed effects and the other effects as random. Across location linear model:
where Yijk is the phenotypic value of the ith genotype, jth environment, kth replication, jk, the effect of kth replication in jth environment and errorijk is the residual.
Variance components were calculated using the linear mixed model:
where Yijko is the phenotypic value of the ith genotype at the jth environment in the kth replication of the oth incomplete block, gi is the effect of the ith genotype, lj, the effect of the jth environment, rkj, the effect of the kth replication at the jth environment, bojk is the effect of the oth incomplete block in the kth replication at the jth environment, and eijko is the residual.
The Tukey’s HSD post-hoc test was performed to determine significant differences among the means of genotypes across environments. This test provides pairwise comparisons while controlling the family-wise error rate. The analysis was conducted using the agricolae package (De Mendiburu, 2021) in R software (R Core Team, 2021).
2.4.2 Determination of combining ability
The General Combining Ability (GCA) effects for each parent were analyzed, as well as the Specific combining ability (SCA) effects for the hybrids were Analyzed with R for Windows (AGD-R) (Rodríguez et al., 2015) software using the Henderson method to estimate variances using the model;
where i = 1, 2, …., 6; j = 1, 2, …., 7; k = 1, 2; q = 1, 2, 3, 4, 5; and Yijkq is the value of the hybrid from mating the jth male line and the ith female line in the kth replication, in the qth environment; μ is the grand mean, gi is the GCA effect for the progeny of the ith female line, gj the general combining ability effect for the progeny of the jth male line, sij the SCA effect for hybrid got from mating the ith female line and the jth male line, yq is the average effect of the qth environment, rk(yq) is the effect of the kth replication nested within the qth environment, (gy)iq and (gy)jq are the interactions between the GCA effects and the environment, (sy)ijq is the interaction between the SCA effect and environment, and eijkq is the residual.
Broad sense (H2) and Narrow Sense (h2) heritabilities were also estimated respectively using the formulae by (Falconer and Mackay, 1996);
Where; h2 = narrow sense heritability; δ2GCA = variance of general combining ability; δ2SCA = variance of specific combining ability of parents; δ2e = error variance.
Where;
H2 = broad sense heritability;
is the genetic variance,
is the genotype-by-environment interaction variance,
is the residual error variance
is the number of environments,
is the number of replications.
The predominant gene action in the inheritance of resistance to FER was estimated using Baker’s ratio (BR) (Baker, 1978), computed as;
3 Results
3.1 Mean performance of the maize hybrids for FER across five test environments
Table 2 presents the performance of 42 different hybrids (labeled JPSH-1 to JPSH-42) across five environments (E1 to E5) and their corresponding mean values. The means ranged from 2.9 to 6.9, with an overall mean of 4.6 across the five environments. The hybrids exhibited different performances across environments, with some performing consistently well or poorly across all environments, while others show more variability. The hybrids with the most outstanding performance for FER resistance were JPSH-3, JPSH-35, JPSH-4, JPSH-1, JPSH-5, JPSH-12, JPSH-16, JPSH-23, JPSH-29, JPSH-32 and JPSH-33 with mean severity scores less than 4.0. The most susceptible hybrids with mean severity scores above 5.0 were JPSH-18, JPSH-15, JPSH-30, JPSH-36, JPSH-42, JPSH-10, JPSH-11, JPSH-13, JPSH-20, JPSH-21 and JPSH-22. The mean scores for each environment (E1 to E5) varied, suggesting that environmental conditions significantly influence hybrid performance. Based on the studied environments, the lowest FER severity score was recorded in E2 followed by E5 and the highest score was in E4. Table 2 also presents the CV which is the measure of relative variability, expressed as a percentage, a higher CV suggests more variability in the data. Environments E2 and E5 had the highest CV values (59% and 59.8%, respectively), suggesting more variability in hybrid performance in those environments compared to E4, which has a lower CV (20.4%). Differences in hybrid performance across environments may be influenced by traits such as cycle length, which was not assessed in this study. Future evaluations could explore the relationship between cycle length and resistance to Fusarium Ear Rot. Also, while yield data were not collected, visual observations suggested varying levels of plant vigor and ear development among hybrids, warranting further investigation into their yield potential.
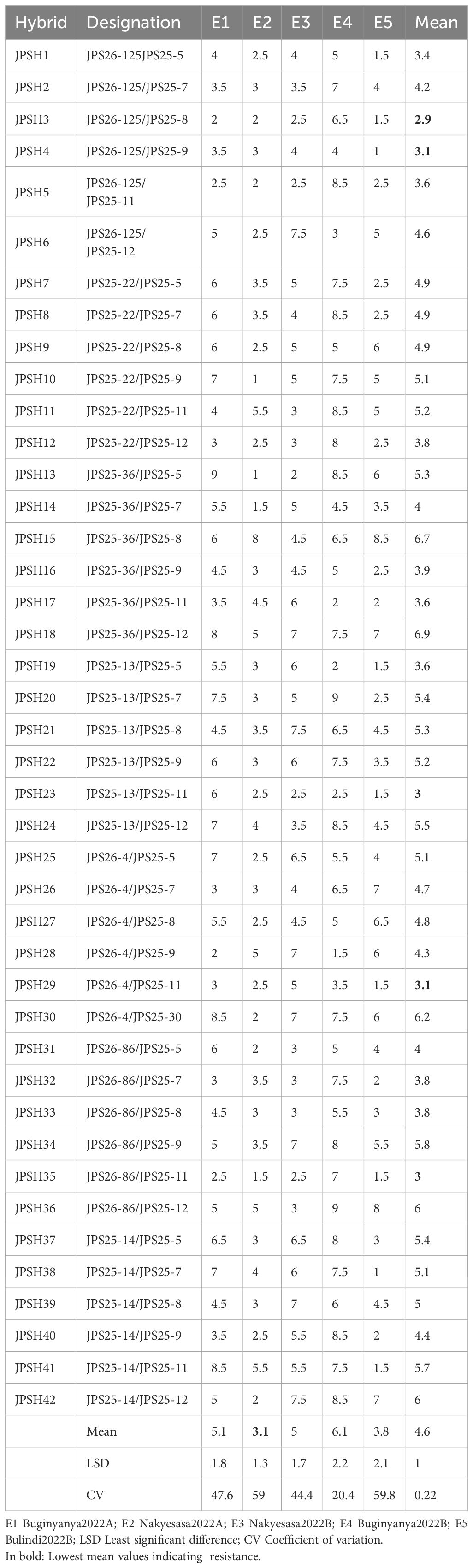
Table 2. Mean Fusarium Ear Rot severities for 42 maize hybrids assessed in five test environments within Uganda.
3.2 ANOVA of the FER severity for the hybrids across five environments
The results of Analysis of variance are shown in Table 3, and varying degrees of F. verticillioides infection were observed across test environments. The combined analysis of variance across the five environments showed that environment and genotypes were highly significant (P < 0.001) for kernel infection, while the Genotype × Environment interaction was significant at P < 0.05. The F1 hybrid mean square was partitioned into male general combining ability (GCAMALE), female general combining ability (GCAFEMALE), and specific combining ability components. The mean squares for (GCAMALE) and (GCAFEMALE) were highly significant (P < 0.001). The mean squares for GCAMALE × environment and GCAFEMALE × Environment interaction effects were highly significant (P < 0.001) and significant at P < 0.05 respectively. The ANOVA results (Table 3) indicate that the overall contributions of both male and female GCA effects are significant, reflecting the importance of additive genetic effects for resistance to Fusarium Ear Rot. However, when examining individual lines in Table 4, JPS26-125 was the only male line with a statistically significant negative GCA effect, while other male and female lines also contributed to resistance but did not reach statistical significance individually.
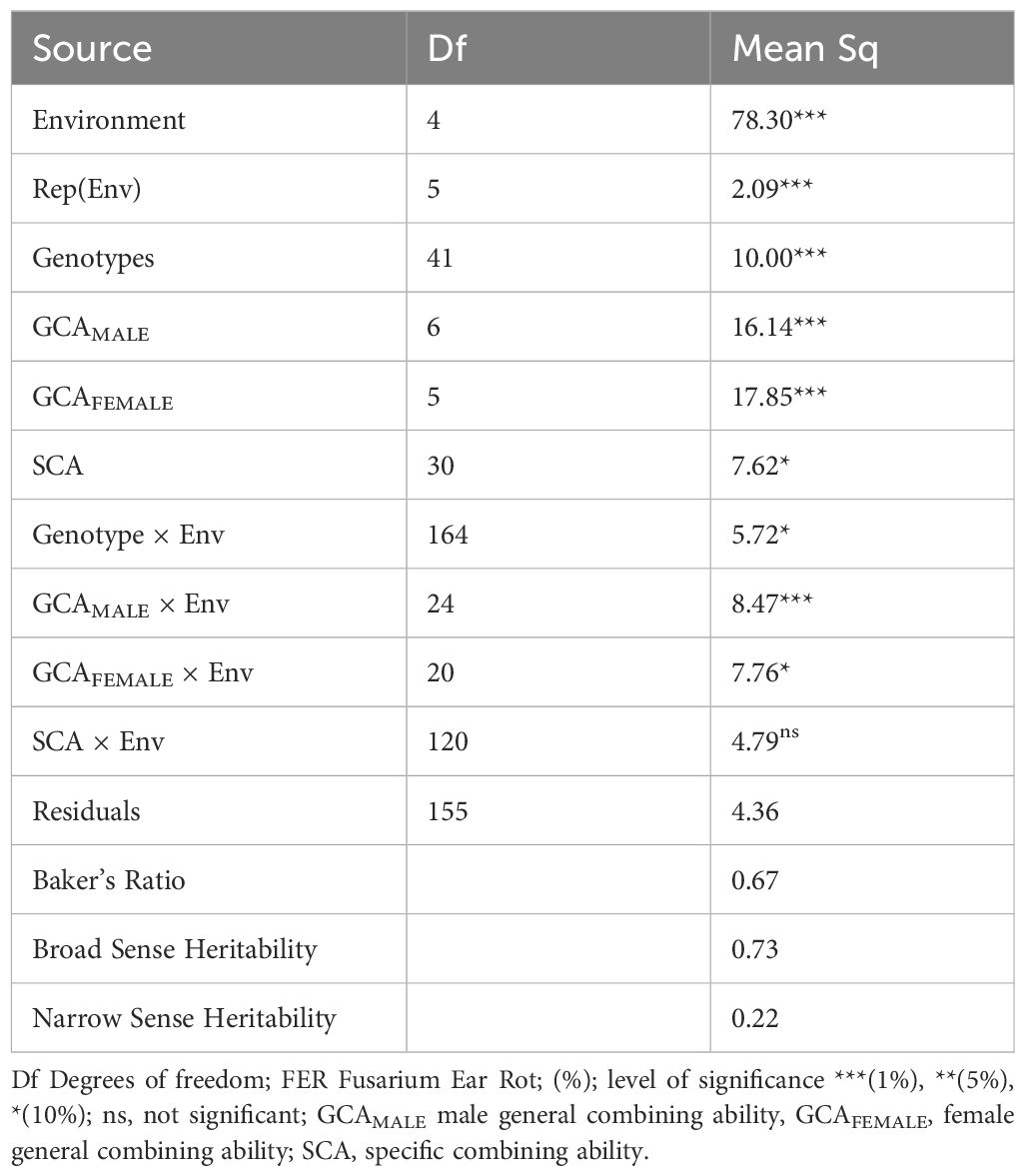
Table 3. Mean squares and degrees of freedom for the combined analysis of variance for percentage kernel infection for 42 tropical maize hybrids in 5 environments of Uganda.
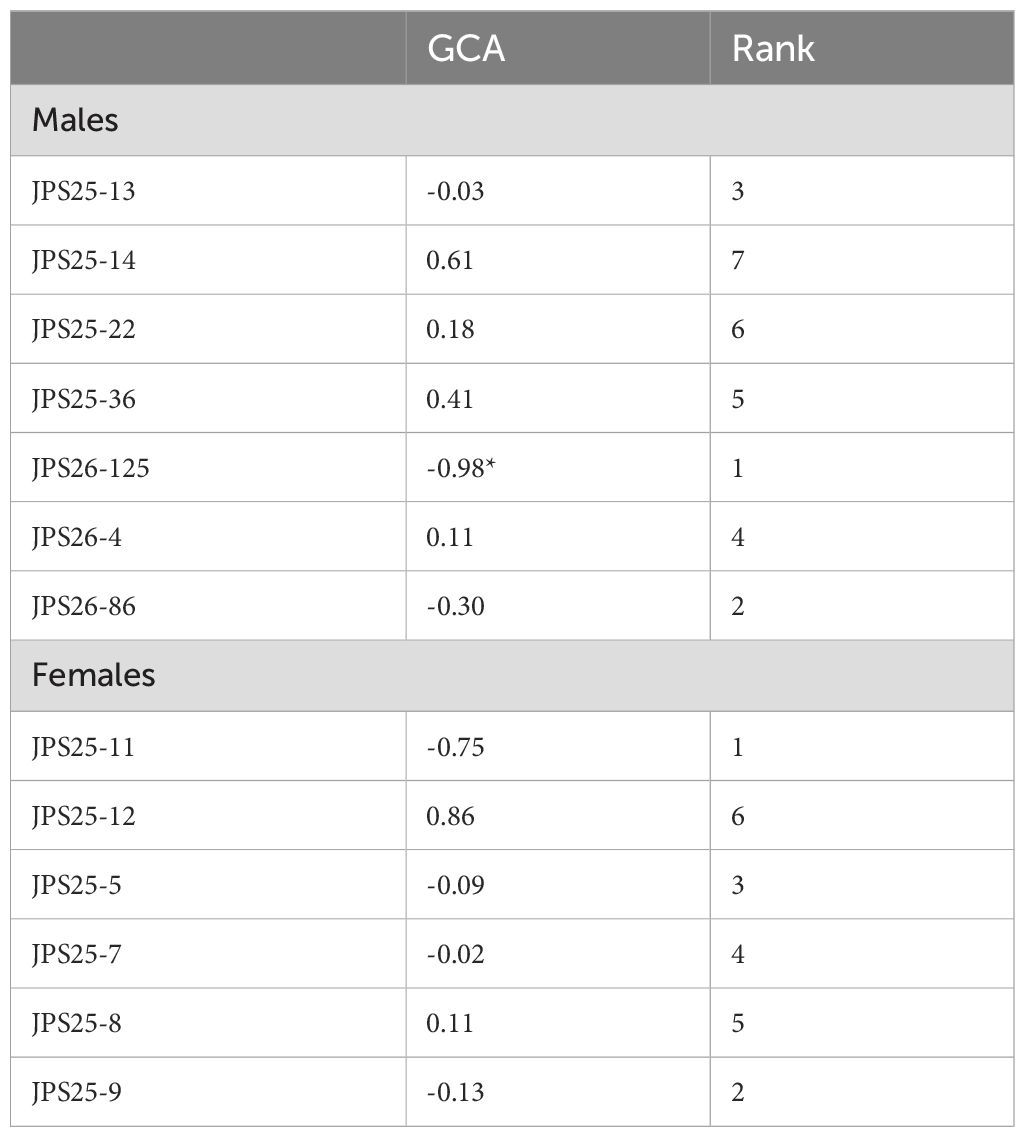
Table 4. General combining ability effects of (GCAFEMALE) and (GCAMALE) for FER infection across the five environments.
3.3 Analysis of general combining ability for Fusarium verticillioides ear rot
The results for the combining ability of the 13 parents for FER severity are presented in Table 4. Based on GCA effects, the best inbred parents for producing hybrids with resistance to FER were those with negative GCA. Male inbred lines JPS26-125, JPS26-86 and JPS25-13, with JPS26-125 having the most negative (-0.98) GCA while JPS25-14 had the most positive effect of 0.61. Among the female inbred lines, JPS25-11 exhibited the most negative GCA effect (-0.75), indicating its strong contribution to resistance to Fusarium Ear Rot (Table 4).
3.4 Analysis of specific combining ability Fusarium verticillioides ear rot
The results of the SCA of the crosses are presented in Table 5. The Specific Combining Ability effects for FER were significant at P < 0.05, whereas the SCA x environment interaction was not significant across the five locations. Out of the 42 hybrids, 21 had negative SCA values but only three were significant, these being JPS25-11//JPS25-13, JPS25-12//JPS25-22 and JPS25-11//JPS26-4, thus considered the best combinations. Hybrid JPS25-12//JPS25-22 had the most negative (-1.97) SCA effects for F. verticillioides ear rot across the environments and it was significant, whereas the highest positive value was found for hybrid JPS 25-9/JPS26-86.
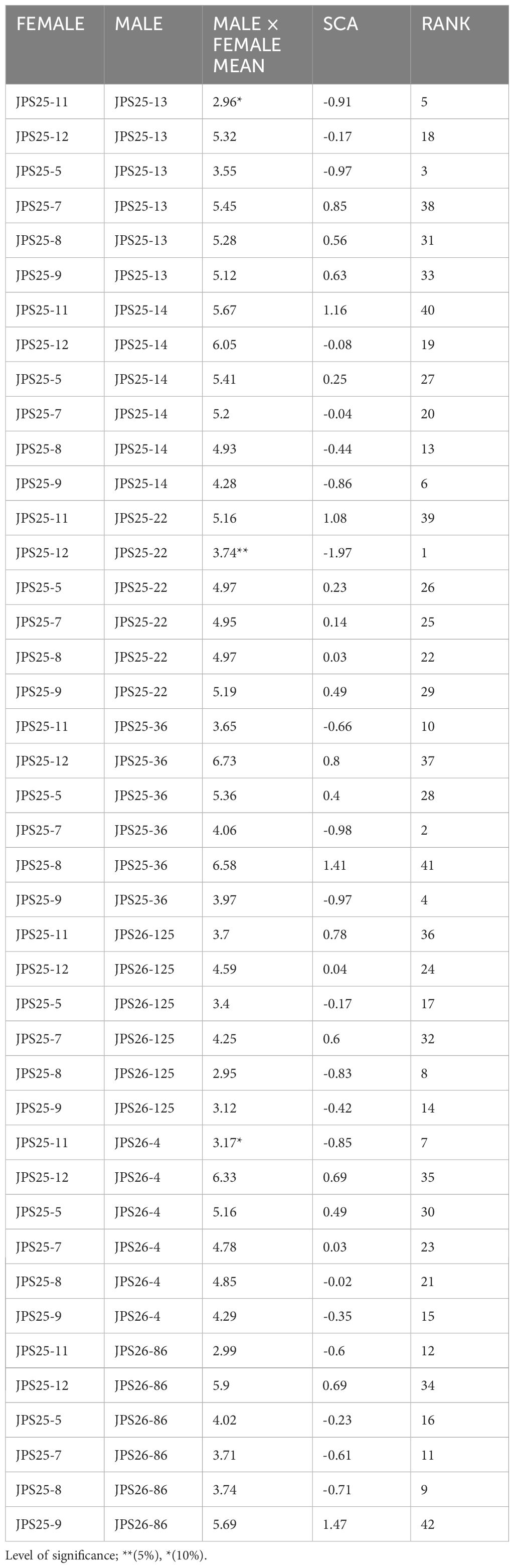
Table 5. Estimates of specific combining ability effects for FER scores of 13 inbred lines across the environments.
4 Discussion
It is important to note that the study involved a small sample of seven males and six females selected for their FER resistance. As such, the conclusions drawn here pertain specifically to the genotypes evaluated and should not be generalized to the broader maize germplasm, including Ugandan maize populations. Never the less, improving maize yield is a key priority for breeders to meet the rising global demand, especially by managing diseases like FER. Breeding for resistance is the most effective strategy for controlling FER, particularly for smallholder farmers who primarily grow maize for subsistence and often lack the resources to implement other control measures (Chen et al., 2016). However, to optimize this approach, it is crucial to identify stable and effective sources of resistance and understand their performance across different environments. This is especially important since FER resistance is a complex trait, controlled by many genes with minor individual effects, exhibiting low to moderate heritability, and highly influenced by environmental factors.
In this study, significant variation was observed among the hybrids (P < 0.001), indicating substantial genetic diversity that could facilitate selection progress for FER resistance under artificial inoculation. This variation is attributed to the hybrids’ differing responses to the fungus and the distinct resistance mechanisms employed (Hung and Holland, 2012). The presence of significant variation among inbred lines and their hybrids for Fusarium verticillioides infection offers an opportunity for genetic improvement in local breeding programs (Clements et al., 2004; Tembo et al., 2022). Moreover, the significant genotype-by-environment interactions (P < 0.1) suggest that the hybrids performed differently across the study locations, highlighting the role of environmental factors in their differential responses (Tembo et al., 2022). This underscores the importance of testing hybrids across multiple environments to select those suited to specific production areas or with broad adaptation potential (Mugisa et al., 2022). Similar findings were reported by Ouko et al. (2020); Tembo et al. (2022), and Netshifhefhe et al. (2018). The observed genotype × environment interactions in this study could be influenced by unmeasured traits such as cycle length, which has been correlated with rotting disease resistance in previous studies (Agrama and Moussa, 1996; Zwonitzer et al., 2010; Chabanne et al., 2020). Incorporating this trait in future evaluations could provide a more comprehensive understanding of FER resistance.
Partitioning the general combining ability (GCA) into male and female components revealed highly significant GCA effects, indicating that additive genetic effects from both male and female parents are crucial. The specific combining ability (SCA) was marginally significant (P < 0.05), suggesting that non-additive genetic effects also play a role, albeit making a smaller contribution. Similar findings were reported by various studies using different materials and in different environments among them; Tembo et al. (2022) and Ouko et al. (2020).
In this study, negative combining ability effects were desirable, as they indicate a line’s contribution to resistance, while positive combining ability effects suggest susceptibility (Mukanga et al., 2010; Tembo et al., 2022). Based on GCA effects, the best inbred parents for producing FER-resistant hybrids were JPS25-13, JPS26-125, JPS26-86, JPS25-11, JPS25-5, JPS25-7, and JPS25-9. Incorporating these resistant lines into breeding programs could strengthen resistance to FER in future hybrids (Ouko et al., 2020). These lines could be used in recurrent selection programs or as testers in hybrid combinations. The significant contribution of lines with high GCA effects highlights the predominance of additive gene action in conferring resistance to FER. Although specific hybrids displayed significant SCA effects, these were limited and represented exceptions rather than the majority of the tested hybrids. The hybrids JPS25-12/JPS25-22, JPS25-11//JPS26-4, and JPS25-11/JPS25-13 stand out for their significant negative SCA effects, showing strong non-additive contributions to resistance. These crosses could serve as models for generating high-performing hybrids in different environments.
The environment significantly influenced the observed variation in fungal colonization, as shown in Table 2, with differing conditions favoring the spread of F. verticillioides. This was evident in the differences in the hybrids’ responses between the five environments. For example, hybrid JPSH-13 exhibited contrasting responses, being susceptible in environments E1, E4, and E5, but resistant in E2 and E3, a pattern seen in several hybrids environment E4 (Buginyanya) had the highest infection scores, likely due to high relative humidity and rainfall, which promote the fungus’s growth and spread. The same result was also observed by Ouko et al. (2020). The significant GCA × environment interactions in this study suggest that selecting parental lines tailored to specific environments could enhance resistance (Mukanga et al., 2010). A visual representation in Figure 1 of pairwise comparisons for Ear Rot among the different environments highlights significant differences in mean values. Notably, the comparison between E2 and E1 shows a highly significant negative difference, indicating that E2 has a considerably lower mean Ear Rot than E1. Similarly, E3 exhibits a significantly higher mean Ear Rot compared to E2, as evidenced by the positive difference and non-overlapping confidence intervals. Other comparisons, such as E5-E1 and E5-E3, reveal significant differences, with E5 generally exhibiting lower mean values. In contrast, some comparisons, like E3-E1 and E4-E1, show no statistical significance, as their confidence intervals include zero, suggesting similar performance between these environments. Overall, the results demonstrate notable variability in Ear Rot severity across environments, with certain environments performing significantly better or worse than others. These findings underscore the importance of environment-specific factors in influencing Ear Rot levels.
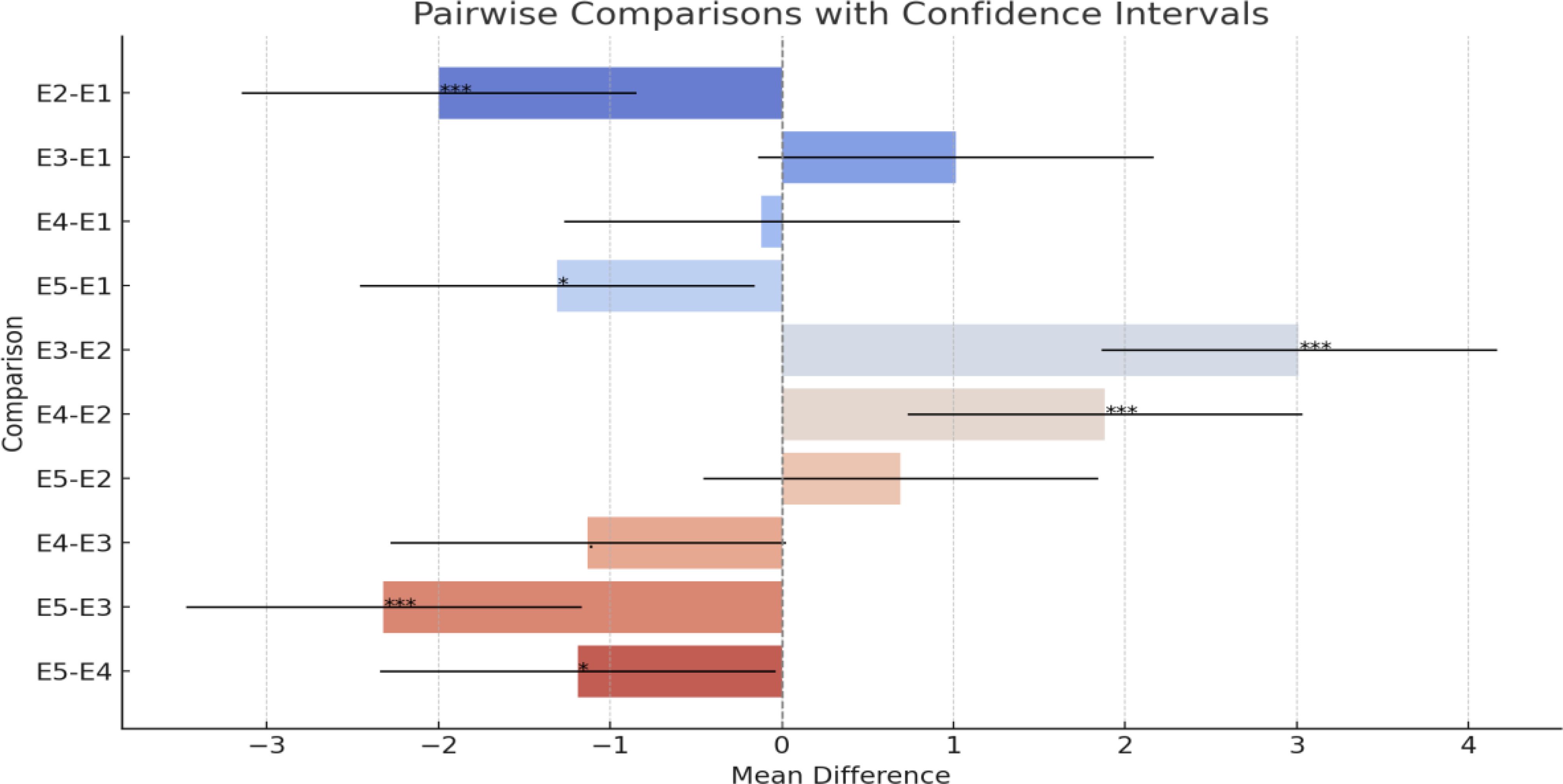
Figure 1. Representation of the pairwise comparisons with their confidence intervals: • The bars represent the mean differences between environments. • The horizontal lines extending from each bar indicate the confidence intervals (95%). • The dashed vertical line at 0 highlights where no difference exists. • The annotations next to the bars indicate the significance levels (***, *,., or none).
The broad-sense heritability in this study was relatively high (0.73), indicating that a large portion of the phenotypic variance was attributable to genetic factors such as additive, dominance, and epistatic effects. This suggests that over 70% of the observed phenotypic variance in the hybrids was inherited from the parents, reflecting similarities between the inbred lines and their hybrids (Netshifhefhe et al., 2018). The high heritability estimates imply that resistance to FER can be effectively achieved by crossing two parental inbred lines with favorable GCA effects (i.e., negative GCA for disease resistance). The strong heritability of resistance to Fusarium verticillioides suggests that resistance levels in the hybrids closely resemble those of their parent inbred lines (Netshifhefhe et al., 2018), making phenotypic selection for improved resistance to FER a viable strategy. This information can therefore guide breeding programs in developing maize hybrids with enhanced resistance to FER. The Baker’s ratio of 0.67 indicates that additive genetic effects, as captured by the GCA, play a more significant role than non-additive effects (SCA) in the inheritance of resistance to FER. This aligns with the ANOVA results, which show highly significant GCA effects for both male and female parents, and these results are consistent with findings by Rose et al. (2016) and Hung and Holland (2012).
The findings of this study are useful to aid breeders in selecting maize lines with resistance to mycotoxigenic fungi for the development of hybrids with improved tolerance to mycotoxin contamination in Uganda and other tropical countries. Additive gene effects were important in conferring resistance to and ear rot. Furthermore, hybrids with improved resistance to F. verticillioides infection were generated, and parental lines served as a good indicator of a hybrid’s performance to infection. Although this study focused on FER resistance, yield is a critical factor for farmer adoption. Future evaluations of these hybrids should prioritize identifying combinations that balance resistance with high productivity, ensuring both economic viability and disease control. Future research should incorporate the evaluation of cycle length and yield potential to ensure the development of hybrids that combine Fusarium Ear Rot resistance with superior agronomic performance.
Data availability statement
The original contributions presented in the study are included in the article/Supplementary Material. Further inquiries can be directed to the corresponding author.
Author contributions
SBA: Data curation, Formal analysis, Investigation, Methodology, Software, Validation, Visualization, Writing – original draft, Writing – review & editing. JPS: Conceptualization, Data curation, Formal analysis, Writing – original draft, Investigation, Software, Validation, Project administration, Resources. EAA: Formal analysis, Software, Writing – original draft, Writing – review & editing. JBS: Investigation, Writing – original draft, Writing – review & editing. IOD: Writing – original draft, Supervision, Validation, Writing – review & editing. PR: Writing – original draft, Writing – review & editing. BMO: Writing – original draft, Writing – review & editing.
Funding
The author(s) declare financial support was received for the research, authorship, and/or publication of this article. The research was funded by USAID FtF Uganda Agriculture Research Activity (Agreement number CGIAR fund 2AID-BFS-10-17-00005) and the Bill and Melinda Gates Foundation (grant number OPP1093174).
Acknowledgments
The authors would also like to thank CIMMYT and NARO for providing germplasm for the study.
Conflict of interest
The authors declare that the research was conducted in the absence of any commercial or financial relationships that could be construed as a potential conflict of interest.
Generative AI statement
The author(s) declare that no Generative AI was used in the creation of this manuscript.
Publisher’s note
All claims expressed in this article are solely those of the authors and do not necessarily represent those of their affiliated organizations, or those of the publisher, the editors and the reviewers. Any product that may be evaluated in this article, or claim that may be made by its manufacturer, is not guaranteed or endorsed by the publisher.
Supplementary material
The Supplementary Material for this article can be found online at: https://www.frontiersin.org/articles/10.3389/fpls.2025.1509859/full#supplementary-material
Supplementary Figure 1 | FER scores 1-9 as scored in the field at harvest.
References
Afolabi, C. G., Ojiambo, P. S., Ekpo, E. J. A., Menkir, A., Bandyopadhyay, R. (2007). Evaluation of maize inbred lines for resistance to fusarium ear rot and fumonisin accumulation in grain in tropical Africa. Plant Dis. 91, 279–286. doi: 10.1094/PDIS-91-3-0279
Agrama, H. A., Moussa, M. E. M. (1996). Mapping QTLs for resistance to stalk rot diseases and grain yield in maize. Euphytica 91, 89–97. doi: 10.1007/BF00035278
Atukwase, A., Kaaya, A. N., Muyanja, C. (2009). Factors associated with fumonisin contamination of maize in Uganda. J. Sci. Food Agri. 89 (14), 2393–2398. doi: 10.1002/jsfa.3734
Atukwase, A., Muyanja, C., Kaaya, A. N. (2012). Potential for Fumonisin Production by the Strains of Gibberella fujikuroi Species Complex Isolated from Maize Produced in Uganda. J. Biol. Sci. 12 (4), 225–231. DOI: 10.3923/jbs.2012.225.231
Ayesiga, B. S., Rubaihayo, P., Dramadri, O. I., Edema, R., Sserumaga, P. J. (2022). Evaluation of tropical maize inbred lines for resistance to Fusarium Ear Rot. Afr. J. Rural Dev. 7, 176–180.
Ayesiga, S. B., Rubaihayo, P., Oloka, B. M., Dramadri, I. O., Sserumaga, J. P. (2023). Genome-wide association study and pathway analysis to decipher loci associated with Fusarium Ear Rot resistance in tropical maize germplasm. Genet. Resour. Crop Evol 71:2435–2448. doi: 10.1007/s10722-023-01793-4
Badu-Apraku, B., Fakorede, M. A. B. (2017). “Maize in Sub-Saharan Africa: Importance and Production Constraints,” in Advances in Genetic Enhancement of Early and Extra-Early Maize for Sub-Saharan Africa (Springer, Cham: Springer International Publishing), 3–10. doi: 10.1007/978-3-319-64852-1_1
Baker, R. J. (1978). Issues in diallel analysis. Crop Sci. 18, 533–536. doi: 10.2135/cropsci1978.0011183X001800040001x
Balconi, C., Berardo, N., Locatelli, S., Lanzanova, C., Torri, A., Redaelli, R. (2014). Evaluation of ear rot (Fusarium verticillioides) resistance and fumonisin accumulation in Italian maize inbred lines. Phytopathol. Mediterr. 53, 14–26. doi: 10.14601/Phytopathol_Mediterr-11776
Butrón, A., Reid, L. M., Santiago, R., Cao, A., Malvar, R. A. (2015). Inheritance of maize resistance to gibberella and Fusarium Ear Rots and kernel contamination with deoxynivalenol and fumonisins. Plant Pathol. 64, 1053–1060. doi: 10.1111/ppa.12351
Cairns, J. E., Prasanna, B. (2018). Developing and deploying climate-resilient maize varieties in the developing world. Curr. Opin. Plant Biol. 45, 226–230. doi: 10.1016/j.pbi.2018.05.004
Chabanne, A., Cadic, A., Lecomte, M., Grondin, M., Caruana, M. L. (2020). Cycle length and disease resistance relationships in diverse crop species: A focus on root and tuber crops. Plant Pathol. 69, 845–857.
Chen, J., Ding, J., Li, H., Li, Z., Sun, X., Li, J., et al. (2012). Detection and verification of quantitative trait loci for resistance to Fusarium Ear Rot in maize. Mol. Breed. 30, 1649–1656. doi: 10.1007/s11032-012-9748-1
Chen, J., Shrestha, R., Ding, J., Zheng, H., Mu, C., Wu, J., Mahuku, G. (2016). Genome-Wide Association Study and QTL Mapping Reveal Genomic Loci Associated with Fusarium Ear Rot Resistance in Tropical Maize Germplasm. G3 Genes Genomes Genetics 6 (12), 3803–3815. doi: 10.1534/g3.116.034561
Clements, M. J., Maragos, C. M., Pataky, J. K., White, D. G. (2004). Sources of resistance to fumonisin accumulation in grain and fusarium ear and kernel rot of corn. Phytopathology® 94, 251–260. doi: 10.1094/PHYTO.2004.94.3.251
Comstock, R. E., Robinson, H. F. (1952). “Estimation of average dominance of genes,” in Heterosis (Iowa State College Press, Ames), 494–516.
de Jong, G., Pamplona, A. K. A., Von Pinho, R. G., Balestre, M. (2018). Genome-wide association analysis of ear rot resistance caused by Fusarium verticillioides in maize. Genomics 110, 291–303. doi: 10.1016/j.ygeno.2017.12.001
De Mendiburu, F. (2021). agricolae: Statistical Procedures for Agricultural Research. R package version 1.3-5. Available online at: https://cran.r-project.org/package=agricolae (Accessed March 28, 2023).
Ding, J.-Q., Wang, X.-M., Chander, S., Yan, J.-B., Li, J.-S. (2008). QTL mapping of resistance to Fusarium Ear Rot using a RIL population in maize. Mol. Breed. 22, 395–403. doi: 10.1007/s11032-008-9184-4
Eller, M. S., Holland, J. B., Payne, G. A. (2008). Breeding for improved resistance to fumonisin contamination in maize. Toxin Rev. 27, 371–389. doi: 10.1080/15569540802450326
Epule, T. E., Dhiba, D., Etongo, D., Peng, C., Lepage, L. (2021). Identifying maize yield and precipitation gaps in Uganda. SN Appl. Sci. 3, 537. doi: 10.1007/s42452-021-04532-5
Falconer, D. S., Mackay, T. F. C. (1996). Introduction to Quantitative Genetics. 4th ed (Harlow: Addison Wesley Longman).
Hung, H., Holland, J. B. (2012). Diallel analysis of resistance to fusarium ear rot and fumonisin contamination in maize. Crop Sci. 52, 2173–2181. doi: 10.2135/cropsci2012.03.0154
Ju, M., Zhou, Z., Mu, C., Zhang, X., Gao, J., Liang, Y., et al. (2017). Dissecting the genetic architecture of Fusarium verticillioides seed rot resistance in maize by combining QTL mapping and genome-wide association analysis. Sci. Rep. 7, 46446. doi: 10.1038/srep46446
Lanubile, A., Maschietto, V., Borrelli, V. M., Stagnati, L., Logrieco, A. F., Marocco, A. (2017). Molecular basis of resistance to fusarium ear rot in maize. Front. Plant Sci. 8. doi: 10.3389/fpls.2017.01774
Lanubile, A., Pasini, L., Lo Pinto, M., Battilani, P., Prandini, A., Marocco, A. (2011). Evaluation of broad spectrum sources of resistance to Fusarium verticillioides and advanced maize breeding lines. World Mycot. J. 4, 43–51. doi: 10.3920/WMJ2010.1206
Logrieco, A., Mulè, G., Moretti, A., Bottalico, A. (2002). Toxigenic Fusarium species and mycotoxins associated with maize ear rot in Europe. Eur. J. Plant Pathol. 108, 597–609. doi: 10.1023/A:1020679029993
Magarini, A., Colombo, F., Cassani, E., Ghidoli, M., Landoni, M., Sangiorgio, S., et al. (2024). The role of husk traits in maize susceptibility to Fusarium verticillioides : A multi-location study in northern Italy. Food Energy Secur. 13. doi: 10.1002/fes3.537
Maschietto, V., Marocco, A., Malachova, A., Lanubile, A. (2015). Resistance to Fusarium verticillioides and fumonisin accumulation in maize inbred lines involves an earlier and enhanced expression of lipoxygenase (LOX) genes. J. Plant Physiol. 188, 9–18. doi: 10.1016/j.jplph.2015.09.003
Ma Teresa, L., Gerpcio-Sta, C., Enrique, C. (1994). Heritability estimates of some root characters in sweetpotato. Philipp. J. CropSci. 19, 27–32.
Mesterházy, Á., Lemmens, M., Reid, L. M. (2012). Breeding for resistance to ear rots caused by Fusarium spp. in maize - a review. Plant Breed. 131, 1–19. doi: 10.1111/j.1439-0523.2011.01936.x
Mugisa, I., Karungi, J., Musana, P., Odama, R., Alajo, A., Chelangat, D. M., et al. (2022). Combining ability and heritability analysis of sweetpotato weevil resistance, root yield, and dry matter content in sweetpotato. Front. Plant Sci. 13. doi: 10.3389/fpls.2022.956936
Mukanga, M., Derera, J., Tongoona, P. (2010). Gene action and reciprocal effects for ear rot resistance in crosses derived from five tropical maize populations. Euphytica 174, 293–301. doi: 10.1007/s10681-010-0178-z
Netshifhefhe, N. E. I., Flett, B. C., Viljoen, A., Rose, L. J. (2018). Inheritance and genotype by environment analyses of resistance to Fusarium verticillioides and fumonisin contamination in maize F1 hybrids. Euphytica 214, 235. doi: 10.1007/s10681-018-2310-4
Niyibituronsa, M., Usabyembabazi, M., Nkundanyirazo, E. (2020). Assessment of aflatoxin and fumonisin contamination levels in maize and mycotoxins awareness and risk factors in Rwanda. African J. Food, Agri. Nutrit. Develop. 20 (05), 16420–16446. doi: 10.18697/ajfand.93.19460
Ouko, A., Okoth, S., Netshifhefhe, N. E., Viljoen, A., Rose, L. J. (2020). Tolerance to Fusarium verticillioides infection and fumonisin accumulation in maize F1 hybrids and subsequent F 2 populations. Agron. J. 112, 2432–2444. doi: 10.1002/agj2.20145
R Core Team. (2021). R: A language and environment for statistical computing. R Foundation for Statistical Computing, Vienna, Austria. Available online at: https://www.R-project.org/.
Reid, L. M., Zhu, X., Parker, A., Yan, W. (2009). Increased resistance to Ustilago zeae and Fusarium verticilliodes in maize inbred lines bred for Fusarium graminearum resistance. Euphytica 165, 567. doi: 10.1007/s10681-008-9782-6
Rodríguez, F., Alvarado, G., Pacheco, A., Burgueño, J., Crossa, J. (2015). Analysis of genetic designs with R for Windows (AGD-R for Windows Software version 2.0). Mexico City: CIMMYT.
Rose, L. J., Mouton, M., Beukes, I., Flett, B. C., van der Vyver, C., Viljoen, A. (2016). Multi-environment evaluation of maize inbred lines for resistance to fusarium ear rot and fumonisins. Plant Dis. 100, 2134–2144. doi: 10.1094/PDIS-11-15-1360-RE
Stagnati, L., Lanubile, A., Samayoa, L. F., Bragalanti, M., Giorni, P., Busconi, M., et al. (2019). A genome wide association study reveals markers and genes associated with resistance to fusarium verticillioides infection of seedlings in a maize diversity panel. G3 Genes|Genomes|Genetics 9, 571–579. doi: 10.1534/g3.118.200916
Tembo, E., Minnaar-Ontong, A., Menkir, A., Marais, G., Magorokosho, C., Labuschagne, M. T. (2022). Inheritance of resistance to Fusarium verticillioides ear rot in maize inbred lines of southern, West and Central Africa origin. Crop Sci. 62, 1818–1833. doi: 10.1002/csc2.20776
UBOS (2020). Uganda Bureau of statistics 2020 statistical abstract (Kampala, Uganda: Uganda Bureau of Statistics).
Warburton, M. L., Brooks, T. D., Krakowsky, M. D., Shan, X., Windham, G. L., Williams, W. P. (2009). Identification and mapping of new sources of resistance to aflatoxin accumulation in maize. Crop Sci. 49, 1403–1408. doi: 10.2135/cropsci2008.12.0696
Xu, Y., Zhang, Z., Lu, P., Li, R., Ma, P., Wu, J., et al. (2023). Increasing Fusarium verticillioides resistance in maize by genomics-assisted breeding: Methods, progress, and prospects. Crop J. 11, 1626–1641. doi: 10.1016/j.cj.2023.07.004
Yli-Mattila, T., Sundheim, L. (2022). Fumonisins in African Countries. Toxins 14 (6), 419. doi: 10.3390/toxins14060419
Zwonitzer, J. C., Coles, N. D., Krakowsky, M. D., Arellano, C., Holland, J. B., McMullen, M. D., et al. (2010). Mapping resistance quantitative trait loci for three foliar diseases in a maize recombinant inbred line population—evidence for clustered resistance loci. Theor. Appl. Genet. 121, 433–441.
Keywords: Zea mays, Fusarium, gene action, heritability, genetics
Citation: Ayesiga SB, Rubaihayo P, Sempiira JB, Adjei EA, Dramadri IO, Oloka BM and Sserumaga JP (2025) Combining ability and gene action for resistance to Fusarium ear rot in tropical maize hybrids. Front. Plant Sci. 16:1509859. doi: 10.3389/fpls.2025.1509859
Received: 11 October 2024; Accepted: 08 January 2025;
Published: 30 January 2025.
Edited by:
Ana Butron, Misión Biológica de Galicia, Spanish Council for Scientific Research (MBG-CSIC), SpainReviewed by:
Thomas Miedaner, University of Hohenheim, GermanyLorenzo Stagnati, Catholic University of the Sacred Heart, Piacenza, Italy
Copyright © 2025 Ayesiga, Rubaihayo, Sempiira, Adjei, Dramadri, Oloka and Sserumaga. This is an open-access article distributed under the terms of the Creative Commons Attribution License (CC BY). The use, distribution or reproduction in other forums is permitted, provided the original author(s) and the copyright owner(s) are credited and that the original publication in this journal is cited, in accordance with accepted academic practice. No use, distribution or reproduction is permitted which does not comply with these terms.
*Correspondence: Julius Pyton Sserumaga, ai5zZXJ1bWFnYUBnbWFpbC5jb20=